- 1Laboratory of Biocomposite Technology, Institute of Tropical Forestry and Forest Products, Universiti Putra Malaysia, UPM Serdang, Selangor, Malaysia
- 2Department of Chemistry, Faculty of Science, Universiti Putra Malaysia, UPM Serdang, Selangor, Malaysia
- 3Centre of Foundation Studies for Agricultural Science, Universiti Putra Malaysia, UPM Serdang, Selangor, Malaysia
Increased environmental awareness has encouraged researchers to seek alternatives to replace the use of hazardous chemicals in the extraction of nanocellulose for environmental conservation. Lately, ionic liquids have been used as a medium for processing cellulose and other biopolymers because they are recyclable and reusable. Recently, ionic liquids have been used to extract nanocellulose for a variety of applications. In this short review, examples of ionic liquids that are usually used for the extraction of nanocellulose are demonstrated. Additionally, sources of cellulose that are employed as raw materials for nanocellulose have also been shown. Moreover, the synthesis of ionic liquids and the process of nanocellulose extraction using ionic liquids are exhibited in this paper. In addition, the properties of nanocellulose extracted by different ionic liquids were also shortly reviewed. On top of that, the knowledge gained from this review provides a clearer comprehension of the process of nanocellulose extraction and the important properties of the extracted nanocellulose. In conclusion, this short review revealed that different mechanisms of nanocellulose extraction using ionic liquids give distinct properties to the extracted nanocellulose.
Introduction
Nanocellulose is a cellulose with nanometric dimensions. Nano dimensional cellulose has turned out to be an interesting material to study due to its larger surface area with superior properties (Sable et al., 2014; Tan et al., 2015; Babicka et al., 2021). The dimensions of nanocellulose are smaller because of the removal of the amorphous phase of cellulose particles (Chowdhury and Hamid, 2016). Other terminologies for nanocellulose are cellulose nanocrystals, nanocrystalline cellulose, cellulose nanowhiskers, etc. Nanocellulose can own at least dimensions of 50 nm in length and 14 nm in diameter, and crystallinity higher than 80% (Gonçalves et al., 2018). Low dimensions of nanocellulose can be obtained by optimizing the process of nanocellulose extraction (Haron et al., 2021). Many processes are used to extract nanocellulose from various lignocellulose materials, involving the physical processes, such as grinding, grating, etc. (Sable et al., 2014; Babicka et al., 2021). In addition, the chemical processes using acids and alkalis have also been utilized for extracting nanocellulose from cellulose–hemicellulose–lignin complex (Grząbka-Zasadzińska et al., 2017; Samsudin et al., 2020). Most recently, the use of ionic liquids in the extraction of nanocellulose has increased because they are often regarded as green solvents, which possess some versatile properties, for example reusable, non-corrosive, non-volatile, non-flammable, etc. (Shamsuri and Md. Jamil, 2020; Shamsuri et al., 2021a).
Ionic liquids are organic salts with a melting point below 100°C and composed entirely of ions (Grząbka-Zasadzińska et al., 2017; Low et al., 2020), they also have good chemical and thermal stabilities. Nowadays, ionic liquids have extensively been used in the dissolution of cellulose and other biopolymers. In addition, the excellent interaction between ionic liquids and biopolymers has provided biopolymer solutions that can be employed in many applications, for instance biopolymer blend, biopolymer composite, biopolymer modification, and so forth (Shamsuri et al., 2021b; Shamsuri and Jamil, 2021). The use of ionic liquids for extracting nanocellulose is an alternative approach because it can avoid the usage of non-environmentally friendly chemicals like mineral acids that require huge volumes of basic solutions for the neutralization process (Onkarappa et al., 2020a). Table 1 shows examples of ionic liquids used for the extraction of nanocellulose. It can be observed that ionic liquids with imidazolium cations are typically used compared to other organic cations. This is due to the fact that they are easily available, and they also have unique properties for use in several usages (Shamsuri et al., 2021d). Moreover, different counter anions, such as chloride, acetate, and hydrogen sulfate are frequently selected for that purpose because of the effectiveness of these anions.
In addition, the preceding studies revealed that [Bmim][Cl], [Emim][OAc], and [Bmim][HSO4] are repeatedly used for the extraction of nanocellulose (Li et al., 2012; Wang et al., 2013; Wang et al., 2015; Xiao et al., 2015; Vinogradova and Chen, 2016; Phanthong et al., 2017; Wang et al., 2017; Al Hakkak et al., 2019; BABICKA et al., 2019; Chowdhury et al., 2019; Dai et al., 2019; Hernani et al., 2019; Huang et al., 2019; Samsudin et al., 2020; Onkarappa et al., 2020b; Huang et al., 2020; Mohd Ishak et al., 2020; Jordan et al., 2020; Haron et al., 2022). Figure 1 exhibits the chemical structures of [Bmim][Cl], [Emim][OAc], and [Bmim][HSO4]. These ionic liquids have different counter anions that give distinct mechanisms for extracting nanocellulose. The mechanism of nanocellulose extraction in [Bmim][Cl] and [Emim][OAc] are involved the dissolution process. [Bmim][Cl] and [Emim][OAc] can disrupt the hydrogen bonding network among cellulose chains, causing the dissolution of cellulose and giving a homogeneous solution (Li et al., 2012; Shamsuri et al., 2021e). On the other hand, the nanocellulose extraction mechanism in [Bmim][HSO4] is involved the swelling process. [Bmim][HSO4] can swell cellulose by increasing the intermolecular distance between the cellulose chains (Chowdhury et al., 2019) and making the extraction of nanocellulose a heterogeneous process (Huang et al., 2019). Therefore, different counter anions of ionic liquids can play an important role in the dissolution and swelling processes of cellulose (Babicka et al., 2021) for extracting nanocellulose.
Numerous sources of cellulose have been employed as raw materials for nanocellulose. Table 2 displays examples of celluloses and ionic liquids used for the extraction of nanocellulose. It can be seen that a wide variety of celluloses from different sources were successfully employed as nanocellulose resources. Besides that, in the last 10 years, several ionic liquids have been used in the extraction of nanocellulose with the intention of increasing the use of ionic liquids and reducing the usage of hazardous chemicals. This can provide a safe workplace and subsequently conserve the environment. The use of ionic liquids as extracting agents can also give other advantages, as stated earlier. Additionally, variations of ionic liquids can provide some options for researchers to choose from to match nanocellulose applications. Hitherto, to the best of the authors’ knowledge, there is no short review has been made, focusing on the syntheses, processes, and properties of nanocellulose extracted by ionic liquids. That is the primary objective of creating a structured review in this paper. Moreover, this short review is narrow, and even though not broad, it is still relevant to other present studies.
Syntheses of Ionic Liquids
Chloride Anion-Based Ionic Liquids
Chloride anion-based ionic liquids can be synthesized via alkylation reaction (Shamsuri et al., 2021f) by reacting N-alkylimidazole with an alkylating agent, such as alkyl chloride. Figure 2 presents the schematic of the alkylation reaction of N-alkylimidazole with alkyl chloride to prepare N-alkyl-N-alkylimidazolium chloride ionic liquid. The alkylation reaction of N-alkylimidazole is commonly carried out under reflux condition for overnight at elevated temperature with stirring (Onkarappa et al., 2020a; Onkarappa et al., 2020b). A polar aprotic solvent like acetonitrile can be employed as a medium for such a reaction (Babicka et al., 2020). In addition, [Emim][Cl], [Pmim][Cl], and [Bmim][Cl] are successfully prepared by means of this reaction. However, [Bmim][Cl] is typically used by most researchers for the extraction of nanocellulose compared to other chloride anion-based ionic liquids, this may be due to its ease of finding and low price.
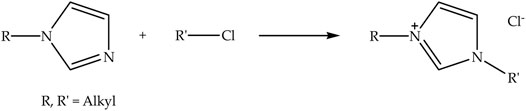
FIGURE 2. Schematic of the alkylation reaction of N-alkylimidazole with alkyl chloride to prepare N-alkyl-N-alkylimidazolium chloride ionic liquid.
Acetate Anion-Based Ionic Liquids
Acetate anion-based ionic liquids can be synthesized through metathesis reaction (Shamsuri et al., 2021e) by exchanging chloride anion of N-alkyl-N-alkylimidazolium with an acetate anion using potassium acetate. Figure 3 shows the schematic of the metathesis reaction of N-alkyl-N-alkylimidazolium chloride with potassium acetate to prepare N-alkyl-N-alkylimidazolium acetate ionic liquid. The metathesis reaction of N-alkyl-N-alkylimidazolium chloride is usually conducted at room temperature with stirring (Shamsuri et al., 2021e; Shamsuri et al., 2021f). Moreover, a polar solvent, such as ethanol, is generally employed as a medium for the reaction. Besides that, potassium chloride is also produced from the reaction; nevertheless, it can easily be removed because it is insoluble in ethanol (Shamsuri et al., 2021f). [Emim][OAc] is often used for nanocellulose extraction as it requires moderate temperature (Al Hakkak et al., 2019).

FIGURE 3. Schematic of the metathesis reaction of N-alkyl-N-alkylimidazolium chloride with potassium acetate to prepare N-alkyl-N-alkylimidazolium acetate ionic liquid.
Hydrogen Sulfate Anion-Based Ionic Liquids
Hydrogen sulfate anion-based ionic liquids can be synthesized via neutralization reaction (Shamsuri et al., 2021a) by reacting N-alkyl-N-alkylimidazolium hydroxide with a mineral acid, in particular sulfuric acid (Grząbka-Zasadzińska et al., 2019). Figure 4 displays the schematic of the neutralization reaction of N-alkyl-N-alkylimidazolium hydroxide with sulfuric acid to prepare N-alkyl-N-alkylimidazolium hydrogen sulfate ionic liquid. The neutralization reaction of N-alkyl-N-alkylimidazolium hydroxide is normally performed by dripping dilute sulfuric acid at a low temperature with stirring (Gonçalves et al., 2018). Water has also been produced from the reaction. Nonetheless, it can straightforwardly be removed by evaporation at elevated temperature under vacuum. Meanwhile, one of the most widely used hydrogen sulfate anion-based ionic liquids for the extraction of nanocellulose is [Bmim][HSO4].

FIGURE 4. Schematic of the neutralization reaction of N-alkyl-N-alkylimidazolium hydroxide with sulfuric acid to prepare N-alkyl-N-alkylimidazolium hydrogen sulfate ionic liquid.
Processes of Nanocellulose Extraction
Dissolution Process
In the dissolution process for extracting nanocellulose, either chloride or acetate anion of ionic liquids interacts well with the hydroxyl groups of cellulose via hydrogen bonding, disrupting the strong intermolecular hydrogen bonding that exists between cellulose chains, consequently inducing the dissolution of cellulose (Onkarappa et al., 2020b). Figure 5 demonstrates the mechanism of the dissolution process of cellulose with chloride or acetate anion-based ionic liquids. Some aspects can affect the effectiveness of dissolution, for example the weight ratio of cellulose to ionic liquid, the dissolution temperature, and the dissolution time (Li et al., 2012). Table 3 indicates ionic liquids, dissolution temperatures, dissolution times, anti-solvents, agitation, and separation processes are applied for the extraction of nanocellulose. It can be perceived that the dissolution time of cellulose decreased with increasing temperature (Wang et al., 2013), especially for [Bmim][Cl] and [Emim][OAc]. However, the dissolution time also depends on the weight ratio of cellulose to ionic liquid. In that case, the dissolution time of cellulose continuously increased with increasing cellulose weight. After the dissolution process has finished, an anti-solvent was added into the cellulose/ionic liquid solution, and instantly the solution converted into a suspension containing cellulose precipitates (Al Hakkak et al., 2019).
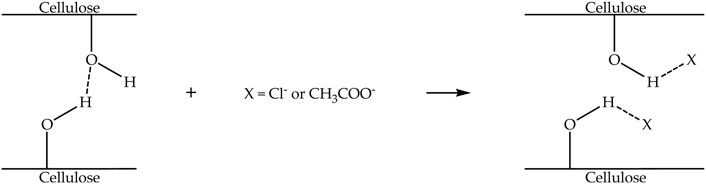
FIGURE 5. Mechanism of the dissolution process of cellulose with chloride or acetate anion-based ionic liquids.
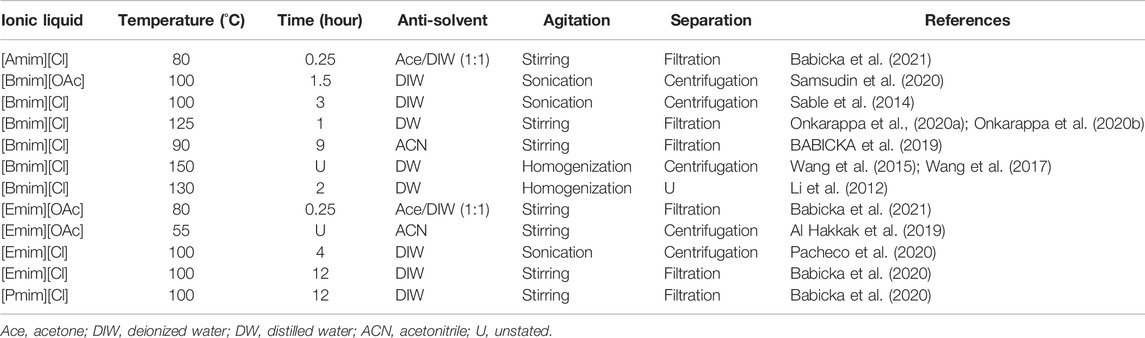
TABLE 3. Ionic liquids, dissolution temperatures, dissolution times, anti-solvents, agitation, and separation processes are applied for the extraction of nanocellulose.
The addition of anti-solvent drastically reduced the solvation of cellulose in ionic liquid. This is because anti-solvent weakened the hydrogen bonding between ionic liquid and cellulose. Water, acetonitrile, and acetone/water mixture are commonly utilized as anti-solvents, nevertheless, water is the most preferred as it is the safest and least expensive anti-solvent. In Table 3, it can also be seen that the agitation process like stirring, sonication, or homogenization has been done during or after the addition of anti-solvent for splitting cellulose into smaller dimensions. Meanwhile, the separation process is a final procedure for isolating nanocellulose from ionic liquid/anti-solvent solution. Therefore, the separation processes, such as filtration and centrifugation, can be applied for such a purpose. On top of that, the extracted nanocellulose that is separated via the filtration process is usually washed with cold distilled water (Onkarappa et al., 2020a; Onkarappa et al., 2020b), acetonitrile (BABICKA et al., 2019; Babicka et al., 2020), or acetone/water mixture (Babicka et al., 2021) to remove residual ionic liquid. Besides that, the extracted nanocellulose that separated through the centrifugation process can also be washed with deionized water (Sable et al., 2014; Pacheco et al., 2020; Samsudin et al., 2020) and acetonitrile (Al Hakkak et al., 2019).
Swelling Process
The swelling process of cellulose in ionic liquids for the extraction of nanocellulose is almost similar to the dissolution process. Nevertheless, in the swelling process, hydrogen sulfate anion of ionic liquids diffuses into the intermolecular space between the cellulose chains, subsequently increasing their distance, causing the swelled formation of cellulose (Low et al., 2020). Figure 6 indicates the mechanism of the swelling process of cellulose with hydrogen sulfate anion-based ionic liquids. Table 4 exhibits ionic liquids, swelling temperatures, swelling times, anti-solvents, agitation, and separation processes are applied for the extraction of nanocellulose. It can be observed that the swelling temperatures of cellulose in [Bmim][HSO4] are from 90 to 120°C. Additionally, the minimum swelling time of cellulose is 0.75 h, and the maximum swelling time is 12 h, depending on the cellulose weight. An anti-solvent was also added into the cellulose/ionic liquid suspension once the swelling process was completed, and off-white cellulose precipitates formed immediately (Haron et al., 2021). The addition of anti-solvent radically rearranged the cellulose chains, which induced the precipitation of cellulose.
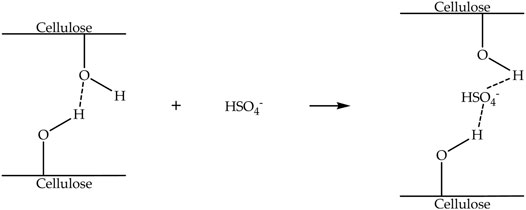
FIGURE 6. Mechanism of the swelling process of cellulose with hydrogen sulfate anion-based ionic liquids.
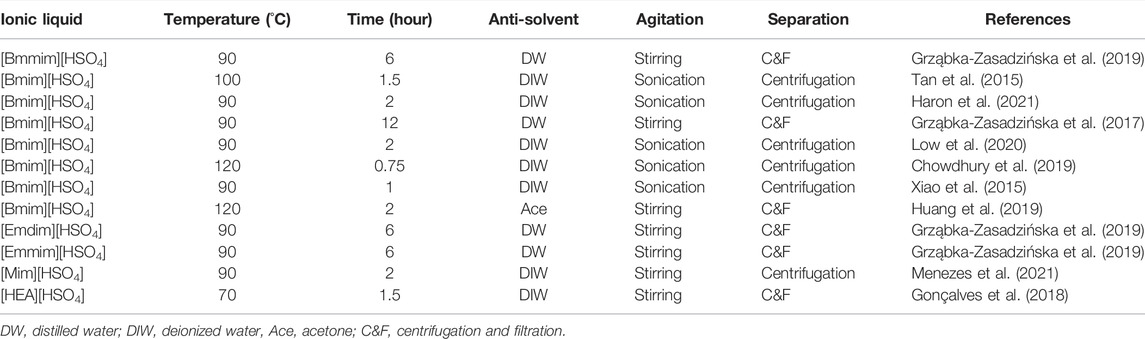
TABLE 4. Ionic liquids, swelling temperatures, swelling times, anti-solvents, agitation, and separation processes are applied for the extraction of nanocellulose.
It can also be seen in Table 4 that water is the most commonly utilized as an anti-solvent compared to an organic solvent. Besides that, the agitation processes, such as stirring and sonication, have been performed during or after the addition of anti-solvent same as the dissolution process. Moreover, the separation processes, such as centrifugation and a combination of centrifugation and filtration, have also been applied for isolating nanocellulose from ionic liquid/anti-solvent solution. Meanwhile, the residual ionic liquid can be removed from the extracted nanocellulose by washing with deionized water (Tan et al., 2015; Xiao et al., 2015; Gonçalves et al., 2018; Chowdhury et al., 2019; Haron et al., 2021) or distilled water (Grząbka-Zasadzińska et al., 2017; Huang et al., 2019) until a neutral pH. On top of that, the ionic liquid used in the swelling and dissolution processes for the extraction of nanocellulose can be recovered by evaporating anti-solvent, followed by freeze-drying (Tan et al., 2015; Samsudin et al., 2020). The recovery yield of ionic liquid usually is high, which is more than 90% (Tan et al., 2015; Low et al., 2020). In addition, unlike mineral acids, the ionic liquid used for extracting nanocellulose can also be recycled and reused at least five times (Huang et al., 2019).
Properties of Extracted Nanocellulose
Properties of Nanocellulose Extracted by Chloride Anion-Based Ionic Liquids
Table 5 shows the properties of nanocellulose extracted by chloride anion-based ionic liquids. The nanocellulose has been extracted from rubber wood cellulose using [Bmim][Cl] (Onkarappa et al., 2020a). The chemical, crystalline, morphological, and thermal properties of the extracted nanocellulose were characterized by Fourier transform infrared spectrometer, X-ray diffractometer, scanning electron microscope, and thermal gravimetric analyzer. The chemical property, such as the infrared spectrum of the extracted nanocellulose indicated that the lignin vibrational peaks are marginally disappeared compared to the rubber wood cellulose. This was due to the residual lignin being removed by [Bmim][Cl] after the dissolution of rubber wood cellulose. However, the crystalline property, such as the intensity of the X-ray diffraction peak of the extracted nanocellulose is slightly broader compared to the rubber wood cellulose. This was attributed to the crystallinity index decreased because of the dissolution of cellulose crystalline regions in [Bmim][Cl]. Besides that, the morphological property, such as the average size of the extracted nanocellulose is between 200 and 300 nm with a fibrous shape and partly translucent character (Onkarappa et al., 2020a). This confirmed that the size of the extracted nanocellulose is in the nanoscale dimension. On the other hand, the thermal property, such as the degradation temperature of the extracted nanocellulose is significantly unchanged, which is the same as the nanocellulose extracted by acid hydrolysis. This suggested that both nanocelluloses have similar thermal stability. Therefore, it can be concluded that the use of [Bmim][Cl] provides rubber wood nanocellulose with low crystallinity and fibrous character.
Meanwhile, the nanocellulose has been extracted from maize husk cellulose using [Bmim][Cl] (Onkarappa et al., 2020b). The chemical, crystalline, morphological, and thermal properties of the extracted nanocellulose were characterized by Fourier transform infrared spectrometer, X-ray diffractometer, transmission electron microscope, and thermal gravimetric analyzer. The chemical property, such as the infrared absorption peaks of the extracted nanocellulose shifted to higher wavenumbers compared to the maize husk cellulose, which exhibited the transition from cellulose I to cellulose II. This verified that during the dissolution process, no other derivational reactions occurred. Nevertheless, the crystalline property, such as the intensity of the X-ray diffraction peak of the extracted nanocellulose is considerably decreased compared to the standard nanocellulose. This was ascribed to [Bmim][Cl] broke the intermolecular and intramolecular hydrogen bonding of cellulose. Furthermore, the morphological property, such as the average size of the extracted nanocellulose is 25.02 nm with a fibrous shape and small nanobundles (Onkarappa et al., 2020b). This proved that the size of the extracted nanocellulose is in the nanoscale dimension. In contrast, the thermal property, such as the degradation temperature of the extracted nanocellulose is higher compared to the nanocellulose extracted from rubber wood cellulose with the same processes using the same kind of ionic liquid. This implied that the extracted nanocellulose has exceptional thermal stability. Hence, it can be inferred that the use of [Bmim][Cl] gives maize husk nanocellulose with low crystallinity and high thermal stability.
The nanocellulose has been extracted from cotton cellulose using [Bmim][Cl] (Wang et al., 2013). The chemical, crystalline, morphological, and thermal properties of the extracted nanocellulose were characterized by Fourier transform infrared spectrometer, X-ray diffractometer, transmission electron microscope, and thermal gravimetric analyzer. The chemical property, such as the infrared spectrum of the extracted nanocellulose is almost the same as the spectrum of the original cellulose. This validated that no other derivational reactions occurred during the dissolution and refining processes. Nonetheless, the crystalline property, such as the crystallinity index of the extracted nanocellulose is decreased compared to the original cellulose. This was caused by the hydrogen bonding of cellulose broken, which induced the collapse of the crystal structure. Additionally, the morphological property, such as the particle size of the extracted nanocellulose is about 20 nm (Wang et al., 2013). This demonstrated that the size of the extracted nanocellulose can be achieved on the scale of a nanometer. Besides, the thermal property, such as the onset decomposition temperature of the extracted nanocellulose is lower compared to the original cellulose. Such an occurrence was because the crystal region between cellulose was destroyed in the extracted nanocellulose. Thus, it can be deduced that the use of [Bmim][Cl] grants cotton nanocellulose with low crystallinity and low thermal stability.
On top of that, the nanocellulose has been extracted from Eucalyptus pulp cellulose using [Bmim][Cl] (Wang et al., 2017). The chemical, crystalline, morphological, and thermal properties of the extracted nanocellulose were characterized by Fourier transform infrared spectrometer, X-ray diffractometer, transmission electron microscope, and thermal gravimetric analyzer. The chemical property, such as the infrared absorption peak of the extracted nanocellulose slightly moved to the blue shift compared to the Eucalyptus pulp cellulose. This displayed that the chemical identity of the extracted nanocellulose is preserved. However, the crystalline property, such as the crystallinity index of the extracted nanocellulose is marginally reduced compared to the Eucalyptus pulp cellulose. This was because the extracted nanocellulose turned out to be an amorphous region. In addition, the morphological property, such as the diameter size of the extracted nanocellulose is ranged from 20 to 100 nm, which is four times smaller compared to Eucalyptus pulp cellulose (900–1300 μm). This verified that the size of the extracted nanocellulose is in the nanoscale dimension. Moreover, the dispersion of the extracted nanocellulose is better than the nanocellulose extracted from cotton cellulose (Wang et al., 2017). Furthermore, the thermal property, such as the initial decomposition temperature of the extracted nanocellulose is lower compared to the original cellulose. This was attributed to the damage in the crystal region between cellulose chains. Therefore, it can be concluded that the use of [Bmim][Cl] provides Eucalyptus pulp nanocellulose with low crystallinity and low thermal stability.
Besides that, the nanocellulose has been extracted from sugarcane bagasse cellulose using [Bmim][Cl] (Li et al., 2012). The chemical, crystalline, morphological, and thermal properties of the extracted nanocellulose were characterized by Fourier transform infrared spectrometer, X-ray diffractometer, transmission electron microscope, and thermal gravimetric analyzer. The chemical property, such as the infrared spectrum of the extracted nanocellulose showed almost no difference from the spectrum of the original cellulose. This suggested that no other chemical reactions occurred during the processes of dissolution and refining. On the other hand, the crystalline property, such as the crystallinity index of the extracted nanocellulose is significantly decreased compared to the original cellulose. This was ascribed to the collapse of the crystal structure of the extracted nanocellulose, which was caused by the broken of the intermolecular hydrogen bonding. Nevertheless, the morphological property, such as the diameter size of the extracted nanocellulose is in the range of 10–20 nm with a circular shape (Li et al., 2012). This confirmed that the size of the extracted nanocellulose can be reached to the nano-level. Conversely, the thermal property, such as the onset decomposition temperature of the extracted nanocellulose is reduced compared to the original cellulose. This was due to the crystal region between cellulose chains is damaged. Hence, it can be inferred that the use of [Bmim][Cl] gives sugarcane bagasse nanocellulose with low crystallinity and low thermal stability.
The nanocellulose has been extracted from blueberry pruning cellulose using [Emim][Cl] (Pacheco et al., 2020). The chemical, crystalline, morphological, and thermal properties of the extracted nanocellulose were characterized by Fourier transform infrared spectrometer, X-ray diffractometer, atomic force microscope, and thermal gravimetric analyzer. The chemical property, such as the transmittance of the infrared absorption peak of the CH2 bending vibration, of the extracted nanocellulose is decreased compared to the raw cellulose, which was caused by the presence of cellulose II. This revealed that during the dissolution process, no other derivational reactions occurred. Nonetheless, the crystalline property, such as the intensity of the X-ray diffraction peak of the extracted nanocellulose is reduced compared to the nanocellulose extracted by TEMPO oxidation. This was owing to the decreased crystallinity index and crystallite size of the extracted nanocellulose. Additionally, the morphological property, such as the diameter size of the extracted nanocellulose is 22.4 ± 4.9 nm with an oval shape (Pacheco et al., 2020). This proved that the extraction process generated particles of cellulose at the nanoscale level. On the contrary, the thermal property, such as the degradation temperature of the extracted nanocellulose is higher compared to the nanocelluloses extracted by TEMPO oxidation and acid hydrolysis. This indicated that the extracted nanocellulose has good thermal stability. Thus, it can be deduced that the use of [Emim][Cl] grants blueberry pruning nanocellulose with low crystallinity and high thermal stability.
Properties of Nanocellulose Extracted by Acetate Anion-Based Ionic Liquids
Table 6 exhibits the properties of nanocellulose extracted by acetate anion-based ionic liquids. The nanocellulose has been extracted from microcrystalline cellulose using [Bmim][OAc] (Samsudin et al., 2020). The chemical, crystalline, morphological, and thermal properties of the extracted nanocellulose were characterized by Fourier transform infrared spectrometer, X-ray diffractometer, field emission scanning electron microscope, and thermal gravimetric analyzer. The chemical property, such as the infrared spectrum of the extracted nanocellulose is almost similar to the spectrum of the microcrystalline cellulose. This demonstrated that the chemical structure of the extracted nanocellulose remains unchanged after the extraction process. However, the crystalline properties, such as the crystallinity index and crystallite size of the extracted nanocellulose are significantly decreased compared to the microcrystalline cellulose. This was ascribed to the breakage of hydrogen bonding within the crystalline domains of the cellulose chains. On top of that, the morphological property, such as the shape of the extracted nanocellulose is cylindrical fibrils with a smooth surface and refined fibrillar structure (Samsudin et al., 2020). Furthermore, the hydrodynamic size of the extracted nanocellulose is as low as 59.34 nm. In contrast, the thermal property, such as the decomposition temperature of the extracted nanocellulose is slightly higher compared to the microcrystalline cellulose. This was because of the antiparallel arrangement in the cellulose chains, which improved the thermal stability. Therefore, it can be concluded that the use of [Bmim][OAc] provides microcrystalline nanocellulose with low crystallinity and high thermal stability.
Besides that, the nanocellulose has been extracted from microcrystalline cellulose using [Emim][OAc] (Babicka et al., 2021). The chemical, crystalline, morphological, and thermal properties of the extracted nanocellulose were characterized by Fourier transform infrared spectrometer, X-ray diffractometer, scanning electron microscope, and thermal gravimetric analyzer. The chemical property, such as the infrared spectrum of the extracted nanocellulose, revealed the absence of new bands. This validated that there was no chemical modification to the extracted nanocellulose. Nonetheless, the crystalline property, such as the crystallinity index of the extracted nanocellulose is reduced compared to the microcrystalline cellulose. This was caused by the cellulose chains broken during the extraction process with [Emim][OAc], which decreased the degree of crystallinity. Moreover, the morphological property, such as the shape of the extracted nanocellulose is regular with a spherical structure (Babicka et al., 2021). Additionally, the average particle size of the extracted nanocellulose is within the range of 30–200 nm. In addition, the thermal property, such as the onset decomposition temperature of the extracted nanocellulose is considerably lesser compared to the microcrystalline cellulose. This was owing to the greater surface area of the extracted nanocellulose exposed to high temperatures, which lessened the thermal properties. Hence, it can be inferred that the use of [Emim][OAc] gives microcrystalline nanocellulose with low crystallinity and low thermal stability.
The nanocellulose fiber has been extracted from wood pulp cellulose using [Emim][OAc] (Vinogradova and Chen, 2016). The crystalline, morphological, and thermal properties of the extracted nanocellulose fiber were characterized by wide angle X-ray diffractometer, scanning electron microscope, and thermal gravimetric analyzer. The crystalline properties, such as the crystallinity index and crystal size of the extracted nanocellulose fiber are marginally reduced compared to the nanocellulose fiber extracted by [Bmim][Cl]. This was due to there is no mechanical shearing of solution during the fiber extrusion because [Emim][OAc] has lower dissolving and spinning temperatures than that of [Bmim][Cl]. Besides that, the morphological property, such as the diameter size of the extracted nanocellulose fiber is within the range of 100–1000 nm with a fine surface structure (Vinogradova and Chen, 2016). This confirmed that the size of the extracted nanocellulose fiber is in the nanoscale dimension. On the contrary, the thermal property, such as the decomposition temperature of the extracted nanocellulose fiber is higher compared to the nanocellulose fiber extracted by [Bmim][Cl]. This indicated that the extracted nanocellulose fiber possessed better thermal stability than the nanocellulose fiber extracted by the chloride anion-based ionic liquid. Thus, it can be deduced that the use of [Emim][OAc] grants wood pulp nanocellulose fiber with low crystallinity and high thermal stability.
Meanwhile, the nanocellulose has been extracted from cellulose powder using [Emim][OAc] (Phanthong et al., 2017). The chemical, crystalline, morphological, and thermal properties of the extracted nanocellulose were characterized by Fourier transform infrared spectrometer, X-ray diffractometer, transmission electron microscope, and thermal gravimetric analyzer. The chemical property, such as the infrared spectrum of the extracted nanocellulose is almost identical to the spectrum of the cellulose powder. This displayed the similarity of their chemical structures and no changes in the functional groups after the extraction process. Nevertheless, the crystalline properties, such as the crystallinity percentage and crystal size of the extracted nanocellulose are lesser compared to the nanocellulose extracted by [Bmim][Cl] using the same processes. This was attributed to the smaller cations of [Emim][OAc] that have a higher ability to interact with cellulose chains than the larger cations of [Bmim][Cl] in the same condition. On top of that, the morphological property, such as the diameter size of the extracted nanocellulose is 10–25 nm with a few micrometers scale in length (Phanthong et al., 2017). This demonstrated the effective extraction of the nanoscale dimension of cellulose by [Emim][OAc]. Conversely, the thermal property, such as the maximum decomposition temperature of the extracted nanocellulose is significantly lower compared to the cellulose powder. This was because of the huge amount of free end chains of nanocellulose and the high exposure surface area to heat. Therefore, it can be concluded that the use of [Emim][OAc] provides powder nanocellulose with low crystallinity and low thermal stability.
Properties of Nanocellulose Extracted by Hydrogen Sulfate Anion-Based Ionic Liquids
Table 7 displays the properties of nanocellulose extracted by hydrogen sulfate anion-based ionic liquids. The nanocellulose has been extracted from microcrystalline cellulose using [Bmim][HSO4] (Tan et al., 2015; Low et al., 2020). The chemical, crystalline, morphological, and thermal properties of the extracted nanocellulose were characterized by Fourier transform infrared spectrometer, X-ray diffractometer, transmission electron microscope, and thermal gravimetric analyzer. The chemical property, such as the infrared spectrum of the extracted nanocellulose is nearly equal to the spectrum of the microcrystalline cellulose. This implied no changes in the functional groups of the extracted nanocellulose. Besides, the absence of peaks of [Bmim][HSO4] in the extracted nanocellulose spectrum suggested the complete removal of [Bmim][HSO4] during the washing process. Interestingly, the crystalline property, such as the crystallinity index of the extracted nanocellulose is increased compared to the microcrystalline cellulose. This was due to the progressive decrease and elimination of the amorphous parts of cellulose. Furthermore, the morphological property, such as the width size of the extracted nanocellulose is around 15–20 nm (Tan et al., 2015) and 12–60 nm (Low et al., 2020) with a rod/needle-like shape. This indicated that the size of the extracted nanocellulose can be achieved on the nanometer scale. On the other hand, the thermal property, such as the decomposition temperature of the extracted nanocellulose is lesser compared to the microcrystalline cellulose. This was ascribed to the high surface area of the extracted nanocellulose, which increased the exposure to heat. Hence, it can be inferred that the use of [Bmim][HSO4] gives microcrystalline nanocellulose with high crystallinity and low thermal stability.
Meanwhile, the nanocellulose has been extracted from Adansonia kilima cellulose using [Bmim][HSO4] (Chowdhury et al., 2019). The chemical, crystalline, morphological, and thermal properties of the extracted nanocellulose were characterized by Fourier transform infrared spectrometer, X-ray diffractometer, transmission electron microscope, and thermal gravimetric analyzer. The chemical property, such as the infrared spectrum of the extracted nanocellulose possessed peaks almost the same as Adansonia kilima cellulose. In addition, the aromatic ring vibration peaks disappeared, which showed the complete elimination of lignin. Intriguingly, the crystalline property, such as the crystallinity index of the extracted nanocellulose is considerably increased compared to the Adansonia kilima cellulose. This was attributed to the removal of the intra-and intermolecular hydrogen bonding because of the cavitation effect, which released the micro- and nano-fibrillated cellulosic fiber. Besides that, the morphological property, such as the width size of the extracted nanocellulose is about 15–20 nm with a needle shape (Chowdhury et al., 2019). This verified that the size of the extracted nanocellulose can be reached to the nano-level. On the contrary, the thermal property, such as the maximum decomposition temperature of the extracted nanocellulose is slightly lower compared to the Adansonia kilima cellulose. This was owing to the enhancement of defibrillation and defragmentation of long cellulose chains. Thus, it can be deduced that the use of [Bmim][HSO4] grants Adansonia kilima nanocellulose with high crystallinity and low thermal stability.
The nanocellulose has been extracted from cotton linter cellulose using [Bmim][HSO4] (Huang et al., 2019). The chemical, crystalline, morphological, and thermal properties of the extracted nanocellulose were characterized by Fourier transform infrared spectrometer, X-ray diffractometer, transmission electron microscope, and thermal gravimetric analyzer. The chemical property, such as the infrared spectrum of the extracted nanocellulose is almost similar to the spectrum of the cotton linter cellulose. This demonstrated that the extraction process had no effect on the chemical composition of cellulose. Additionally, the crystalline property, such as the crystallinity index of the extracted nanocellulose is increased compared to the cotton linter cellulose. This was caused by the amorphous regions of cellulose selectively removed, whereas the crystalline structure was preserved as crystalline regions retained in the extracted nanocellulose. Moreover, the morphological property, such as the diameter size of the extracted nanocellulose is ranged from 50 to 100 nm with a rod-like shape (Huang et al., 2019). This proved that the extraction process generated individual cellulose crystallites at the nanoscale level. Conversely, the thermal property, such as the onset degradation temperature of the extracted nanocellulose is significantly reduced compared to the cotton linter cellulose. This was due to the tinier cellulose dimensions that led to a larger surface area exposed to heat. Therefore, it can be concluded that the use of [Bmim][HSO4] provides cotton linter nanocellulose with high crystallinity and low thermal stability.
On top of that, the nanocellulose has been extracted from microcrystalline cellulose using [HEA][HSO4] (Gonçalves et al., 2018). The chemical, crystalline, morphological, and thermal properties of the extracted nanocellulose were characterized by Fourier transform infrared spectrometer, X-ray diffractometer, transmission electron microscope, and thermal gravimetric analyzer. The chemical property, such as the infrared spectrum of the extracted nanocellulose exhibited there were no bands of aliphatic amine group and hydrogen sulfate group. This validated the complete removal of [HEA][HSO4] during the washing process. On the other hand, the crystalline property, such as the intensity of the X-ray diffraction peak of the extracted nanocellulose is lesser compared to the microcrystalline cellulose. This was ascribed to some degradations in the crystalline regions of the nanocellulose during the extraction process. Furthermore, the morphological property, such as the diameter size of the extracted nanocellulose is within the range of 0–3 nm with a needle shape (Gonçalves et al., 2018). This showed the effective extraction of the nanoscale dimension of cellulose by [HEA][HSO4]. In contrast, the thermal property, such as the onset decomposition temperature of the extracted nanocellulose is considerably lessened compared to the microcrystalline cellulose. This was because of the high surface area of the extracted nanocellulose, which is more exposed to heat than macroscopic cellulose. Hence, it can be inferred that the use of [HEA][HSO4] gives microcrystalline nanocellulose with low crystallinity and low thermal stability.
Conclusion
In this paper, ionic liquid examples, cellulose sources, ionic liquid syntheses, and the nanocellulose extraction processes were shortly reviewed. In addition, the important properties, for instance chemical, crystalline, morphological, and thermal properties of the extracted nanocellulose were also revisited in this short review. Ionic liquids used in the extraction of nanocellulose are mostly comprised of chloride, acetate, and hydrogen sulfate anion-based ionic liquids. Chloride anion-based ionic liquids are usually synthesized via alkylation reaction, while acetate anion-based ionic liquids are commonly synthesized through metathesis reaction, whereas hydrogen sulfate anion-based ionic liquids are generally synthesized via neutralization reaction. In most studies, the use of ionic liquids has not changed the chemical structure and functional groups of the extracted nanocellulose. On top of that, nanocelluloses extracted by chloride and acetate anion-based ionic liquids have a low crystallinity index. On the other hand, nanocelluloses extracted by hydrogen sulfate anion-based ionic liquids have a high crystallinity index. Besides that, the diameter size of the nanocellulose extracted by chloride and acetate anion-based ionic liquids is ranged from 10 to 200 nm. On the contrary, the diameter size of the nanocellulose extracted by hydrogen sulfate anion-based ionic liquids is ranged from 3 to 100 nm. Nevertheless, the thermal stability of the extracted nanocellulose is frequently lower than the raw cellulose. Therefore, this short review may be helpful not only for the extraction of nanocellulose but also for researchers in expanding the applications of ionic liquids.
Author Contributions
AAS: Writing of entire manuscript and outline. SM: Original draft and analysis. KA: Review and editing.
Funding
This short review was supported by the Universiti Putra Malaysia under the Grant Putra IPM Scheme (project number: GP-IPM/2021/9697900).
Conflict of Interest
The authors declare that the research was conducted in the absence of any commercial or financial relationships that could be construed as a potential conflict of interest.
Publisher’s Note
All claims expressed in this article are solely those of the authors and do not necessarily represent those of their affiliated organizations, or those of the publisher, the editors and the reviewers. Any product that may be evaluated in this article, or claim that may be made by its manufacturer, is not guaranteed or endorsed by the publisher.
Acknowledgments
The authors would like to thank reviewers for valuable comments on this short review.
References
Al Hakkak, J., Grigsby, W. J., Kathirgamanathan, K., and Edmonds, N. R. (2019). Generation of Spherical Cellulose Nanoparticles from Ionic Liquid Processing via Novel Nonsolvent Addition and Drying. Adv. Mater Sci. Eng. 2019, 1–6. doi:10.1155/2019/2081027
Babicka, M., Woźniak, M., Dwiecki, K., Borysiak, S., and Ratajczak, I. (2020). Preparation of Nanocellulose Using Ionic Liquids: 1-Propyl-3-Methylimidazolium Chloride and 1-Ethyl-3-Methylimidazolium Chloride. Molecules 25, 1544. doi:10.3390/molecules25071544
Babicka, M., Woźniak, M., Szentner, K., Bartkowiak, M., Peplińska, B., Dwiecki, K., et al. (2021). Nanocellulose Production Using Ionic Liquids with Enzymatic Pretreatment. Mater. (Basel) 14 (12), 3264. doi:10.3390/ma14123264
Babicka, M., Ratajczak, I., and Dwiecki, K. (2019). A Comparison of Methods for Obtaining Nanocellulose Using Acid and Ionic Liquid Hydrolysis Reactions. Ann. WULS SGGW For. Wood Technol. 107, 19–23. doi:10.5604/01.3001.0013.7630
Chowdhury, Z. Z., Chandran, R. R. R., Jahan, A., Khalid, K., Rahman, M. M., Al-Amin, M., et al. (2019). Extraction of Cellulose Nano-Whiskers Using Ionic Liquid-Assisted Ultra-sonication: Optimization and Mathematical Modelling Using Box-Behnken Design. Symmetry (Basel). 11 (9), 1148. doi:10.3390/sym11091148
Chowdhury, Z. Z., and Hamid, S. B. A. (2016). Preparation and Characterization of Nanocrystalline Cellulose Using Ultrasonication Combined with a. BioResources 11 (2), 3397–3415. doi:10.15376/biores.11.2.3397-3415
Dai, H., Zhang, H., Ma, L., Zhou, H., Yu, Y., Guo, T., et al. (2019). Green pH/magnetic Sensitive Hydrogels Based on Pineapple Peel Cellulose and Polyvinyl Alcohol: Synthesis, Characterization and Naringin Prolonged Release. Carbohydr. Polym. 209 (381), 51–61. doi:10.1016/j.carbpol.2019.01.014
Gonçalves, A. P., Oliveira, E., Mattedi, S., and José, N. M. (2018). Separation of Cellulose Nanowhiskers from Microcrystalline Cellulose with an Aqueous Protic Ionic Liquid Based on Ammonium and Hydrogensulphate. Sep. Purif. Technol. 196, 200–207.
Grząbka-Zasadzińska, A., Amietszajew, T., and Borysiak, S. (2017). Thermal and Mechanical Properties of Chitosan Nanocomposites with Cellulose Modified in Ionic Liquids. J. Therm. Anal. Calorim. 130, 143–154. doi:10.1007/s10973-017-6295-3
Grząbka-Zasadzińska, A., Skrzypczak, A., and Borysiak, S. (2019). The Influence of the Cation Type of Ionic Liquid on the Production of Nanocrystalline Cellulose and Mechanical Properties of Chitosan-Based Biocomposites. Cellulose 26 (8), 4827–4840. doi:10.1007/s10570-019-02412-1
Haron, G. A. S., Noh, H. B., and Moniruzzaman, M. (2021). Ionic Liquid-Assisted Nanocellulose Preparation from Microcrystalline Cellulose. J. Phys. Conf. Ser. 1793 (1). doi:10.1088/1742-6596/1793/1/012046
Haron, G. A. S., Mahmood, H., Noh, H. B., Goto, M., and Moniruzzaman, M. (2022). Cellulose Nanocrystals Preparation from Microcrystalline Cellulose Using Ionic Liquid-DMSO Binary Mixture as a Processing Medium. J. Mol. Liq. 346, 118208. doi:10.1016/j.molliq.2021.118208
Hernani, H., Mudzakir, A., and Fauzi, B. (2019). Chemistry Concepts in Nanocellulose Isolation and its Potential for Green Chemistry Learning. Unnes Sci. Educ. J. 6 (3), 234–244. doi:10.15294/USEJ.V713.25790
Huang, J., Hou, S., and Chen, R. (2019). Ionic Liquid-Assisted Fabrication of Nanocellulose from Cotton Linter by High Pressure Homogenization. BioResources 14 (4), 7805–7820. doi:10.15376/biores.14.4.7805-7820
Huang, J., Lin, C., Chen, R., Xiong, W., Wen, X., and Luo, X. (2020). Ionic Liquid-Assisted Synthesis of Nanocellulose Adsorbent and its Adsorption Properties. Cailiao Yanjiu Xuebao/Chinese J. Mater Res. 34 (9), 674–682. doi:10.11901/1005.3093.2020.017
Jordan, J. H., Easson, M. W., and Condon, B. D. (2020). Cellulose Hydrolysis Using Ionic Liquids and Inorganic Acids under Dilute Conditions: Morphological Comparison of Nanocellulose. RSC Adv. 10 (65), 39413–39424. doi:10.1039/d0ra05976e
Li, J., Wei, X., Wang, Q., Chen, J., Chang, G., Kong, L., et al. (2012). Homogeneous Isolation of Nanocellulose from Sugarcane Bagasse by High Pressure Homogenization. Carbohydr. Polym. 90 (4), 1609–1613. doi:10.1016/j.carbpol.2012.07.038
Low, F. W., Samsudin, N. A., Yusoff, Y., Tan, X. Y., Lai, C. W., Amin, N., et al. (2020). Hydrolytic Cleavage of Glycosidic Bonds for Cellulose Nanoparticles (CNPs) Production by BmimHSO4 Ionic Liquid Catalyst. Thermochim. Acta 684, 178484. doi:10.1016/j.tca.2019.178484
Menezes, D. B., Diz, F. M., Romanholo Ferreira, L. F., Corrales, Y., Baudrit, J. R. V., Costa, L. P., et al. (2021). Starch-based Biocomposite Membrane Reinforced by Orange Bagasse Cellulose Nanofibers Extracted from Ionic Liquid Treatment. Cellulose 28 (7), 4137–4149. doi:10.1007/s10570-021-03814-w
Miao, J., Yu, Y., Jiang, Z., and Zhang, L. (2016). One-pot Preparation of Hydrophobic Cellulose Nanocrystals in an Ionic Liquid. Cellulose 23 (2), 1209–1219. doi:10.1007/s10570-016-0864-7
Mohd Ishak, N. A., Khalil, I., Abdullah, F. Z., and Muhd Julkapli, N. (2020). A Correlation on Ultrasonication with Nanocrystalline Cellulose Characteristics. Carbohydr. Polym. 246, 116553. doi:10.1016/j.carbpol.2020.116553
Onkarappa, H. S., Prakash, G. K., Pujar, G. H., Rajith Kumar, C. R., Radha, V., Latha, M. S., et al. (2020). Synthesis and Characterization of Nanocellulose Using Renewable Resources through Ionic Liquid Medium. Adv. Nat. Sci. Nanosci. Nanotechnol. 11 (3), 035001. doi:10.1088/2043-6254/ab9d23
Onkarappa, H. S., Prakash, G. K., Pujar, G. H., Rajith Kumar, C. R., Latha, M. S., and Betageri, V. S. (2020). Hevea Brasiliensis Mediated Synthesis of Nanocellulose: Effect of Preparation Methods on Morphology and Properties. Int. J. Biol. Macromol. 160, 1021–1028. doi:10.1016/j.ijbiomac.2020.05.188
Pacheco, C. M., Bustos A, C., and Reyes, G. (2020). Cellulose Nanocrystals from Blueberry Pruning Residues Isolated by Ionic Liquids and TEMPO-Oxidation Combined with Mechanical Disintegration. J. Dispersion Sci. Technol. 41 (11), 1731–1741. doi:10.1080/01932691.2020.1775092
Phanthong, P., Karnjanakom, S., Reubroycharoen, P., Hao, X., Abudula, A., and Guan, G. (2017). A Facile One-step Way for Extraction of Nanocellulose with High Yield by Ball Milling with Ionic Liquid. Cellulose 24 (5), 2083–2093. doi:10.1007/s10570-017-1238-5
Sable, I., Vikele, L., Treimanis, A., and Anteina, L. (2014). Comparative Study of Nanocellulose Preparation Methods. Seville: 13th Eur Work Lignocellul Pulp, 727–730.
Samsudin, N. A., Low, F. W., Yusoff, Y., Shakeri, M., Tan, X. Y., Lai, C. W., et al. (2020). Effect of Temperature on Synthesis of Cellulose Nanoparticles via Ionic Liquid Hydrolysis Process. J. Mol. Liq. 308, 113030. doi:10.1016/j.molliq.2020.113030
Shamsuri, A. A., Daik, R., and Jamil, S. N. A. (2021). A Succinct Review on the Pvdf/imidazolium-Based Ionic Liquid Blends and Composites: Preparations, Properties, and Applications. Processes 9 (5), 761. doi:10.3390/pr9050761
Shamsuri, A. A., Abdan, K., and Jamil, S. N. A. M. (2021). Properties and Applications of Cellulose Regenerated from Cellulose/imidazolium-Based Ionic Liquid/co-Solvent Solutions: A Short Review. e-Polymers 21 (1), 869–880. doi:10.1515/epoly-2021-0086
Shamsuri, A. A., Abdan, K., and Kaneko, T. (2021). A Concise Review on the Physicochemical Properties of Biopolymer Blends Prepared in Ionic Liquids. Molecules 26 (1), 216. doi:10.3390/molecules26010216
Shamsuri, A. A., Jamil, S. N. A. M., and Abdan, K. (2021). Processes and Properties of Ionic Liquid-Modified Nanofiller/Polymer Nanocomposites-A Succinct Review. Processes 9 (3), 480. doi:10.3390/pr9030480
Shamsuri, A. A., and Jamil, S. N. A. M. (2021). Application of Quaternary Ammonium Compounds as Compatibilizers for Polymer Blends and Polymer Composites-A Concise Review. Appl. Sci. 11 (7), 3167. doi:10.3390/app11073167
Shamsuri, A. A., Md. Jamil, S. N. A., and Abdan, K. (2021). A Brief Review on the Influence of Ionic Liquids on the Mechanical, Thermal, and Chemical Properties of Biodegradable Polymer Composites. Polymers 13 (16), 2597. doi:10.3390/polym13162597
Shamsuri, A. A., and Md. Jamil, S. N. A. (2020). Compatibilization Effect of Ionic Liquid-Based Surfactants on Physicochemical Properties of PBS/Rice Starch Blends: An Initial Study. Materials 13 (8), 1885. doi:10.3390/ma13081885
Tan, X. Y., Abd Hamid, S. B., and Lai, C. W. (2015). Preparation of High Crystallinity Cellulose Nanocrystals (CNCs) by Ionic Liquid Solvolysis. Biomass Bioenergy 81, 584–591. doi:10.1016/j.biombioe.2015.08.016
Vinogradova, Y. S., and Chen, J. Y. (2016). Micron- and Nano-Cellulose Fiber Regenerated from Ionic Liquids. J. Text. Inst. 107 (4), 472–476. doi:10.1080/00405000.2015.1040693
Wang, Y., Wei, X., Li, J., Wang, F., Wang, Q., Chen, J., et al. (2015). Study on Nanocellulose by High Pressure Homogenization in Homogeneous Isolation. Fibers Polym. 16 (3), 572–578. doi:10.1007/s12221-015-0572-1
Wang, Y., Wei, X., Li, J., Wang, F., Wang, Q., Zhang, Y., et al. (2017). Homogeneous Isolation of Nanocellulose from eucalyptus Pulp by High Pressure Homogenization. Industrial Crops Prod. 104, 237–241. doi:10.1016/j.indcrop.2017.04.032
Wang, Y., Wei, X., Li, J., Wang, Q., Wang, F., and Kong, L. (2013). Homogeneous Isolation of Nanocellulose from Cotton Cellulose by High Pressure Homogenization. Msce 01 (05), 49–52. doi:10.4236/msce.2013.15010
Keywords: nanocellulose, ionic liquid, extraction, dissolution, swelling
Citation: Shamsuri AA, Md. Jamil SNA and Abdan K (2022) Nanocellulose Extraction Using Ionic Liquids: Syntheses, Processes, and Properties. Front. Mater. 9:919918. doi: 10.3389/fmats.2022.919918
Received: 14 April 2022; Accepted: 09 May 2022;
Published: 24 May 2022.
Edited by:
Yasir Nawab, National Textile University, PakistanReviewed by:
Khubab Shaker, National Textile University, PakistanR.A. Ilyas, University of Technology Malaysia, Malaysia
Copyright © 2022 Shamsuri, Md. Jamil and Abdan. This is an open-access article distributed under the terms of the Creative Commons Attribution License (CC BY). The use, distribution or reproduction in other forums is permitted, provided the original author(s) and the copyright owner(s) are credited and that the original publication in this journal is cited, in accordance with accepted academic practice. No use, distribution or reproduction is permitted which does not comply with these terms.
*Correspondence: Ahmad Adlie Shamsuri, YWRsaWVAdXBtLmVkdS5teQ==; Khalina Abdan, a2hhbGluYUB1cG0uZWR1Lm15