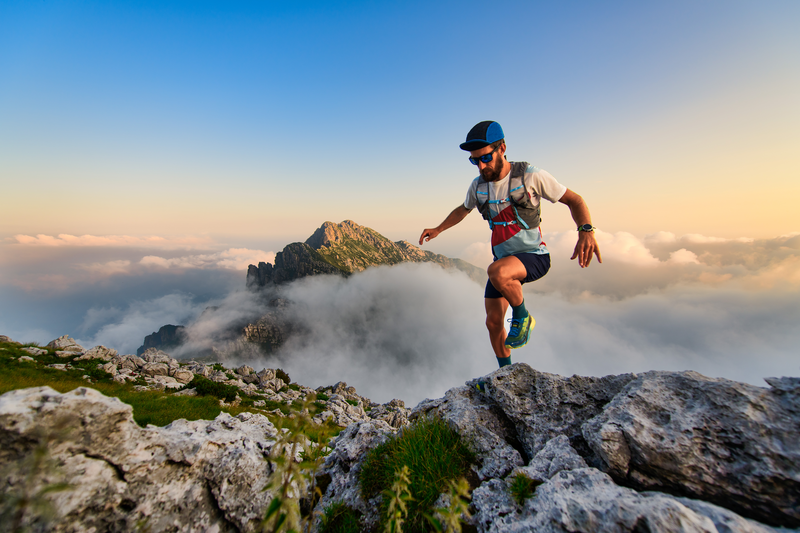
94% of researchers rate our articles as excellent or good
Learn more about the work of our research integrity team to safeguard the quality of each article we publish.
Find out more
ORIGINAL RESEARCH article
Front. Mater. , 30 May 2022
Sec. Semiconducting Materials and Devices
Volume 9 - 2022 | https://doi.org/10.3389/fmats.2022.913804
Flexible NaxV2O5/rGO papers were successfully prepared via hydrothermal method followed by vacuum filtration as a high-performance cathode for SIBs. The as-prepared NaxV2O5/rGO combined flexibility and high conductivity that can buffer stress and facilitate the fast transportation of electrons during the charge-discharge process. As a result, the as-prepared NaxV2O5-rGO paper can exhibit a reversible Na-ion storage capacity of ∼197 mA h g−1 at 100 mA g−1 and a good cycling performance with 81% capacity retention for 400 cycles at a high current density of 500 mA g−1, showing great potential in flexible energy storage devices.
With the increase in environmental concerns, people are eager to search for and develop safe and green energy storage systems (ESS). (Xu et al., 2017; Li et al., 2019). In recent years lithium-ion batteries (LIBs) have been applied to commercial electric vehicles (EVs) and provided a high energy density for large-scale energy storage systems (Xu et al., 2013; Huang et al., 2020), but the costs of lithium and the uneven global distribution of lithium resources gradually restrict their further applications. (Li et al., 2014). Therefore, safe and new cheap ESS that can partially replace LIBs need to be explored (Li et al., 2021). Among the new EES, sodium ion batteries (SIBs) have attracted increasing attention due to the rich potential sources of raw materials and suitable redox potentials (Jiang et al., 2014; Zhao et al., 2020). However, they have approximately 70% larger Na+ than Li+, which can bring sluggish diffusion kinetics to the solid state electrode materials, leading to the fast capacity decay and poor rate performance for SIBs (Liang et al., 2018; Huang et al., 2019). Thus, it is necessary to constantly study high-performance cathode materials that can meet the demands of both low cost and good electrochemical performance. (Wang et al., 2020). High-performance electrode materials should have several important traits including good electrical conductivity, abundant electrochemical active sites and desirable structure. (Lu et al., 2016; Pei et al., 2017). The above traits should be considered when designing and preparing high-performance sodium ion batteries.
To date, a large variety of sodium ion conductors such as pyrophosphates (Niu et al., 2019), fluorophosphates (Lim et al., 2014), sulfates (Wu et al., 2014), sodium transition-metal oxides (Liu et al., 2020a), polyanions (Senthilkumar et al., 2020), and the Prussian blue metalates (Liu et al., 2020b; Huang et al., 2021) have been proposed as SIB cathodes. Among these different types of SIB cathode materials, vanadium-based oxides have attracted significant attention due to their high Na-storage capacities, diverse structures, and high electrochemical activity. (Córdoba et al., 2019; Jo et al., 2020). In recent years, a variety of sodium vanadates including V2O5 polymorphs (Baddour-Hadjean et al., 2018), NaxVO2 (Guignard et al., 2013), NaVO3 (Venkatesh et al., 2014), NaV3O8 (Kang et al., 2015), Na0.33V2O5 (Shang et al., 2019), and γ-Na0.96V2O5 (Emery et al., 2018) have been investigated as the SIB cathode materials. Given their good electrochemical performances, people expect to design and prepare high-performance new electrode materials for sodium ion batteries from the various sodium vanadates. More recently, Jingjie Feng et al. reported that NaxV2O5·nH2O/KB shows a good electrochemical performance for SIB cathode. A high Na-storage capacity of 239 mAh g−1 was acquired at 20 mA g−1 and a good discharge capacity retention rate of 91% can be maintained after 90 cycles at 200 mA g−1 (Feng et al., 2018). Then, Nicolas Emery et al. reported that the γ-Na0.96V2O5 cathode material shows a reversible Na+ insertion/extraction behavior and can deliver a specific capacity of 125 mAh g−1 at 0.2°C (Emery et al., 2018). Lately, Yifan Dong et al. studied the NaV6O15 microflowers as an SIB cathode material, which shows a reversible Na-storage capacity of 126 mAh g−1at 100 mA g−1. It also demonstrates good cycle stability and 87% capacity retention over 2000 cycles at 5 A g−1 (Dong et al., 2020). These studies inspired us to develop a promising SIBs cathode material by controllable preparation of sodium vanadates with desirable morphology, structure and composition. A one dimensional (1D) structure can reduce ion diffusion path and promote the full use of active sites, which could endow cathode material with enhanced Na-ion storage performance (Zhu et al., 2019). In addition, the high electrochemical performance of NaxV2O5 is restricted by its low electron conductivity and large volume change. Design of a composite consisting of 1D NaxV2O5·nH2O nanobelts and conductive carbon is a viable approach, which has both high electrochemical performance and good mechanical properties (Osman et al., 2021). Graphene, as a typical two-dimensional carbon, can provide elastic matrix for loading cathode materials and simultaneously acts as conductive network for strengthening the dynamical process both of electrons and ion transportation (Zhou et al., 2019; Qu et al., 2021). If we can controll fabrication of the 1D NaxV2O5 composite with the reduced graphene oxide (rGO), the freestanding NaxV2O5/rGO composite cathode with enhanced electrochemical performance would be acquired.
In this work, a freestanding composite (denoted as NaxV2O5/rGO) consisting of δ-NaxV2O5 nanobelts and rGO was fabricated by a facile hydrothermal method combined with vacuum filtration. The freestanding composite composed of the δ-NaxV2O5 nanobelts and rGO offers several advantages. For instance, the bilayered δ-NaxV2O5 nanobelts with a large interlayer spacing and 1D belt-shaped morphology can reduce diffusion distance and provide a small diffusion for fast Na+ ion insertion/extraction reactions (Xu et al., 2020). In addition, the rational introduction of rGO can reduce the aggregation of NaxV2O5 nanobelts and improve the conductivity of the whole composite material (Ma et al., 2021), which can strengthen the mechanical integrity of the composite, thus improving the electrochemical performance of the electrode. Besides, compared with the traditional cathodes, the as-prepared freestanding electrode does not use non-conductive binders that can further improve electron transport (Xu et al., 2020). As a result, the as-prepared NaxV2O5/rGO electrode could exhibit a Na-storage capacity of ∼197 mA h g−1 at 100 mA g−1 and good cycling performance with 91% of capacity retention after 150 cycles at 100 mA g−1, and high rate performance with ∼91 mAh g−1 at 500 mA g−1. Moreover, a full Na-ion battery fabricated from the NaxV2O5/rGO cathode and hard carbon anode can deliver a Na-storage capacity of 101 mAh g−1 at 500 mA g−1 and good cycling stability with a capacity retention of >75% over 100 cycles.
The NaxV2O5 was synthesized by a hydrothermal reaction as follows: 3 mmol of sodiumiodide and 1.5 mmol of vanadium pentoxide were put into the 40 ml of 1 mol/L sodium chloride aqueous solution and constantly stirred for 8 h. The mixture was put into a 40 ml Teflon-lined autoclave and placed into an electric oven at 200°C for 24 h. Finally, the obtained products were washed with deionized water and ethanol serval times and dried at 80°C to obtain NaxV2O5.
The free-standing NaxV2O5/rGO paper was prepared as follows: 120 mg of the NaxV2O5 was synthesized by hydrothermal method and 60 mg of graphene oxide (GO) was synthesized via the modified Hummer’s method. The mixture of NaxV2O5 and GO was ultrasonicated for 2 h followed by constantly stirring overnight until it became homogeneous. The mixed solution of NaxV2O5 and GO was put into the Teflon-lined autoclave and placed at 180°C for 24 h. After the hydrothermal reaction, the product was re-dispersed in deionized water and subsequent vacuum filtration. The paper-like product via peeling was dried at 80°C for 24 h to obtain the free-standing NaxV2O5/rGO composite. The mass loading of the NaxV2O5/rGO electrodes is ∼3 mg/cm2.
The NaxV2O5/rGO paper was directly cut into a round electrode with a diameter of 1 cm as the cathode and the active mass is ∼1.2 mg. The homemade sodium sheets were used both as counter electrodes and reference electrodes. Whatman glass microfiber filters (GF/D) were used as the separator and 1 M NaClO4 in ethylene carbonate (EC): propylene carbonate (PC) (1:1 by v/v) with 5.0% fluoroethylene carbonate (FEC) as the electrolyte.
The Na-ion full battery was fabricated in an argon-filled glove box composed of NaxV2O5/rGO cathode and hard carbon anode. The hard carbon anode was prepared by mixing hard carbon, Super P, and polyvinylidene difluoride (8:1:1 wt%) in N-Methylpyrrolidone, and then the slurry was spread onto Cu foil. We controlled the negative capacity/positive capacity ratio, which was ∼1.1 to guarantee cell balance. The anode was firstly cycled between 0.01 and 1.5 V in a SIB half cell before preparing the full SIB cell.
Figure 1 schematically illustrates the synthesis procedure of the NaxV2O5/rGO paper. The mixture of the NaxV2O5 and rGO was prepared by hydrothermal treatment of the NaxV2O5 and GO. Then, the freestanding NaxV2O5/rGO paper was obtained by facile vacuum filtration. The crystal structures of the as-synthesized samples (NaxV2O5 and NaxV2O5/rGO) are characterized by X-Ray diffraction (XRD). As shown in Figure 2, the XRD patterns of the synthesized NaxV2O5 can be matched with a pure bilayer δ-NaxV2O5 phase, which is consistent with previously reported results (Feng et al., 2018). NaxV2O5/rGO exhibits similar diffraction patterns compared to that of the NaxV2O5 sample, indicating NaxV2O5 still exhibits high phase purity and GO has no effect on the formation of NaxV2O5·nH2O after the GO is added into the hydrothermal process. Then, the sodium to vanadium ratio was determined for the as-synthesized NaxV2O5 sample by use of inductively coupled plasma optical emission spectroscopy (ICP-OES). The Na to V ratios in NaxV2O5 samples were determined to be 0.16. When the vanadium level in each formula is set equal to 2.0, the corresponding sodium level in the NaxV2O5 samples equals 0.32. (Supplementary Table S1). To confirm the morphology, SEM and TEM images were recorded on the as-synthesized NaxV2O5 and NaxV2O5/rGO paper. As shown in Figure 3A, one can see that the as-prepared NaxV2O5 shows a long nanobelt morphology with a smooth surface and tens of micrometers in length and about 100–200 nm in width. The detailed crystal structures of NaxV2O5 nanobelts were further characterized by a transmission electron microscope (TEM) (Figures 3B,C). The low-magnification TEM image (Figure 3B) further confirmed that the as-synthesized NaxV2O5 nanobelts have a nanobelt structure, which is consistent with the observation in the SEM images. The high resolution (HR) TEM image demonstrates that these nanobelts are single crystals with an interplanar spacing of 0.36 nm, which corresponds to the (110) plane of the monoclinic phase NaxV2O5 (Figure 3C). A typical scanning TEM image of a single NaxV2O5 nanobelt and the corresponding element mapping images are recorded to further investigate the elemental distribution of the NaxV2O5 nanobelt (Figure 3D). The elements Na, V, and O are homogeneously dispersed in the NaxV2O5 nanobelt. Figures 3E,F exhibit the typical SEM images of NaxV2O5/rGO paper. The interconnected NaxV2O5 and rGO networks were observed, indicating the uniform intimate contact between NaxV2O5 and rGO, which could increase the mechanical strength of freestanding NaxV2O5/rGO hybrid paper to enhance cycling performance. Figures 3G–I shows optical images of freestanding NaxV2O5/rGO paper, which can exhibit the excellent flexibility of NaxV2O5/rGO paper and no obvious cracks were observed at the different bending states. Interestingly, based on the practical requirement, the as-fabricated NaxV2O5/rGO paper can be easily cut into the desired shapes to be directly used as the electrode of flexible SIBs.
FIGURE 3. (A) SEM of as-synthesized NaxV2O5 powder;(B) TEM; (C) SAED; (D) EDS spectrum and Na, V, and O concentration in the NaxV2O5 nanobelts. (E,F) SEM images of NaxV2O5/rGO film; (G–I) Optical images of NaxV2O5/rGO film at different bending states.
To study the electrochemical performance of NaxV2O5/rGO paper as cathode material for SIBs, the cyclic voltammetry (CV) test of NaxV2O5/rGO nanocomposite electrode was first assessed during the initial three cycles at 0.1 mV s−1 (Figure 4A). The CV curves of NaxV2O5/rGO are overlapping, suggesting the highly reversible electrochemical behaviors. The slightly different shape between the first CV curve and subsequent CV curves may be caused by the electrode polarization. Furthermore, It exhibits six main anodic current peaks around 1.91, 2.75, 2.82, 3.01, 3.15, and 3.65 V (vs. Na+/Na), respectively, and six cathodic peaks around 1.65, 2.75, 2.91, 3.12, and 3.50 V (vs. Na+/Na). The multiple redox peaks correspond to the multi-step insertion/extraction of Na+ in the different available locations with energy differences. Two oxidation peaks (1.91 and 3.65 V) and reduction peaks (1.65 and 3.50 V) with high peak current demonstrate high Na + diffusion kinetics of the NaxV2O5/rGO paper electrode. The cycling profiles of the NaxV2O5/rGO electrode at 100 mA g−1 in the voltage range of 1.5–4.1 V are depicted in Figure 4B. The NaxV2O5/rGO paper electrode shows analogous profiles corresponding well to the redox peaks of CV curves, indicating the good reversibility of the as-fabricated electrode. The dominated discharge/charge plateaus at about 3.6 V attributed to the reduction of V5+ to V4+ indicate a high electrochemical activity of V4+/V5+(He et al., 2016; Feng et al., 2018).
FIGURE 4. (A) The initial three CV curves of the NaxV2O5/rGO electrode at a scanning rate of 0.1 mV s−1; (B) Initial three charge/discharge curves of the NaxV2O5/rGO electrode at a current density of 100 mA g−1. The electrochemical performance of NaxV2O5/rGO electrode: (C) cycling performance; and (D) rate capability. (E) Cycling performance of NaxV2O5/rGO electrode at a current density of 500 mAg−1.
The cycling performance of NaxV2O5/rGO paper as cathode materials for SIBs is further studied, as shown in Figure 4C. The cyclability of the NaxV2O5/rGO paper electrode was first tested at a low current density of 100 mA g−1 (Figure 4C). It can deliver an initial charge/discharge capacity of 84 mAh g−1/197 mAh g−1. In the second and third cycles, the discharge capacity was almost no decay, demonstrating good Na-storage reversibility. After 150 cycles, a discharge specific capacity of 181 mAh g−1 was retained with a high capacity retention of 91% compared with that in the 1st cycle (Figure 4C). On the contrary, the NaxV2O5 electrode exhibit degraded electrochemical performance. The first discharge capacity of the NaxV2O5 electrode could reach 185 mAh g−1, but the discharge capacity was only retained at 131 mAh g−1 after 150 cycles with a capacity retention of 70%. EIS tests are investigated to study the electrode reaction kinetics. Supplementary Figures S1A,B, the semicircle in high frequency (interfacial impedance, Rf) and middle frequency (charge transfer impedance, Rct) become larger after 10 cycles for NaxV2O5 and NaxV2O5/rGO. An equivalent circuit model was established, as shown in Supplementary Figure S1, where Rs is the internal resistance, Rf is the interfacial impedance, Rct represents the charge transfer resistance. As listed in Supplementary Table S2, the cycled NaxV2O5/rGO shows lower Rf and Rct than those of NaxV2O5, indicating faster reaction kinetics of NaxV2O5/rGO.
From the above results, the NaxV2O5/rGO paper electrochemical showed superior cycling stability and strengthened reaction kinetics compared with the rare NaxV2O5 electrodes. The rate performance of the NaxV2O5/rGO paper at different current densities is shown in Figure 4D. The NaxV2O5/rGO paper cathodes could exhibit average specific discharge capacities of 200, 163, 129, 92, and 65 mAh g−1 at current densities of 100, 200, 500, 1000, and 2000 mA g−1, respectively. When the current density comes back to 100 mA g−1, an average specific discharge capacity of 187 mAh g−1 could be preserved, showing the excellent electrochemical performance of the NaxV2O5/rGO paper electrode at the different current densities. Considering the high reversibility of the as-prepared NaxV2O5/rGO paper electrodes, we further evaluated the NaxV2O5/rGO electrode at 500 mA g−1 for 400 cycles as shown in Figure 4E. A specific discharge capacity of 91 mAh g−1 was retained after 400 cycles with a capacity retention of 81%. Therefore, we believe the as-prepared NaxV2O5/rGO paper electrodes exhibited a high rate performance and good cycling stability, indicating the enhanced electrochemical performance of the freestanding electrodes in the present work. To confirm the benefits of the NaxV2O5/rGO paper, a comparison of the Na-storage performance between the as-prepared NaxV2O5/rGO and other vanadium-based cathode materials is listed in Table 1. The electrochemical performance of our synthesized NaxV2O5/rGO is superior to the vanadium-based cathode materials of SIBs recently reported. The good electrochemical performance of our NaxV2O5/rGO films may be due to the following reasons: first, the bilayered NaxV2O5 nanobelts with a large interlayer spacing and 1D belt-shaped morphology can reduce diffusion distance and provide a small diffusion for fast Na+ ion insertion/extraction reactions. The other is that the presence of rGO can reduce the aggregation of NaxV2O5 nanobelts and improve the conductivity of the whole composite material, which can strengthen the mechanical integrity of the composite and thus improve the cycling stability of the electrode.
TABLE 1. Comparison of the electrochemical performance of the electrode materials between this work and previous reports.
Due to the high chemical activity of Na metal, the metallic Na anode would be highly dangerous in practical application. Na-ion full batteries based on hard carbon and NaxV2O5/rGO paper as the anode and cathode electrodes, respectively, were assembled to further study the electrochemical behavior of full SIBs. Figure 5A depicts the typical voltage profiles of the hard carbon anode and NaxV2O5/rGO paper cathode measured in the Na half-cell. Both the NaxV2O5/rGO and hard carbon could achieve reversible Na-ion transport from their respective skeleton structures. Figure 5B shows the charge–discharge profiles of the full battery at 500 mA g−1 with cut-off voltages of 3.8 and 1.0 V. The initial discharge-specific capacities could attain 109 mAh g−1. Charge-discharge specific capacities could also retain 134/135 mAh g−1 with a high Coulombic efficiency of 99% in the second cycle. As shown in Figure 5C, after 100 cycles, the full Na-ion battery retained a discharge specific capacity of 101 mAh g−1 with a capacity retention of >75%, showing the good cycling performance of SIBs based on NaxV2O5/rGO paper electrode and hard carbon electrode. Importantly, the average Coulombic efficiency was higher than 98% during the 100 cycles, suggesting the highly reversible Na-ion storage behavior for the full Na-ion battery. Besides the high capacity retention, the full Na-ion battery also exhibited a good rate of performance. As shown in Figure 5D, the full Na-ion battery delivered average specific discharge capacities of 218, 197, 172, 137, 113, and 97 mAh g−1 at constant current densities of 50, 100, 200, 500, 1000, and 2000 mA g−1 respectively. When the current density was recovered to 50 mA g−1, the reversible capacity came back to 193 mAh g−1, indicating a good tolerance for the rapid Na-ion insertion/extraction cycles. The current work shows that the NaxV2O5/rGO has great potential as the freestanding cathode material for stable sodium ion batteries.
FIGURE 5. Demonstration of SIBs based on NaxV2O5/rGO || hard carbon configuration. (A) Typical charge–discharge curves of NaxV2O5/rGO film || Na and hard carbon || Na in a half-cell configuration; (B) Electrochemical charge–discharge voltage profiles of SIBs, cycled at a current rate of 500 mA g−1; (C) Cycling performance of SIBs at a constant current of 500 mA g−1; (D) Rate capability of SIBs at different current rates, increasing from 50 mA g−1 to 2A g−1.
In summary, a flexible NaxV2O5/rGO paper was successfully prepared via the hydrothermal method followed by vacuum filtration as a high-performance cathode for SIBs. The as-prepared NaxV2O5/rGO possessed flexibility and high conductivity that can buffer stress and facilitate the fast transportation of electrons during the charge-discharge process. As a result, the as-prepared NaxV2O5-rGO paper can deliver a reversible Na-ion storage capacity of ∼197 mA h g−1 at 100 mA g−1 and showed a good cycling performance with 81% capacity retention for 400 cycles at a high current density of 500 mA g−1, showing great potentials in flexible energy storage devices.
The original contributions presented in the study are included in the article/Supplementary Material, further inquiries can be directed to the corresponding author.
JX conceived the concept and directed the research. QW and XL designed the project. QW and XL carried out material synthesis. YY and XYL performed material characterization. JX and XW wrote the paper. All authors discussed the results and commented on the paper.
This work was supported by the Students’ Innovation and Entrepreneurship Training Program of Zhengzhou University (No.2020cxcy101).
The authors declare that the research was conducted in the absence of any commercial or financial relationships that could be construed as a potential conflict of interest.
All claims expressed in this article are solely those of the authors and do not necessarily represent those of their affiliated organizations, or those of the publisher, the editors and the reviewers. Any product that may be evaluated in this article, or claim that may be made by its manufacturer, is not guaranteed or endorsed by the publisher.
The Supplementary Material for this article can be found online at: https://www.frontiersin.org/articles/10.3389/fmats.2022.913804/full#supplementary-material
Baddour-Hadjean, R., Safrany Renard, M., Emery, N., Huynh, L. T. N., Le, M. L. P., and Pereira-Ramos, J. P. (2018). The Richness of V2O5 Polymorphs as Superior Cathode Materials for Sodium Insertion. Electrochimica Acta 270, 129–137. doi:10.1016/j.electacta.2018.03.062
Córdoba, R., Kuhn, A., Pérez-Flores, J. C., Morán, E., Gallardo-Amores, J. M., and García-Alvarado, F. (2019). Sodium Insertion in High Pressure β-V2O5: A New High Capacity Cathode Material for Sodium Ion Batteries. J. Power Sources 422, 42–48. doi:10.1016/j.jpowsour.2019.03.018
Dong, Y., Xu, J., Chen, M., Guo, Y., Zhou, G., Li, N., et al. (2020). Self-assembled NaV6O15 Flower-like Microstructures for High-Capacity and Long-Life Sodium-Ion Battery Cathode. Nano Energy 68, 104357. doi:10.1016/j.nanoen.2019.104357
Emery, N., Baddour-Hadjean, R., Batyrbekuly, D., Laïk, B., Bakenov, Z., and Pereira-Ramos, J.-P. (2018). γ-Na0.96V2O5: A New Competitive Cathode Material for Sodium-Ion Batteries Synthesized by a Soft Chemistry Route. Chem. Mat. 30 (15), 5305–5314. doi:10.1021/acs.chemmater.8b02066
Feng, J., Xiong, Z., Zhao, L., Huang, C., Liu, H., Chen, S., et al. (2018). One-pot Hydrothermal Synthesis of NaxV2O5·nH2O/KB Nanocomposite as a Sodium-Ion Battery Cathode for Improved Reversible Capacity and Rate Performance. J. Power Sources 396, 230–237. doi:10.1016/j.jpowsour.2018.06.021
Guignard, M., Didier, C., Darriet, J., Bordet, P., ElkaïmDelmas, E. C., and Delmas, C. (2013). P2-NaxVO2 System as Electrodes for Batteries and Electron-Correlated Materials. Nat. Mater 12 (1), 74–80. doi:10.1038/nmat3478
He, G., Kan, W. H., and Manthiram, A. (2016). A 3.4 V Layered VOPO4 Cathode for Na-Ion Batteries. Chem. Mat. 28 (2), 682–688. doi:10.1021/acs.chemmater.5b04605
Huang, H., Xu, R., Feng, Y., Zeng, S., Jiang, Y., Wang, H., et al. (2020). Sodium/Potassium‐Ion Batteries: Boosting the Rate Capability and Cycle Life by Combining Morphology, Defect and Structure Engineering. Adv. Mat. 32 (8), 1904320. doi:10.1002/adma.201904320
Huang, J., Wei, Z., Liao, J., Ni, W., Wang, C., and Ma, J. (2019). Molybdenum and Tungsten Chalcogenides for Lithium/sodium-Ion Batteries: Beyond MoS2. J. Energy Chem. 33, 100–124. doi:10.1016/j.jechem.2018.09.001
Huang, T., Niu, Y., Yang, Q., Yang, W., and Xu, M. (2021). Self-Template Synthesis of Prussian Blue Analogue Hollow Polyhedrons as Superior Sodium Storage Cathodes. ACS Appl. Mat. Interfaces 13 (31), 37187–37193. doi:10.1021/acsami.1c09678
Jiang, Y., Hu, M., Zhang, D., Yuan, T., Sun, W., Xu, B., et al. (2014). Transition Metal Oxides for High Performance Sodium Ion Battery Anodes. Nano Energy 5, 60–66. doi:10.1016/j.nanoen.2014.02.002
Jo, J. H., Choi, J. U., Park, Y. J., Ko, J. K., Yashiro, H., and Myung, S.-T. (2020). A New Pre-sodiation Additive for Sodium-Ion Batteries. Energy Storage Mater. 32, 281–289. doi:10.1016/j.ensm.2020.07.002
Kang, H., Liu, Y., Shang, M., Lu, T., Wang, Y., and Jiao, L. (2015). NaV3O8 Nanosheet@polypyrrole Core-Shell Composites with Good Electrochemical Performance as Cathodes for Na-Ion Batteries. Nanoscale 7 (20), 9261–9267. doi:10.1039/c5nr02064f
Li, S., Dong, Y., Xu, L., Xu, X., He, L., and Mai, L. (2014). Effect of Carbon Matrix Dimensions on the Electrochemical Properties of Na3V2(PO4)3Nanograins for High-Performance Symmetric Sodium-Ion Batteries. Adv. Mat. 26 (21), 3545–3553. doi:10.1002/adma.201305522
Li, X., Fu, J., Sun, Y., Sun, M., Cheng, S., Chen, K., et al. (2019). Design and Understanding of Core/branch-Structured VS2 nanosheets@CNTs as High-Performance Anode Materials for Lithium-Ion Batteries. Nanoscale 11 (28), 13343–13353. doi:10.1039/c9nr03581h
Li, X., Zhuang, C., Xu, J., Li, L., Xu, T., Dai, S., et al. (2021). Rational Construction of K0.5V2O5 nanobelts/CNTs Flexible Cathode for Multi-Functional Potassium-Ion Batteries. Nanoscale 13 (17), 8199–8209. doi:10.1039/d1nr00993a
Liang, J., Wei, Z., Wang, C., and Ma, J. (2018). Vacancy-induced Sodium-Ion Storage in N-Doped Carbon Nanofiber@MoS2 Nanosheet Arrays. Electrochimica Acta 285, 301–308. doi:10.1016/j.electacta.2018.07.230
Lim, S. Y., Kim, H., Chung, J., Lee, J. H., Kim, B. G., Choi, J.-J., et al. (2014). Role of Intermediate Phase for Stable Cycling of Na 7 V 4 (P 2 O 7 ) 4 PO 4 in Sodium Ion Battery. Proc. Natl. Acad. Sci. U.S.A. 111 (2), 599–604. doi:10.1073/pnas.1316557110
Liu, Q., Hu, Z., Chen, M., Zou, C., Jin, H., Wang, S., et al. (2020a). The Cathode Choice for Commercialization of Sodium‐Ion Batteries: Layered Transition Metal Oxides versus Prussian Blue Analogs. Adv. Funct. Mat. 30 (14), 1909530. doi:10.1002/adfm.201909530
Liu, Y., He, D., Cheng, Y., Li, L., Lu, Z., Liang, R., et al. (2020b). A Heterostructure Coupling of Bioinspired, Adhesive Polydopamine, and Porous Prussian Blue Nanocubics as Cathode for High‐Performance Sodium‐Ion Battery. Small 16 (11), 1906946. doi:10.1002/smll.201906946
Lu, J., Chen, Z., Ma, Z., Pan, F., Curtiss, L. A., and Amine, K. (2016). The Role of Nanotechnology in the Development of Battery Materials for Electric Vehicles. Nat. Nanotech 11 (12), 1031–1038. doi:10.1038/nnano.2016.207
Ma, C., Xu, T., Yan, C., Xu, J., Kong, D., Zhang, Z., et al. (2021). Mechanism Investigation of High Performance Na3V2(PO4)2O2F/reduced Graphene Oxide Cathode for Sodium-Ion Batteries. J. Power Sources 482, 228906. doi:10.1016/j.jpowsour.2020.228906
Niu, Y., Zhang, Y., and Xu, M. (2019). A Review on Pyrophosphate Framework Cathode Materials for Sodium-Ion Batteries. J. Mat. Chem. A 7 (25), 15006–15025. doi:10.1039/c9ta04274a
Osman, S., Zuo, S., Xu, X., Shen, J., Liu, Z., Li, F., et al. (2021). Freestanding Sodium Vanadate/Carbon Nanotube Composite Cathodes with Excellent Structural Stability and High Rate Capability for Sodium-Ion Batteries. ACS Appl. Mat. Interfaces 13 (1), 816–826. doi:10.1021/acsami.0c21328
Pei, Z., Li, H., Huang, Y., Xue, Q., Huang, Y., Zhu, M., et al. (2017). Texturing In Situ: N,S-enriched Hierarchically Porous Carbon as a Highly Active Reversible Oxygen Electrocatalyst. Energy Environ. Sci. 10 (3), 742–749. doi:10.1039/c6ee03265f
Qu, J., Bai, Y., Li, X., Song, K., Zhang, S., Wang, X., et al. (2021). Rational Design of NiSe2@rGO Nanocomposites for Advanced Hybrid Supercapacitors. J. Mater. Res. Technol. 15, 6155–6161. doi:10.1016/j.jmrt.2021.11.036
Senthilkumar, B., Rambabu, A., Murugesan, C., Krupanidhi, S. B., and Barpanda, P. (2020). Iron-Based Mixed Phosphate Na4Fe3(PO4)2P2O7 Thin Films for Sodium-Ion Microbatteries. ACS Omega 5 (13), 7219–7224. doi:10.1021/acsomega.9b03835
Shang, C., Hu, L., Lin, Q., Fu, X., Wang, X., and Zhou, G. (2019). Integration of NaV6O15·nH2O Nanowires and rGO as Cathode Materials for Efficient Sodium Storage. Appl. Surf. Sci. 494, 458–464. doi:10.1016/j.apsusc.2019.07.192
Venkatesh, G., Pralong, V., Lebedev, O. I., Caignaert, V., Bazin, P., and Raveau, B. (2014). Amorphous Sodium Vanadate Na1.5+yVO3, a Promising Matrix for Reversible Sodium Intercalation. Electrochem. Commun. 40, 100–102. doi:10.1016/j.elecom.2013.11.004
Wang, Y., Lim, Y. V., Huang, S., Ding, M., Kong, D., Pei, Y., et al. (2020). Enhanced Sodium Storage Kinetics by Volume Regulation and Surface Engineering via Rationally Designed Hierarchical Porous FeP@C/rGO. Nanoscale, 12 (7), 4341–4351. doi:10.1039/c9nr09278a
Wu, L., Lu, H., Xiao, L., Qian, J., Ai, X., Yang, H., et al. (2014). A Tin(ii) Sulfide-Carbon Anode Material Based on Combined Conversion and Alloying Reactions for Sodium-Ion Batteries. J. Mat. Chem. A 2 (39), 16424–16428. doi:10.1039/c4ta03365e
Xu, G., Liu, X., Huang, S., Li, L., Wei, X., Cao, J., et al. (2020). Freestanding, Hierarchical, and Porous Bilayered NaxV2O5·nH2O/rGO/CNT Composites as High-Performance Cathode Materials for Nonaqueous K-Ion Batteries and Aqueous Zinc-Ion Batteries. ACS Appl. Mat. Interfaces 12 (1), 706–716. doi:10.1021/acsami.9b17653
Xu, J., Dou, S., Liu, H., and Dai, L. (2013). Cathode Materials for Next Generation Lithium Ion Batteries. Nano Energy 2 (4), 439–442. doi:10.1016/j.nanoen.2013.05.013
Xu, J., Tang, H., Xu, T., Wu, D., Shi, Z., Tian, Y., et al. (2017). Porous NiO Hollow Quasi-Nanospheres Derived from a New Metal-Organic Framework Template as High-Performance Anode Materials for Lithium Ion Batteries. Ionics 23, 3273–3280. doi:10.1007/s11581-017-2160-4
Zhao, H., Zhuang, C., Xu, J., Zhang, Z., Shen, W., Tang, H., et al. (2020). Synergistically Enhanced Sodium/potassium Ion Storage Performance of SnSb Alloy Particles Confined in Three-Dimensional Carbon Framework. Ionics 26 (10), 5019–5028. doi:10.1007/s11581-020-03641-2
Zhou, W., Chen, J., He, C., Chen, M., Xu, X., Tian, Q., et al. (2019). Hybridizing δ-type NaxV2O5·nH2O with Graphene towards High-Performance Aqueous Zinc-Ion Batteries. Electrochimica Acta 321, 134689. doi:10.1016/j.electacta.2019.134689
Keywords: NaxV2O5/rGO, flexibility, sodium ion batteries, cathode, freestanding
Citation: Wang Q, Li X, Xu J, Yuan Y, Li X and Wang X (2022) Rational Synthesis of Freestanding NaxV2O5-rGO Paper as the Stable Cathode for Sodium Ion Batteries. Front. Mater. 9:913804. doi: 10.3389/fmats.2022.913804
Received: 06 April 2022; Accepted: 22 April 2022;
Published: 30 May 2022.
Edited by:
Haibin Tang, Hefei Institutes of Physical Science (CAS), ChinaReviewed by:
Zhengfei Dai, Xi’an Jiaotong University, ChinaCopyright © 2022 Wang, Li, Xu, Yuan, Li and Wang. This is an open-access article distributed under the terms of the Creative Commons Attribution License (CC BY). The use, distribution or reproduction in other forums is permitted, provided the original author(s) and the copyright owner(s) are credited and that the original publication in this journal is cited, in accordance with accepted academic practice. No use, distribution or reproduction is permitted which does not comply with these terms.
*Correspondence: Junmin Xu, anVubWlueHVAenp1LmVkdS5jbg==
†These authors have contributed equally to this work
Disclaimer: All claims expressed in this article are solely those of the authors and do not necessarily represent those of their affiliated organizations, or those of the publisher, the editors and the reviewers. Any product that may be evaluated in this article or claim that may be made by its manufacturer is not guaranteed or endorsed by the publisher.
Research integrity at Frontiers
Learn more about the work of our research integrity team to safeguard the quality of each article we publish.