- 1Instituto Nacional de Ciencias Médicas y Nutrición Salvador Zubirán, Ciudad de México, Mexico
- 2Tecnológico de Monterrey—School of Engineering and Science, Ciudad de México, Mexico
- 3Instituto Politécnico Nacional, Escuela Superior de Ingeniería Mecánica y Eléctrica, Sección de Estudios de Posgrado e Investigación Edificio 5, Ciudad de México, Mexico
As a result of the pandemic, cases requiring endotracheal intubation have increased to support breathing through mechanical ventilation; this procedure is necessary when a patient cannot breathe on their own due to respiratory failure. For it, the laryngoscope is used. Even when using a laryngoscope, it is possible to injure the patient due to poor visibility at intubation. As a solution, some video-laryngoscopes present many advantages, such as reducing injuries, lowering the number of attempts to secure the airway and the image’s quality, and easy recognition of laryngeal structures. However, this type of device is not accessible to many health institutions due to the price. Therefore, this study proposes the design of a video-laryngoscope in the Computer-Aided-Design (CAD) software and constructed by additive manufacturing. For this design, the identification of the needs of the medical personnel in the anesthesiology and emergency medicine departments from the Instituto Nacional de Ciencias Médicas y Nutrición Salvador Zubirán (INCMNSZ) was taken into account. The design specifications provided by the users were used to create a proposal solution at the conceptual design level. The material and camera for the prototype instrumentation are presented with the model’s geometric parameters. Subsequently, virtual modeling of the device was carried out, and rapid prototypes were built to validate the performance of the design proposal. The final device was constructed by Selective Laser Sintering (SLS) using Nylon 12 as a construction material. Finally, the device was evaluated using airway simulators with the support of medical specialists to determine its functionality.
1 Introduction
Endotracheal intubation (EI) is an invasive procedure performed to induce anesthesia in patients by inhalation and provide mechanical ventilation (Jaber et al., 2006, 2010). The technique consists of using a laryngoscope to free the line of sight of the larynx and inserting an endotrachealtube through the glottis (Sun et al., 2005). Generally, it is performed in ailments that alter the respiratory function (Lapinsky, 2015).
The growing demand from patients infected with the SARS-CoV-2 virus has led to the need for the process of endotracheal intubation to have life support through mechanical ventilation (Huang et al., 2020; Meng et al., 2020). The procedure requires the doctor to approach the mouth of the infected patient to have a view of the glottis, causing them to be at risk of infection due to the droplets or aerosols that emanate from the patient’s mouth (Pasnick et al., 2020; Brewster et al., 2020). For reducing risk exposure, it is recommended to use video-laryngoscopes that allow a greater visual field, facilitating the procedure (Kaplan et al., 2002; Ludwin et al., 2020). However, these types of devices are not easily accessible to health institutions, causing them not to have sufficient devices to meet the demand (Meng et al., 2020).
In order to carry out EI, an endotracheal tube, a malleable metal guidewire, a plastic guidewire, a 10-ml syringe, oxygen intake, a mask, a Yankauer suction catheter, some drugs, and a laryngoscope are needed (Winton et al., 2008; Orebaugh and Bigeleisen, 2012; Barash et al., 2018). According to the technical specification certificate of the Centro Nacional de Excelencia Tecnológica en Salud (CENETEC), the laryngoscope is defined as an instrument used for direct visualization of the larynx, whose purpose is to perform EI to administer inhalational anesthetics and oxygen (Burkle et al., 2004; Salud, 2007). The procedure for managing the airway begins by taking the laryngoscope with the left hand and inserting it into the mouth, taking care not to hurt the lips and teeth. The blade should be inserted along the curvature of the anterior part of the pharynx, and then the blade is moved forward and in the midline direction to push the tongue to the left side and free the line of vision. In case of not having good vision, it is necessary to raise the blade in the direction of the long axis of the handle without levering until the glottis is visualized. Immediately, the tip of the laryngoscope blade is placed within the vallecula, lifting it to visualize the laryngeal structures. Finally, the endotracheal tube is inserted through the vocal cords without presenting any resistance (Finucane et al., 2003; Orebaugh and Bigeleisen, 2012; Garvin, 2020; Soria et al., 2021). Figure 1 shows how the laryngoscope is held and the endotracheal tube is inserted.
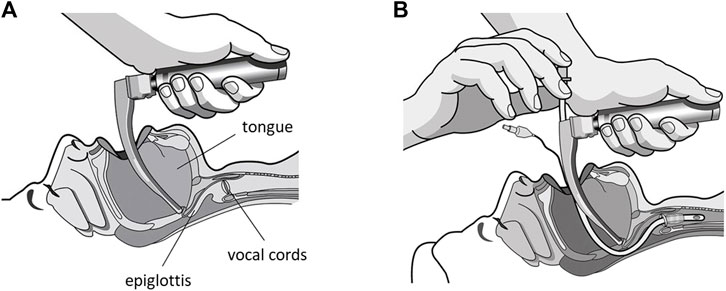
FIGURE 1. Endotracheal intubation process: (A) placement of the laryngoscope over the patient’s epiglottis and (B) insertion of the endotracheal tube into the patient’s glottis (Schächter, 2019).
When laryngoscopy is performed and the laryngeal structures are not visualized or the endotracheal tube cannot be inserted after several attempts, it can be considered a difficult airway (DA) (Henderson et al., 2004; Frerk et al., 2015). This is defined as the clinical case where a specialist has difficulty performing the EI (Frova and Sorbello, 2009). DA has an incidence of 1.15–3.8% and failed intubation from 0.13 to 0.3%, resulting in upper airway injuries up to death (Crosby et al., 1998). EI is a high-risk procedure that can release blood secretions, droplets, and aerosols (Rosen et al., 2006). The main reason why it is required to carry out EI is that the patient has some complications in the respiratory function, cardiorespiratory arrest, difficult airway, cranioencephalic trauma (Gupta et al., 2005), etc. In addition, this procedure is one of the pillars for the training of anesthesiologists since it represents a constant challenge for their training (Mayrose et al., 2003; Rajeswaran et al., 2018). Furthermore, the erroneous handling of a DA is one of the main reasons for complications that exist in the practice of anesthesiology (Sagarin et al., 2005; Jaber et al., 2010), becoming one of the leading causes of death related to this medical specialty (Lavery and McCloskey, 2008).
Due to the complexity of managing a DA and the risk of the SARS-CoV-2 contagion, alternatives and developments of new technologies have been sought for airway management (Orser, 2020; Fuentes-Alvarez et al., 2021), providing anesthesiologists with greater safety and ease (Kaplan et al., 2002; Weissman et al., 2020). Among these new technologies, video-laryngoscopes stand out, which are devices for managing the airway, whose design facilitates glottic vision and EI (Sun et al., 2005; Noppens et al., 2012).
With the emergence of these devices, the perspective of anesthesiology has changed since it allows a more direct view at the entrance of the glottis, regardless of the line of sight of the larynx (Coloma, 2017). The video-laryngoscope placement technique is different from traditional laryngoscopy since it is not required to align the oral, pharyngeal, and laryngeal axes (Figure 2). However, a good oral opening must be achieved for the device to enter down the midline and follow the shape of the palate and pharynx (Garvin, 2020; Soria et al., 2021). A difficulty that can arise in this technique is inserting the endotracheal tube, caused by the displacement of the tongue to the right side or by not having a sufficient oral opening (Frerk et al., 2015). Due to this complication, video-laryngoscopes have been designed to have a channel for the displacement of the endotracheal tube (Sun et al., 2005; Noppens et al., 2012).
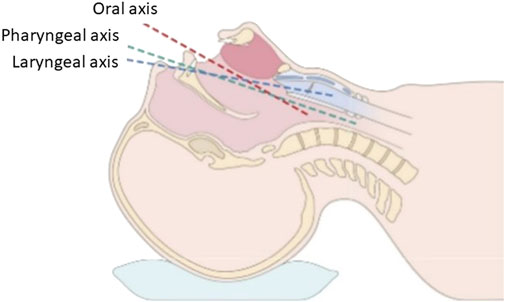
FIGURE 2. Example of the patient’s position to align the oral, pharyngeal, and laryngeal axes to perform endotracheal intubation (Coloma, 2017).
Despite being very useful devices, their main limitation in institutions with limited resources is their cost (∼ USD $20,000.00) (Agro et al., 2003). As an example of the high prices of devices, the STORZ price, C-MAC #3 is ∼ USD $16,000.00, and the STORZ, C-MAC #4 have a price of ∼ USD $16,500.00. With the following general characteristics, we just include one disposable blade and a monitor of 7”. On the other hand, the King Vision is an economical video-laryngoscope with a price of ∼ USD $ 2,500.00 as its main characteristics are a 4.0” monitor and a single disposable blade. Therefore, the development of a device with the appropriate characteristics that assist the EI procedure and, consequently, facilitate the management of the DA is needed. In addition, the device must help reduce the spread of COVID-19 to health personnel.
As a consequence of the limited resources, diverse video-laryngoscope designs were produced and are currently being used in hospitals by patients and medical workers. Therefore, to contribute to the reduction of active cases of medical personnel due to COVID-19 and have a device that has the technical requirements that allow optimizing the EI process, the design of a video-laryngoscope in CAD software and its construction using the SLS addition printing technique is carried out, allowing this prototype to have an affordable cost and be easy to manufacture and instrument (Longhitano et al., 2021). Three-dimensional (3D) printed models enable physicians and engineers to consider ergonomic concerns and design alternatives without compromising the price and quality in a timely and cost-effective manner. However, information about the application of 3D printing in anesthesiology or emergency medicine is sparse.
This work proposes the development of a low-cost video-laryngoscope device due to the need during the pandemic since there was a shortage of this type of equipment in Mexico and the available devices required the specialist doctor to place his face close to the mouth to perform the intubation procedure. The main contribution of the presented device lies in the integration of a low-cost, high-resolution screen and the design of a tip for the laryngoscope blade, whose measurement turns out to be the average between MAC 3 and MAC 4, which are the most used blades for performing emergency intubations in Mexico. In addition, the device has a fastening system that seeks to improve the device’s ergonomics during the process mentioned above; this last part was added in the conclusion of the manuscript.
2 Materials and Methods
2.1 Conceptual Design
The design proposal called VidLa-SZ was based on the measurement of three video-laryngoscopes: Welch Allyn with Macintosh No. 3 blade, Airtraq (small and regular), and the Glidescope. In this process, each of the device positive and negative characteristics was analyzed. The main measurements were as follows: handle (length, width, and height), blade (total length, width, and curvature), and geometry (cylindrical; quadratic).
In order to carry out a new design, the requirements and specifications of the specialists in the Anesthesiology and Emergency Medicine Departments of the INCMNSZ were taken into account, which are areas that constantly perform the EI process. The characteristics and observations that medical specialists consider an ideal video-laryngoscope should provide are a rechargeable battery, wireless charging, Wi-Fi/Bluetooth/wired connection, image quality, capacity for direct laryngoscopy, water- and impact-resistant, sterilizable, channel for an endotracheal tube, ergonomic handle, and proper angle for intubation. Once the most important characteristics were determined, the manufacturing method, the materials, and the electronic components (camera and screen) were selected.
The final design considered a device with a 5.5-mm diameter camera with LED light and a 4.5-inch screen to visualize the glottis. In addition, in this design, the length and curvature of the blade and the shape of the handle were considered. According to the characteristics desired by the medical specialists, the parameters to consider for the design of the 3D model are as follows:
• Handle length: The length of the handle should be between 6 and 10 cm to allow adequate grip to prevent it from getting in the way when performing an EI procedure in patients with obesity and/or dense breasts.
• The shape of the handle: Avoiding a quadratic handle since it can hurt the health worker’s hand when using the device, resulting in not exerting adequate force to carry out the EI process. It is recommended that the shape be cylindrical or round with at least 3 cm diameter.
• Blade length: The traditional Mac No. 3 blade is considered the most widely used for EI processes within the INCMNSZ. However, there is a record of the use of Mac No. 4 blade is also widely used for this process. Therefore, the ideally designed blade is slightly longer than Mac No. 3 without exceeding the length of Mac No. 4.
• Blade angle: The proposed blade angle is based on the Mac No. 3 blade, modifying the angle of the tip to raise the glottis and obtain a greater field of vision.
• Camera position: The camera angle must be tilted toward the blade’s tip to view it.
The parameterized model was designed on the SolidWorks® software to later build the 3D printed prototypes. It is worth mentioning that the model is made up of a blade and a handle that are consolidated as a single piece and support for the display screen, which is removable. The blade design is based on the traditional Mac No. 3 laryngoscope, the most widely used EI procedure performed at the INCMNSZ. However, it has a greater angle and length than a said blade, considering that it is unnecessary to align the laryngeal, pharyngeal, and oral axes to visualize the glottis while performing EI. Figure 3 shows the design proposal. The video-laryngoscope blade is made up of the parts specified in Figure 4, which are as follows:
• Spatula (purple): The side of the blade is in contact with the tongue while performing the laryngoscopy.
• Tip (yellow): This is the distal portion of the blade placed in the vallecula to raise the epiglottis.
• Guide (green): The opposite face of the spatula serves to direct the endotracheal tube.
• Flange (blue): Its function is to maintain the opening distance for the endotracheal tube to pass.
• Camera cavity (orange): Space where the camera is placed to achieve an adequate glottic view.
• Camera channel (strong blue): Channel where the camera slides and the camera cable are stored.
3 Results
3.1 Static Analysis of the VidLa-SZ Model
The model of the video-laryngoscope must support the loads produced at the time of performing the EI. It can be deformed by applying loads to the model, causing external loads to produce internal reactions and forces within the solid. This can result in displacements, strains, and stresses calculated in a static analysis. Hence, it is necessary to carry out simulations to determine if the design will resist these forces. The analysis of the proposed model VidLa-SZ was carried out with the simulation SolidWorks Simulation Tool®. This tool uses Finite Element Analysis (FEA), a numerical method for analyzing designs in the engineering area. This type of analysis divides a continuous elastic structure into small, well-defined substructures called elements. In this way, a complex problem is replaced by many simple problems that are solved simultaneously (Budynas et al., 2011).
The model with which the simulation was carried out for the static analysis was considered a solid, and its volumetric characteristics were: mass (0.123,403 Kg), volume (0.0000987227 m3), density (1,250 kg/m3), and weight (1,209.35 N).
For the static analysis, the following assumptions were considered:
• Due to the difficulty of obtaining continuous force measurements during laryngoscopy, the maximal force obtained in the studies cited above (Russell et al., 2012; Cordovani et al., 2019) is used and is considered static since the time of study of interest is when the maximum force is applied (during EI).
• The force is applied to the two distal quarters of the spatula with a total magnitude of 21 N, as this was the largest value recorded in the force study using the GlideScope video-laryngoscope (Cordovani et al., 2019).
• Loads are applied gradually until they reach full magnitude and remain constant without varying over time, allowing inertial forces due to insignificant accelerations to be ignored.
• The relationship between the applied loads and the model response is linear; if the magnitude of the loads is doubled, the model response is also doubled. It is assumed that the boundary conditions are constant and should not vary in magnitude, direction, and distribution while the model is deformed.
• The analysis is performed on the part of the blade and the handle. Not including the screen support because the area of interest for the analysis is the blade. The force exerted to hold the tongue and elevate the epiglottis during the laryngoscopy process is applied to the blade.
• Loads applied to the handle are considered clamp loads.
The static analysis begins with the assignment of the model’s material of construction. The material selected for the simulation was PLA. Subsequently, the model is subjected to loads and restrictions during laryngoscopy. For this study, only two types of loads are considered: clamping applied to the handle and normal force applied to the spatula of the blade. This is because the handle is where the medical specialist holds the device and must be held firmly to support the tongue and elevate the epiglottis, while the endotracheal tube is slid. Therefore, the displacements at the moment in which the maximum force is applied can be considered zero. Then the applied load can be considered as a fixed geometry fixture.
In the case of the forces that act on the blade, they are applied to the faces that encompass the two distal quarters of the spatula without reaching the blade’s tip because, in the force study during laryngoscopy (Cordovani et al., 2019), pressure sensors are placed over this area. In addition, the value of the maximum recorded force of 21 N was obtained by averaging the value of the three sensors that were placed, which indicates that the magnitude of the force is distributed throughout the selected area, as shown in Figure 5, which corresponds to the area of the three selected faces shown in blue where the normal force indicated in orange is applied.
In static analysis, the software sections the model into small elements connected at common points called nodes to form a network of interconnected discrete elements known as a mesh. The number of nodes and elements depends on the model’s geometry, size of the elements, and mesh tolerance. Simulation estimates the size of the elements that make up the mesh considering the volume surface area, among other geometric aspects. For the analysis of the proposed model, the mesh was made with solid tetrahedral elements of the parabolic type or also known as second-order or higher-order elements. These elements are defined by six central nodes, six edges, and four angular nodes. This node type was chosen because curved contours have higher precision and produce better mathematical approximations, although they consume more computational resources than linear solid elements.
Another parameter to determine the quality of the mesh is the aspect ratio. Ideally, the tetrahedral elements have the same edge length; however, the mesh cannot have perfect elements for many geometries. These differences in geometry, such as the size of an element’s edges, can affect the results. The aspect ratio measures the tetrahedral element’s longest and shortest edge. The larger it is, the worse the mesh quality. It is sought that the value of the coefficient is close to 1.0.
Figure 6 graphically shows the aspect ratio with a color scale. In blue are the elements with a coefficient of 1.0, which is the majority of the model, while the elements with a coefficient greater than 10 are red, showing that few elements do not fit properly. Therefore, the quality of the mesh is good, and the static analysis can be performed.
3.1.1 Resulting Displacements
The first aspect of analysis is the displacements suffered by the model after applying the force on the spatula at the blade’s tip. The URES scale is used to allow displacements to be detailed on a color scale where blue means null displacements and red maximum displacements. Figure 7 details the displacements that the VidLa-SZ design proposal underwent. Displacements decrease proximal to the blade. The largest displacements are located at the peak of the prototype (2,102 mm of displacement) due to the force exerted when performing the laryngoscopy process. Subsequently, it decreases in the area of camera cavity with a magnitude of 0.8–1.4 mm of displacement. In the middle area of the model sheet, the displacements range from 0.350 to 0.526 mm. Finally, in the handle and the proximal quarter of the blade, the displacements are minimal with a scale of 0–0.175 mm.
3.1.2 Strain
This refers to the rate of length change concerning the original length being a dimensionless quantity. As in the previous analysis, this deformation is denoted by a color scale, where the minimum is 0.001 (blue color) and the maximum value is 0.003 (red color). Figure 8 shows the deformations caused in the study. It is shown that along the flange, there are deformations with a value of 0.002, as in the proximal area of the spatula (green color). Most of the model has deformations close to 0, indicated in strong blue. Therefore, the design’s deformations are small; however, the junction of the guide with the proximal portion of the flange indicates that it is the area with the greatest deformation in the design. This deformation does not significantly affect the VidLa-SZ design proposal.
3.1.3 von Mises Stress
Finally, the last study performed in the static analysis of the design was the von Mises stress. In this study, there are forces to which the model is subjected after applying the clamping loads on the handle and the normal forces on the spatula of the blade. The resulting stresses are measured on a color scale, as shown in Figure 9. The blue color indicates the minimum stress (0 MPa) located at the top of the handle. The maximum stress (red color) is found in the place of maximum deformation, which is at the junction of the guide with the flange and has a value of 14.221 MPa. The maximum stress to which the model is subjected does not exceed the material’s elastic limit, so there is no breaking point. In the approach of Figure 9, it is observed that in the proximal part of the flange, the model is subjected to stresses of the order of 5–9 MPa indicated in green, and along the flange, it has values close to 2–4 MPa.
One of the areas of interest for the static analysis study is the force exerted on the tip of the video-laryngoscope, as shown in Figure 10. This figure shows that most of the tip of the video-laryngoscope has a magnitude of 1–3 MPa, and the portion where the greatest stress accumulates is in the distal part of the flange (near the camera cavity), with values close to 4–7 MPa.
3.1.4 Safety Factor Analysis
The SolidWorks Simulation Tool® allows you to assess design safety using safety factor (SF) criteria. After executing the static analysis, the SF can be evaluated at each node, and the SF is plotted throughout the model, as shown in Figure 11. Usually, an SF value less than 1.0 means that the material has failed in that area; if it is equal to 1.0, the material may start to fail, and if it is greater than 1.0, it means that the material is in good condition and safe.
For the calculation of the SF, the simulation uses the von Mises maximum stress criterion, which indicates that a ductile material begins to yield when the von Mises stress is equal to the stress limit. For this analysis, the SF was calculated from the rupture limit. Figure 11 shows that the plot of the SF has a color scale; red shows the minimum SF, while blue shows the maximum SF. The result obtained from the analysis shows that most of the model has an SF greater than 10 (excellent SF), whose maximum value identified is 4,966, 836, 000, which is located in the node at the top of the handle. The minimum value of the SF is indicated in the close-up of Figure 11 (red color), which corresponds to the zone where maximum deformation and maximum tension are found. The value of the SF is 3.375, showing that although it is the area with the greatest risk, it does not represent a problem for the design since the SF has a value greater than 1.0.
One of the areas of interest for the simulation is the tip of the video-laryngoscope since it is the element that must withstand the pressure exerted on the vallecula to elevate the epiglottis during EI. Figure 12 shows that most of the tip has an SF greater than 10 (blue). However, a green stripe with an approximate SF of 6 is perceived, located on the flange in front of the cavity where the camera is located. Despite this, the SF has an acceptable value to ensure the resistance of the design.
The static analysis (displacement, deformation, stress, and safety factor) showed that the geometry of the design proposed for the construction of the video-laryngoscope is safe since it resists the forces applied to the spatula of the device. However, due to the limitations of the simulation, it is necessary to create rapid prototypes that allow resistance and viability to be verified and evaluate its functionality with the help of medical personnel.
3.2 Construction and Instrumentation of the VidLa-SZ Video-Laryngoscope
The additive manufacturing method (3D printing) was chosen for its construction. Acrylonitrile butadiene styrene (ABS) and polylactic acid (PLA) materials were considered to manufacture preliminary prototypes throughout fused deposition modeling (FDM), which, due to their mechanical properties, are suitable for tensile strength and elongation. However, these preliminary prototypes were useful in determining the ideal geometric characteristics of the VidLa-SZ model only. The layer-by-layer fabrication method caused the models to be porous and difficult to sterilize in the absence of additional coats (Fuentes-Alvarez et al., 2021). In addition, the considered materials are not certified as biocompatible and do not withstand high temperatures to be sterilized, making the models non-optimal for being tested on patients. Therefore, an integral prototype of the VidLa-SZ model was developed using Nylon 12 (DuraForm® ProX PA), which is a resistant, biocompatible, and sterilizable thermoplastic that supports applications in the food, engineering, and medicine areas (Berry et al., 1997; Mazzoli, 2013). Nylon 12 also provided an improvement on esthetic attributes without compromising mechanical properties. In the study by Zarringhalam et al. (2006), some of the mechanical properties of Nylon 12 are mentioned, such as being stable over time, preserving its dimensions and mechanical properties.
The VidLa-SZ prototype was manufactured directly by 3D SYSTEMS to ensure a better fabrication quality aiming to carry out sterilization tests on the material and future tests on patients. The 3D printed model parts include the display screen support and handle with blade fused into one piece, as shown in Figure 13. Finally, the medical specialists evaluated the 3D printed device. This evaluation was performed using upper airway simulators available at the CEDDEM of the INCMNSZ.
The instrumentation of the VidLa-SZ model consists of placing the camera inside the cavity located in the distal part of the blade. For this design, it is only allowed to place cameras of 5.5 mm in diameter. The camera cable is placed inside the channel on the side of the blade tab. It passes through the circular hole and the handle and support for the display screen. The cable exits through the top of the support for the display screen and connects to the 4.5-inch screen. The final integration cost of the proposed video-laryngoscope is ∼ USD $400.00. This price includes the acquisition of the 4.5-inch screen, the SLS manufacturing process, transportation, and taxes.
3.3 Evaluation of the VidLa-SZ Video-Laryngoscope
Design validation was the most important evaluation for the design process of the VidLa-SZ since it is an indicator of the performance that it will have to carry out the EI in tests with patients. As a first step, rapid tests of the prototypes made with ABS, PLA, and PLActive were carried out to refine the angle of the camera and length of the blade.
The measurements mentioned above were validated and adjusted by using prototypes of the VidLa-SZ video-laryngoscope. Those were brought in for testing with a simulator of the upper airway. The tests were performed by a resident physician from the anesthesiology service of the INCMNSZ, who has extensive experience in the use of video-laryngoscopes.
The purpose of the tests was to determine the appropriate measurements of the design. The curvature of the proposed blade was evaluated, and the appropriate angle of view was determined to observe the entrance of the glottis. Figure 14 shows the tests carried out with the prototypes, identifying achieving an adequate length of the blade and good camera inclination. It was considered that the VidLa-SZ will show adequate vision according to the Cormack–Lehane classification and will have good properties resisting the endotracheal intubation process. Similarly, with the Nylon 12 prototype, the same device evaluation was carried out in the upper airway simulator.
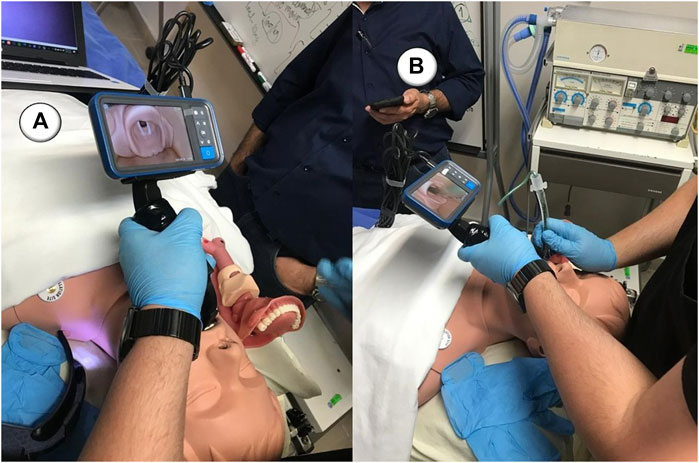
FIGURE 14. Test in the upper airway simulator with the VidLa-SZ video-laryngoscope: (A) vision of the entrance of the glottis and (B) insertion of the endotracheal tube.
The evaluation was performed in the EI procedure using the simulator of the upper airway, repeating the cycle 12 times. Intubation time, the number of attempts, and device status were measured for each iteration. The device’s state was satisfactory because no fracture or crack was detected in the device. At the same time, the iterations were carried out, indicating that the Nylon 12 material resists the loads subjected by the EI procedure in the simulator, despite being made of a rigid material that is difficult to manipulate. On the other hand, it is observed that in the first iterations, more attempts were required and, consequently, longer times to carry out the EI. Figure 15 shows a photograph of the VidLa-SZ video-laryngoscope camera in the airway simulator used in the tests. The epiglottis and the entrance of the glottis can be observed. However, as it is a manikin, the epiglottis does not rise to free the glottic view. When performing the procedure on a patient, placing the video-laryngoscope over the vallecula causes the epiglottis to rise, allowing the inlet of the glottis to be seen. Despite this limitation with the simulator, intubations could be performed.
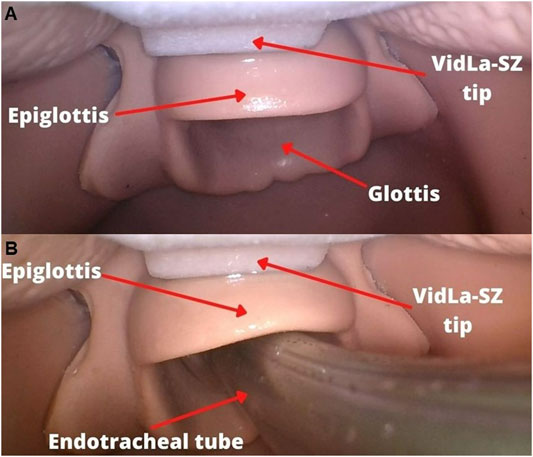
FIGURE 15. Test in the upper airway simulator with the VidLa-SZ video-laryngoscope: (A) vision of the entrance of the glottis and (B) insertion of the endotracheal tube.
As indicated in Section 2, the camera position was estimated so that the tip of the model could be observed in the camera view. This indicated that physicians could more easily locate within the oral cavity and place the video-laryngoscope in the corresponding position to perform a rapid EI.
During the tests, applying lidocaine to the tube and the simulator helped the tube slide easily, which was reflected in a decrease in intubation times after the third iteration. In practice with patients, the tubes tend to slide easily since the anatomical structures are naturally lubricated, although adding lidocaine to the endotracheal tube is common. Expectations were met regarding the anesthesiology resident’s experience using the device with the simulator. The angle of the blade allowed the tube to be placed easily. However, it had to be used as a bougie as a guide to give the blade’s curve to the endotracheal tube. The shape of the handle allowed the device to be held firmly and comfortably, allowing minimal effort compared to a traditional laryngoscope, and finally, the image quality is comparable to that of high-end video-laryngoscopes.
4 Discussion
The development of devices that allow assisting in the endotracheal intubation of patients who require life support through mechanical ventilation is an area that faces challenges derived from the anatomical variations of patients with DA. The devices available today present solutions that although they fulfil their function still leave gaps in the experience of the specialists who perform the EI procedure. The results generated in this work presented a design process, building an integral prototype through additive manufacturing of a video-laryngoscope proposal that allows assisting medical specialists in the EI procedure.
The pandemic caused by the SARS-CoV-2 virus created a shortage of this type of device in hospital units to care for infected patients and protect health personnel. The VidLa-SZ video-laryngoscope is designed to assist in the EI of adult patients who can be intubated with the Macintosh No. 3 blade of the traditional laryngoscope, considering that it is a light, comfortable, and sterilizable device with good image quality and resistance to the efforts at the time of carrying out an EI.
5 Conclusion
The design process made it possible to identify the needs and align the parameters of the concepts with the most important requirements for medical specialists who perform EI. Greater importance was given to the essential criteria for them, which allowed for evaluating the concepts and selecting the materials, the manufacturing process, and the electronic components for device construction.
The static analysis of the model determined the behavior of the proposed geometry when subjected to the loads reported in the literature in force studies during laryngoscopy, demonstrating that the design can avoid great displacements, deformations, or stresses which can affect the structural integrity during the operation of the device due to the safety factor of 3.375.
The construction of the device through additive manufacturing by FDM using ABS, PLA, and PLActive as materials allowed rapid prototypes to validate the design in conjunction with medical specialists in the area of anesthesiology and emergency medicine of INCMNSZ. The device’s construction using SLS using Nylon 12 was the best option for accessibility, resistance, sterilizability, and biocompatibility. The final integration cost of the proposed video-laryngoscope is ∼ USD $400.00. This price includes acquiring the 4.5-inch screen, the SLS manufacturing process, transportation, and taxes. When compared to video-laryngoscopes available on the market, it becomes an affordable option for low-resource healthcare units.
The instrumentation of the device with the 5.5-mm diameter Teslong endoscopic camera and the 4.5-inch screen was easy to assemble and guaranteed a clear, high-quality image.
The evaluation of the device using the upper airway simulator available at the CEDDEM of the INCMNSZ with the medical specialists confirmed that the proposed angle and the position of the camera facilitate insertion of the endotracheal tube using a bougie as a guide, in addition to providing a high-quality image and a comfortable and firm grip for the doctor, reducing the effort compared to a traditional laryngoscope.
Data Availability Statement
The original contributions presented in the study are included in the article/Supplementary Material, further inquiries can be directed to the corresponding author.
Author Contributions
IM-M: conceptualization, literature search and data analysis, original draft, critical revision of the work, and editing. AP: conceptualization, literature search and data analysis, and original draft. RF-A: conceptualization, literature search and data analysis, original draft, critical revision of the work, and editing. JB: critical revision of the work and editing. TH-G: conceptualization and literature search. PS: conceptualization and literature search. LR-V: conceptualization and literature search and critical revision of the work. MR: critical revision of the work. MA-P: literature search, data analysis, original draft, critical revision of the work, and editing.
Conflict of Interest
The authors declare that the research was conducted in the absence of any commercial or financial relationships that could be construed as a potential conflict of interest.
Publisher’s Note
All claims expressed in this article are solely those of the authors and do not necessarily represent those of their affiliated organizations, or those of the publisher, the editors, and the reviewers. Any product that may be evaluated in this article, or claim that may be made by its manufacturer, is not guaranteed or endorsed by the publisher.
Acknowledgments
The authors would like to thank all the doctors, nurses, biomedical engineers, and hospital staff who are fighting this pandemic. We would like you to know that we stand by you in the fight against COVID-19. In addition, the authors would like to thank Fanny Alvarado Chávez from INCMNSZ, Diana Paola Ayala Roldán, and Andrés Kaleb Salinas Arellano from Tecnológico de Monterrey for their valuable help.
References
Agrò, F., Barzoi, G., and Montecchia, F. (2003). Tracheal Intubation Using a Macintosh Laryngoscope or a GlideScope in 15 Patients with Cervical Spine Immobilization. Br. J. Anaesth. 90, 705–706. doi:10.1093/bja/aeg560
Barash, P. G., Cullen, B. F., Stoelting, R. K., Cahalan, M. K., Stock, M. C., Ortega, R., et al. (2018). Anestesia Clínica. Alphen aan den Rijn, Netherlands: Wolters Kluwer.
Berry, E., Brown, J. M., Connell, M., Craven, C. M., Efford, N. D., Radjenovic, A., et al. (1997). Preliminary Experience with Medical Applications of Rapid Prototyping by Selective Laser Sintering. Med. Eng. Phys. 19, 90–96. doi:10.1016/s1350-4533(96)00039-2
Brewster, D. J., Chrimes, N., Do, T. B., Fraser, K., Groombridge, C. J., Higgs, A., et al. (2020). Consensus Statement: Safe Airway Society Principles of Airway Management and Tracheal Intubation Specific to the COVID ‐19 Adult Patient Group. Med. J. Aust. 212, 472–481. doi:10.5694/mja2.50598
Budynas, R. G., and Nisbett, J. K. (2011). Shigley’s Mechanical Engineering Design, Vol. 9. New York: McGraw-Hill.
Burkle, C. M., Zepeda, F. A., Bacon, D. R., and Rose, S. H. (2004). A Historical Perspective on Use of the Laryngoscope as a Tool in Anesthesiology. The J. Am. Soc. Anesthesiologists 100, 1003–1006. doi:10.1097/00000542-200404000-00034
Coloma, R. (2017). Manejo De Vía Aérea No Difícil. Desde La Ventilación Con Bolsa Hasta Intubación Oro Traqueal. Revista Médica Clínica Las Condes 28, 691–700. doi:10.1016/j.rmclc.2017.08.004
Cordovani, D., Russell, T., Wee, W., Suen, A., and Cooper, R. M. (2019). Measurement of Forces Applied Using a Macintosh Direct Laryngoscope Compared with a Glidescope Video Laryngoscope in Patients with Predictors of Difficult Laryngoscopy. Eur. J. Anaesthesiology— EJA 36, 221–226. doi:10.1097/eja.0000000000000901
Crosby, E. T., Cooper, R. M., Douglas, M. J., Doyle, D. J., Hung, O. R., Labrecque, P., et al. (1998). The Unanticipated Difficult Airway with Recommendations for Management. Can. J. Anaesth. 45, 757–776. doi:10.1007/bf03012147
Finucane, B. T., Santora, A. H., and Tsui, B. C.-H. (2003). Principles of Airway Management. Berlin, Germany: Springer.
Frerk, C., Mitchell, V. S., McNarry, A. F., Mendonca, C., Bhagrath, R., Patel, A., et al. (2015). Difficult Airway Society 2015 Guidelines for Management of Unanticipated Difficult Intubation in Adults † †This Article Is Accompanied by Editorials Aev298 and Aev404. Br. J. Anaesth. 115, 827–848. doi:10.1093/bja/aev371
Frova, G., and Sorbello, M. (2009). Algorithms for Difficult Airway Management: a Review. Minerva Anestesiol 75, 201–209.
Fuentes-Alvarez, R., Alfaro-Ponce, M., Alvarado, F., Mora-Galvan, J. A., Fuentes-Aguilar, R. Q., and Matehuala Moran, I. (2021). Full-face Mask Adapter as Covid-19 Personal Protective Equipment: Design, Tests and Validation. IEEE Latin Am. Trans. 19, 986–993. doi:10.1109/tla.2021.9451244
Garvin, R. (2020). Intubating the Critically Ill Patient: A Step-by-step Guide for Success in the ED and ICU. Berlin, Germany: Springer.
Gupta, S., Sharma, R., and Jain, D. (2005). Airway Assessment: Predictors of Difficult Airway. Indian J. Anaesth. 49, 257–262.
Henderson, J. J., Popat, M. T., Latto, I. P., and Pearce, A. C. (2004). Difficult Airway Society Guidelines for Management of the Unanticipated Difficult Intubation. Anaesthesia 59, 675–694. doi:10.1111/j.1365-2044.2004.03831.x
Huang, Z., Zhuang, D., Xiong, B., Deng, D. X., Li, H., and Lai, W. (2020). Occupational Exposure to Sars-Cov-2 in burns Treatment during the Covid-19 Epidemic: Specific Diagnosis and Treatment Protocol. Biomed. Pharmacother. 127, 110176. doi:10.1016/j.biopha.2020.110176
Jaber, S., Amraoui, J., Lefrant, J.-Y., Arich, C., Cohendy, R., Landreau, L., et al. (2006). Clinical Practice and Risk Factors for Immediate Complications of Endotracheal Intubation in the Intensive Care Unit: A Prospective, Multiple-center Study*. Crit. Care Med. 34, 2355–2361. doi:10.1097/01.ccm.0000233879.58720.87
Jaber, S., Jung, B., Corne, P., Sebbane, M., Muller, L., Chanques, G., et al. (2010). An Intervention to Decrease Complications Related to Endotracheal Intubation in the Intensive Care Unit: a Prospective, Multiple-center Study. Intensive Care Med. 36, 248–255. doi:10.1007/s00134-009-1717-8
Kaplan, M. B., Ward, D. S., and Berci, G. (2002). A New Video Laryngoscope-An Aid to Intubation and Teaching. J. Clin. Anesth. 14, 620–626. doi:10.1016/s0952-8180(02)00457-9
Lapinsky, S. E. (2015). Endotracheal Intubation in the Icu. Crit. Care 19, 1–3. doi:10.1186/s13054-015-0964-z
Lavery, G. G., and McCloskey, B. V. (2008). The Difficult Airway in Adult Critical Care. Crit. Care Med. 36, 2163–2173. doi:10.1097/ccm.0b013e31817d7ae1
Longhitano, G. A., Nunes, G. B., Candido, G., and da Silva, J. V. L. (2021). The Role of 3d Printing during Covid-19 Pandemic: a Review. Prog. Addit Manuf 6, 19–37. doi:10.1007/s40964-020-00159-x
Ludwin, K., Bialka, S., Czyzewski, L., Smereka, J., Dabrowski, M., Dabrowska, A., et al. (2020). Video Laryngoscopy for Endotracheal Intubation of Adult Patients with Suspected/confirmed Covid-19. A Systematic Review and Meta-Analysis of Randomized Controlled Trials. Disaster Emerg. Med. J. 5, 85–97. doi:10.5603/demj.a2020.0023
Mayrose, J., Kesavadas, T., Chugh, K., Joshi, D., and Ellis, D. G. (2003). Utilization of Virtual Reality for Endotracheal Intubation Training. Resuscitation 59, 133–138. doi:10.1016/s0300-9572(03)00179-5
Mazzoli, A. (2013). Selective Laser Sintering in Biomedical Engineering. Med. Biol. Eng. Comput. 51, 245–256. doi:10.1007/s11517-012-1001-x
Meng, L., Qiu, H., Wan, L., Ai, Y., Xue, Z., Guo, Q., et al. (2020). Intubation and Ventilation amid the COVID-19 Outbreak. Anesthesiology 132, 1317–1332. doi:10.1097/aln.0000000000003296
Noppens, R. R., Geimer, S., Eisel, N., David, M., and Piepho, T. (2012). Endotracheal Intubation Using the C-MAC® Video Laryngoscope or the Macintosh Laryngoscope: a Prospective, Comparative Study in the ICU. Crit. Care 16, R103–R108. doi:10.1186/cc11384
Orebaugh, S. L., and Bigeleisen, P. E. (2012). Atlas of Airway Management: Techniques and Tools. Philadelphia, Pennsylvania, USA: Lippincott Williams & Wilkins.
Orser, B. A. (2020). Recommendations for Endotracheal Intubation of Covid-19 Patients. Anesth. Analgesia 130, 1109–1110. doi:10.1213/ane.0000000000004803
Pasnick, S., Carlos, W. G., Dela Cruz, C. S., Gross, J. E., Garrison, G., and Jamil, S. (2020). Sars-cov-2 Transmission and the Risk of Aerosol-Generating Procedures. Am. J. Respir. Crit. Care Med. 202, P13–P14. doi:10.1164/rccm.2024p13
Rajeswaran, P., Hung, N.-T., Kesavadas, T., Vozenilek, J., and Kumar, P. (2018). “Airwayvr: Learning Endotracheal Intubation in Virtual Reality,” in 2018 IEEE conference on virtual reality and 3D user interfaces (VR) (Tuebingen, Germany: IEEE), 669–670. doi:10.1109/vr.2018.8446075
Rosen, P., Sloane, C., Ban, K. M., Lanigra, M., and Wolfe, R. (2006). Difficult Airway Management. Int. Emerg. Med 1, 139–147. doi:10.1007/bf02936542
Russell, T., Khan, S., Elman, J., Katznelson, R., and Cooper, R. M. (2012). Measurement of Forces Applied during Macintosh Direct Laryngoscopy Compared with GlideScopevideolaryngoscopy*. Anaesthesia 67, 626–631. doi:10.1111/j.1365-2044.2012.07087.x
Sagarin, M. J., Barton, E. D., Chng, Y.-M., and Walls, R. M.National Emergency Airway Registry Investigators (2005). Airway Management by Us and canadian Emergency Medicine Residents: a Multicenter Analysis of More Than 6,000 Endotracheal Intubation Attempts. Ann. Emerg. Med. 46, 328–336. doi:10.1016/j.annemergmed.2005.01.009
Schächter, S. (2019). Destrezas médicas para salvar vidas (una guía de primeros auxilios). Mexico City: Schächter, Salomón.
Soria, C. S., Lee, D. E., Lee, M., and Manecke, G. R. (2021). Anesthesiology Resident Manual of Procedures: A Step-by-step Guide. Berlin, Germany: Springer Nature.
Sun, D. A., Warriner, C. B., Parsons, D. G., Klein, R., Umedaly, H. S., and Moult, M. (2005). The GlideScope Video Laryngoscope: Randomized Clinical Trial in 200 Patients. Br. J. Anaesth. 94, 381–384. doi:10.1093/bja/aei041
Weissman, D. N., De Perio, M. A., and Radonovich, L. J. (2020). Covid-19 and Risks Posed to Personnel during Endotracheal Intubation. Jama 323, 2027–2028. doi:10.1001/jama.2020.6627
Winton, J., Celenza, A., and Jackson, T. (2008). Improving Documentation of Endotracheal Intubation in an Adult Emergency Department. Emerg. Med. Australasia 20, 488–493. doi:10.1111/j.1742-6723.2008.01134.x
Keywords: additive manufacturing, finite element method, video-laryngoscope, endotracheal intubation, biomedical design
Citation: Matehuala-Morán I, Pino Pérez AY, Fuentes-Alvarez R, Beltrán Fernández JA, Hernandez-Gilsoul T, Saldaña Villaseñor PA, Rojas-Vega L, Ramírez Cadena MdJ and Alfaro-Ponce M (2022) Design and Additive Construction of a Video-Laryngoscope for Endotracheal Intubation of Adult Patients. Front. Mater. 9:906851. doi: 10.3389/fmats.2022.906851
Received: 29 March 2022; Accepted: 13 April 2022;
Published: 27 May 2022.
Edited by:
Mika SalmiMika Salmi, Aalto University, FinlandReviewed by:
Anju Gupta, All India Institute of Medical Sciences, IndiaNina Pirlich, Johannes Gutenberg University Mainz, Germany
Copyright © 2022 Matehuala-Morán, Pino Pérez, Fuentes-Alvarez, Beltrán Fernández, Hernandez-Gilsoul, Saldaña Villaseñor, Rojas-Vega, Ramírez Cadena and Alfaro-Ponce. This is an open-access article distributed under the terms of the Creative Commons Attribution License (CC BY). The use, distribution or reproduction in other forums is permitted, provided the original author(s) and the copyright owner(s) are credited and that the original publication in this journal is cited, in accordance with accepted academic practice. No use, distribution or reproduction is permitted which does not comply with these terms.
*Correspondence: Ruben Fuentes-Alvarez, am9ydS5mdWFAdGVjLm14