- 1Department of Mechanical Engineering, The Hong Kong Polytechnic University, Hong Kong, China
- 2The Hong Kong Polytechnic University Shenzhen Research Institute, Shenzhen, China
- 3Department of Materials Science and Engineering, Harbin Institute of Technology (Shenzhen), Shenzhen, China
- 4Department of Materials Science and Engineering, City University of Hong Kong, Hong Kong, China
- 5State Key Laboratory for Advanced Metals and Materials, University of Science and Technology Beijing, Beijing, China
Advanced structural materials with superior mechanical properties are of technological importance for industrial applications. Multicomponent precipitation provides a potential approach for designing high-performance alloys and has been receiving increasing attention from both academia and industry. In this Perspective, we highlight the recent advances and future perspectives in multicomponent-precipitation-strengthened alloys, including multicomponent steels, superalloys, and high-entropy alloys. The emphasis is placed on the unique microstructural features and challenges of multicomponent precipitation as well as their impacts on the strengthening behavior. Finally, future research areas for this class of materials are critically discussed.
Introduction
Metallic materials with excellent mechanical properties are highly desirable for a wide range of engineering applications, such as aerospace, transportation, construction, marine, and energy industries (Reed, 2008; Fine et al., 2010; Yen et al., 2011; Kapoor et al., 2014; Miracle and Senkov, 2017; George et al., 2019). It is commonly understood that the mechanical properties of crystalline materials are determined by the microstructure and dislocation interactions. Basically, strengthening crystalline materials involves the controlled creation of internal defects to impede the motion of dislocations. Intermetallic precipitation strengthening has been extensively used in many metallic systems, such as steels and superalloys (Raabe et al., 2009; Mulholland and Seidman, 2011; He et al., 2016; Bleck et al., 2017; Sun et al., 2017; Liang et al., 2018; Yang et al., 2018). The degree of precipitation strengthening is highly dependent on the microstructure and micro-mechanical properties of precipitates as well as the nature of the dislocation-precipitate interaction. Recent studies reveal that intermetallic precipitates in multicomponent metallic systems are often of multicomponent type, the formation of which involves complicated elemental partitioning and sublattice occupancies. The complex compositions and lattice structures can significantly influence their nucleation, growth, and coarsening behaviors, thereby affecting the precipitate microstructure and strengthening behavior (Jiang et al., 2015; Trotter et al., 2016; Song et al., 2017; Zhao et al., 2018). For example, B2-ordered Ni(Al,Mn)-type precipitates can form in Fe-Ni-Al-Mn multicomponent alloys, in which Mn partitions to the precipitates and occupies the Al sublattice (Zhang et al., 2013). The Mn partitioning significantly reduces the critical energy for nucleation and increases the number density of precipitates by more than one order of magnitude, leading to a doubled strengthening effect. In fact, the beneficial effects of multicomponent precipitates on the thermal, physical, and mechanical properties of materials are frequently observed in many precipitation-strengthened systems. As a result, the development of multicomponent-precipitation-strengthened alloys has attracted extensive and increasing interest from both academia and industry. In this Perspective, we highlight the recent development of multicomponent-precipitation-strengthened alloys and critically discuss their unique features, challenges, and future visions, as schematically illustrated in Figure 1. In particular, we focus on the complicated elemental partitioning and sublattice occupancies in multicomponent steels, superalloys, and high-entropy alloys. The unique microstructural features bring new challenges for understanding and control of the precipitation and strengthening behaviors. To further accelerate the advancement of the multicomponent-precipitation-strengthened alloys, fundamental and technological areas should be further explored in future studies, such as computation-aided alloy design, atomic structure, intrinsic characteristics, and overall properties of these materials.
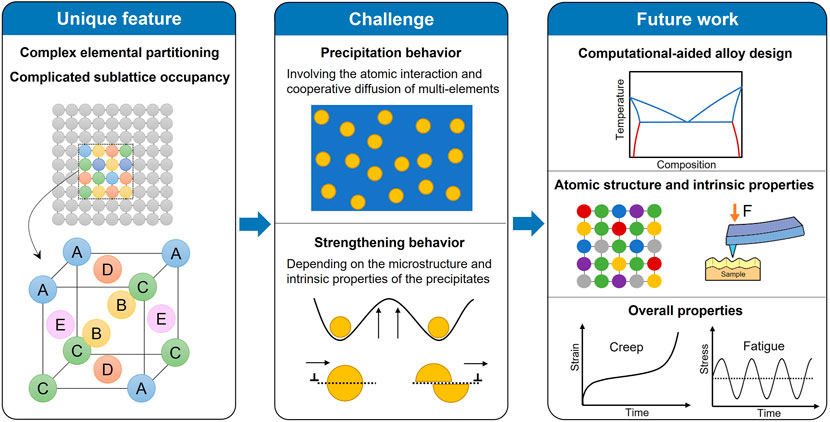
FIGURE 1. Schematic summarizing the unique features, challenges, and future work of multicomponent-precipitation-strengthened alloys.
Multicomponent Precipitates in Intermetallic-Strengthened Alloys
Multicomponent Precipitates in Steels
Fe-based steels undergo a phase transformation from a face-centered cubic (fcc) austenite phase at high temperatures to a body-centered cubic (bcc) structure at low temperatures. Some fcc elements, such as Ni, Al, and Cu, can be dissolved to a considerable extent in the fcc austenite phase but have a low solubility in the bcc structure, which results in the precipitation of fcc elements at low temperatures. For example, B2-ordered NiAl particles can precipitate coherently from the ferritic/martensitic matrix, and their compositions often deviate from the stoichiometric composition of binary NiAl, especially at an early stage of precipitation (Teng et al., 2012; Kim et al., 2015; Jiang et al., 2017; Song et al., 2017). Depending on the alloy composition and processing conditions, Fe can partition to the B2-ordered precipitates by occupying the Ni or Al sublattice, leading to the formation of (Ni,Fe)Al or Ni(Al,Fe) precipitates. In addition, Mn can also partition to NiAl-type precipitates by replacing parts of Al, resulting in the formation of Ni(Al,Mn) precipitates (Jiao et al., 2015). In terms of mechanical properties, the Ni(Al,Mn) precipitates increase the yield strength of a Fe–5Ni–1Al–3Mn (wt%) steel from 685 MPa in the as-quenched condition to 1225 MPa in the peak-aged condition, which is almost a double increase; meanwhile, the ductility decreases slightly from 16% in the as-quenched condition to 14% in the peak-aged condition (Jiao et al., 2015). Another example is the Ni3Ti-type precipitation in maraging steels. In the initial stages of precipitation, Mo partitions to the Ni3Ti-type precipitates by occupying the Ti sublattice, which leads to the formation of high densities of Ni3(Ti,Mo) precipitates (Niu et al., 2021). These multicomponent precipitates provide effective strengthening responses, leading to the development of advanced ultrahigh-strength steels with 2 GPa strength and >10% ductility.
Multicomponent Precipitates in Superalloys
Ni-based superalloys are known to have a complex composition, with more than ten alloying elements in most alloys. They are key structural materials for high-temperature applications in aerospace, particularly the turbine blades of jet engines (Pope and Ezz, 1984; Pollock and Tin, 2006; Reed, 2008). Precipitation-strengthened superalloys have a unique γ/γ′ microstructure, in which ordered γ′ precipitates are distributed in the γ matrix. The γ phase is an fcc solid solution with randomly distributed different species of atoms, whereas the γ′ phase is a L12-ordered Ni3Al-type intermetallic compound. Generally, the Ni sublattice is comprised of mostly electronegative elements, such as Ni and Co., whereas the Al sublattice constitutes more electropositive elements, such as Al, Ti, Nb, and Ta (Reed, 2008). Generally, dislocations move into the L12-ordered γ′ phase by forming antiphase boundaries, whereas dislocations travel across the γ matrix by forming stacking faults. The antiphase boundary energy of the γ′ phase is higher than the intrinsic stacking fault energy of the γ phase, which thus hinders the motion of dislocations in the γ phase. The high volume fractions of coherent γ′ precipitates with high strength and thermal stability enable the γ/γ′ superalloys to achieve superior mechanical properties at high temperatures (up to 0.9 Tm) (Reed, 2008). Similar precipitation behaviors are also observed in Co-based γ/γ′ superalloys, in which the γ′ phase is the L12-ordered Co3Al-type intermetallic compound. The binary Co3Al phase is known to be metastable at high temperatures, but which can be stabilized by alloying elements, such as Cr, V, Nb, Ta, and W. In the recent decades, a series of novel Co-based superalloys strengthened by stable γ′ precipitates, such as Co3(Al,W), Co3(Al,Cr,Ta), and Co3(Al,Ti,V,Nb), have been developed (Sato et al., 2006; Oohashi et al., 2008; Xue et al., 2013; Suzuki et al., 2015). These materials show promising mechanical properties as potential candidates for high-temperature applications.
Multicomponent Precipitates in High-Entropy Alloys
High-entropy alloys, also known as multiple-principal-element alloys, have emerged as a new class of metallic materials because of their unique microstructure and excellent mechanical properties at cryogenic temperatures (Gludovatz et al., 2014). Among these alloys, the face-centered cubic (FCC) alloys, such as CoCrFeMnNi and CoCrNi, have received considerable attention because of their exceptional ductility and toughness, even down to the liquid nitrogen temperature. The yield strength of these FCC high-entropy alloys are generally in the range of 200–500 MPa (Wu et al., 2014). Recent studies reveal that intermetallic precipitation is effective in improving the mechanical strength of high-entropy alloys without causing a significant reduction in ductility (Zhang et al., 2014; Wang et al., 2018). Relating to the multicomponent nature of high-entropy alloys, the intermetallic precipitates are also complex in composition and sublattice occupancy. For instance, L12-ordered (Ni,Co)3(Al,Ti) precipitates can coherently form in the Fe-Co-Ni-Cr-Al-Ti system, which leads to the development of precipitation-strengthened high-entropy alloys with gigapascal strengths (Daoud et al., 2015; He et al., 2016; Liu et al., 2018; Zhao et al., 2018). Recently, even more complicated L12-ordered precipitates with a composition of 43.3Ni-23.7Co-10.0Fe-14.4Ti-8.6Al (at%) are formed in Fe-Co-Ni-Al-Ti alloys (Yang et al., 2018). The sublattice occupancy in the multicomponent precipitates was investigated by atomistic modelling, and the results indicate that these L12-type multicomponent precipitates can be regarded as the (Ni43.3Co23.7Fe8)3(Ti14.4Al8.6Fe2) phase (Yang et al., 2018). In addition, multicomponent B2-(Ni,Co)(Al,Cr,Fe) precipitates strengthened high-entropy alloys are developed, which exhibit outstanding fatigue resistance (Feng et al., 2021). This research area is still immature and may lead to new exciting developments.
Discussion on the Multicomponent Precipitation and Strengthening Behaviors
Precipitation Behavior
As the formation of multicomponent precipitates involves the atomic interaction and cooperative diffusion of various species of elements, their precipitation behavior can be significantly different from that of compositionally simple precipitates, especially in the nucleation stage. According to the classical nucleation theory, the critical energy for nucleation depends on the interplay among the chemical driving force, interfacial energy, and strain energy. Any factors affecting the chemical driving force, interfacial energy, and/or strain energy can have an impact on the nucleation behavior. An accelerated or decelerated nucleation behavior is often observed in multicomponent-precipitation-strengthened alloys. For example, Fe partitions to B2 precipitates in maraging steels, leading to the formation of Ni(Al,Fe)-type precipitates. The lattice misfit between Ni(Al,Fe) and bcc-Fe is much smaller than that between NiAl and bcc-Fe, which results in an extremely small strain energy between the precipitates and matrix, thereby substantially accelerating the NiAl nucleation (Jiang et al., 2017). When Cu is added to NiAl-strengthened steels, Cu partitions to NiAl precipitates in the early state of precipitation, which leads to the formation of B2-Ni(Al,Cu) precipitates. The incorporation of Cu into NiAl precipitates enhances the attractive interaction between Ni and Al, which substantially increases the chemical driving force for nucleation, thereby promoting the NiAl precipitation (Shen et al., 2017; Zhou et al., 2021). In addition, the coarsening behavior of multicomponent precipitates is also influenced by the precipitate composition and lattice structures. In high-entropy alloys, for example, the long-range diffusion is found to be sluggish because of the necessity for cooperative diffusion of solute atoms to maintain a proper elemental partitioning and sublattice occupancy. Resulting from sluggish diffusion, the precipitate coarsening rate in L12-(Ni,Co)3(Al,Ti)-strengthened high-entropy alloys is one to two orders of magnitude lower than that in traditional Ni-based superalloys (Zhao et al., 2018). Therefore, the compositional complexity of multicomponent precipitates can result in a considerable variation in the thermodynamic, structural, and physical properties, which provides a useful approach to tailoring the precipitate microstructure. Meanwhile, it brings new challenges for understanding and control of the precipitation behavior.
The processing method also has an important impact on the precipitation behavior of multicomponent alloys. Traditionally, metallic parts are manufactured by casting and forging processes, and the precipitate microstructure can be controlled through heat-treatments. There is now a growing interest in processing multicomponent alloys using new technologies for better material utilization, higher degree of design freedom, and/or better physical and mechanical properties. For example, the electron beam melting of CoCrFeNiMn-xTi results in the formation of multicomponent σ, γ, and η precipitates, the growth of which is limited at the boundaries of FCC columnar grains due to the rapid solidification nature of the process (Cagirici et al., 2021). The selective laser melting of a (FeCoNi)86Ti7Al7 alloy produces a heterogeneous precipitate microstructure consisting of L12 precipitates inside cellular structures and B2 precipitates along the cellular boundaries, which is quite different from the traditionally heat-treated microstructure with a uniform distribution of L12 nanoparticles (Huang et al., 2022). The sputtered Inconel 718 films generally show the precipitation of multicomponent δ-Ni3Nb precipitates, whereas in bulk 718 alloys metastable γ′′-Ni3Nb precipitates form first and transform to the stable δ-Ni3Nb phase after prolonged aging times (Burns et al., 2014). These observations suggest that different processing methods can result in different microstructures in terms of precipitate types, sizes, volume fractions, morphologies, and distributions. The new processing technologies provide new opportunities for tailoring the precipitate microstructure for improved properties. However, a clear understanding of the underlying precipitation mechanism and processing-microstructure correlation of these alloys remains elusive and needs further investigation.
Strengthening Behavior
The precipitation strengthening is based mainly on the interaction of dislocations with precipitates (Gladman, 1999). There are two types of dislocation-precipitate interaction depending on the precipitate size (inter-precipitate spacing). When the precipitate size is larger than a critical size, the Orowan bypassing mechanism is operative; when the precipitate size is smaller than the critical one, the dislocations cut through or shear the precipitates. In the precipitate shearing mechanism, three factors contribute to the strength increase, i.e., coherency strengthening, modulus strengthening, and order strengthening. From the strengthening models, the precipitation strengthening effect depends on not only the precipitate microstructure (precipitate size, volume fraction, and morphology) but also the intrinsic properties of the precipitates, such as lattice constant, shear modulus, Young’s modulus, and antiphase-boundary energy (Embury et al., 1971; Ardell, 1985). When modelling the strengthening of multicomponent precipitates, the values of shear modulus, Young’s modulus, and antiphase boundary energy are often borrowed from compositionally simple intermetallic compounds, such as binary Ni3Al and NiAl. However, it is known that a small change in composition and lattice occupancy can have a great impact on the intrinsic properties of intermetallic compounds. For example, Vittori et al. found that the antiphase-boundary energy of Ni3(Al,Ti) can be two times higher than that of binary Ni3Al (Vittori and Mignone, 1985). Kim et al., (2010) reported that the partial substitution of Ni by Cr can significantly reduce the shear modulus of Ni3Al. Thus, the intrinsic mechanical properties of multicomponent precipitates can be significantly different from those of stoichiometric precipitates, and the use of parameters of stoichiometric precipitates for strengthening modelling may produce large inaccuracies and even wrong conclusions. Therefore, for a better understanding of the strengthening mechanism of multicomponent precipitates, a quantitative study of the intrinsic mechanical properties of multicomponent precipitates is essential.
Outlook
Although multicomponent-precipitation-strengthened alloys are promising in terms of physical and mechanical properties, several fundamental and technological areas should be further explored in future studies.
Computation-Aided Alloy Design
Because of the complex interaction among various alloying elements, the classical “trial-and-error” method no longer suffices for the design of multicomponent-precipitation-strengthened alloys. Alternatively, computational calculations and genetic algorithms offer a potentially effective way for rapid alloy screening and design. For example, ab initio calculations can compute thermodynamic and structural properties for both stable and metastable phases, whereas thermodynamic calculations can provide useful information on phase equilibrium and transformations (Kresse and Hafner, 1993). For example, Kannan et al. developed a thermo-kinetic model to predict the precipitation and austenite reversion kinetics during various post-fabrication heat treatments in multicomponent steels (Kannan and Nandwana, 2021). In addition, machine learning can capture the highly-complex non-linear input/output relationships, to help filter or select the potential good materials rapidly (Zhao et al., 2021). These computational methods can serve to guide experimental efforts by effectively narrowing down the search space. Future work should place an emphasis on the integrated computational-prediction and experimental-validation approach for designing novel multicomponent-precipitation-strengthened alloys with desired properties.
Atomic Structures and Intrinsic Micro-Mechanical Properties
For a full understanding and control of the multicomponent precipitation behavior, the knowledge of the structure, chemistry, and energetics of multicomponent precipitates is necessary. The multicomponent precipitates are complex in terms of lattice occupancy, elemental partitioning, and interfacial segregation, and in some cases, the precipitates undergo phase transformations during the growth and coarsening processes. Future attention needs to be paid to the atomic-level characterization of multicomponent precipitates through advanced nano-analytical tools (such as aberration-corrected transmission electron microscopy and 3D atom probe tomography) (Seidman, 2007; Miller, 2012) and computational calculations (such as ab initio calculations) (Kresse and Hafner, 1993). In addition, as aforementioned, the strengthening effect of multicomponent precipitates is related to their micro-mechanical properties, such as Young’s modulus, shear modulus, and anti-phase boundary energy. The state-of-the-art peak-force tapping atomic force microscopy allows simultaneous capture of topography and maps of nano-mechanical properties, thus providing new opportunities to understand the micro-mechanical properties of multicomponent precipitates. Furthermore, atomistic modellings can play an important role in the understanding of intrinsic mechanical properties of multicomponent precipitates, such as elastic properties and energetics. Therefore, it is of fundamental importance to obtain detailed insights into the atomic structure and intrinsic mechanical properties of multicomponent precipitates through a combination of experimental and computational approaches.
Overall Properties
While most studies on multicomponent-precipitation-strengthened alloys focus on the general mechanical properties, such as strength, ductility, and strain hardening, the understanding of their overall properties, including processability, toughness, fatigue, creep, corrosion, and welding properties, remains limited. Particularly, alloys for industrial applications are generally used in various forms, such as sheets, plates, bars, tubes, and other structures. Thus, the processability of these materials is critical for manufacturing and industrial applications and needs to be carefully evaluated. In addition, creep resistance is critically important for applications that require long-term dimensional stability under load at elevated temperatures, whereas impact toughness is a key parameter in influencing the performance of engineering applications at low temperatures. Fatigue resistance is an essential property in preventing catastrophic failure of engineering components in service conditions. In order to develop advanced multicomponent-precipitation-strengthened alloys for industrial applications, future work needs to evaluate the overall properties of these materials under different conditions. In addition, it is also important to understand the fundamental mechanisms and key factors governing the overall properties of multicomponent-precipitation-strengthened alloys and to establish the relationship between the precipitate microstructure/properties and bulk alloys properties.
Concluding Remarks
This Perspective highlights the recent advances in the multicomponent precipitation and strengthening of intermetallic-strengthened alloys. The formation of multicomponent precipitates involves complicated elemental partitioning and sublattice occupancies, which results in a considerable variation in their structural, physical, and mechanical properties, thereby influencing the precipitation and strengthening behaviors. Understanding the composition–microstructure–property relationship is essential for both fundamental understanding and technological applications of multicomponent-precipitation-strengthened alloys. Future work should place on the computation-aided alloy design of advanced alloys with superior mechanical properties and the fundamental understanding of the atomic structure and micro/macro-mechanical properties of multicomponent-precipitation-strengthened alloys.
Data Availability Statement
The original contributions presented in the study are included in the article/Supplementary Material, further inquiries can be directed to the corresponding author.
Author Contributions
ZJ designed the research. JF, WL, TY, YW, and ZJ discussed and wrote the manuscript. All authors read and approved the final manuscript.
Funding
This research was supported by the National Natural Science Foundation of China (52171162 and 51801169), Research Grants Council of Hong Kong (25202719, 15227121, 21205621, C1017-21GF, and C1020-21GF), Shenzhen Science and Technology Program (JCYJ20210324142203009), State Key Laboratory for Advanced Metals and Materials Open Fund (2021-ZD04), Research Institute for Advanced Manufacturing at PolyU (P0041364), and the Guangdong Basic and Applied Basic Research Foundation (2020A1515110647).
Conflict of Interest
The authors declare that the research was conducted in the absence of any commercial or financial relationships that could be construed as a potential conflict of interest.
Publisher’s Note
All claims expressed in this article are solely those of the authors and do not necessarily represent those of their affiliated organizations, or those of the publisher, the editors and the reviewers. Any product that may be evaluated in this article, or claim that may be made by its manufacturer, is not guaranteed or endorsed by the publisher.
References
Ardell, A. J. (1985). Precipitation Hardening. Metall. Trans. A 16 (12), 2131–2165. doi:10.1007/bf02670416
Bleck, W., Song, W. W., and Zimmermann, A. (2017). “Intermetallic Phases in New Steels,” in Materials Science Forum (Zurich: Trans Tech Publications), 9–14.
Burns, D. E., Zhang, Y., Weihs, T. P., and Hemker, K. J. (2014). Properties of Sputter Deposited Ni-Base Superalloys for Microelectromechanical Systems. Thin Solid Films 558, 20–23. doi:10.1016/j.tsf.2014.02.051
Cagirici, M., Wang, P., Ng, F. L., Nai, M. L. S., Ding, J., and Wei, J. (2021). Additive Manufacturing of High-Entropy Alloys by Thermophysical Calculations and In Situ Alloying. J. Mater. Sci. Technol. 94, 53–66. doi:10.1016/j.jmst.2021.03.038
Daoud, H. M., Manzoni, A. M., Wanderka, N., and Glatzel, U. (2015). High-temperature Tensile Strength of Al10 Co25 Cr8 Fe15 Ni36 Ti6 Compositionally Complex Alloy (High-entropy Alloy). Jom 67 (10), 2271–2277. doi:10.1007/s11837-015-1484-7
Embury, J., Kelly, A., and Nicholson, R. (1971). Strengthening Methods in Crystals. Composites 2 (4), 252.
Feng, R., Rao, Y., Liu, C., Xie, X., Yu, D., Chen, Y., et al. (2021). Enhancing Fatigue Life by Ductile-Transformable Multicomponent B2 Precipitates in a High-Entropy Alloy. Nat. Commun. 12 (1), 1–10. doi:10.1038/s41467-021-23689-6
Fine, M. E., Vaynman, S., Isheim, D., Chung, Y.-W., Bhat, S. P., and Hahin, C. H. (2010). A New Paradigm for Designing High-Fracture-Energy Steels. Metall Mat Trans A 41 (13), 3318–3325. doi:10.1007/s11661-010-0485-y
George, E. P., Raabe, D., and Ritchie, R. O. (2019). High-entropy Alloys. Nat. Rev. Mater 4 (8), 515–534. doi:10.1038/s41578-019-0121-4
Gladman, T. (1999). Precipitation Hardening in Metals. Mater. Sci. Technol. 15 (1), 30–36. doi:10.1179/026708399773002782
Gludovatz, B., Hohenwarter, A., Catoor, D., Chang, E. H., George, E. P., and Ritchie, R. O. (2014). A Fracture-Resistant High-Entropy Alloy for Cryogenic Applications. Science 345 (6201), 1153–1158. doi:10.1126/science.1254581
He, J. Y., Wang, H., Huang, H. L., Xu, X. D., Chen, M. W., Wu, Y., et al. (2016). A Precipitation-Hardened High-Entropy Alloy with Outstanding Tensile Properties. Acta Mater. 102, 187–196. doi:10.1016/j.actamat.2015.08.076
Huang, J., Li, W., He, J., Zhou, R., Chou, T.-H., Yang, T., et al. (2022). Dual Heterogeneous Structure Facilitating an Excellent Strength-Ductility Combination in an Additively Manufactured Multi-Principal-Element Alloy. Mater. Res. Lett. 10 (9), 575–584. doi:10.1080/21663831.2022.2067790
Jiang, L., Cao, Z. Q., Jie, J. C., Zhang, J. J., Lu, Y. P., Wang, T. M., et al. (2015). Effect of Mo and Ni Elements on Microstructure Evolution and Mechanical Properties of the CoFeNixVMoy High Entropy Alloys. J. Alloys Compd. 649, 585–590. doi:10.1016/j.jallcom.2015.07.185
Jiang, S., Wang, H., Wu, Y., Liu, X., Chen, H., Yao, M., et al. (2017). Ultrastrong Steel via Minimal Lattice Misfit and High-Density Nanoprecipitation. Nature 544 (7651), 460–464. doi:10.1038/nature22032
Jiao, Z. B., Luan, J. H., Miller, M. K., Yu, C. Y., and Liu, C. T. (2015). Effects of Mn Partitioning on Nanoscale Precipitation and Mechanical Properties of Ferritic Steels Strengthened by NiAl Nanoparticles. Acta Mater. 84, 283–291. doi:10.1016/j.actamat.2014.10.065
Kannan, R., and Nandwana, P. (2021). Thermodynamics and kinetics of precipitation and austenite reversion during aging of Ti-free grade 300 maraging steel manufactured by laser powder bed fusion (LPBF). J. Mater. Sci. 56 (33), 18722–18739.
Kapoor, M., Isheim, D., Ghosh, G., Vaynman, S., Fine, M. E., and Chung, Y.-W. (2014). Aging Characteristics and Mechanical Properties of 1600MPa Body-Centered Cubic Cu and B2-NiAl Precipitation-Strengthened Ferritic Steel. Acta mater. 73, 56–74. doi:10.1016/j.actamat.2014.03.051
Kim, D. E., Shang, S. L., and Liu, Z. K. (2010). Effects of Alloying Elements on Elastic Properties of Ni3Al by First-Principles Calculations. Intermetallics 18 (6), 1163–1171. doi:10.1016/j.intermet.2010.02.024
Kim, S.-H., Kim, H., and Kim, N. J. (2015). Brittle Intermetallic Compound Makes Ultrastrong Low-Density Steel with Large Ductility. Nature 518 (7537), 77–79. doi:10.1038/nature14144
Kresse, G., and Hafner, J. (1993). Ab Initiomolecular Dynamics for Liquid Metals. Phys. Rev. B 47 (1), 558–561. doi:10.1103/physrevb.47.558
Liang, Y. J., Wang, L., Wen, Y., Cheng, B., Wu, Q., Cao, T., et al. (2018). High-content Ductile Coherent Nanoprecipitates Achieve Ultrastrong High-Entropy Alloys. Nat. Commun. 9 (1), 4063–4068. doi:10.1038/s41467-018-06600-8
Liu, W. H., Yang, T., and Liu, C. T. (2018). Precipitation Hardening in CoCrFeNi-Based High Entropy Alloys. Mater. Chem. Phys. 210, 2–11. doi:10.1016/j.matchemphys.2017.07.037
Miller, M. K. (2012). Atom Probe Tomography: Analysis at the Atomic Level. New York: Springer Science & Business Media.
Miracle, D. B., and Senkov, O. N. (2017). A Critical Review of High Entropy Alloys and Related Concepts. Acta Mater. 122, 448–511. doi:10.1016/j.actamat.2016.08.081
Mulholland, M. D., and Seidman, D. N. (2011). Nanoscale Co-precipitation and Mechanical Properties of a High-Strength Low-Carbon Steel. Acta Mater. 59 (5), 1881–1897. doi:10.1016/j.actamat.2010.11.054
Niu, M. C., Yin, L. C., Yang, K., Luan, J. H., Wang, W., and Jiao, Z. B. (2021). Synergistic Alloying Effects on Nanoscale Precipitation and Mechanical Properties of Ultrahigh-Strength Steels Strengthened by Ni3Ti, Mo-Enriched, and Cr-Rich Co-precipitates. Acta Mater. 209, 116788. doi:10.1016/j.actamat.2021.116788
Oohashi, T., Okamoto, N. L., Kishida, K., Tanaka, K., and Inui, H. (2008). Microstructures and Mechanical Properties of Co3 (Al, W) with the L12 Structure. MRS Online Proc. Libr. Opl. 1128, 526. doi:10.1557/proc-1128-u05-26
Pollock, T. M., and Tin, S. (2006). Nickel-based Superalloys for Advanced Turbine Engines: Chemistry, Microstructure and Properties. J. Propuls. power 22 (2), 361–374. doi:10.2514/1.18239
Pope, D. P., and Ezz, S. S. (1984). Mechanical Properties of Ni3AI and Nickel-Base Alloys with High Volume Fraction of γ'. Int. Met. Rev. 29 (1), 136–167. doi:10.1179/imtr.1984.29.1.136
Raabe, D., Ponge, D., Dmitrieva, O., and Sander, B. (2009). Nanoprecipitate-hardened 1.5 GPa Steels with Unexpected High Ductility. Scr. Mater. 60 (12), 1141–1144. doi:10.1016/j.scriptamat.2009.02.062
Reed, R. C. (2008). The Superalloys: Fundamentals and Applications. New York: Cambridge University Press.
Sato, J., Omori, T., Oikawa, K., Ohnuma, I., Kainuma, R., and Ishida, K. (2006). Cobalt-base High-Temperature Alloys. Science 312 (5770), 90–91. doi:10.1126/science.1121738
Seidman, D. N. (2007). Three-dimensional Atom-Probe Tomography: Advances and Applications. Annu. Rev. Mat. Res. 37, 127–158. doi:10.1146/annurev.matsci.37.052506.084200
Shen, Q., Chen, H., and Liu, W. (2017). Effect of Cu on Nanoscale Precipitation Evolution and Mechanical Properties of a Fe-NiAl Alloy. Microsc. Microanal. 23 (2), 350–359. doi:10.1017/s1431927616012599
Song, G., Sun, Z., Poplawsky, J. D., Gao, Y., and Liaw, P. K. (2017). Microstructural Evolution of Single Ni2TiAl or Hierarchical NiAl/Ni2TiAl Precipitates in Fe-Ni-Al-Cr-Ti Ferritic Alloys during Thermal Treatment for Elevated-Temperature Applications. Acta Mater. 127, 1–16. doi:10.1016/j.actamat.2017.01.011
Sun, W. W., Marceau, R. K. W., Styles, M. J., Barbier, D., and Hutchinson, C. R. (2017). G Phase Precipitation and Strengthening in Ultra-high Strength Ferritic Steels: towards Lean ‘maraging’metallurgy. Acta Mater. 130, 28–46. doi:10.1016/j.actamat.2017.03.032
Suzuki, A., Inui, H., and Pollock, T. M. (2015). L12-strengthened Cobalt-Base Superalloys. Annu. Rev. Mat. Res. 45, 345–368. doi:10.1146/annurev-matsci-070214-021043
Teng, Z., Ghosh, G., Miller, M. K., Huang, S., Clausen, B., Brown, D., et al. (2012). Neutron-diffraction Study and Modeling of the Lattice Parameters of a NiAl-Precipitate-Strengthened Fe-Based Alloy. Acta mater. 60 (13-14), 5362–5369. doi:10.1016/j.actamat.2012.05.033
Trotter, G., Hu, B., Sun, A. Y., Harder, R., Miller, M. K., Yao, L., et al. (2016). Precipitation Kinetics during Aging of an Alumina-Forming Austenitic Stainless Steel. Mater. Sci. Eng. A 667, 147–155. doi:10.1016/j.msea.2016.04.081
Vittori, M., and Mignone, A. (1985). On the Antiphase Boundary Energy of Ni3 (Al, Ti) Particles. Mater. Sci. Eng. 74 (1), 29–37. doi:10.1016/0025-5416(85)90107-7
Wang, Q., Li, Z., Pang, S., Li, X., Dong, C., and Liaw, P. (2018). Coherent Precipitation and Strengthening in Compositionally Complex Alloys: a Review. Entropy 20 (11), 878. doi:10.3390/e20110878
Wu, Z., Bei, H., Pharr, G. M., and George, E. P. (2014). Temperature Dependence of the Mechanical Properties of Equiatomic Solid Solution Alloys with Face-Centered Cubic Crystal Structures. Acta Mater. 81, 428–441. doi:10.1016/j.actamat.2014.08.026
Xue, F., Zhou, H. J., Ding, X. F., Wang, M. L., and Feng, Q. (2013). Improved High Temperature γ′ Stability of Co-al-W-base Alloys Containing Ti and Ta. Mater. Lett. 112, 215–218. doi:10.1016/j.matlet.2013.09.023
Yang, T., Zhao, Y. L., Tong, Y., Jiao, Z. B., Wei, J., Cai, J. X., et al. (2018). Multicomponent Intermetallic Nanoparticles and Superb Mechanical Behaviors of Complex Alloys. Science 362 (6417), 933–937. doi:10.1126/science.aas8815
Yen, H.-W., Chen, P.-Y., Huang, C.-Y., and Yang, J.-R. (2011). Interphase Precipitation of Nanometer-Sized Carbides in a Titanium-Molybdenum-Bearing Low-Carbon Steel. Acta Mater. 59 (16), 6264–6274. doi:10.1016/j.actamat.2011.06.037
Zhang, Y., Zuo, T. T., Tang, Z., Gao, M. C., Dahmen, K. A., Liaw, P. K., et al. (2014). Microstructures and Properties of High-Entropy Alloys. Prog. Mater. Sci. 61, 1–93. doi:10.1016/j.pmatsci.2013.10.001
Zhang, Z., Liu, C. T., Miller, M. K., Wang, X. L., Wen, Y., Fujita, T., et al. (2013). A Nanoscale Co-precipitation Approach for Property Enhancement of Fe-Base Alloys. Sci. Rep. 3 (1), 1327. doi:10.1038/srep01327
Zhao, D. Q., Pan, S. P., Zhang, Y., Liaw, P. K., and Qiao, J. W. (2021). Structure Prediction in High-Entropy Alloys with Machine Learning. Appl. Phys. Lett. 118 (23), 231904. doi:10.1063/5.0051307
Zhao, Y. Y., Chen, H. W., Lu, Z. P., and Nieh, T. G. (2018). Thermal Stability and Coarsening of Coherent Particles in a Precipitation-Hardened (NiCoFeCr)94Ti2Al4 High-Entropy Alloy. Acta Mater. 147, 184–194. doi:10.1016/j.actamat.2018.01.049
Keywords: multicomponent precipitation, precipitation strengthening, precipitation-strengthened alloys, high-entropy alloy, alloy design
Citation: Fang JYC, Liu WH, Yang T, Wu Y and Jiao ZB (2022) Multicomponent Precipitation and Strengthening in Intermetallic-Strengthened Alloys. Front. Mater. 9:931098. doi: 10.3389/fmats.2022.931098
Received: 28 April 2022; Accepted: 10 June 2022;
Published: 28 June 2022.
Edited by:
Sheng Guo, Chalmers University of Technology, SwedenReviewed by:
Pan Wang, Singapore Institute of Technology, SingaporeEhsan Ghassemali, Jönköping University, Sweden
Copyright © 2022 Fang, Liu, Yang, Wu and Jiao. This is an open-access article distributed under the terms of the Creative Commons Attribution License (CC BY). The use, distribution or reproduction in other forums is permitted, provided the original author(s) and the copyright owner(s) are credited and that the original publication in this journal is cited, in accordance with accepted academic practice. No use, distribution or reproduction is permitted which does not comply with these terms.
*Correspondence: Z.B. Jiao, zb.jiao@polyu.edu.hk