- 1Hunan Provincial Communications Planning, Survey and Design Institute Co. Ltd, Changsha, China
- 2Changsha Institute of Technology Testing and Consulting Co. Ltd., Changsha University of Science and Technology, Changsha, China
Due to the significant temperature difference and high solar radiation in high-altitude areas, the asphalt pavement has been in freeze-thaw cycles and radiation service conditions for a long time, resulting in a shorter service life. To extend the service life of asphalt pavement in high-altitude areas, this study uses basalt fiber to toughen the asphalt mixture, and the service characteristics of the asphalt pavement under the coupled effects of freeze-thaw cycles and aging were evaluated. First, the freeze-thaw cycles, aging time, and basalt fiber content were selected as independent variables to conduct the Box–Behnken design (BBD) test design. Consequently, the corresponding response surface model was established. Based on the rutting test, three-point bending test, and indirect tensile test, the optimum content of basalt fiber was obtained. Finally, the fatigue properties of the asphalt mixture by the four-point bending fatigue test were compared under the optimum content. The results showed the consequences of freeze-thaw cycles will reduce the road properties of asphalt and give rise to damage to the asphalt; among them, the indirect tensile strength was decreased by 21.7% at most (the number of freeze-thaw cycles from 10 to 30). Aging will reduce the fatigue life of the asphalt mixture, but its dynamic stability, flexural tensile strength, and slitting strength will be enhanced to a certain extent; among them, the dynamic stability can be improved by up to 28.1% (aging from 4 to 12 h). Basalt fiber can increase the flexural tensile strength, dynamic stability, and spitting strength; the optimum content is 0.35% of asphalt mixtures. Furthermore, the fatigue life of basalt fiber-reinforced asphalt mixture can be significantly improved, which can reduce the influence of freeze-thaw cycles and aging. In addition, the unit price of basalt fiber-reinforced asphalt mixture is only 6.9% higher than that of SBS-modified asphalt mixture, which has significant economic benefits. Under experimental conditions, the effect of aging on the properties of the asphalt mixture is greater than that of the freeze-thaw cycle. This research shows that careful consideration of the road behavior of basalt fiber-reinforced asphalt mixture under freeze-thaw cycles and aging is essential for the pavement durability design.
1 Introduction
Asphalt pavement is a structure with high comfort, superior performance, and simple construction and maintenance (Wu et al., 2019; Guo et al., 2020; Lv et al., 2021b). It is widely used in high-grade highway construction. New asphalt mixtures and recycled asphalt mixtures have been paid attention to in low latitude and hot climate areas. Researchers have used materials such as nano-silica and silica fume (Yunchao et al., 2021) and waste crumb rubber (Tang et al., 2021) to improve their high-temperature performance. However, for high-altitude areas, the service environment of highway asphalt pavement is complex and flawed. Because of its particularity of low temperature, high altitude, significant temperature difference, and intense solar radiation, it has become one of the difficulties of highway construction. On the one hand, a significant temperature difference leads to enormous temperature stress in asphalt in a low-temperature environment. In addition, with ultraviolet radiation, asphalt aging occurs, making asphalt pavement prone to cracking (Nian et al., 2018a; Wu et al., 2019). On the other hand, the cyclic temperature change causes the asphalt pavement to repeatedly freeze-thaw in high-altitude areas, and the fatigue resistance decreases significantly (Fan et al., 2020). Therefore, it is essential to add the modifier to improve the cracking resistance of the asphalt mixture (Lv et al., 2021a; Lv et al., 2021c).
Fibrous materials, such as polyester fiber and mineral fiber, are generally added to enhance the working properties of asphalt. Wu et al. explained that when polyester fiber is added to asphalt mixture, it is found that the flexural tensile strength of the asphalt mixture increases by 86.6% under the condition of optimal fiber content (0.4% by mass of asphalt mixture) (Chen F et al., 2019; Wu, J. et al., 2020). Mohammed et al. added an appropriate amount of mineral fibers to the asphalt mixture to enhance its mechanical properties. They found that the permanent deformation of stone mastic asphalt mixtures could be reduced by 50.1% at 0.3% fiber content (Lavasani et al., 2015).
The advantages of fiber and asphalt mixture can be fully utilized by adding fiber into the asphalt mixture (Wang et al., 2018). The fibers play a role in strengthening, stabilization, crack resistance, adsorption, etc. (Xing et al., 2019). The asphalt mixture can make the deformation resistance and the resistance of the asphalt mixture better. The effect of a fatigue crack is more significant (Abdelsalam et al., 2020). Numerous studies have shown that these fiber modifiers are effective means for improving the property of asphalt mixtures (Wang et al., 2018; Abdelsalam et al., 2020; Guo et al., 2020;; Zhao et al., 2020).
Basalt fiber, an inorganic material, has excellent properties, such as environmental protection, high-temperature resistance, high strength, and low water absorption (Zhao et al., 2020). Basalt fiber has been extensively used to take the place of polyester fibers and lignin-fiber, etc., In asphalt mixtures, it enhances low-temperature crack resistance, fatigue resistance, and high-temperature stability (Cheng et al., 2018a; Xing et al., 2019; Liu et al., 2020). The incorporation of basalt fiber into asphalt is expected to reduce the attenuation of its strength and increase its service life under the action of freeze-thaw cycles (Cheng et al., 2018b).
However, during the use of asphalt pavement, on account of prolonged exposure to the air, under the impaction of heat, oxygen, water, and other environmental elements, asphalt will undergo volatilization, oxidation, polymerization, and even internal structure changes (Ma et al., 2021; Qu et al., 2018; Zhang, Q. et al., 2021). At the same time, the property changes will also give rise to the deterioration of road performance, which immediately affects road durability. At the same time, the current research on basalt fiber reinforced asphalt mixture mainly focuses on road performance and only studies aging or freeze-thaw cycles (Cheng et al., 2019b; Huang et al., 2022; Wu Z. et al., 2020; Zhou et al., 2020), and the coupling effect of the two on the performance and life evolution of the asphalt mixture is relatively small. Therefore, it is indispensable to comprehensively consider the impact of freeze-thaw cycles and the coupling effect of asphalt aging to evaluate the comprehensive performance of basalt fibers more accurately.
Because the coupling impact of freeze-thaw cycles and asphalt aging needs to be considered and the effect of basalt fiber content on it is not apparent, the number of freeze-thaw cycles (Badeli et al., 2018; Nian et al., 2018b), aging time (Sirin et al., 2019; Zhang, Z. et al., 2021), and basalt fiber content will have an obvious effect on the asphalt (Guo et al., 2020; Wang et al., 2018). Since the performance index of asphalt involves multiple independent variables, the data are complex, and there are many influencing factors. Consequently, it is indispensable to seek a method to process and analyze the data to comprehensively analyze the effect of the change of independent variables on the performance of asphalt.
Response surface methodology (RSM) is a method used to analyze variables and response values together (Liu et al., 2021). By searching for the relationship between the response index and each variable, the model is built first, and then the optimal test is found. (Hamzah et al., 2017; Omranian et al., 2018; Saha and Biligiri, 2016; Wang et al., 2018). The response surface method used by Cheng to optimize the performance of environmentally friendly porous asphalt mixtures completed modeling and optimization of porous asphalt mixtures with sufficient precision and determined the asphalt to the aggregate ratio of colloidal particles and basalt fibers. The optimum contents were 4.51, 11.21, and 0.42%, respectively (Cheng et al., 2019a). Gong et al. (2020) studied nano-TiO2, CaCO3 (NTC), and basalt fiber by RSM. Thus, it indicates that the RSM is extensively used, and it can analyze the change rule of the independent variables to obtain the best response value. Applying the response surface method to this research can comprehensively analyze multiple factors to find their respective changes—the law of impact on the performance index of asphalt. Finally, the optimum basalt fiber content can be obtained by integrating the performance index of asphalt.
In summary, an army of researchers enjoys paying attention to basalt fibers to improve the influence of freeze-thaw cycles on asphalt mixtures. Still, aging also has an important impact on asphalt mixtures (Fu et al., 2022). The on-road research properties of lower basalt fiber-reinforced asphalt mixture under the combined action impact of freeze-thaw and aging are currently lacking.
This study uses the Box–Behnken Design method, with the basalt fiber content, aging time, and several freeze-thaw cycles as independent variables, through a rutting test, three-point bending test, and indirect tensile test study to develop its road performance indicators. At the same time, taking dynamic stability, 60 min cumulative deformation, flexural tensile strength, breaking strength, and fatigue life as the response values, the response surface was plotted by Design-Expert software, and the corresponding fitting equation was obtained. Based on the performance indexes, the optimum basalt fiber content was obtained. Four-point bending fatigue tests were used to analyze the interaction between aging and freeze-thaw cycles. In the experiment, the asphalt mixture was modified with different amounts of basalt fibers and then subjected to varying degrees of thermal aging when preparing the asphalt mixture. Then, the low of the different freezing-thawing cycles was analyzed based on the asphalt mixture. The impacts of basalt fiber content, aging degree, and freeze-thaw processes on the road behavior of asphalt mixture were analyzed through a three-point bending test, rutting test, indirect tensile test, and breaking fatigue test. Figure 1 shows the test procedure.
2 Raw Materials and Experimental Design
2.1 Raw Materials
The material test in this study is carried out according to “Standard Test Methods of Asphalt and Asphalt Mixtures for Highway Engineering” (JTG E20-2011).
2.1.1 Asphalt
The SBS modified asphalt used in this study is produced by Xiamen Huate Group Co., LTD. Various indexes of the asphalt were tested, as shown in Table 1.
2.1.2 Thick and Fine Stone
The asphalt mixture is a multiphase composite material prepared by proportional asphalt and mineral materials (fillers, coarse, and fine aggregates) by a specific molding method. Generally speaking, the ratio of mineral materials is 95% of the quality of the asphalt mixture. Therefore, the physical and chemical properties of fillers and coarse and fine aggregates have an essential impact on the performance of asphalt mixtures (Pouranian and Haddock, 2020; Zulkati et al., 2012). Limestone is selected as an aggregate. The density of aggregates with different particle sizes was measured, and the results are listed in Table 2.
2.1.3 Basalt Fiber
The fiber stabilizer used in the experiment was golden brown basalt fiber with a diameter of 13 μm and a length of 6 mm by Jilin Tongxin Basalt Technology Co., Ltd., and the fiber content was 0.34% (data provided by the manufacturer) (Guo et al., 2020). The technical indicators are demonstrated in Table 3.
2.2 Mixture Design
The AC-13 gradation, which is extensively used in China, is selected, and the SBS modified asphalt is generally used in the upper layer of asphalt pavement. The classification design is demonstrated in Table 4, and the gradation curve is shown in Figure 2.
The Marshall test was used to determine the optimum asphalt-stone ratio of AC-13, and the test results are demonstrated in Table 5.
According to the raw material test, the physical properties of aggregate, asphalt, and other materials were determined. All the results met the requirements of the specification. The gradation design of the mixture was completed, and the optimum content of the SBS modified asphalt mixture and basalt fiber-reinforced asphalt mixture were determined through the Marshall test.
2.3 Experimental Design
The experiment was designed by the response surface method, referring to relevant research (Zhang et al., 2017; Cheng et al., 2018a; Cheng et al., 2019c), selecting the basalt fiber content, which is between 0.1 and 0.5%, the aging time in the field of 4–12 h, and the freeze-thaw cycles within the scope of 10-30 times. The factor coding table is as follows, as shown in Table 6. 17 experiments were included using the Box–Behnken design (BBD), as shown in Table 7.
Suppose the relationship between the performance index parameters y and the independent variable xi is as follows:
If there is a nonlinear relationship between the response value and the influencing factors, performance index parameters y and the independent variable xi, it is necessary to carry out a second-order design and use the multivariate function regression equation in statistics to fit the second-order model:
Here
By the analysis of the variance test of significance, multivariate analysis of the second-order polynomial model is set up. The model is used to evaluate the effect of each impacting factor variable on the response value and finally generate the response surface. According to the response value’s setting conditions, the test’s optimal response value and the corresponding combination of influencing elements are given.
2.4 Test Method
2.4.1 Asphalt Mixture Preparation
The asphalt, crushed stone, and basalt fibers were first placed in an oven, in which the asphalt was kept at 150°C for 2 h to make it enjoy favorable fluidity, and the basalt fibers were observed at 150°C for 4 h. After weighing the crushed stone according to the mixing ratio, it was stirred in the mixing pot for 1.5 min after the preparation process of asphalt. The weighed asphalt, basalt fiber (0.1, 0.3, and 0.5% of the asphalt mixture mass), and mineral powder were added in sequence. Each addition was stirred for 1.5 min. The mixed asphalt mixture is a basalt fiber-reinforced asphalt mixture.
The asphalt mixture was put in the aging tray, subjected to different degrees of aging (4, 8, and 12 h) in the oven, and formed into the corresponding test pieces according to other test methods. The formed asphalt mixture was cooled, de-molded, and cut. Then the asphalt mixture was carried out with the water-saturated treatment in a vacuum container, frozen at −18°C for 16 h, placed at room temperature to dry the moisture, and then put at 60°C for 24 h of soaking. This operation is repeated at different times, and finally, the corresponding specimens required for the study are obtained. The preparation process of the asphalt mixture is shown in Figure 3.
2.4.2 Rutting Test
The high-temperature stability of an asphalt mixture is obtained by a rutting test, which is a method to analyze the properties of the mix based on permanent deformation with dynamic stability and vertical deformation as indicators. Generally, the compound has high dynamic stability, the vertical deformation is small, and the resistance to permanent deformation is strong. Therefore, this section chooses the rutting test to study the asphalt mixture’s high-temperature performance. The rutting test refers to specification JTG E20 (T 0719) and AASHTO TP63-07.
1) Specimen production: first, the compaction method is used, and then the wheel rolling process is used. The load of the compaction line is 300N/cm. First, one direction of the plate is moved back and forth twice and then unloaded, and the other direction of the container is changed to be rolled back and on 12 times.
2) Sample size: the sample length is 300 mm, width is 300 mm, and height is 300 mm.
3) Test piece curing temperature and curing time: adjust the temperature of the environmental box to 60 ± 1°C. After the temperature is stable, cool the test piece into it and keep it at a constant temperature for 6 h.
4) According to the requirements of T 0719-2011, the rolling rate of 42 times/min is adopted for the plate, the traveling direction of the wheel is consistent with the main focus of the rolling molding of the test piece, and the traveling distance of the wheel is 230 ± 10 mm. The test ends when the total round-trip time of the revolution reaches 60 min, or the maximum deformation reaches 25 mm.
The dynamic stability is calculated using Eq. 3.
where DS is the dynamic stability of the asphalt mixture, times/min;d1 is the deformation of the rutting plate at the time of t1, mm;d2 is deformation of the rutting plate at time t2, mm;C1 is the correction factor type testing machine;C2 is the coefficient of the specimen, was 1.0 for the specimen with a width of 300 mm prepared in the laboratory and 0.8 for the specimen with a width of 150 mm cut from the road surface. In this test, 1.0 was taken;N is the reciprocating rolling rate of the test wheel, for this experiment it is 42 times/min;t1 is generally 45 min, and t2 is generally 60 min. If the rut depth is too large, and the deformation exceeds 25 mm before 60 min, the 25 mm is taken as d2, the corresponding time is t2, 15 min before t2 is t1, and the corresponding deformation is as d1.
2.4.3 Indirect Tensile Test
The asphalt mixture specimens used in the indirect tensile test were formed by the Marshall standard compaction method. The diameter of the asphalt mixture specimens was 101.6 ± 0.25 mm, and the height was 63.5 ± 1.3 mm. A universal testing machine carries out the indirect tensile test. Based on the specification, the asphalt is fixed with a bead at the top and bottom, one at the top and one at the bottom. The width of the bead is 12.7 mm, the inner radius of curvature is 50.8 mm, and both ends of the bead should be rounded. A temperature control box controlled the test temperature of this experiment, the temperature of the test was 15 ± 0.5°C. The test results for asphalt are obtained automatically by the computer controlling the universal testing machine. The indirect tensile test refers to specification JTG E20 (T 0716), ASTM D 4123, and AASHTO T 283. In this experiment, the indirect tensile strength is used as the performance index for evaluating the asphalt mixture. The split tensile strength RT is obtained by Eq. 4.
Here, RT is the indirect tensile strength (MPa);PT is the maximum force value (N) of the test load; h is the height of the specimen.
2.4.4 Three-Point Bending Test
The three-point bending test refers to specification JTG E20 (T 0715). The three-point bending test is carried out as follows:
1) Specimen production: first, the plate specimen with a size of 300 mm × 300 mm × 50 mm was formed by the roller rolling method, and then the trabecular extent required by the test was cut with a cutting machine.
2) Specimen size: the length of the specimen is 250 mm, the width is 30 mm, the height is 35 mm, and the size error is ±2 mm.
3) Test specimen curing temperature and the curing time: the temperature is adjusted to 15 ± 0.5°C; when the temperature is stable, the specimen is cooled, added, and kept at a constant temperature for 1 h.
4) According to T 0715-2011, the test temperature is −10°C, a loading rate of 50 mm/min is applied to the trabecular, and it ends when the trabecula breaks.
The flexural tensile strength RB is calculated according to Eq. 5:
where L is the span of the specimen in mm;b is the width of the cross-interruption interview piece in mm; h is the height of the cross-interruption interview piece in mm; PB is the maximum load when the specimen fails, N.
2.4.5 Four-Point Bending Fatigue Test
In this study, the fatigue test of the asphalt mixture under the combined action of freeze-thaw cycles and aging is carried out. The fatigue life of the fatigue test affects the life of the pavement and is a significant parameter. The four-point bending test mainly reflects the flexural and tensile properties of the asphalt mixture, which is close to the service life of asphalt pavement in actual engineering. Moreover, the four-point bending fatigue test is less discrete than other fatigue tests. The four-point bending fatigue test refers to specification JTG E20 (T 0739) and AASHTO T 321-03. A universal testing machine was used to carry out the four-point bending experiment in this test. The test temperature was 15°C, the test frequency was 10 Hz, and four different strains of 300 × 10-6, 400 × 10-6, 500 × 10-6, and 600 × 10-6 were selected for the fatigue test.
3 Test Results
The road performance tests were carried out, and all the test consequences are listed in Table 8. Design-Expert software was used to draw all performance index analyses in this study. The software removes the corresponding response surface, and the corresponding fitting equation is obtained.
3.1 Rutting Test
Three parallel tests were carried out on each asphalt mixture during the rutting test. The specimen and the test mold before the test are placed in the rutting tester for more than 5 h, and then the rutting test machine is set up according to the test procedures. After the test is completed, record the data. The dynamic stability results are demonstrated in Figure 4, and the corresponding fitting equation is shown in Eq. 6. To facilitate the fitting, this study uses the cumulative deformation of asphalt at 60 min as the index to evaluate the rutting deformation. The cumulative deformation of asphalt is demonstrated in Figure 5; the corresponding fitting equation is shown in Eq. 7.
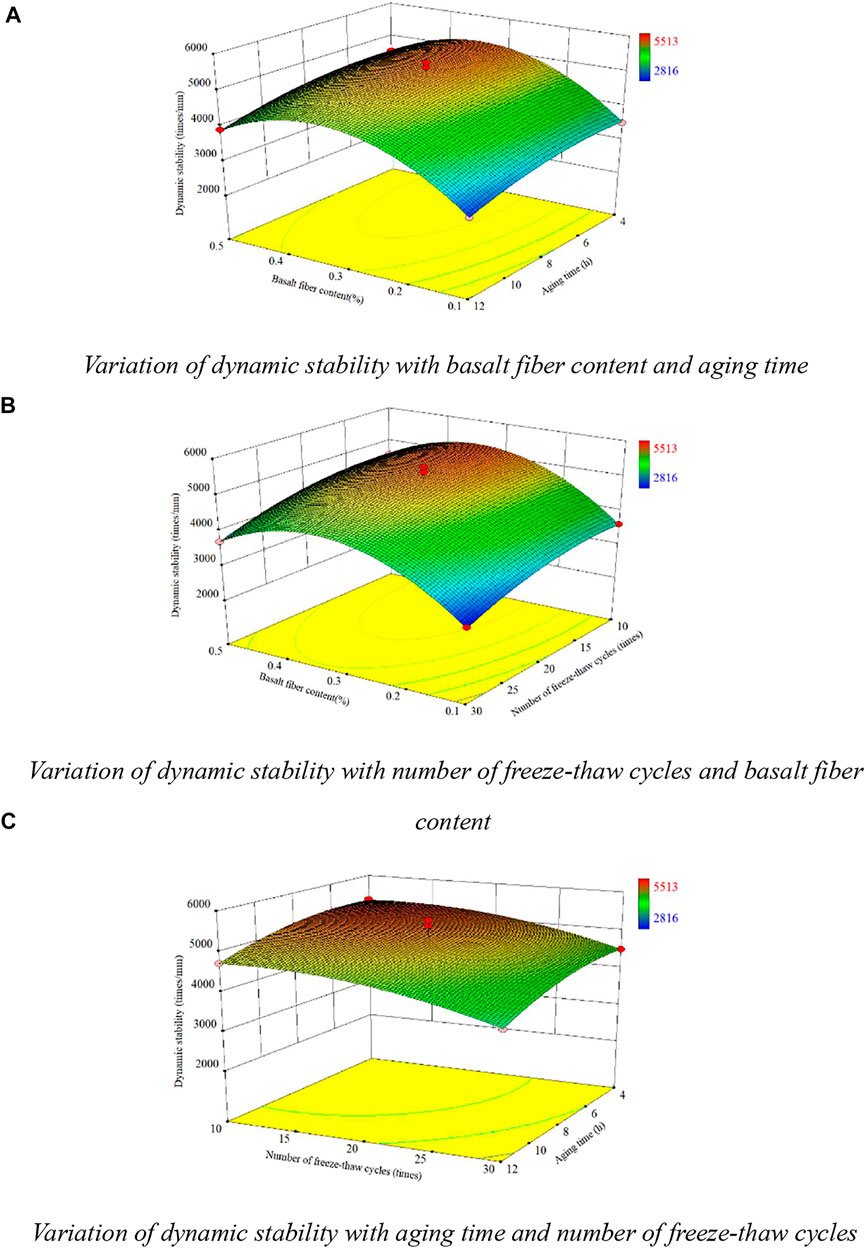
FIGURE 4. Test result of dynamic stability. (A) Variation of dynamic stability with the basalt fiber content and aging time. (B) Variation of dynamic stability with the number of freeze-thaw cycles and basalt fiber content. (C) Variation of dynamic stability with aging time and the number of freeze-thaw cycles.
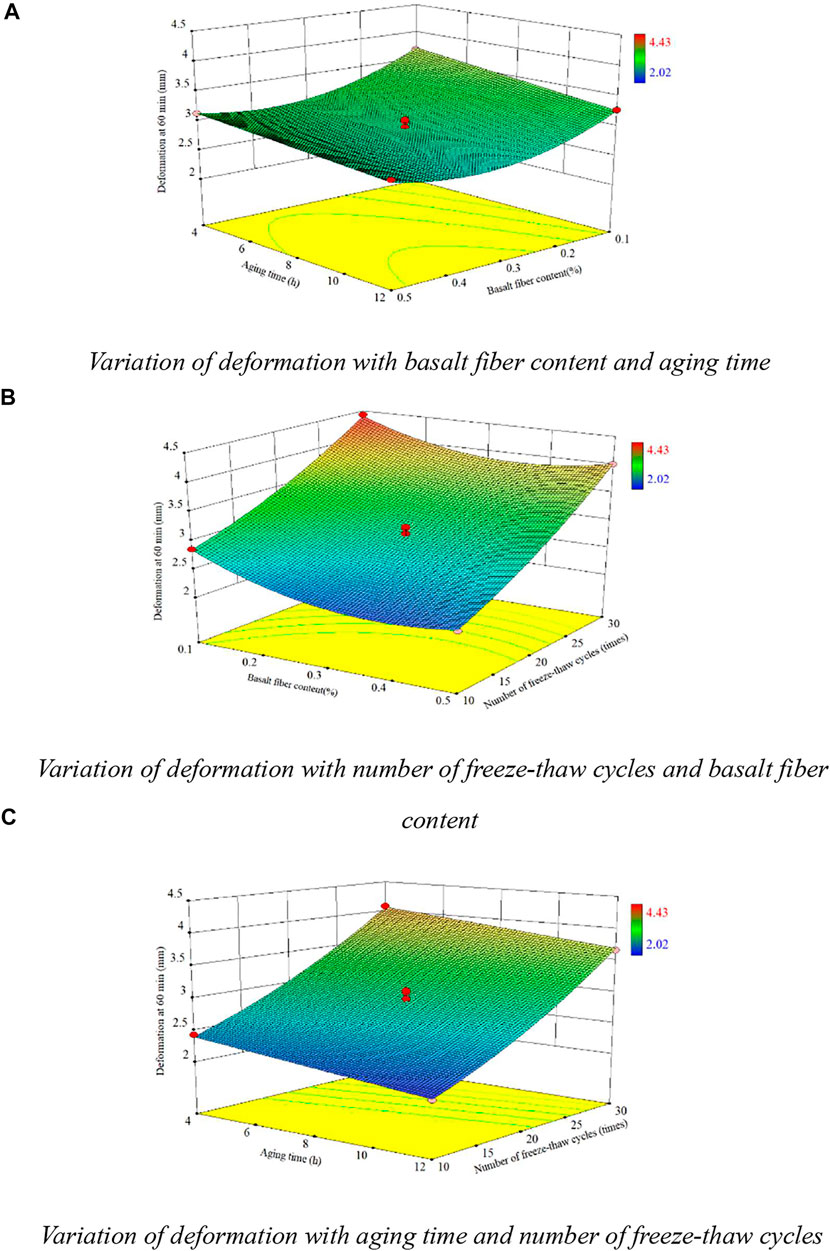
FIGURE 5. Test result of deformation. (A) Variation of deformation with the basalt fiber content and aging time. (B) Variation of deformation with the number of freeze-thaw cycles and basalt fiber content. (C) Variation of deformation with aging time and the number of freeze-thaw cycles.
A rutting test can characterize the high-temperature properties of asphalt. Figure 4 shows that freeze-thaw cycles, aging time, and basalt fiber content positively impact dynamic stability. When the aging time and the basalt fiber content are constant, the more the times of freeze-thaw cycles, the emotional strength of the asphalt mixture will decrease significantly. There are three tests with the same aging time and basalt fiber content. As the quantity of freeze-thaw cycles increases, the dynamic stability reduces to varying degrees. The number of freeze-thaw cycles grew from 10 to 30 times, and the dynamic stability degrees dropped by 21.9%. This indicates that the asphalt mixture is damaged to a certain extent, and its strength is reduced during the freezing and thawing process. When the quantity of freeze-thaw cycles and the content of basalt fibers are constant, the longer the aging time, the higher the dynamic stability. This is because the aging of the asphalt will reduce the ratio of light elements to heavy elements. As it increases, the asphalt becomes more challenging, and its strength increases. When the aging time and the times of freeze-thaw cycles are constant, as the basalt fiber content increases, the dynamic stability of the asphalt mixture first increases and then decreases. Basalt fiber can significantly increase the dynamic stability of asphalt. Basalt fiber can form a three-dimensional grid structure in an asphalt mixture, play a reinforcing role, and improve the dynamic modulus of asphalt. On the other hand, excessive basalt fiber may cause uneven fiber dispersion and excessive porosity of the mixture, which reduces the performance of the asphalt mixture (Zhao et al., 2020).
Asphalt is prone to rutting deformation in a high-temperature environment, and rutting deformation is also a paramount indicator of the high-temperature properties of asphalt. Figure 5 shows that freeze-thaw cycles, aging time, and basalt fiber content have remarkable influences on the dynamic stability of asphalt. When the aging time and the basalt fiber content are constant, the more the quantity of freeze-thaw cycles, the cumulative deformation of the asphalt mixture reduces significantly. The amount of freezing-thawing process and basalt fiber content are constant, the longer the time of aging, the greater the cumulative deformation of the asphalt mixture. Under certain conditions of aging time and freeze-thaw cycles, with the increase of basalt fiber content, the cumulative deformation of the asphalt mixture decreases first and then increases. There are three tests with the same aging time and freeze-thaw cycle times. The cumulative deformation has been reduced to varying degrees as the basalt content increases. The basalt content increased from 0.1 to 0.5%, and the maximum cumulative deformation was reduced by 22.1%. Certain basalt fibers can reduce the rutting deformation of the asphalt mixture and enhance its high-temperature performance.
3.2 Three-Point Bending Test
Three parallel tests were carried out on each asphalt mixture during the three-point bending test. First, the specimens were placed in a heated water tank at −10°C for 1 hour. After the heat preservation, start the trial immediately and record the data after the test. Figure 6 shows the three-point bending test results and corresponding fitting equation demonstrated in formula (8).
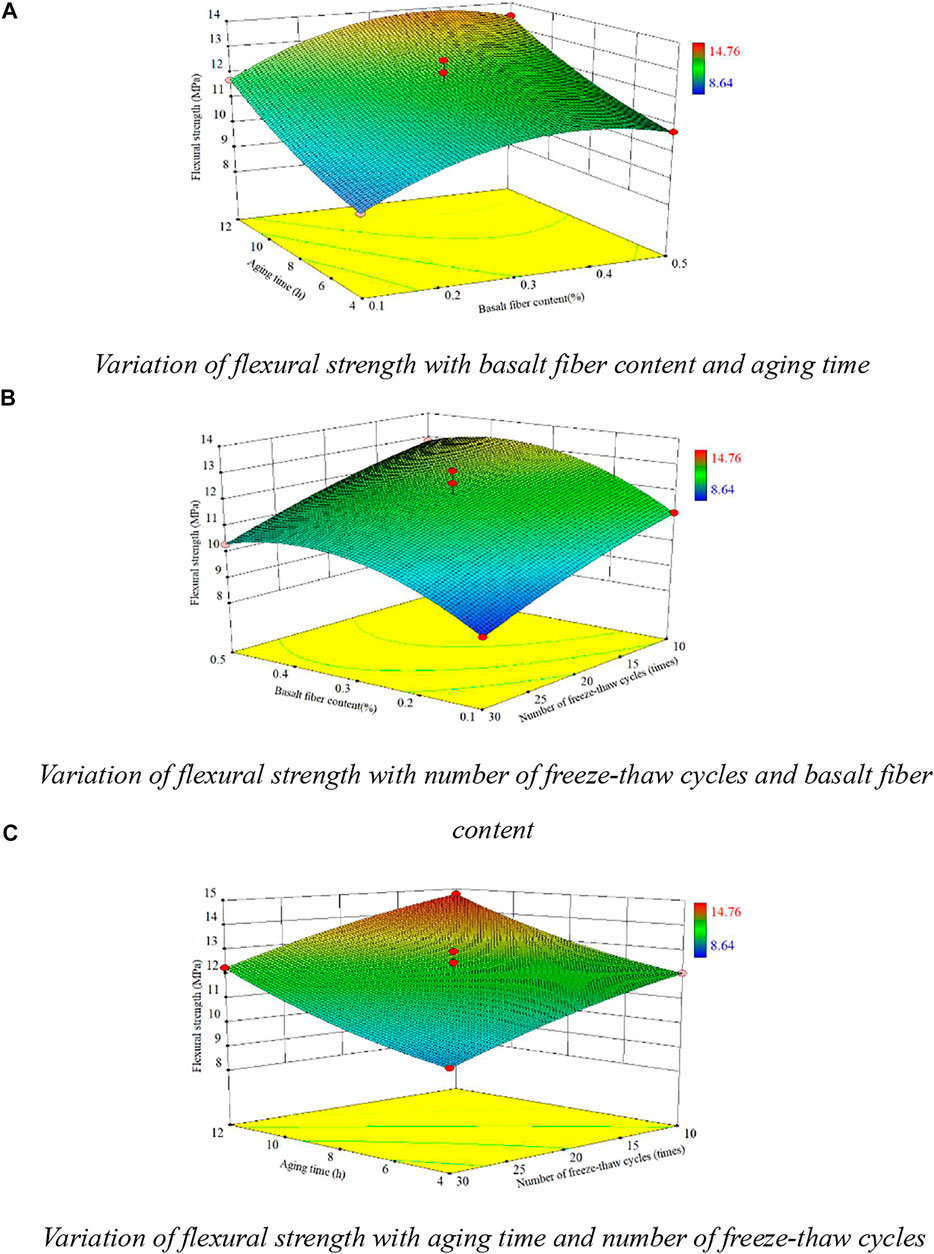
FIGURE 6. Three-point bending test results. (A) Variation of flexural strength with the basalt fiber content and aging time. (B) Variation of flexural strength with the number of freeze-thaw cycles and basalt fiber content. (C) Variation of flexural strength with aging time and the number of freeze-thaw cycles.
A three-point bending test can be utilized to analyze the low-temperature behavior of the asphalt mixtures (Guo et al., 2020; Wang et al., 2019; Wang et al., 2018). Figure 6 shows that the freeze-thaw cycle, aging time, and basalt fiber content have stimulating effects on the flexural tensile intensity of asphalt. When the aging time and the basalt fiber content are constant, the more the quantity of freeze-thaw cycles, the more the flexural tensile strength of the asphalt mixture reduces significantly. Freeze-thaw cycles were increased from 10 to 30 times, and maximum bending tensile strength was decreased by 22.5%. The freeze-thaw process causes unavoidable damage to the asphalt structure, thereby reducing the flexural tensile strength. When the amounts of freeze-thaw cycles and the content of basalt fibers are constant, the longer the aging time, the greater the flexural tensile intensity of the asphalt mixture, which is suggestive that the asphalt aging increases the power of the asphalt, which harms the low-temperature behavior of the asphalt. It has an obvious positive effect. When aging time and freeze-thaw cycles are constant, the higher the content of basalt fiber, the higher the flexural tensile strength of the asphalt mixture. With the basalt content increasing, the flexural strength of the asphalt mixture rises first and then decreases. When the basalt content is increased from 0.1 to 0.5%, the maximum tensile strength can be increased by 19.2%. Basalt fibers form a three-dimensional grid structure in an asphalt mixture. At the same time, basalt fiber has a reinforcing influence on the asphalt. When the asphalt is stressed, it will produce a specific resistance to deformation, increasing the flexural tensile intensity of the asphalt. Basalt fiber was beneficial to the low-temperature properties of the asphalt mixture.
3.3 Indirect Tensile Test
Three parallel tests were conducted in the indirect tensile test. Asphalt mixture specimens are taken out immediately after completing the freeze-thaw cycle (if indispensable) and subjected to an indirect tensile test at 50 mm/min loading speed. Figure 7 shows the recorded test results and the corresponding fitting equation demonstrated in Eq. 9.
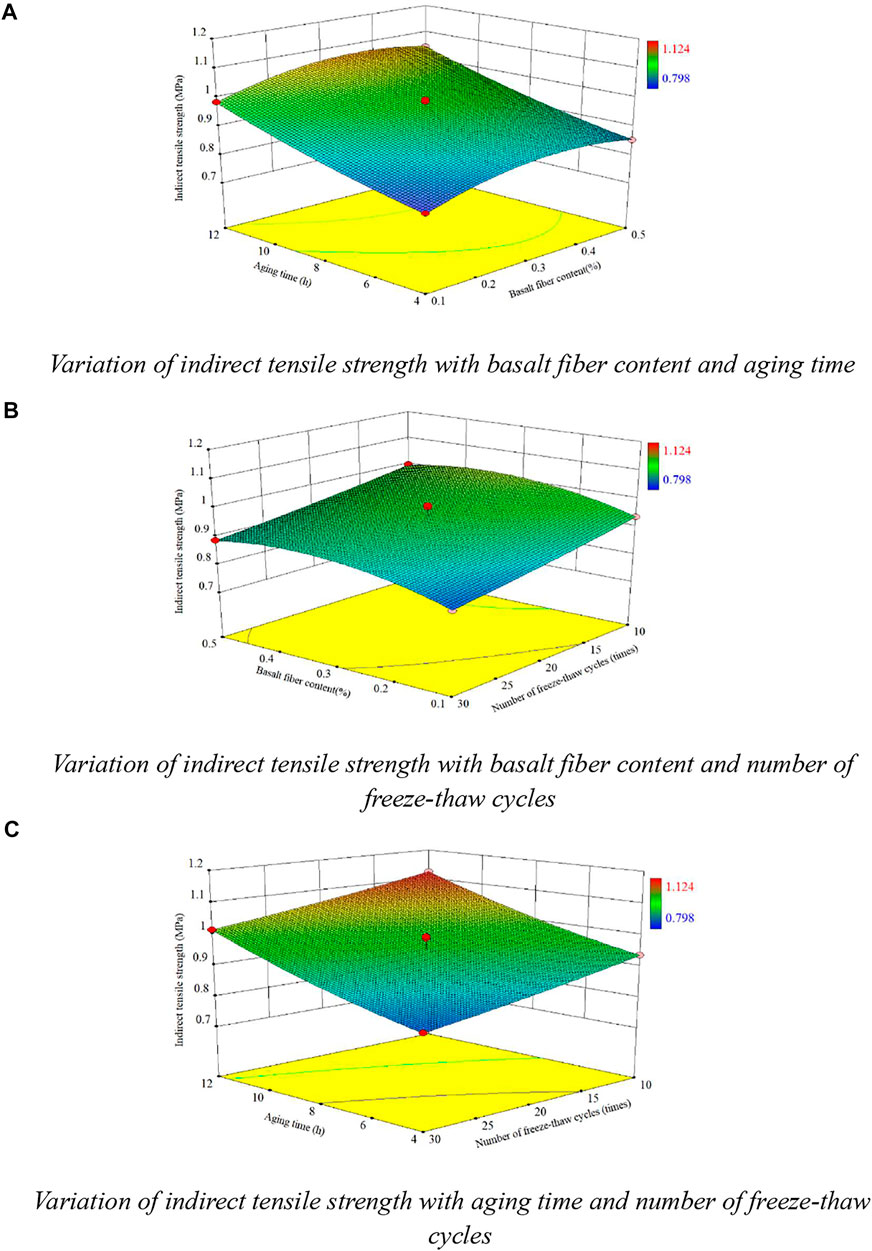
FIGURE 7. Indirect tensile test results. (A) Variation of indirect tensile strength with the basalt fiber content and aging time. (B) Variation of indirect tensile strength with the basalt fiber content and number of freeze-thaw cycles. (C) Variation of indirect tensile strength with aging time and the number of freeze-thaw cycles
The indirect tensile test was used to analyze the strength behavior of the asphalt mixture. Figure 7 shows that freeze-thaw cycles, aging time, and basalt fiber content positively impact the indirect tensile strength. When the aging time and the basalt fiber content are constant, with more freeze-thaw cycles, the indirect tensile strength of the asphalt mixture will decrease significantly. The freeze-thaw cycles increased from 10 to 30 times, and the indirect tensile strength decreased by 12.2%. Relevant studies have shown that freeze-thaw cycles can weaken the freeze-thaw indirect tensile intensity of the asphalt mixture. When the times of freeze-thaw cycles and basalt fiber content is constant, the more the aging time is, the greater the indirect tensile intensity of the asphalt mixture appears. The aging time increased from 4 to 12 h, and the maximum indirect tensile strength increased by 23.5%. When the freezing-thawing cycles and freezing-thawing cycles are the same, the indirect tensile strength of the asphalt mixture rises first and then decreases with the basalt fiber content increasing. The basalt content increased from 0.1 to 0.5%, and the maximum indirect tensile strength increased by 8.0%. This shows that basalt fiber plays a specific role in enhancing the resilience of asphalt, which is not enough to reduce the negative impact of asphalt received by freeze-thaw cycles.
3.4 Analysis of Variance
To ensure that the meaning of the established 3D response surface and polynomial fitting equation, using analysis variance to test it (Parchami et al., 2017), and the recorded results of the study of conflict are demonstrated in Table 9.
The size of the p-value checks the significance of a variable. The variable is more significant while the p-value is smaller. Table 8 shows that all p-values are less than 0.001, indicating that the test model has good reliability. Generally speaking, for the rutting test, the greater the dynamic stability of the asphalt mixture is, the smaller the cumulative deformation is, indicating that the asphalt mixture has a better ability to resist rutting; similarly, for the three-point bending test, the greater the bending and tensile strength of asphalt, the better the low-temperature behavior of asphalt. For the indirect tensile test of asphalt, the greater the indirect tensile strength of asphalt, the better the performance (the greater the indirect tensile strength after the freeze-thaw cycle, the better the water stability). Therefore, Design-Expert software is used to synthesize the road performance test results of the four asphalt mixtures. Under the corresponding conditions, the optimal basalt fiber content can be obtained at 0.35%.
3.5 Four-Point Bending Fatigue Test
A fatigue test can best reflect the service behavior of the asphalt pavement. To better compare the coupling effects of the freezing-thawing cycle and aging on basalt fiber-reinforced asphalt mixture and analyze their interaction rules, this study adopts a four-point bending fatigue test to study basalt fiber-reinforced asphalt mixture under the coupling effects of the freezing-thawing cycle and aging. The best basalt fiber content obtained by the road strength test is 0.35% in the fatigue test. Figure 8 shows the recorded test results, and Figure 9 shows parameter variation of the fatigue fitting equation.
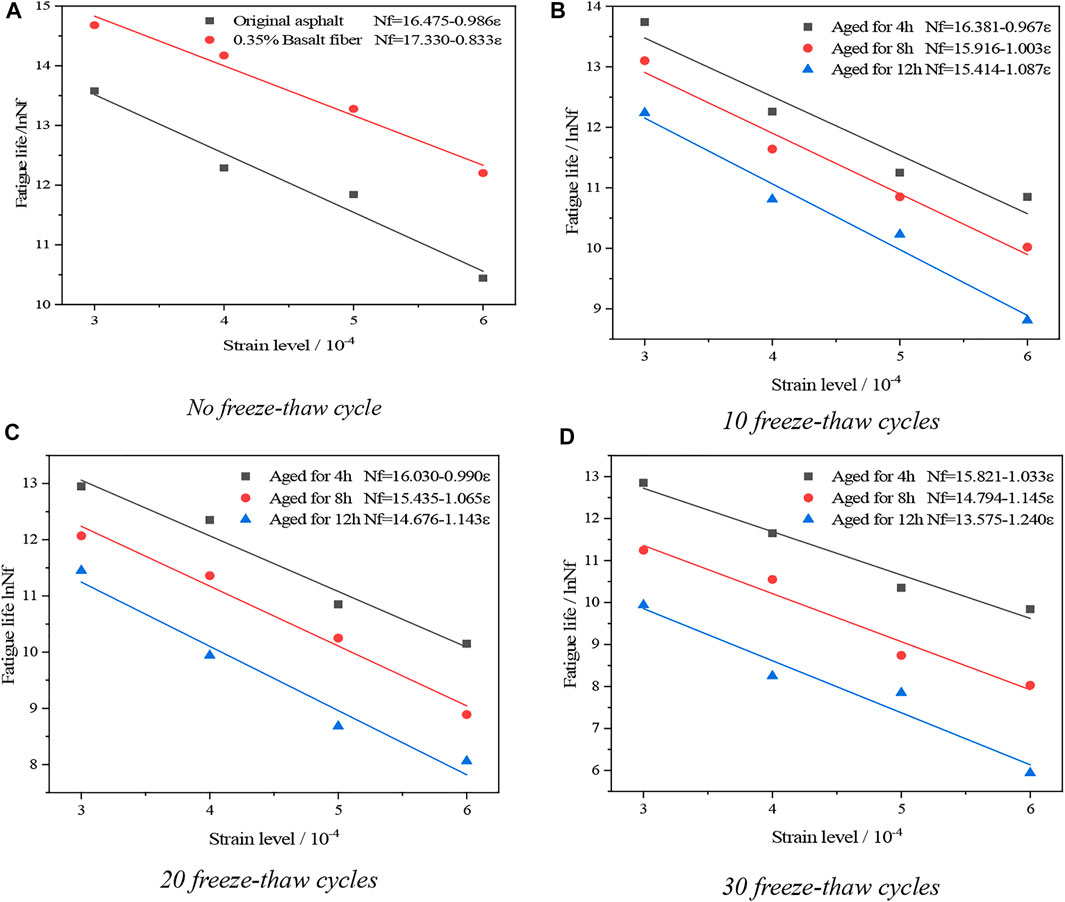
FIGURE 8. Fatigue test results. (A) No freeze-thaw cycle; (B) 10 freeze-thaw cycles; (C) 20 freeze-thaw cycles; (D) 30 freeze-thaw cycles.
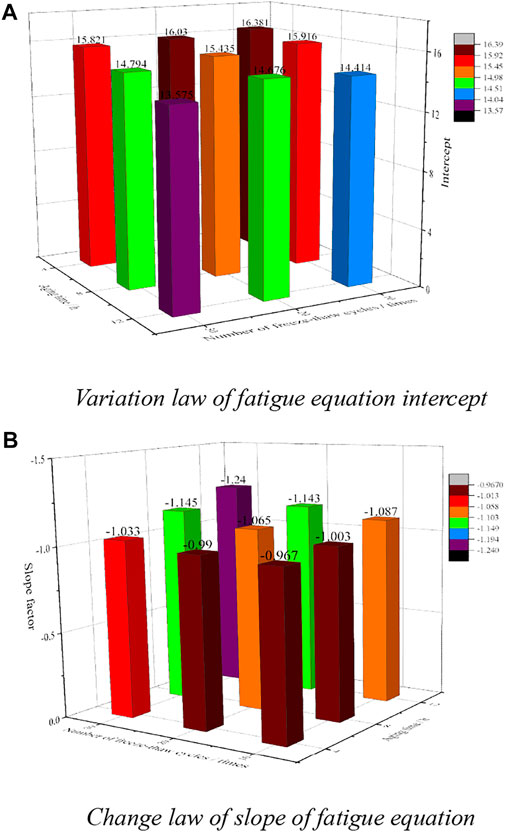
FIGURE 9. Variation law of fatigue equation parameters. (A) Variation law of the fatigue equation intercept. (B) Change law of the slope of the fatigue equation.
A four-point bending fatigue test obtained the fatigue performance of the asphalt mixture. The fatigue properties of an asphalt mixture are better if the fatigue life is longer, which means that asphalt has a better service life in application and engineering practice. Figure 8 reveals that the fatigue life of the asphalt mixture is significantly improved after the addition of 0.35% basalt fiber, which indicates that basalt fiber has a significant enhancement on the asphalt mixture properties. An asphalt mixture can show more toughness when it is damaged by fatigue to withstand more stress. The basalt fiber acts as a reinforcement in the asphalt. The asphalt mixture can be transmitted when it is stressed, and the stress is not easy to damage, so the fatigue life of the asphalt mixture is improved. Ten times of the freeze-thaw cycle and 4 hours after aging of basalt fiber-reinforced asphalt mixture, the fatigue performance is slightly lower than the original asphalt mixture. The freeze-thaw cycle and aging will affect the performance of the asphalt mixture, and basalt fiber can improve the performance of the asphalt mixture, improving the performance of both the freeze-thaw cycle and aging degradation. By comparing the curves in the figure, it can be found that with the increase in aging degree, the fatigue life of the asphalt mixture gradually decreases. Although aging increases the strength of the asphalt mixture, at the same time, the asphalt mixture becomes brittle and complex due to the appearance of the asphalt components. The fatigue life of the asphalt mixture decreases when the frequency of freeze-thaw cycles increases. This is because the freeze-thaw revolutions destroy the asphalt mixture to a certain extent, and the more the freeze-thaw cycles, the shorter the fatigue life of the asphalt.
In the fitting equation, the equation slope represents the sensitivity of the fatigue life of the asphalt mixture to the strain level. In contrast, the intercept theoretically represents the fatigue life of the asphalt mixture at no strain. Therefore, the larger the intercept of the asphalt mixture is, the better the fatigue properties of the asphalt mixture are. The smaller the slope is, the greater the influence of the stress level is. The asphalt mixture becomes more sensitive to stress levels after aging and freeze-thaw cycles. By comparing the fatigue life curves of nine asphalt mixtures with different freezing-thawing cycles and different aging times, it can be found that asphalt is slightly more affected by aging than freezing-thawing cycles. In practical engineering applications, both hurt the service life of asphalt pavement, but overall, aging may affect the asphalt pavement’s service behavior more. The coupling effect of the freezing-thawing cycle and aging should be considered in pavement design in alpine regions, especially the influence of aging.
The price of basalt fiber used in this study is 1,620.3 dollars per ton; the cost of SBS asphalt mixture is 1,325.7 dollars per ton; and the price of the stone is 14.73 dollars per ton. According to the conditions in this study, the cost per hundred tons of SBS modified asphalt mixture and basalt fiber-reinforced asphalt mixture is shown in Table 10.
For basalt fiber-reinforced asphalt mixture, the unit cost of basalt fiber-reinforced asphalt mixture increases by 6.9% compared with SBS modified asphalt mixture, and the average fatigue life of basalt fiber-reinforced asphalt mixture under four-strain conditions is increased by nearly three times, showing significant economic benefits. Basalt fiber is a very economical and efficient material to improve the life of an asphalt mixture, but the problem of segregation still needs to be solved in engineering applications (Zhao et al., 2020).
4 Conclusion
In this work, the influence of the freezing-thawing cycle and aging on the behavior of basalt fiber-reinforced asphalt mixture was comprehensively evaluated based on the response surface methodology. Furthermore, the fatigue behavior of basalt fiber-reinforced asphalt mixtures was investigated under the optimum basalt fiber content. This study provides references for the asphalt pavement structure and material design in extremely cold and high-altitude areas. The main conclusions are as follows:
1) The high-temperature properties of asphalt can be enhanced by basalt fiber, and the rutting resistance of asphalt can also be enhanced by aging. The dire influence of freeze-thaw cycles on bending and tensile intensity can be offset when aging or basalt fibers are incorporated.
2) The low-temperature properties of asphalt can be enhanced by basalt fiber. In contrast, aging can improve the bending and tensile intensity of asphalt, while the freeze-thaw cycle has a stimulating negative effect on the low-temperature properties of asphalt. Adverse effects of freeze-thaw cycles on flexural and tensile strength, when aged or incorporated into basalt fibers, can be counteracted.
3) The strength and fatigue performance of asphalt mixture are enhanced by basalt fiber. Aging and the freeze-thaw cycle have an apparent adverse effect on fatigue performance; short aging times (4 h), freeze-thaw cycles under 10 times, and basalt fibers can offset their harmful impact on the asphalt mixture fatigue life. In general, the effect of aging on the fatigue life of the asphalt mixture is greater than that of the freeze-thaw cycle under the conditions of this study.
4) The influence of the basalt fiber content on asphalt pavement properties and aging time was studied, as well as the times of freeze-thaw cycles. However, the selected asphalt grade is single, and the fiber type is single, which is not suitable for all asphalt pavement in alpine regions. Asphalt pavement involves more complex applicable conditions, so it needs a more comprehensive evaluation of its life evolution law.
Data Availability Statement
The original contributions presented in the study are included in the article/Supplementary Material; further inquiries can be directed to the corresponding authors.
Author Contributions
RL: conception, methodology and writing—review and editing. WY: visualization of data and design of the study. ZL: interpretation of the data, experimental works, and translation.
Conflict of Interest
RL was employed by Hunan Provincial Communications Planning, Survey, and Design Institute Co., Ltd.
WY and ZL were employed by the company, Changsha Institute of Technology Testing and Consulting Co., Ltd.
Publisher’s Note
All claims expressed in this article are solely those of the authors and do not necessarily represent those of their affiliated organizations, or those of the publisher, the editors, and the reviewers. Any product that may be evaluated in this article, or claim that may be made by its manufacturer, is not guaranteed or endorsed by the publisher.
Acknowledgments
The authors would like to thank all our enthusiastic colleagues, editors, and reviewers who provided guidance and advice on this article.
References
Abdelsalam, M., Yue, Y., Khater, A., Luo, D., Musanyufu, J., and Qin, X. (2020). Laboratory Study on the Performance of Asphalt Mixes Modified with a Novel Composite of Diatomite Powder and Lignin Fiber. Appl. Sci. 10, 5517. doi:10.3390/app10165517
Badeli, S., Carter, A., and Doré, G. (2018). Complex Modulus and Fatigue Analysis of Asphalt Mix after Daily Rapid Freeze-Thaw Cycles. J. Mater. Civ. Eng. 4, 1–13. doi:10.1061/(asce)mt.1943-5533.0002236
Chen, F., Huang, C., Wang, J., and Gao, D. (2019). Experimental Analysis on Flexural-Tensile Performance of Polyester Fiber Asphalt Concrete. Acsm 43, 81–88. doi:10.18280/acsm.430203
Cheng, Y., Chai, C., Zhang, Y., Chen, Y., and Zhu, B. (2019a). A New Eco-Friendly Porous Asphalt Mixture Modified by Crumb Rubber and Basalt Fiber. Sustainability 11, 5754. doi:10.3390/su11205754
Cheng, Y., Li, L., Zhang, Y., Lv, Z., and Zhu, C. (2019b). Quantitative Analysis of Effect and Interaction of Diatomite and Basalt Fiber on Asphalt Performance. J. Mater. Civ. Eng. 12. doi:10.1061/(asce)mt.1943-5533.0002948
Cheng, Y., Li, L., Zhou, P., Zhang, Y., and Liu, H. (2019c). Multi-Objective Optimization Design and Test of Compound Diatomite and Basalt Fiber Asphalt Mixture. Materials 12, 1461. doi:10.3390/ma12091461
Cheng, Y., Wang, W., Gong, Y., Wang, S., Yang, S., and Sun, X. (2018a). Comparative Study on the Damage Characteristics of Asphalt Mixtures Reinforced with an Eco-Friendly Basalt Fiber under Freeze-Thaw Cycles. Materials 11, 2488. doi:10.3390/ma11122488
Cheng, Y., Yu, D., Tan, G., and Zhu, C. (2018b). Low-Temperature Performance and Damage Constitutive Model of Eco-Friendly Basalt Fiber-Diatomite-Modified Asphalt Mixture under Freeze-Thaw Cycles. Materials 11, 2148. doi:10.3390/ma11112148
Fan, Z., Xu, H., Xiao, J., and Tan, Y. (2020). Effects of Freeze-Thaw Cycles on Fatigue Performance of Asphalt Mixture and Development of Fatigue-Freeze-Thaw (FFT) Uniform Equation. Constr. Build. Mater. 242, 118043. doi:10.1016/j.conbuildmat.2020.118043
Fu, Z., Tang, Y., Ma, F., Wang, Y., Shi, K., Dai, J., et al. (2022). Rheological Properties of Asphalt Binder Modified by Nano-TiO2/ZnO and Basalt Fiber. Constr. Build. Mater. 320, 126323. doi:10.1016/j.conbuildmat.2022.126323
Gong, Y., Song, J., Bi, H., and Tian, Z. (2020). Optimization Design of the Mix Ratio of a Nano-TiO2/CaCO3-Basalt Fiber Composite Modified Asphalt Mixture Based on Response Surface Methodology. Appl. Sci. 10, 4596. doi:10.3390/app10134596
Guo, F., Li, R., Lu, S., Bi, Y., and He, H. (2020). Evaluation of the Effect of Fiber Type, Length, and Content on Asphalt Properties and Asphalt Mixture Performance. Materials 13, 1556. doi:10.3390/ma13071556
Hamzah, M. O., Teh, S. Y., Golchin, B., and Voskuilen, J. (2017). Use of Imaging Technique and Direct Tensile Test to Evaluate Moisture Damage Properties of Warm Mix Asphalt Using Response Surface Method. Constr. Build. Mater. 132, 323–334. doi:10.1016/j.conbuildmat.2016.11.092
Huang, T., He, H., Zhang, P., Lv, S., Jiang, H., Liu, H., et al. (2022). Laboratory Investigation on Performance and Mechanism of Polyphosphoric Acid Modified Bio-Asphalt. J. Clean. Prod. 333, 130104. doi:10.1016/j.jclepro.2021.130104
Lavasani, M., Latifi Namin, M., and Fartash, H. (2015). Experimental Investigation on Mineral and Organic Fibers Effect on Resilient Modulus and Dynamic Creep of Stone Matrix Asphalt and Continuous Graded Mixtures in Three Temperature Levels. Constr. Build. Mater. 95, 232–242. doi:10.1016/j.conbuildmat.2015.07.146
Liu, J., Lv, S., Peng, X., and Yang, S. (2021). Improvements on Performance of Bio-Asphalt Modified by castor Oil-Based Polyurethane: An Efficient Approach for Bio-Oil Utilization. Constr. Build. Mater. 305, 124784. doi:10.1016/j.conbuildmat.2021.124784
Liu, L., Liu, Z., and Liu, J. (2020). Effects of Silane-Coupling Agent Pretreatment on Basalt Fibers: Analyzing the Impact on Interfacial Properties and Road Performance. J. Mater. Civ. Eng. 4, 1–8. doi:10.1061/(asce)mt.1943-5533.0003097
Lv, S., Liu, J., Peng, X., and Jiang, M. (2021a). Laboratory Experiments of Various Bio-Asphalt on Rheological and Microscopic Properties. J. Clean. Prod. 320, 128770. doi:10.1016/j.jclepro.2021.128770
Lv, S., Liu, J., Peng, X., Liu, H., Hu, L., Yuan, J., et al. (2021b). Rheological and Microscopic Characteristics of Bio-Oil Recycled Asphalt. J. Clean. Prod. 295, 126449. doi:10.1016/j.jclepro.2021.126449
Lv, S., Yuan, J., Peng, X., Borges Cabrera, M., Liu, H., Luo, X., et al. (2021c). Standardization to Evaluate the Lasting Capacity of Rubberized Asphalt Mixtures with Different Testing Approaches. Constr. Build. Mater. 269, 121341. doi:10.1016/j.conbuildmat.2020.121341
Ma, L., Wang, F., Cui, P., Yunusa, M., and Xiao, Y. (2021). Effect of Aging on the Constitutive Models of Asphalt and Their Mixtures. Constr. Build. Mater. 272, 121611. doi:10.1016/j.conbuildmat.2020.121611
Nian, T., Li, P., Mao, Y., Zhang, G., and Liu, Y. (2018a). Connections between Chemical Composition and Rheology of Aged Base Asphalt Binders during Repeated Freeze-Thaw Cycles. Constr. Build. Mater. 159, 338–350. doi:10.1016/j.conbuildmat.2017.10.097
Nian, T., Li, P., Wei, X., Wang, P., Li, H., and Guo, R. (2018b). The Effect of Freeze-Thaw Cycles on Durability Properties of SBS-Modified Bitumen. Constr. Build. Mater. 187, 77–88. doi:10.1016/j.conbuildmat.2018.07.171
Omranian, S. R., Hamzah, M. O., Valentin, J., and Hasan, M. R. M. (2018). Determination of Optimal Mix from the Standpoint of Short Term Aging Based on Asphalt Mixture Fracture Properties Using Response Surface Method. Constr. Build. Mater. 179, 35–48. doi:10.1016/j.conbuildmat.2018.05.078
Parchami, A., Nourbakhsh, M., and Mashinchi, M. (2017). Analysis of Variance in Uncertain Environments. Complex Intell. Syst. 3, 189–196. doi:10.1007/s40747-017-0046-8
Pouranian, M. R., and Haddock, J. E. (2020). Effect of Aggregate Gradation on Asphalt Mixture Compaction Parameters. J. Mater. Civ. Eng. 9, 1–17. doi:10.1061/(asce)mt.1943-5533.0003315
Qu, X., Liu, Q., Guo, M., Wang, D., and Oeser, M. (2018). Study on the Effect of Aging on Physical Properties of Asphalt Binder from a Microscale Perspective. Constr. Build. Mater. 187, 718–729. doi:10.1016/j.conbuildmat.2018.07.188
Saha, G., and Biligiri, K. P. (2016). Cracking Performance Analysis of Asphalt Mixtures Using Response Surface Methodology: Experimental Investigations and Statistical Optimization. Mater Struct. 50, 33. doi:10.1617/s11527-016-0906-5
Sirin, O., Paul, D. K., Khan, M. S., Kassem, E., and Darabi, M. K. (2019). Effect of Aging on Viscoelastic Properties of Asphalt Mixtures. J. Transp. Eng. Part B Pavements 145, 04019034. doi:10.1061/jpeodx.0000137
Tang, Y., Feng, W., Chen, Z., Nong, Y., Guan, S., and Sun, J. (2021). Fracture Behavior of a Sustainable Material: Recycled Concrete with Waste Crumb Rubber Subjected to Elevated Temperatures. J. Clean. Prod. 318, 128553. doi:10.1016/j.jclepro.2021.128553
Wang, S., Kang, A., Xiao, P., Li, B., and Fu, W. (2019). Investigating the Effects of Chopped Basalt Fiber on the Performance of Porous Asphalt Mixture. Adv. Mater. Sci. Eng. 2019, 1–12. doi:10.1155/2019/2323761
Wang, W., Cheng, Y., and Tan, G. (2018). Design Optimization of SBS-Modified Asphalt Mixture Reinforced with Eco-Friendly Basalt Fiber Based on Response Surface Methodology. Materials 11, 1311. doi:10.3390/ma11081311
Wu, H., Li, P., Nian, T., Zhang, G., He, T., and Wei, X. (2019). Evaluation of Asphalt and Asphalt Mixtures' Water Stability Method under Multiple Freeze-Thaw Cycles. Constr. Build. Mater. 228, 117089. doi:10.1016/j.conbuildmat.2019.117089
Wu, J.-r., Li, F., and Ma, Q.-y. (2020). Effect of Polyester Fiber on Air Voids and Low-Temperature Crack Resistance of Permeable Asphalt Mixture. Adv. Civ. Eng. 2020, 1–12. doi:10.1155/2020/2381504
Wu, Z., Zhang, C., Xiao, P., Li, B., and Kang, A. (2020). Performance Characterization of Hot Mix Asphalt with High RAP Content and Basalt Fiber. Materials 13, 3145. doi:10.3390/ma13143145
Xing, X., Pei, J., Shen, C., Li, R., Zhang, J., Huang, J., et al. (2019). Performance and Reinforcement Mechanism of Modified Asphalt Binders with Nano-Particles, Whiskers, and Fibers. Appl. Sci. 9, 2995. doi:10.3390/app9152995
Yunchao, T., Zheng, C., Wanhui, F., Yumei, N., Cong, L., and Jieming, C. (2021). Combined Effects of Nano-Silica and Silica Fume on the Mechanical Behavior of Recycled Aggregate Concrete. Nanotechnol. Rev. 10, 819–838. doi:10.1515/ntrev-2021-0058
Zhang, Q., Cai, X., Wu, K., Chen, L., Zhang, R., Xiao, H., et al. (2021). Shear Performance of Recycled Asphalt Mixture Based on Contact Interface Parameter Analysis. Constr. Build. Mater. 300, 124049. doi:10.1016/j.conbuildmat.2021.124049
Zhang, X., Gu, X., Lv, J., and Zou, X. (2017). 3D Numerical Model to Investigate the Rheological Properties of Basalt Fiber Reinforced Asphalt-like Materials. Constr. Build. Mater. 138, 185–194. doi:10.1016/j.conbuildmat.2017.01.110
Zhang, Z., Han, S., Han, X., Dong, S., and Yao, T. (2021). Performance Changes of Hot Recycled Asphalt Mixture in Different Layers under Coupling of Multiple Aging Factors. Constr. Build. Mater. 269, 121343. doi:10.1016/j.conbuildmat.2020.121343
Zhao, H., Guan, B., Xiong, R., and Zhang, A. (2020). Investigation of the Performance of Basalt Fiber Reinforced Asphalt Mixture. Appl. Sci. 10, 1561. doi:10.3390/app10051561
Zhou, T., Kabir, S. F., Cao, L., Luan, H., Dong, Z., and Fini, E. H. (2020). Comparing Effects of Physisorption and Chemisorption of Bio-Oil onto Rubber Particles in Asphalt. J. Clean. Prod. 273, 123112. doi:10.1016/j.jclepro.2020.123112
Keywords: basalt fiber-reinforced asphalt mixture, aging, the freeze-thaw cycle, response surface methodology, fatigue
Citation: Liang R, Yu W and Luo Z (2022) Laboratory Investigation on Pavement Performance of Basalt Fiber-Reinforced Asphalt Mixture Under the Coupling Effect of Freeze-Thaw Cycles and Aging. Front. Mater. 9:930056. doi: 10.3389/fmats.2022.930056
Received: 27 April 2022; Accepted: 09 June 2022;
Published: 12 July 2022.
Edited by:
Nicola Maria Pugno, University of Trento, ItalyReviewed by:
Liseane Padilha Thives, Federal University of Santa Catarina, BrazilYunchao Tang, Zhongkai University of Agriculture and Engineering, China
Copyright © 2022 Liang, Yu and Luo. This is an open-access article distributed under the terms of the Creative Commons Attribution License (CC BY). The use, distribution or reproduction in other forums is permitted, provided the original author(s) and the copyright owner(s) are credited and that the original publication in this journal is cited, in accordance with accepted academic practice. No use, distribution or reproduction is permitted which does not comply with these terms.
*Correspondence: Wei Yu, 820276413@qq.com; Zengjie Luo, 584574448@qq.com