- 1School of Mechanical Engineering, Jiangsu University of Science and Technology, Zhenjiang, China
- 2Zhenjiang Stomatological Hospital, Zhenjiang, China
Dental health is closely related with people’s quality of life. Teeth are subject to different problems and risks over time. Therefore, studying the influence of age on mechanical properties of tooth enamel is of considerable importance. In this study, the collected human teeth were divided into four groups: deciduous (aged 6–12 years), juvenile permanent (aged 14–20 years), young adult permanent (aged 25–45 years), and older adult permanent (aged over 60 years) teeth. The mechanical properties of tooth enamel of all age groups were evaluated using a microhardness tester and laser confocal microscope. The results revealed that hardness of the deciduous teeth was much lower than that of permanent teeth. Hardness of young adult permanent teeth was the highest, followed by that of the older adult and juvenile permanent teeth. Deciduous teeth enamel had the highest fracture toughness, followed by that of young adult, juvenile, and older adult permanent teeth. The different chemical compositions and microstructures of enamel at different age groups resulted in the significant differences in mechanical properties. This research accords with the strategic requirement of improving peoples’ dental health, and its results are noteworthy in developing specific protocols for dental hygiene and clinical prevention.
Introduction
Teeth are among the most critical oral organs in the human body. In addition to cutting, biting, and chewing food to provide energy, they also ensure correct enunciation and good appearance. If the teeth do not develop or are not maintained properly, tooth loss due to decay occurs, which affects food intake and causes the lips and cheeks to lose support and sag, deforming the face. Thus, dental health is closely related to people’s quality of life.
The teeth are mainly constituted of dentine, with the outer layer comprising enamel (in the crown part) and cementum (in the root part). The enamel covers the outer layer of the crown and protects the inner dentine and pulp against damage from external loads. However, as an individual advances in age, the mechanical properties of enamel can change due to long-term exposure to the complex oral environment. Therefore, studying the influence of ageing on the mechanical properties of enamel for clinical treatment is of great importance.
Tooth enamel is the hardest mineralized substance in the human body. It has excellent mechanical properties and plays a key long-term role in the complex oral environment. Enamel is composed of 96–97% inorganic minerals and a small proportion of organic matter and water. The inorganic materials are mainly hydroxyapatite crystals but also contain a small amount of calcium fluoride and calcium phosphate (Robinson et al., 2000; Simmer and Hu, 2001; Beniash et al., 2009). Nanoscale hexagonal hydroxyapatite crystals are the basic constituent units of enamel. These long strips of hydroxyapatite particles are bonded with proteins to form the glaze column and interstitial glaze by employing numerous arrangements and orientations (He and Swain, 2008; Pan et al., 2008; Moradian-Oldak, 2012). The column is shaped like a keychain, and the hydroxyapatite particles that constitute the enamel pillar are arranged longitudinally, perpendicular to the enamel occlusal surface. The hydroxyapatite particles in the enamel interstitial region are deflected in orientation, which leads to a discontinuity of the enamel pillar and interstitium, thus forming a gap. This gap is called as enamel sheath and is rich in sheathlin known as ameloblastin and amelogenin (Zeichner-David et al., 1997). Moreover, the orientation of hydroxyapatite crystals on the occlusal surface and tooth enamel section differs as does the arrangement of inner and outer enamel, which results in a complex microscopic structure of tooth enamel and differences in the mechanical properties of tooth enamel at distinct positions (Cui and Ge, 2007; Shieh et al., 2011; Niu et al., 2017; Shen et al., 2020).
The mechanical properties and biological functions of enamel are not only attributed to its excellent structure but also to the effective combination of inorganic minerals and organic proteins. General materials will produce plastic damage close to atomic scale when impacted, thus showing very low fracture toughness (Li et al.,2022). According to the pull-shear chain model of proteins, under tensile stress, hard inorganic mineral crystals mainly bear tensile stress along the long axis, whereas soft organic protein layers mainly bear shear force (Ji and Gao, 2004). Tooth enamel wear entails the destruction of the interfacial protein bonds between hydroxyapatite crystals in nanofibers, which consumes considerable energy (Lei et al., 2020). Protein removal results in a reduction in fracture toughness of approximately 40% compared with a complete protein control. The loss of organic content reduces protein contribution to total fracture energy by more than 80%. This degradation manifests in the embrittlement of the unfractured bridging ligament and a subsequent reduction in fracture closure stress. Although the organic content of enamel is low, it is essential to enhance strength by promoting the formation of unbroken ligaments and enhancing enamel resilience (Yahyazadehfar and Dwayne, 2015). Concentrations of Mg and other minor ions on the surface of the microcrystalline layer (and adjacent media) also change systematically during the formation of human tooth enamel. This may affect the mineral phases and interactions of enamel matrix proteins and their degradation products with each other (Derocher et al., 2020). The water content in enamel can improve its fracture toughness and protect the surface from impact wear (Zheng et al., 2016). Compared with dehydrated enamel, hydrated enamel demonstrates superior wear resistance despite its lower hardness and higher coefficient of friction and sliding (Hua et al., 2019).
The mechanical properties of enamel, in addition to its microstructure and chemical composition, are also influenced by oral environmental factors such as pH and salivary lubrication (Galbany et al., 2014; Adhani, 2015; Antoine and Hillson, 2015).
After 1 min of adsorption in saliva, tooth enamel forms a dense protein membrane, which is the initial membrane of the tooth enamel surface and can effectively reduce tooth enamel wear (Zhang et al., 2013; Steiger-Ronay et al., 2020). The enamel wears more rapidly in citrate media than in artificial saliva. In conditions of citric acid erosion, the enamel surface forms a softened layer, and the enamel surface wear mechanism mainly manifests as adhesion peeling. The enamel surface is mainly ploughed and accompanied by peeling in the artificial saliva medium (Zheng et al., 2011). The hardness and elastic modulus of the enamel also decrease with pH (Barbour et al., 2010).
The oral environment as well as the microstructure and chemical components of the enamel change with age, which may lead to changes in their mechanical properties. However, most studies on the teeth of individuals from distinct age groups have focused on the correlation between age and dentine. Montoya et al., 2015 investigated the effects of age on dentine microstructure, hardness, and chemical composition, observing no significant difference in the tooth tube density or diameter, with the major difference exhibited in the occlusion rate of the dental tubule. Older dentine has a higher rate of dental tubule occlusion, thus leading to a higher mineral–collagen ratio. Thus, older dentine has higher hardness than younger dentine because of its higher mineral–collagen ratio. Mandra et al., 2020 discovered that the strength of the microshear bond between the dentine surface and composite resin adhesive decreased with age under different bonding systems, indicating a significant negative effect of ageing on the bonding strength of the dentine surface. Ryou et al. (2015) studied the effect of ageing on the mechanical properties of dentine, noting that the maximum bending stress and fracture energy of dentine decreased with age. The fracture toughness and fatigue strength of dentine in older adults was nearly 70% lower than in younger adults.
To date, few studies have investigated the age-related properties and the effects of age on enamel materials. Tooth and enamel rod sheath colours vary with age, and studies have revealed that the crystal gap and enamel rod sheath inhibit the reflection of lower dentin colour, with a tendency to scatter light from the surface to the deep layer. Young dental enamel rods are clear, but the number of crystal gaps and enamel rods decrease rapidly with age. The colour of the dentine is thus strongly reflected, resulting in a darker appearance (Miake et al., 2016). The thickness of enamel also begins to decrease from age 50 years onwards, and after 65 years, the amount of enamel is approximately one third less than that in younger people’s teeth (Carvalho and Lussi, 2017). These reports have not discussed the effect of age on the mechanical properties of enamel or fully analysed the changes in the mechanical properties of human enamel throughout its life cycle.
In the present study, the mechanical properties of human enamel throughout its life cycle were examined. In accordance with human enamel’s growth characteristics, its life cycle was divided into four parts: 1) the deciduous teeth group (aged 6–12 years), 2) juvenile permanent teeth group (aged 14–20 years), 3) young adult permanent teeth group (aged 25–45 years), and 4) older permanent teeth group (aged over 60 years). We investigated the mechanical properties of dental enamel at distinct ages. By using a microhardness tester and laser confocal scanning microscope and examining the Vickers hardness of the enamel surfaces at different ages, we studied the influence of age on enamel mechanical properties, providing strong support for further analysis of the connection between enamel microstructures and material performance.
Material and Methods
Sixteen molar teeth extracted from patients at Zhenjiang Stomatological Hospital were divided into deciduous teeth (aged 9–12 years), juvenile permanent teeth (aged 14–20 years), young adult permanent teeth (aged 25–45 years), and older adult permanent teeth (aged over 60 years) groups, with four teeth in each group. The collected teeth were soaked in deionised water and stored in a refrigerator at 4°C.
The dental inclusion criteria were as follows: 1) the teeth were third molars extracted due to orthodontics, periodontal disease, or trauma, or retained second deciduous molars and 2) crown was normal without caries. Written informed consent was obtained from all of the patients and their guardians.
For sample preparation, plaque and stones were first removed by sonication, and then the roots were partially removed (with the crown portion retained) using a handheld saw blade. Then, each sample was embedded in a soft plastic mould (25 mm × 18 mm) with resin, with the teeth occlusal surface faced downward. After solidification, the samples were polished successively with 400-, 800-, 1,500-, and 2,000-grit sandpapers in water cooling conditions and then polished successively with 5, 2.5, 1, and 0.5 μm water-soluble diamond polishing paste in water cooling conditions until the average surface roughness (Ra) was ≤0.1 μm. In order to reduce the influence of enamel thickness on the experimental results, the thickness of enamel polishing was controlled between 0.2 and 0.3 mm.
First, a microhardness tester (HVS-1000AT/EOS100B, China) was used to load each sample surface with different loads (0.1, 0.5, 1, 2, 5, and 10 N). The loading time for each load was 15 s and the ambient temperature was 25°C. Next, their Vickers hardness was measured based on the indentation morphology. At each load, 2 values were measured for each of the 16 samples, yielding a total of 192 values. Data relating to the same age group and load were averaged.
After the Vickers hardness measurement was completed, the indentation morphology of each sample was observed using a laser confocal scanning microscope (LCSM, XK-X1000, KEYENCE, Japan), and the indentation depth was measured. The process of measuring enamel indentation depth were depicted (see Figure 1).
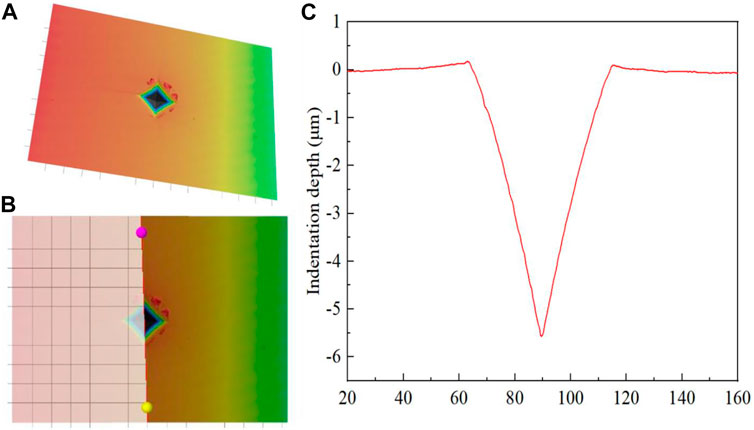
FIGURE 1. Indentation depth measurement process of dental enamel. (A) Indentation topography; (B) Indentation depth measurement; (C) Indentation depth.
The crack length of each indentation was then measured (see Figure 2). The fracture toughness of the enamel was calculated using Eq. 1 (Rivera et al., 2013):
Where E is the elastic modulus of the enamel (with E = 7.27 × 1,010 Pa), HV is the Vickers hardness of the enamel, c is the average length of four cracks, F is the normal load, and L is the average length of the indentation diagonal. The Chinese units are all the basic units of the international unit system.
Finally, the Ca and P elements in enamel of all ages were analyzed using X-ray energy spectrometer (Zeiss Crossbeam 540).
Results
Vickers Hardness
As illustrated by Figure 3, the relationship curve of the enamel surface Vickers hardness varied with normal loading among the age groups. The results suggest that at the same load, the young adult permanent teeth group exhibited the highest Vickers hardness of tooth enamel, followed successively by the older adult permanent, juvenile permanent, and deciduous teeth groups. Although the vickers hardness of enamel in different age groups decreased with the increase of loading. But under the same load, the size relationship between them is still quite obvious. The results of microhardness test revealed that when the normal load was 0.1 N, the enamel hardness values (HV’s) of the young adult permanent, older permanent, juvenile permanent, and deciduous teeth were approximately 567, 548, 527, and 469, respectively. With an increase in the normal load, the Vickers hardness of the enamel of all four groups decreased. When the normal load was increased to 10 N, the Vickers HV’s of the young adult permanent, older permanent, juvenile permanent, and deciduous tooth enamel decreased to approximately 330, 327, 323, and 313, respectively, (see Figure 3). In addition, as depicted in Figure 3, Nonetheless, the Vickers hardness of enamel of the permanent teeth of all ages did not differ markedly, whereas that of the deciduous tooth enamel was substantially lower than that of the permanent tooth enamel (of all ages). Therefore, from the perspective of Vickers hardness, the mechanical properties of the tooth enamel materials of different age groups differ considerably.
Depth of the Indentation
Figures 4, 5 present and compare the tooth enamel indentation depth for each age group at a load of 5 N. The depth of enamel indentation was the highest in the deciduous teeth group, followed by the juvenile, older adult, and young adult permanent teeth groups (in that order). From the Angle of indentation depth, there are significant differences in the compressive ability of enamel materials in different ages.
Fracture Toughness
As illustrated in Figure 6, the enamel surface indentation topography with a load of 5 N varies by age group, with the shortest crack propagation observed in the enamel of the young adult permanent teeth, followed successively by that of the juvenile permanent, deciduous, and older adult permanent teeth. Table 1 shows the mean crack propagation length of enamel indentation at different ages.
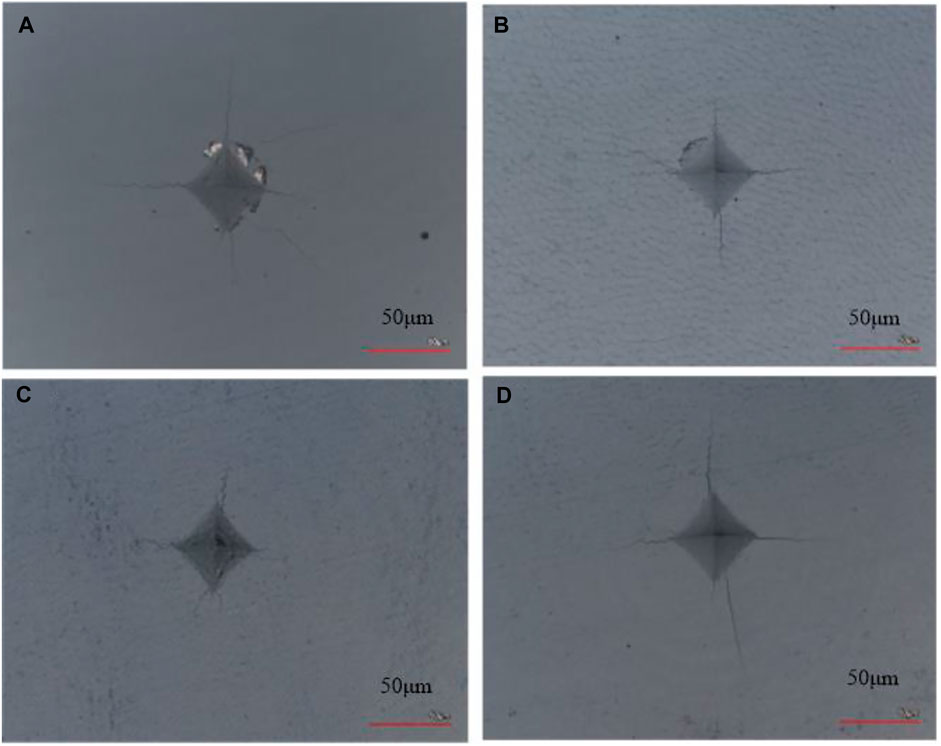
FIGURE 6. Topography of the enamel surface of different age groups at a load of 5 N. (A) Deciduous teeth group. (B) Juvenile permanent teeth group. (C) Young adult permanent teeth group. (D) Older adult permanent teeth group.
Figure 7 displays the relationship curves of enamel fracture toughness and normal load, which varied among the age groups. Within the test range (0.1–10 N), the fracture toughness of the enamel in all age groups presented a trend of increase with an increase in load. The fracture toughness values for the enamel in the older adult permanent, juvenile permanent, young adult permanent, and deciduous teeth were 0.457–0.597, 0.489–0.612, 0.507–0.643, and 0.526–0.682 MPa m0.5 (see Figure 7), respectively. Thus, the enamel surface fracture toughness values were the lowest in the older adult permanent teeth group, followed by the juvenile permanent, young adult permanent, and deciduous teeth groups.
Surface Topography
As illustrated in Figure 8, the surface topography of enamel changes with age. Compared with permanent teeth, the diameter of enamel rod of deciduous teeth is smaller and the arrangement is relatively scattered. The inter rod enamel of deciduous teeth is much wider. Water and protein are mainly stored in the inter rod enamel, so deciduous teeth have a lower hardness. The diameter of enamel rod of Juvenile permanent teeth is a litter smaller than young adult permanent teeth, they showed a more regular round shape. As the number of chewing increases, the enamel rod of older adult permanent teeth is flattened into an oval shape.
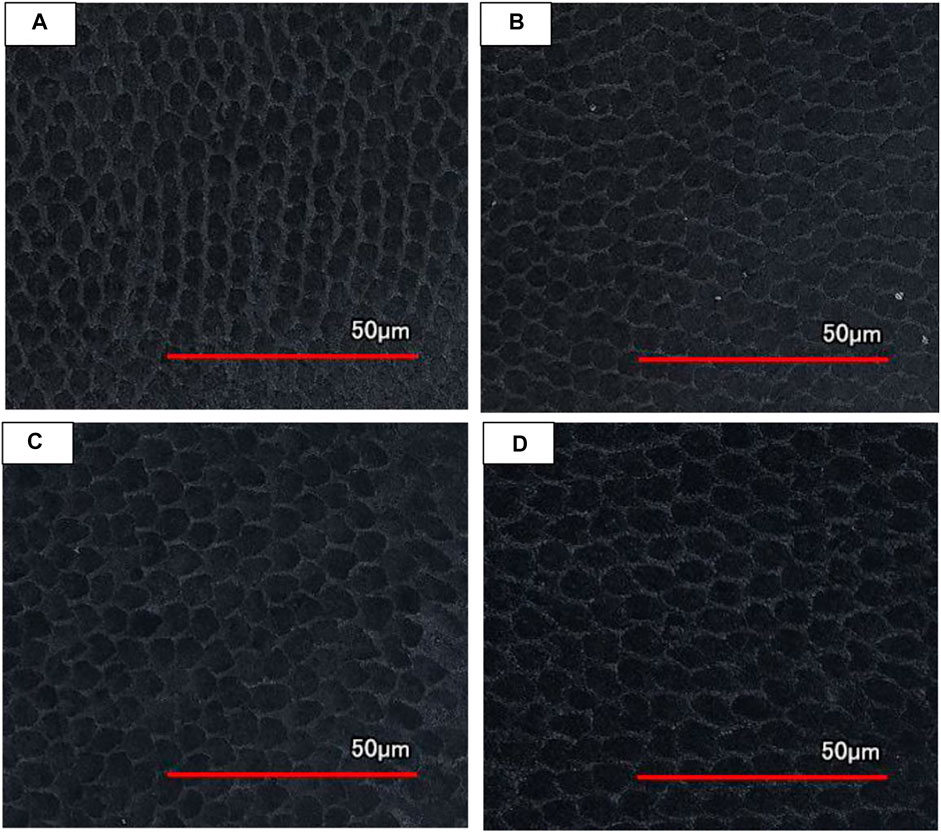
FIGURE 8. LCSM topography of enamel surface of different age groups after slight acid etching. (A) Deciduous teeth group. (B) Juvenile permanent teeth group. (C) Young adult permanent teeth group. (D) Older adult permanent teeth group.
EDS Results
Table 2 shows the Ca/P values of enamel surfaces at different ages. And what we can see is, we can see that the young adult group has the highest Ca/P, followed by the older adult permanent, juvenile permanent, and deciduous teeth groups. This is consistent with the law of Vickers hardness in different age groups.
Discussion
Studying the mechanical properties of tooth enamel is a crucial scientific venture. Due to the complex microstructure of tooth enamel and substantial limitations of quasistatic and dynamic testing methods, the use of the traditional indentation method is feasible (Xu et al., 2017). Microhardness testing indicated that at the same load, the Vickers hardness of the deciduous teeth was much lower than that of the permanent teeth. Although differences in Vickers hardness among the three age-based permanent teeth groups was not as obvious as that between the deciduous and permanent teeth, increasing age also altered the Vickers hardness in permanent teeth. This was possibly because of the lower enamel thickness, lower mineralisation, and higher organic content of deciduous teeth than of permanent teeth, which leads to the lower Vickers hardness in deciduous tooth enamel than in permanent tooth enamel. After an individual initially develops permanent teeth, the degree of mineralisation of the teeth increases as they grow. Zaichick and Zaichick, 2016 observed that the content of Ca, Cl, K, Mg and P in the teeth of people aged 25–35 years were the highest, and the content decreased with increasing age. In addition, the content of the minerals Ca and P in enamel determined changes in enamel hardness (Qiu et al., 2003; Zajcev et al., 2003). We noted that the changes in the Vickers hardness of enamel across ages coincide with the changes in Ca/P.
Fracture toughness is a measure of a material’s ability to resist crack propagation. For many ceramics, it depends on the phase composition as well as microstructure (e.g., crystal size and shape, distribution of glass and secondary crystal phases, closed porosity) (Medvedovski, 2001). The distinct chemical compositions and microstructures of tooth enamel at different ages also contribute to the differences in fracture toughness. Studies have revealed that when tooth enamel is subjected to an external load, the protein undergoes shear deformation (Li and Swain, 2007), and the enamel dissipates energy during chewing through this protein microdamage behaviour, thus increasing the toughness of the tooth enamel. Proteins are essential for the development of nanobridged ligaments, and their mechanisms include crack deflection, branching, and mineralised crack bridges. Removal of protein reduces the fracture toughness of the longitudinal and transverse directions of crack growth by approximately 40% (Yahyazadehfar and Dwayne, 2015). The protein content of the enamel in mature deciduous teeth was also noted to be nearly twice that in permanent teeth (Chen et al., 1988). The fracture toughness of deciduous tooth enamel is much higher than that of permanent tooth enamel, which may be related to the substantial difference in protein content between deciduous and permanent tooth enamel. At the same time, the protein content of permanent teeth of different ages is not different, but with the increase of age, the mineralization degree of enamel of permanent teeth is deepening, and the enamel column keeps growing, the enamel glaze column is arranged more closely. The microporous structure of the enamel is greatly reduced in young adults, it could promote the constant fang enamel fracture toughness increases in young adults. The enamel of older adult permanent teeth is exposed to the complex oral environment for a long time. Over time, a large amount of fluoride ions in the oral cavity erodes the hydroxyapatite crystals on the surface of the enamel, transforming those crystals and the microscopic structure of the tooth enamel (Brudevold et al., 1982; Leventouri et al., 2009). Furthermore, the thickness of older adult permanent tooth enamel is also one third less than that of young permanent tooth enamel because of the complex oral environment (Carvalho and Lussi, 2017). All of these factors potentially reduce the fracture toughness in older adults’ permanent tooth enamel.
The mechanical properties of deciduous and permanent teeth differ considerably, as do those of young and older adult permanent teeth and those of young adult and juvenile permanent teeth. However, Vickers hardness and fracture toughness can, notably, only indicate the mechanical properties of human tooth enamel in distinct ages from certain dimensions. The two are not directly related to one another and cannot completely represent the mechanical properties of human tooth enamel in different age groups.
Conclusion
In this paper, the surface mechanical properties of human tooth enamel of different age groups were characterised using a microhardness instrument and a laser confocal microscope to study the Vickers hardness, indentation depth, and fracture toughness. The results suggest the following conclusions.
(1) At the same load, the young adult permanent teeth group exhibited the highest Vickers hardness of enamel, followed successively by the older age permanent, juvenile permanent, and deciduous teeth groups. Nevertheless, the Vickers hardness of the enamel of the permanent teeth (of all ages) did not markedly differ, whereas the Vickers hardness of the deciduous tooth enamel was far less than that of the permanent tooth enamel.
(2) At the same load, the enamel indentation depth of the deciduous teeth was the highest, followed by that of the juvenile, older adult, and young adult permanent teeth.
(3) At the same load, the fracture toughness of the deciduous tooth enamel was the highest, followed by that of the young adult, juvenile, and older adult permanent tooth enamel.
(4) The different chemical compositions and microstructures of enamel at different age groups resulted in the significant differences in mechanical properties.
Data Availability Statement
The datasets presented in this study can be found in online repositories. The names of the repository/repositories and accession number(s) can be found below: https://figshare.com/s/d314f1285f989af449a9.
Ethics Statement
Ethical review and approval was not required for the study on human participants in accordance with the local legislation and institutional requirements. Written informed consent to participate in this study was provided by the participants’ legal guardian/next of kin.
Author Contributions
XJ, YZ, LK, HX and FY conceived the study and designed the experiments, XJ, YZ and LJ conducted the experiments, and XJ, YZ and FY analyzed the results and wrote the paper, ZJ collected the teeth samples.
Funding
This work was supported by the Natural Science Foundation of China (Grant number 51805226) and Science and Technology Innovation Fundation of Zhenjiang (Grant numbers SH2020070 and SH2019040).
Conflict of Interest
The authors declare that the research was conducted in the absence of any commercial or financial relationships that could be construed as a potential conflict of interest.
Publisher’s Note
All claims expressed in this article are solely those of the authors and do not necessarily represent those of their affiliated organizations, or those of the publisher, the editors and the reviewers. Any product that may be evaluated in this article, or claim that may be made by its manufacturer, is not guaranteed or endorsed by the publisher.
References
Antoine, D., and Hillson, S. (2015). Enamel Structure and Properties, 29. John Wiley & Sons, 223–243. doi:10.1002/9781118845486.ch16
Barbour, M. E., Parker, D. M., Allen, G. C., and Jandt, K. D. (2003). Human Enamel Dissolution in Citric Acid as a Function of pH in the Range 2.30≤pH≤6.30 - a Nanoindentation Study. Eur. J. Oral Sci. 111 (3), 258–262. doi:10.1034/j.1600-0722.2003.00039.x
Beniash, E., Metzler, R. A., Lam, R. S. K., and Gilbert, P. U. P. A. (2009). Transient Amorphous Calcium Phosphate in Forming Enamel. J. Struct. Biol. 166 (2), 133–143. doi:10.1016/j.jsb.2009.02.001
Brudevold, F., Aasenden, R., and Bakhos, Y. (1982). A Preliminary Study of Posteruptive Maturation of Teeth In Situ. Caries Res. 16, 243–248. doi:10.1159/000260604
Carvalho, T. S., and Lussi, A. (2017). Age-related Morphological, Histological and Functional Changes in Teeth. J. Oral Rehabil. 44 (4), 291–298. doi:10.1111/joor.12474
Chen, W. Y., Wu, A. J., Shao, J. J., and Li, L. Q. (1988). Analysis of Enamel Protein in Different Age Groups. Chin. J. Stomatology 05, 287–290.
Cui, F.-Z., and Ge, J. (2007). New Observations of the Hierarchical Structure of Human Enamel, from Nanoscale to Microscale. J. Tissue Eng. Regen. Med. 1 (3), 185–191. doi:10.1002/term.21
Derocher, K. A., Smeets, P. J. M., Goodge, B. H., Zachman, M. J., Balachandran, P. V., Stegbauer, L., et al. (2020). Chemical Gradients in Human Enamel crystallitesImpact Wear Behavior of Human Tooth Enamel under Simulated Chewing Conditions. NatureJournal Mech. Behav. Biomed. Mater. 583 (7814), 66119–71127. doi:10.1038/s41586-020-2433-3
Galbany, J., Romero, A., Mayo-Alesón, M., Itsoma, F., Gamarra, B., Pérez-Pérez, A., et al. (2014). Age-related Tooth Wear Differs between forest and savanna Primates. PLoS ONE 9 (4), e94938. doi:10.1371/journal.pone.0094938
He, L. H., and Swain, M. V. (2007). Contact Induced Deformation of Enamel. Appl. Phys. Lett. 90 (17), 171916. doi:10.1063/1.2450649
He, L. H., and Swain, M. V. (2008). Understanding the Mechanical Behaviour of Human Enamel from its Structural and Compositional Characteristics. J. Mech. Behav. Biomed. Mater. 1 (1), 18–29. doi:10.1016/j.jmbbm.2007.05.001
Hua, L. C., Wang, W. Y., Swain, M. V., Zhu, C. L., Huang, H. B., Du, J. K., et al. (2019). The Dehydration Effect on Mechanical Properties of Tooth Enamel. J. Mech. Behav. Biomed. Mater. 95, 210–214. doi:10.1016/j.jmbbm.2019.04.013
Jeng, Y.-R., Lin, T.-T., Hsu, H.-M., Chang, H.-J., and Shieh, D.-B. (2011). Human Enamel Rod Presents Anisotropic Nanotribological Properties. J. Mech. Behav. Biomed. Mater. 4, 515–522. doi:10.1016/j.jmbbm.2010.12.002
Ji, B., and Gao, H. (2004). Mechanical Properties of Nanostructure of Biological Materials. J. Mech. Phys. Sol. 52 (9), 1963–1990. doi:10.1016/j.jmps.2004.03.006
Lei, L., Zheng, L., Xiao, H., Zheng, J., and Zhou, Z. (2020). Wear Mechanism of Human Tooth Enamel: the Role of Interfacial Protein Bonding between HA Crystals. J. Mech. Behav. Biomed. Mater. 110, 103845. doi:10.1016/J.JMBBM.2020.103845
Leventouri, T., Antonakos, A., Kyriacou, A., Venturelli, R., Liarokapis, E., and Perdikatsis, V. (2009). Crystal Structure Studies of Human Dental Apatite as a Function of Age. Int. J. Biomater. 2009 (1), 1–6. doi:10.1155/2009/698547
Li, C., Piao, Y., Meng, B., Hu, Y., Li, L., and Zhang, F. (2022). Phase Transition and Plastic Deformation Mechanisms Induced by Self-Rotating Grinding of GaN Single Crystals. Int. J. Mach. Tools Manuf. 172, 103827. doi:10.1016/j.ijmachtools.2021.103827
Mandra, J. V., Votyakov, S. L., Smirnova, M. E., Zholudev, S. E., and Grigorjev, S. S. (2020). Comparative Evaluation of Adhesive Performance to Dentin: Study in Patients of Different Ages. BIO Web Conf. 22 (1), 01026. doi:10.1051/bioconf/20202201026
Medvedovski, E. (2001). Wear-resistant Engineering Ceramics. Wear 249 (9), 821–828. doi:10.1016/S0043-1648(01)00820-1
Miake, Y., Tsutsui, S., and Eshita, Y. (2016). Variation in the Color of Japanese Teeth and Structural Changes in Enamel Rod Sheath Associated with Age. J. Hard Tissue Biol. 25 (2), 131–136. doi:10.2485/jhtb.25.131
Montoya, C., Arango-Santander, S., Peláez-Vargas, A., Arola, D., and Ossa, E. A. (2015). Effect of Aging on the Microstructure, Hardness and Chemical Composition of Dentin. Arch. Oral Biol. 60 (12), 1811–1820. doi:10.1016/j.archoralbio.2015.10.002
Moradian-Oldak, J. (2012). Protein-mediated Enamel Mineralization. Front. Biosci. 17 (6), 1996–2023. doi:10.2741/4034
Niu, L., Zhang, H., Dong, S., Wang, Y., and Zou, R. (2017). The Microstructure Properties of Human Enamel on the Multi-Scale. J. Shanxi Med. Univ. 48, 1075–1078. doi:10.13753/j.issn.1007-6611.2017.10.022
Pan, H., Tao, J., Yu, X., Fu, L., Zhang, J., Zeng, X., et al. (2008). Anisotropic Demineralization and Oriented Assembly of Hydroxyapatite Crystals in Enamel: Smart Structures of Biominerals. J. Phys. Chem. B 112 (24), 7162–7165. doi:10.1021/jp802739f
Qiu, Y. N., Liu, W. M., Li, T. S., and Liu, L. Z. (2003). Comparative Study of Friction and Wear Behavior of Different Human Enamel In Vitro. Zhonghua Kou Qiang Yi Xue Za Zhi 38 (3), 213–216. doi:10.1007/BF02974893
R, A. (2015). Effect pH on Demineralization Dental Erosion. Ijcea 6 (2), 138–141. doi:10.7763/IJCEA.2015.V6.468
Rivera, C., Arola, D., and Ossa, A. (2013). Indentation Damage and Crack Repair in Human Enamel. J. Mech. Behav. Biomed. Mater. 21 (Complete), 178–184. doi:10.1016/j.jmbbm.2013.02.020
Robinson, C., Shore, R. C., Brookes, S. J., Strafford, S., Wood, S. R., and Kirkham, J. (2000). The Chemistry of Enamel Caries. Crit. Rev. Oral Biol. Med. 11 (4), 481–495. doi:10.1177/10454411000110040601
Ryou, H., Romberg, E., Pashley, D. H., Tay, F. R., and Arola, D. (2015). Importance of Age on the Dynamic Mechanical Behavior of Intertubular and Peritubular Dentin. J. Mech. Behav. Biomed. Mater. 42, 229–242. doi:10.1016/j.jmbbm.2014.11.021
Shen, L., Barbosa de Sousa, F., Tay, N., Lang, T. S., Kaixin, V. L., Han, J., et al. (2020). Deformation Behavior of normal Human Enamel: a Study by Nanoindentation. J. Mech. Behav. Biomed. Mater. 108, 103799. doi:10.1016/J.JMBBM.2020.103799
Simmer, J. P., and Hu, J. C.-C. (2001). Dental Enamel Formation and its Impact on Clinical Dentistry. J. Dental Education 65 (9), 896–905. doi:10.1002/j.0022-0337.2001.65.9.tb03438.x
Steiger-Ronay, V., Kuster, I. M., Wiedemeier, D. B., Attin, T., and Wegehaupt, F. J. (2020). Erosive Loss of Tooth Substance Is Dependent on Enamel Surface Structure and Presence of Pellicle - an In Vitro Study. Arch. Oral Biol. 112, 104686. doi:10.1016/j.archoralbio.2020.104686
Xu, Y. Z., Yang, Q. X., Wang, R. R., and Zhang, D. S. (2017). Effects of Aging and Location on Mechanical Properties of Human Dentin. Yiyong Shengwu Lixue/Journal Med. Biomech. 32 (6), 519–523. doi:10.16156/j.1004-7220.2017.06.006
Yahyazadehfar, M., and Arola, D. (2015). The Role of Organic Proteins on the Crack Growth Resistance of Human Enamel. Acta Biomater. 19, 33–45. doi:10.1016/j.actbio.2015.03.011
Zaichick, V., and Zaichick, S. (2016). The Effect of Age on Ca, Cl, K, Mg, Mn, Na, P and Sr Contents in Roots of Human Female Permanent Teeth. J. Radioanal. Nucl. Chem. 309, 295–301. doi:10.1007/s10967-016-4803-8
Zajcev, V. M., Lifljandskij, V. G., and Mirinkin, V. I. (2003). Applied Medical Statistics (Tutorial). St. Petersburg: Foliantpress.
Zeichner-David, M., Vo, H., Tan, H., Diekwisch, T., Berman, B., Thiemann, F., et al. (1997). Timing of the Expression of Enamel Gene Products during Mouse Tooth Development. Int. J. Dev. Biol. 41 (1), 27–38. doi:10.1007/s11626-997-0027-0
Zhang, Y. F., Zheng, J., Zheng, L., Shi, X. Y., Qian, L. M., and Zhou, Z. R. (2013). Effect of Adsorption Time on the Lubricating Properties of the Salivary Pellicle on Human Tooth Enamel. Wear 301 (1-2), 300–307. doi:10.1016/j.wear.2012.11.037
Zheng, J., Huang, H., Shi, M. Y., Zheng, L., Qian, L. M., and Zhou, Z. R. (2011). In Vitro study on the Wear Behaviour of Human Tooth Enamel in Citric Acid Solution. Wear 271 (9-10), 2313–2321. doi:10.1016/j.wear.2010.11.027
Keywords: age, dental enamel, fracture toughness, mechanical propertie, biomaterials
Citation: Jing X, Zhipeng Y, Kaiyong L, Junjie L, Xinyuan H, Juan Z and Yujie F (2022) Effect of Age on Mechanical Properties of Human Tooth Enamel. Front. Mater. 9:888638. doi: 10.3389/fmats.2022.888638
Received: 03 March 2022; Accepted: 22 March 2022;
Published: 13 April 2022.
Edited by:
Guijian Xiao, Chongqing University, ChinaReviewed by:
Licheng Hua, Ningbo University, ChinaYafeng Zhang, Southwest University of Science and Technology, China
Peng Zhang, Sichuan University, China
Copyright © 2022 Jing, Zhipeng, Kaiyong, Junjie, Xinyuan, Juan and Yujie. This is an open-access article distributed under the terms of the Creative Commons Attribution License (CC BY). The use, distribution or reproduction in other forums is permitted, provided the original author(s) and the copyright owner(s) are credited and that the original publication in this journal is cited, in accordance with accepted academic practice. No use, distribution or reproduction is permitted which does not comply with these terms.
*Correspondence: Xia Jing, eGlhamluZ2R1bWlAeWVhaC5uZXQ=; Zhang Juan, MTU5MDUyODU5ODJAMTM5LmNvbQ==; Fan Yujie, ZmFueXVqaWVAanVzdC5lZHUuY24=