- 1Department of Engineering “Enzo Ferrari”, University of Modena and Reggio Emilia, Modena, Italy
- 2Department of Chemical Sciences and Materials Technologies-DSCTM, Institute of Condensed Matter Chemistry and Technologies for Energy-ICMATE, National Research Council of Italy-CNR, Padova, Italy
- 3School of Science and Technology, University of Camerino, Camerino, Italy
- 4Department of Industrial Chemistry ‘‘Toso Montanari”, University of Bologna, Bologna, Italy
This article highlights recent experimental advances in the use of inorganic substances in the encapsulation of pollutants and, in particular, discusses the potential applicability and constraints of the geopolymerization process for the treatment of wastewater containing chromium. A great percentage of waste containing chromium salts is produced by the leather industry during the tannery process. Such industrial waste is in the form of liquor containing almost 40% of the initial chromium combined with many other pollutants. The stabilization/solidification (S/S) treatment of this type of waste must be combined with chromium encapsulation in an economic, environmentally friendly and efficient process to be industrially feasible. Here we present a novel process in which the wastewater is used as a component of the formulation together with a clay by-product and with the addition of NaOH pellets with the goal of a no-water plus no-waste technology approach. The final solidified “ceramic-like” material successfully immobilized the heavy metal cations as well as anions and macromolecules of surfactants, avoiding environmental damages to soil and groundwater. The article is completed by mentioning other S/S processes where wastewater has been treated and the resulting sludge encapsulated. The future of the S/S technologies in the tannery industry should progress in the direction of significantly reducing the amount of wastewater directed to the treatment plants, with associated reductions in transport and their CO2 emissions. This article intends to be a contribution in the direction of preventing waste, aligning circular economy and waste management objectives.
Introduction
Heavy metal cations are well-known environmental issues opposed, yet not resolved, by efficient encapsulation technologies which can be accompanied by stabilization/solidification processes. As an example, cesium can be encapsulated in a sulfoaluminate cement matrix by a physical effect (Li et al., 2021) whilst for mercury, the sulfur polymer stabilization/solidification, or the chemically bonded phosphate ceramic encapsulation, as well as the polyethylene encapsulation are the adopted strategies (Randalla and Chattopadhyay, 2004). Among the numerous encapsulation or stabilization/solidification methods: in phosphate matrices (Tang et al., 2013), in ceramic products (Wei et al., 2005; Castañeda Bocanegra et al., 2017), or in complex oxides (Kuganathan et al., 2019), those based on cement (Giergiczny and Król, 2008; Chen et al., 2009) or zeolite-like inorganic materials are recognised worldwide as being among the cheapest and simplest in terms of number of operations (Pimraksa et al., 2013).
The application of the “green economy” concept of re-use has encouraged many researchers to optimize the reuse of heavy metal-bearing wastes and residues for the reproduction or making new products. Zeolite-like materials obtained from reuse of residues (Pimraksa et al., 2013; Wang et al., 2019) have been mostly optimized for high efficiency removal of heavy metals in wastewater. Also zinc slag is used by Duan et al. (2021) to process copper smelting wastewater containing high arsenic concentration. The cementification of heavy metals suffers from certain limitations due to the co-presence of anions, chief among them being chlorides, and the possible excess of water and/or organic and oily substances (Ebert and Kirkelund, 2022). In order to minimize such adverse effects on cement fresh paste or hardened properties, several admixtures of minerals: zeolites (Bagosi and Csetenyi 1999), bagasse ash (Tantawy et al., 2012), metakaolin (Lancellotti et al., 2015), slag (Zhang et al., 2017), fly ash based geopolymer (Kupwade-Patil et al., 2014; Wang et al., 2020) or macromolecules: latexes and acrylic polymers, naphthalene- and chitosan—derivatives have been proposed (Stampino et al., 2009).
Aim of This Perspective Article
The reduction of costs in S/S technologies will assure their adoption whenever they are required as a substitution to landfilling. Thus a number of recent investigations have been carried out mainly in those countries where disposal is reduced or forbidden (Silva et al., 2007; Study IP/G/STOA/FWC/2013-001/LOT 3/C3, 2017; DIRECTIVE 2018/850, 2018; UN-The Sustainable Development Goals Report, 2018; Ferronato and Torretta 2019). The heavy metal encapsulation for re-use and even for stable disposal is required more and more to avoid dangerous leaching of toxic species (Al-Kindi, 2019). Some studies differentiate the cases of heavy metals that include nickel (Ni), lead (Pb), cadmium (Cd), chromium (Cr), copper (Cu), cobalt (Co.), zinc (Zn), manganese (Mn), aluminum (Al) and mercury (Hg), and heavy metalloids, antimony (Sb), arsenic (As), and tellurium (Te), the latest being more sensitive to basic environments with respect to the metals. It should be noted that As, Pb, Cd and Hg are included in the top 20 Hazardous Substances of the Agency for Toxic Substances and Disease Registry (ATSDR, 2012) and the United States Environmental Protection Agency (US EPA). Chromium is one of the most dangerous heavy metals, being present in two forms: the cationic one, i.e., Cr+3 ions, and the anionic form, typical of oxidative environment, the Cr+6 within the chromate molecular group, CrO42- (Pettine and Capri, 2005; Iyaka, 2009; Liang et al., 2021). In Italy, the highest Cr(VI) concentrations allowed in soil is 15 mg/kg, depending on whether it is exploited for parkland or industrial uses (Decree 471, 1999). Although Cr3+ is stable in the environment, it can be oxidized, under certain conditions, to the more dangerous Cr6+. Therefore, industrial wastes with a high concentration of chromium (III) salts, for example in tanning industry, require appropriate treatments to stabilize Cr3+ to prevent the oxidation processes (Giovannini et al., 2008; Zhang et al., 2022). A recent encapsulation process has been presented by Zhao and colleagues adopting the TiO2 precipitation route in a ZnCr2O4 spinel like crystal at 1,000°C, by adding the same amount of ZnO2 and TiO2 20wt%, (Zhao et al., 2022)., Other authors approached a less expensive and more friendly encapsulation methods as reported and discussed below (Montañés et al., 2014; Pantazopoulou and Zouboulis 2018; Oproiu et al., 2021; Boldrini et al., 2021).
In this perspective article we want to present the case of the Cr-bearing wastewater and their efficient encapsulation technologies, concluding with novel experimental results of a preliminary study carried out to compare the advantages and disadvantages of metakaolin- and uncalcined clay-based geopolymerization as S/S technology employed for treating leather wastewater. Since a modern and eco-friendly approach for waste management recommends the combination of wastes and by-products (Daniil et al., 2018), we propose the employment of a stabilizing matrix deriving from industrial by-products in the S/S process of wastewaters.
Encapsulation Treatments of Cr-Bearing Wastewater From Tannery Industry
The leather industry utilizes almost 90% of chromium-based tanning agents for converting raw skins/hides into leather. About 40% of the used chromium (III) salts are discarded as wastewater (Pradeep et al., 2021).
Apart from chromium, metals such as aluminum, titanium, iron, and zirconium are widely used for various end-applications. Hence, the effluent after the tanning processes contains high concentration of heavy metals and very high concentration of anions, among them Cl1- and SO42- (Boldrini et al., 2021). This great amount of liquor contains a high concentration of chromium sulphate, and can be processed to recover, and hence to re-use, the tanning salt by water evaporation or treated by chemical methods to remove the Cr3+ by precipitation producing large amounts of slurry to be disposed of (El-Shafey et al., 2005). Though Cr(III) is less toxic than Cr(VI), there is a higher risk of oxidation during the subsequent treatment processes. Therefore, several methodologies have been developed to remove the heavy metals from the effluent before processing it in wastewater purification plants (Šillerová et al., 2015). Many approaches have been pursued to stabilize the tannery waste, rich mainly in the cationic form, Cr3+, and poor in the anionic form, CrO42- (Nur-E-Alam et al., 2020). In a recent review, the tannery sludge and wastewater have been listed together with other Cr-bearing wastes treated with glass-ceramics, sintered ceramics, cement, and geopolymers (Zhang et al., 2022). Most of those technologies require thermal treatments, while only cementification and alkali activation, sometimes indicated as geopolymerization, can occur at room temperature. The last two technologies have attracted several researchers from industry (Leonelli et al., 2014; Ponzoni et al., 2015) stating the actual feasibility on-site in the tannery plant.
Among the encapsulation technologies, those efficient at room temperature can be listed in function of the starting native waste and the number of process’ stages (Figure 1). A number of processes start from the ash (Daniil et al., 2018) or the char (Oh et al., 2014) derived from post thermal treatments of tannery sludge, as indicated in the 4-steps process 1 (Figure 1). In the process 2 (Pantazopoulou and Zouboulis 2018) and in process 3 (Montañés et al., 2014; Haque et al., 2019) of Figure 1 the industrial tannery wastes first undergo a chemical/physical treatment to precipitate-concentrate chromium salt in sludge at various water contents. After this preliminary step, filterpressing may occur (process 2), followed by the true S/S or encapsulation processes using different host materials and different synthetic routes to immobilize Cr in solid, safe for disposal in landfills for non-hazardous wastes. All these processes are water-, energy-, and time-consuming. These first three processes when compared in terms of Cr-retention percentage results above 95%. Recently, we have proposed an innovative single-step process (process 4 in Figure 1) based on metakaolin alkali activation to encapsulate chromium in native wastewater derived from tanning industries (Boldrini et al., 2021). The wastewater was used coming from the tanning drum without any treatment and, hence, without any addition of new clean water, energy or time. The process is efficient also in the stabilization of all the other polluting substances present in the liquor with a performance equal to or better than the process 2 and 3 (Figure 1). A direct comparison of our process with literature is possible with process 3 (Montañés et al., 2014) finding a similar increase in final product mass, but with a lower amount of chromium in the leachate, 0.024 vs. 194.7 ppm for process 4 and process 3, respectively (Boldrini et al., 2021). The chromium content in the leachate from process 2 and process 3 is greater than the fixed limit by the European regulation for disposal in non-hazardous landfill. The leaching out of total Cr of the final materials achieved from process 1 and process 4, as well as the leaching of other cations and anions, are suitable for re-use with economic advantage over the disposal costs, advantages that are more evident for single-step process 4. Cr-retention percentage for process 4 was recorded to be above 99% (for more details on the efficiency of the retention process, refer to details reported in Table 3, Boldrini et al., 2021).
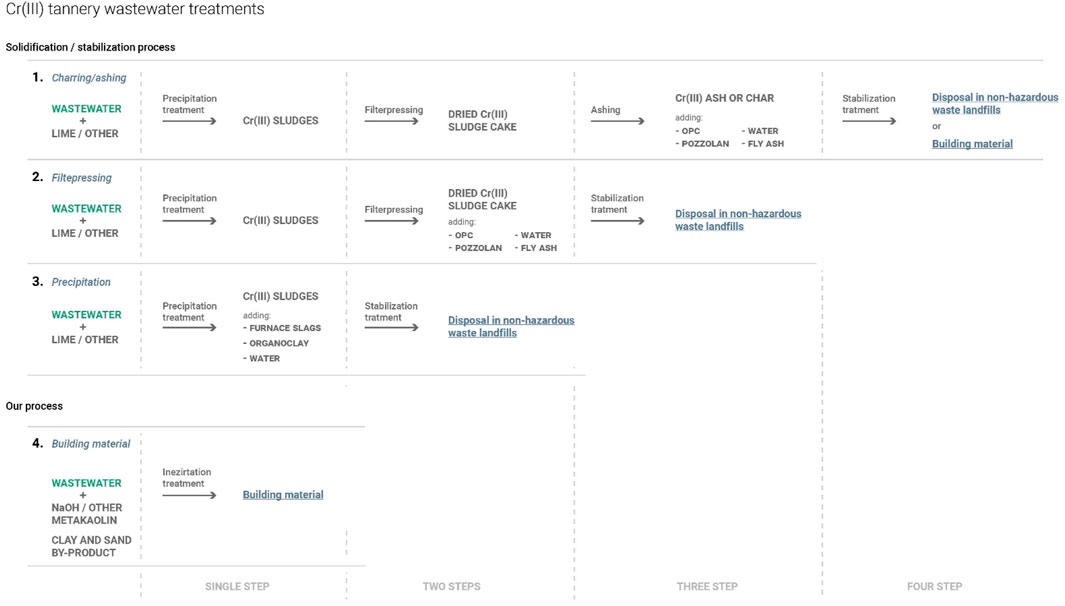
FIGURE 1. Simplified sketch summarizing the encapsulating or solidification/stabilization procedures adopted for Cr(III)-containing tannery wastewater elaborated from Refs Montañés et al. (2014), Daniil et al. (2018), Pantazopoulou and Zouboulis (2018), Haque et al. (2019), Boldrini et al. (2021).
Our process (Boldrini et al., 2021), shows the limitation of costly raw materials, i.e., metakaolin and sodium silicate solution. A new perspective of the geopolymeration approach will be presented hereafter with the use of unfired clay and activation solution composed only of NaOH, both reactants of lower economic impact for the tannery industry.
Direct Geopolymerization of Cr-Bearing Wastewater From Tannery Industry
New experimental results are hereafter presented to substantiate the possibility for production of an efficient encapsulating matrix for Cr-bearing wastewater using a clay by-product combined with a sandy by-product, both deriving from mine processing (Sgarlata et al., 2021). A successful formulation of a geopolymer capable of encapsulating up to 6,600 ppm of Cr in the native wastewater has been already optimized via alkali activation of a commercial metakaolin (Boldrini et al., 2021). The metakaolin-based geopolymer had a formulation which allowed a proper Cr encapsulation, including different anions as well as other macromolecules, such as surfactants, in a zeolite-like bonding environment for Cr+3, demonstrating also the total absence of Cr6+ (Giorgetti et al., 2020; Pinakidou et al., 2021). In the paper by Giorgetti et al. (2020) the EXAFS analysis highlighted around the chromium atom a first shell of 6 oxygens and a second shell of 7/8 Al or Si atoms demonstrating a strong chemical link to the aluminosilicate skeleton.
In this paragraph we present the recent results proving the feasibility of low-cost aluminosilicate powder as base for the formulation of a geopolymer capable of encapsulating a large number of contaminants present in one real waste from the leather industry: Cr-bearing wastewater of the tannery process rich also in Cl1- and SO42- anions.
The novel experimental results presented hereafter are related to one formulation where a clayey mining by-product (Sgarlata et al., 2022) totally replaced a commercial metakaolin (Argical™ M1000, produced by Imerys, France) with quartz, muscovite/illite, and anatase as principal crystalline phases and 55-59 wt% SiO2, 34-40 wt% Al2O3 as major chemical constituents, D90 = 40 ± 2 µm (complete particle size distribution curves are presented in Supplementary Figure S1), and surface area = 18 ± 2 m2/g. The mining clayey by-product was the result of an efficient industrial waste recovery process for reuse as secondary raw materials mined in Lazio, in the province of Latina, Italy. Resulting composed of 35%–38% halloysite clay, i.e., 52-55 wt% SiO2 and 26-30 wt% Al2O3. The need for reactive silica was compensated with the addition of 100% of fine sand (sand being the same weight of the clay in all the mixtures). This sand is another mining by-product derived from quartz sand processing in the Robilante plant (Italy), with 67 wt% of quartz and 27 wt% of mica/illite, and primarily characterized by oxides: 82 wt% SiO2, 10wt% Al2O3 and 4wt% K2O. These two by-products have been chosen also for their fineness, having a D90 of 18 ± 1 µm (Supplementary Figure S1) for the clay and 23 ± 1 µm (Supplementary Figure S1) and surface area of 36 ± 4 m2/g the clay and 4.58 ± 0.07 m2/g the sand, both calculated with B.E.T. method. (Please notice that the error accompanying these measurements represent the variability of the raw materials). The Cr-bearing industrial wastewater was used as received (4,100 ppm of total Cr) and after concentration (6,642 ppm of total Cr) to produce the alkali activating solution after addition of NaOH pellets (Carlo Erba, Italy, reactant grade) to reach 8 mol/L concentration. The complete chemical composition of the wastewater has already been reported (Boldrini et al., 2021). The alkali activated paste was produced by mixing 30.5% uncalcined clay, 30.5% sand, 3.4% NaOH in 11.2% wastewater plus additional water, depending on the concentration of heavy metals (percentages are calculated on the weight of the overall fresh paste). The fresh paste thus obtained was stirred with a mechanical mixer for approx. 10 min, and when a good homogeneity was assured, it was poured into a mould. Finally, the samples were cured at 40°C and controlled relative humidity for 7 days, and then at room temperature until reaching the 28th day of ageing prior further characterization.
The effective capability to retain the waste components has been tested on the final solid material according to the Italian and European regulation at diverse ageing times, 30 days, and 5 months, by performing EN 12457–2: 2004 leaching tests (EN 12457-2:2004., 2004). The methodologies and instruments used are detailed in ref. Boldrini et al. (2021).
Uncalcined Clay Geopolymer and its Encapsulation Efficiency for Heavy Metals
Leaching test results of the uncalcined clay (this work) and metakaolin-based geopolymers (Boldrini et al., 2021) are reported in Figure 2. The values are expressed as retention percentage calculated on the basis of the total wastewater load and the leached fraction. Together with Cr, we have also investigated the retention ability of other heavy metal cations as well as the anions present in the wastewater. In the Supplementary Material we report the complete characterization with leaching values in ppm vs. regulation limits for disposal in landfill of inert waste, non-hazardous waste and hazardous waste (Supplementary Table S1). The effect of concentration of the contaminants in the wastewater has been investigated by adding to the geopolymer paste in one case 636 ppm of Cr (Figure 2A) and 1,023 ppm in the second case (Cr amounts are referred to the overall fresh paste) (Figure 2B).
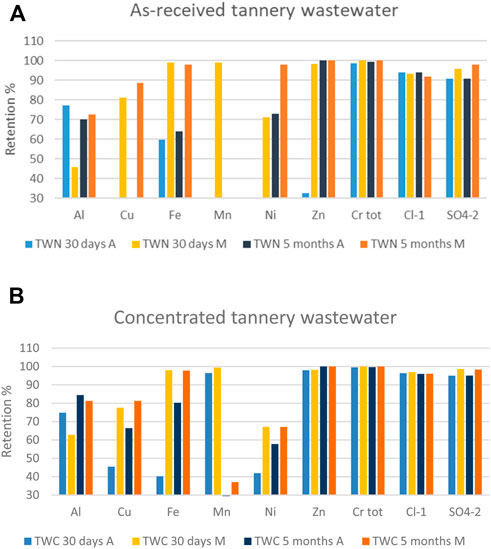
FIGURE 2. Comparison of retained percentage of elements and anions by part of clay-based geopolymers (indicated with A) vs. metakaolin-based geopolymers (indicated with M) after 30 days and 5 months of curing time. The tannery wastewater was added as-received (A) or concentrated 10 times (B).
The cations and anions retention values show that the uncalcined clay is equivalent to the metakaolin encapsulating efficiency in the proposed alkali activation process. Uncalcined clay-base formulation (indicated as A in Figure 2) has a slightly lower retention values for some cations only after 30 days of curing, while after 5 months the two matrices have consolidated an efficient 3D aluminosilicate network capable to chemically entrap up to 100% of all the contaminants.
From a regulation point of view, the retention efficiency is not the point, but the attention is posed upon the leachate amount. The results of the leachate cations and anions, accordingly to the EN 12457–2-2004 Test, are reported in the Supplementary Table S2, SupplementaryFigure S2 and show that the A series has leaching values below legal limit for the disposal site of non-hazardous waste (2003/33/EC, 1999) for all the anions (Cl1- and SO42-) and for all the cations, except Cr. Jointly with this observation, the leaching values for A formulation are slightly above the legal limits for disposal sites of inert waste. Such an achievement is really significant for its similarity with leaching values recorded for the metakaolin-based formulation, which are 60% lower, reaching the legal values suitable for re-use. Both geopolymer formulations, based on metakaolin or unfired clay, present a dense and homogeneous solid microstructure (see as an example Supplementary Figure S3) suitable to produce building materials with compressive strength around 25–33 MPa. Additionally, the workability range of the fresh pastes is suitable for industrial mold filling, thus assuring a feasible scale-up of the process 4.
Summary and Future Research Directions
Within the role of waste management in the context of a circular economy transition, the novel results presented in this perspective article substantiated the advancement in the Cr ions encapsulation in alkali activated aluminosilicate powders. Even though the entrapment mechanism still deserve additional investigations, mainly as far as anions are concerned, the feasibility has been demonstrated for processing in a single step an industrial wastewater containing high amounts of chromium via alkali activation of two industrial by-products, an halloysite clay and a sand washing residue. These two powdery raw materials can substitute, if used in appropriate proportion, the more expensive metakaolin and the sodium silicate in a cheaper formulation. The workability of the paste encounters the requirements for a fast scale-up and the mechanical performance falls in the range of non-structural building materials.
The experimental data from process 3 and process 4 (Figure 1) have been obtained using a particularly high concentrated Cr-bearing wastewater (up to 6,600 ppm). The consolidated geopolymeric dense product contains up to 607 and 977 ppm using native and concentrated wastewater, respectively. The high retention capability of the materials tested in these two processes are above 99%, being 99,55% for native waste and 99.64% for concentrated waste. The leachate content of Cr ions is very close to the legal limit for reuse. In the case of clay by-product, the amount of wastewater should be lower than the amount used in the metakaolin matrix. Considering the most typical Cr-content in tannery wastewater, around 1,000–3,000 ppm (Nur-E-Alam et al., 2018; Parañaque et al., 2020), we can propose the unfired clay formulation as appropriate for the Cr-entrapment. Process 1 and process 2 (Figure 1), stabilizing wastes with up to 385 ppm, resulted in consolidated materials to be disposed of in hazardous waste dumps since retention was not that high.
The driving force of the industrial encapsulation technology of wastes is the reduction of the cost of the process in terms of:
1) number of step process,
2) energy costs,
3) reactants costs,
4) water consumption,
We are confident that the proposed process (process 4 in Figure 1) could be further investigated being its Cr-retention higher that 99%. Its on-site application could take place in those countries where the leather industry produces the majority of Cr-bearing wastes. The same process should be applied to other classes of heavy metals-bearing wastes, not necessarily deriving from the tannery industry. The solid final product could be proposed for non-structural applications in the building sector concurring to achieve a ECOLABEL being prepared from 3 different wastes or by-products: wastewater, clay and sand. Additional investigations have seen the unfired clay maintained as the principal component of the encapsulating matrix, while limited (typically 5wt% of the unfired clay mass) additions of metakaolin and other fired clay have been found to higher retention values. These studies are still under completion, as well as those proposing, Al-bearing additives representing a source of Al+3 species in the alkaline media. Such availability of aluminium ions for the 3D reticulation of the aluminosilicate network is functional to increment the encapsulating power for Cr+3 ions in the aluminosilicate matrix.
Improving the efficiency and effectiveness of the current system, process 4 in Figure 1, with better formulation or curing treatment is another challenge. The research of the investigation to provide a suitable matrix for the stabilization of Cr-rich tannery waste should be consistent with the “end-of-waste” concept, whereby wastes are re-used as raw materials (Waste Framework Directive, 2008).
Data Availability Statement
The original contributions presented in the study are included in the article/Supplementary Material, further inquiries can be directed to the corresponding authors.
Author Contributions
All authors listed have made a substantial, direct, and intellectual contribution to the work and approved it for publication.
Conflict of Interest
The authors declare that the research was conducted in the absence of any commercial or financial relationships that could be construed as a potential conflict of interest.
Publisher’s Note
All claims expressed in this article are solely those of the authors and do not necessarily represent those of their affiliated organizations, or those of the publisher, the editors and the reviewers. Any product that may be evaluated in this article, or claim that may be made by its manufacturer, is not guaranteed or endorsed by the publisher.
Acknowledgments
The authors wish to thank Eng. Alessandria Formia, SIBELCO Ankerpoort NV, Maastricht, The Netherlands, and Eng. Francesco Ferrari, Sibelco Italia S.p.A., Maranello (MO), Italy; for providing the uncalcined clayey by-product and the sandy by-product. Authors are also grateful to Dr. Mirko Braga and Mr. Pasquale Pansini from Ingessil, Montorio, Verona, Italy for providing the sodium silicate solutions used in this work and JH Conceria del Chienti spa Tolentino, Italy, for the tannery wastewater. Authors are grateful to Eng. Gregorio Vaccari, University of Modena and Reggio Emilia, for his help with the preparation of the figures as well as to Eng. Joseph Cross for the careful revision of the use of the English language.
Supplementary Material
The Supplementary Material for this article can be found online at: https://www.frontiersin.org/articles/10.3389/fmats.2022.904580/full#supplementary-material
References
2003/33/EC (1999). 2003/33/EC: Council Decision of 19 December 2002 Establishing Criteria and Procedures for the Acceptance of Waste at Landfills Pursuant to Article 16 of and Annex II to Directive 1999/31/EC. Available at: https://eur-lex.europa.eu/eli/dec/2003/33(1)/oj (Accessed May 23, 2022).
Al-Kindi, G. (2019). Evaluation the Solidification/stabilization of Heavy Metals by Portland Cement. J. Ecol. Eng. 20 (3), 91–100. doi:10.12911/22998993/99739
Bagosi, S., and Csetenyi, L. J. (1999). Immobilization of Caesium-Loaded Ion Exchange Resins in Zeolite-Cement Blends. Cem. Concr. Res. 29, 479–485. doi:10.1016/s0008-8846(98)00190-2
Boldrini, G., Sgarlata, C., Lancellotti, I., Barbieri, L., Giorgetti, M., Ciabocco, M., et al. (2021). Efficient Chemical Stabilization of Tannery Wastewater Pollutants in a Single Step Process: Geopolymerization. Sustain. Environ. Res. 31, 33. doi:10.1186/s42834-021-00106-7
Castañeda Bocanegra, J. J., Espejo Mora, E., and Cubillos González, G. I. (2017). Encapsulation in Ceramic Material of the Metals Cr, Ni, and Cu Contained in Galvanic Sludge via the Solidification/stabilization Method. J. Environ. Chem. Eng. 5 (4), 3834–3843. doi:10.1016/j.jece.2017.07.044
Chen, Q. Y., Tyrer, M., Hills, C. D., Yang, X. M., and Carey, P. (2009). Immobilization of Heavy Metal in Cement - Based Solidification/stabilisation: A Review. Waste Manag. 29, 390–403. doi:10.1016/j.wasman.2008.01.019
Daniil, A., Dimitrakopulos, G. P., Varitis, S., Vourlias, G., Kaimakamis, G., Pantazopoulou, E., et al. (2018). Stabilization of Cr-Rich Tannery Waste in Fly Ash Matrices. Waste Manag. Res. 36 (9), 818–826. doi:10.1177/0734242X18775488
Decree 471 (1999). Decreto Ministeriale N. 471, Gazzetta Ufficiale Supplemento Ordinario N. 293. Available at: https://www.arpa.veneto.it/suolo/docs/leggi/DM471_25_12_99_D49.pdf.
DIRECTIVE (EU) 2018/850 (2018). Directive (EU) 2018/850 of the European Parliament and of the Council of 30 May 2018. Available at: https://eurlex.europa.eu/legalcontent/EN/TXT/PDF/?uri=CELEX:32018L0850&rid=3 (Accessed May 23, 2022).
Duan, X., Li, X., Li, Y., Qi, X., Li, G., Lu, Z., et al. (2021). Separation and Stabilization of Arsenic in Copper Smelting Wastewater by Zinc Slag. J. Clean. Prod. 312, 127797. doi:10.1016/j.jclepro.2021.127797
Ebert, B. A. R., and Kirkelund, G. M. (2022). Effects of Chlorides and Sulphates on Heavy Metal Leaching from Mortar with Raw and Electrodialytically Treated MSWI Fly Ash. Waste Biomass Valor 13, 2673–2688. doi:10.1007/s12649-022-01686-0
El-Shafey, E. I., Correia, P. F. M., and De Carvalho, J. M. R. (2005). Tannery Waste Treatment: Leaching, Filtration and Cake Dewatering Using a Membrane Filter Press (A Pilot Plant Study). Sep. Sci. Technol. 40 (11), 2297–2323. doi:10.1080/01496390500201474
EN 12457-2:2004 (2004). Characterisation of Waste - Leaching - Compliance Test for Leaching of Granular Waste Materials and Sludges - Part 2: One Stage Batch Test at a Liquid to Solid Ratio of 10 L/kg for Materials with Particle Size below 4 Mm (Without or with Size Reduction). Published date: 01-01-2004. Available at: https://standards.iteh.ai/catalog/standards/cen/db6fbdf3-1de7-457c-a506-46c4898e3f09/en-12457-2-2002 (Accessed May 23, 2022).
Ferronato, N., and Torretta, V. (2019). Waste Mismanagement in Developing Countries: A Review of Global Issues. Ijerph 16 (6), 1060. doi:10.3390/ijerph16061060
Giergiczny, Z., and Król, A. (2008). Immobilization of Heavy Metals (Pb, Cu, Cr, Zn, Cd, Mn) in the Mineral Additions Containing Concrete Composites. J. Hazard. Mater. 160 (2-3), 247–255. doi:10.1016/j.jhazmat.2008.03.007
Giorgetti, M., Berrettoni, M., Aquilanti, G., Boldrini, G., Lancellotti, I., and Leonelli, C. (2020). The Coordination Core and Charge of Chromium in Metakaolin-Geopolymers as Revealed by X-Ray Absorption Spectroscopy. Mater. Lett. 270, 127741. doi:10.1016/j.matlet.2020.127741
Giovannini, J. G., Tavares, G. A., and Bendassolli, J. A. (2008). Avaliação das técnicas de precipitação química e encapsulamento no tratamento e destinação conjunta de resíduos líquidos contendo cromo e vidrarias de laboratório. Quím. Nova 31 (3), 676–679. doi:10.1590/s0100-40422008000300035
Haque, M. A., Chowdhury, R. A., Chowdhury, W. A., Baralaskar, A. H., Bhowmik, S., and Islam, S. (2019). Immobilization Possibility of Tannery Wastewater Contaminants in the Tiles Fixing Mortars for Eco-Friendly Land Disposal. J. Environ. Manag. 242, 298–308. doi:10.1016/j.jenvman.2019.04.069
Iyaka, Y. A. (2009). Chromium in Soils: a Review of its Distribution and Impacts. J. Environ. Sci. 3, 13–18. doi:10.4314/afrrev.v3i3.47511
Kuganathan, N., Grimes, R. W., and Chroneos, A. (2019). Encapsulation of Heavy Metals by a Nanoporous Complex Oxide 12CaO · 7Al2O3. J. Appl. Phys. 125 (16), 165103. doi:10.1063/1.5090119
Kupwade-Patil, K., Allouche, E. N., Islam, M. R., and Gunasekaran, A. (2014). Encapsulation of Solid Waste Incinerator Ash in Geopolymer Concretes and its Applications. ACI Mat. J. 111 (6), 691–700. doi:10.14359/51686834
Lancellotti, I., Barbieri, L., and Leonelli, C. (2015). “Use of Alkali-Activated Concrete Binders for Toxic Waste Immobilization,” in Handbook of Alkali-Activated Cements, Mortars and Concretes. (Sawston, UK: Woodhead Publishing), 539–554. doi:10.1533/9781782422884.4.539
Leonelli, C., Barbieri, L., Lancellotti, I., Kamseu, E., and Ponzoni, C. (2014). A Process for Inertisation of Liquid Waste. Modena, Italy: University of Modena and Reggio Emilia. Italian Patent N. 0001410758.
Li, J., Xu, D., Wang, X., Liu, K., Mao, Y., Wang, M., et al. (2021). Encapsulation of Cesium with a Solid Waste-Derived Sulfoaluminate Matrix: A Circular Economy Approach of Treating Nuclear Wastes with Solid Wastes. J. Hazard. Mater. 416, 126156. doi:10.1016/j.jhazmat.2021.126156
Liang, J., Huang, X., Yan, J., Li, Y., Zhao, Z., Liu, Y., et al. (2021). A Review of the Formation of Cr(VI) via Cr(III) Oxidation in Soils and Groundwater. Sci. Total Environ. 774, 145762. doi:10.1016/j.scitotenv.2021.145762
Montañés, M. T., Sánchez-Tovar, R., and Roux, M. S. (2014). The Effectiveness of the Stabilization/solidification Process on the Leachability and Toxicity of the Tannery Sludge Chromium. J. Environ. Manag. 143, 71–79. doi:10.1016/j.jenvman.2014.04.026
Nur-E-Alam, M., Abu Sayid Mia, M., Ahmad, F., and Mafizur Rahman, M. (2018). Adsorption of Chromium (Cr) from Tannery Wastewater Using Low-Cost Spent Tea Leaves Adsorbent. Appl. Water Sci. 8, 129. doi:10.1007/s13201-018-0774-y
Nur-E-Alam, M., Mia, M. A. S., Ahmad, F., and Rahman, M. M. (2020). An Overview of Chromium Removal Techniques from Tannery Effluent. Appl. Water Sci. 10, 205. doi:10.1007/s13201-020-01286-0
Oh, S., Bade, R., Li, F., and Shin, W. S. (2014). Solidification/stabilization of Heavy Metals in Tannery Sludge Char with Various Binders. Desalin. Water Treat. 52 (4-6), 889–899. doi:10.1080/19443994.2013.827311
Oproiu, C.-L., Voicu, G., Bădănoiu, A., and Nicoară, A.-I. (2021). The Solidification/Stabilization of Wastewater (From a Landfill Leachate) in Specially Designed Binders Based on Coal Ash. Materials 14, 5610. doi:10.3390/ma14195610
Pantazopoulou, E., and Zouboulis, A. (2018). Chemical Toxicity and Ecotoxicity Evaluation of Tannery Sludge Stabilized with Ladle Furnace Slag. J. Environ. Manag. 216, 257–262. doi:10.1016/j.jenvman.2017.03.077
Parañaque, J. E. L., Maguyon-Detras, M. C., Migo, V. P., and Alfafara, C. G. (2020). Chromium Removal from Chrome-Tannery Effluent after Alkaline Precipitation by Adsorption Using Municipal Solid Waste-Derived Activated Biochar. IOP Conf. Ser. Mat. Sci. Eng. 778, 012134. doi:10.1088/1757-899X/778/1/012134
Pettine, M., and Capri, S. (2005). Digestion Treatments and Risks of Cr(III)–Cr(VI) Interconversions during Cr(VI) Determination in Soils and Sediments—A Review. Anal. Chim. Acta 540 (2), 231–238. doi:10.1016/j.aca.2005.03.040
Pimraksa, K., Chindaprasirt, P., Huanjit, T., Tang, C., and Sato, T. (2013). Cement Mortars Hybridized with Zeolite and Zeolite-like Materials Made of Lignite Bottom Ash for Heavy Metal Encapsulation. J. Clean. Prod. 41, 31–41. doi:10.1016/j.jclepro.2012.10.003
Pinakidou, F., Katsikini, M., Varitis, S., Komninou, P., Schuck, G., and Paloura, E. C. (2021). Probing the Structural Role of Cr in Stabilized Tannery Wastes with X-Ray Absorption Fine Structure Spectroscopy. J. Hazard. Mater. 402, 123734. doi:10.1016/j.jhazmat.2020.123734
Ponzoni, C., Lancellotti, I., Barbieri, L., Spinella, A., Saladino, M. L., Martino, D. C., et al. (20152015). Chromium Liquid Waste Inertization in an Inorganic Alkali Activated Matrix: Leaching and NMR Multinuclear Approach. J. Hazard. Mater. 286, 474–483. doi:10.1016/j.jhazmat.2014.12.054
Pradeep, S., Sundaramoorthy, S., Sathish, M., Jayakumar, G. C., Rathinam, A., Madhan, B., et al. (2021). Chromium-free and Waterless Vegetable-Aluminium Tanning System for Sustainable Leather Manufacture. Chem. Eng. J. Adv. 7, 100108. doi:10.1016/j.ceja.2021.100108
Randall, P., and Chattopadhyay, S. (2004). Advances in Encapsulation Technologies for the Management of Mercury-Contaminated Hazardous Wastes. J. Hazard. Mater. 114, 211–223. doi:10.1016/j.jhazmat.2004.08.010
Sgarlata, C., Formia, A., Ferrari, F., and Leonelli, C. (2021). “Effects of Temperature on Reactivity and Geopolymerization Process of Mining By-Product Untreated Clay,” in Book of Abstracts 3rd European Mineralogical Conference EMC 2020 (Cracow, Poland: Miner. Soc. of Poland-PTMin).
Sgarlata, C., Formia, A., Siligardi, C., Ferrari, F., and Leonelli, C. (2022). Mine Clay Washing Residues as a Source for Alkali-Activated Binders. Materials 15 (1), 83. doi:10.3390/ma15010083
Šillerová, H., Komárek, M., Liu, C., Poch, J., and Villaescusa, I. (2015). Biosorbent Encapsulation in Calcium Alginate: Effects of Process Variables on Cr(VI) Removal from Solutions. Int. J. Biol. Macromol. 80, 260–270. doi:10.1016/j.ijbiomac.2015.06.032
Silva, M. A. R., Mater, L., Souza-Sierra, M. M., Corrêa, A. X. R., Sperb, R., and Radetski, C. M. (2007). Small Hazardous Waste Generators in Developing Countries: Use of Stabilization/solidification Process as an Economic Tool for Metal Wastewater Treatment and Appropriate Sludge Disposal. J. Hazard. Mater. 147 (3), 986–990. doi:10.1016/j.jhazmat.2007.01.128
Stampino, P. G., Zampori, L., Dotelli, G., Meloni, P., Sora, I. N., and Pelosato, R. (2009). Use of Admixtures in Organic-Contaminated Cement-Clay Pastes. J. Hazard. Mater. 161, 862–870. doi:10.1016/j.jhazmat.2008.04.056
Study IP/G/STOA/FWC/2013-001/LOT 3/C3 (2017). Towards a Circular Economy - Waste Management in the EU. Available at: https://www.europarl.europa.eu/RegData/etudes/STUD/2017/581913/EPRS_STU%282017%29581913_EN.pdf.
Tang, P., Zhou, Y.-C., and Xie, Z.-M. (2013). Immobilization of Heavy Metals in Sludge Using Phosphoric Acid and Monobasic Calcium Phosphate. J. Zhejiang Univ. Sci. A 14 (3), 177–186. doi:10.1631/jzus.a1200263
Tantawy, M. A., El-Roudi, A. M., and Salem, A. A. (2012). Immobilization of Cr(VI) in Bagasse Ash Blended Cement Pastes. Constr. Build. Mater. 30, 218–223. doi:10.1016/S0008-8846(98)00190-210.1016/j.conbuildmat.2011.12.016
UN-The Sustainable Development Goals Report (2018). The Sustainable Development Goals Report. New York, NY, USA: United Nations. Available at: https://unstats.un.org/sdgs/files/report/2018/TheSustainableDevelopmentGoalsReport2018-EN.pdf.
Wang, Y., Ni, W., Zhang, S., Li, J., and Suraneni, P. (2020). Optimal Mixture Designs for Heavy Metal Encapsulation in Municipal Solid Waste Incineration Fly Ash. Appl. Sci. 10 (19), 1–21. doi:10.3390/app10196948
Wang, Z., Tan, K., Cai, J., Hou, S., Wang, Y., Jiang, P., et al. (2019). Silica Oxide Encapsulated Natural Zeolite for High Efficiency Removal of Low Concentration Heavy Metals in Water. Colloids Surfaces A Physicochem. Eng. Aspects 561, 388–394. doi:10.1016/j.colsurfa.2018.10.065
Waste Framework Directive (2008/98/EC) (2008). Directive 2008/98/EC of the European Parliament and of the Council of 19 November 2008 on Waste and Repealing Certain Directives. Available at: https://eur-lex.europa.eu/legal-content/EN/ALL/?uri=CELEX%3A32008L0098 (Accessed May 23, 2022).
Wei, Y.-L., Hsieh, H.-F., Yang, Y.-W., Lee, J.-F., and Liang, W.-S. (2005). Molecular Study of Thermal Immobilization of Chromium(VI) with Clay. J. Air & Waste Manag. Assoc. 55 (4), 411–414. doi:10.1080/10473289.2005.10464641
Zhang, M., Yang, C., Zhao, M., Yang, K., Shen, R., and Zheng, Y. (2017). Immobilization Potential of Cr(VI) in Sodium Hydroxide Activated Slag Pastes. J. Hazard. Mater. 321, 281–289. doi:10.1016/j.jhazmat.2016.09.019
Zhang, S., Zhang, Y., Wu, S., Yu, K., and Zhao, Z. (2022). The Preparation of Inorganic Materials to Consume and Stabilize Chromium-Containing Waste: A Review. JOM 74, 1360–1375. doi:10.1007/s11837-022-05153-y
Zhao, R., Guo, Y., Huang, X., Qian, J., Wu, Y., Li, Z., et al. (2022). Synergistic Immobilization of Chromium in Tannery Sludge by ZnO and TiO2 and the Oxidation Mechanism of Cr(III) under Alkaline in High Temperature. J. Hazard. Mater. 424, 127290. doi:10.1016/j.jhazmat.2021.127290
ATSDR (2012). Agency for Toxic Substances and Disease Registry. Available at: https://www.atsdr.cdc.gov/.
Keywords: Cr(III) ions, heavy metals, encapsulation, alkali activated aluminosilicate, leather industry waste, uncalcined clay, leaching, immobilization
Citation: Sgarlata C, Leonelli C, Lancellotti I, Mortalò C, Berrettoni M, Fattobene M, Zamponi S and Giorgetti M (2022) Sustainable Chromium Encapsulation: Alkali Activation Route. Front. Mater. 9:904580. doi: 10.3389/fmats.2022.904580
Received: 25 March 2022; Accepted: 14 June 2022;
Published: 07 July 2022.
Edited by:
Antonio Caggiano, Darmstadt University of Technology, GermanyReviewed by:
Ajeng Arum Sari, National Research and Innovation Agency, IndonesiaFrancesca Zanoni, Sphera Encapsulation srl, Italy
Copyright © 2022 Sgarlata, Leonelli, Lancellotti, Mortalò, Berrettoni, Fattobene, Zamponi and Giorgetti. This is an open-access article distributed under the terms of the Creative Commons Attribution License (CC BY). The use, distribution or reproduction in other forums is permitted, provided the original author(s) and the copyright owner(s) are credited and that the original publication in this journal is cited, in accordance with accepted academic practice. No use, distribution or reproduction is permitted which does not comply with these terms.
*Correspondence: Cristina Leonelli, Y3Jpc3RpbmEubGVvbmVsbGlAdW5pbW9yZS5pdA==; Mario Berrettoni, bWFyaW8uYmVycmV0dG9uaUB1bmljYW0uaXQ=