- 1Department of Chemistry, School of Science, University of Management and Technology, Lahore, Pakistan
- 2Department of Chemistry, School of Natural Sciences (SNS), National University of Science and Technology (NUST), Islamabad, Pakistan
- 3Department of Pharmaceutics and Pharmaceutical Technology, Taif University, Taif, Saudi Arabia
- 4Chemistry Department, Faculty of Science, King Khalid University, Abha, Saudi Arabia
- 5Biology Department, Faculty of Science, King Khalid University, Abha, Saudi Arabia
- 6Department of Semi Pilot Plant, Nuclear Materials Authority, El Maadi, Egypt
- 7Department of Pharmaceutics and Industrial Pharmacy, College of Pharmacy, Taif University, Taif, Saudi Arabia
- 8Biology Department, College of Science, Princess Nourah Bint Abdulrahman University, Riyadh, Saudi Arabia
- 9Department of Physics, College of Science, Princess Nourah Bint Abdulrahman University, Riyadh, Saudi Arabia
- 10Department of Pharmaceutical Sciences, College of Pharmacy, AImaarefa University, Riyadh, Saudi Arabia
- 11Ibn-E-Sina Institute of Technology, Islamabad, Pakistan
- 12Department of Chemistry, GC Women University, Sialkot, Pakistan
In this study, a green synthesis strategy was used to construct CuO-GO-Ag nanocomposites (NCs) by using biological components of Fagonia arabica. Copper Oxide (CuO) nanoparticles and silver (Ag) nanoparticles were fabricated by using a plant extract of Fagonia arabica. Graphene oxide was synthesized by hummer’s method and hydrothermal approach. The characterization techniques used in this work were Energy Dispersive X-ray (EDX), Scanning electron microscopy (SEM), X-ray Diffraction (XRD), and ultra-violet visible spectroscopy (UV-Vis). As an innovative approach graphene oxide (GO) was firstly assorted with green synthesized CuO NPs and then Ag nanoparticles were incorporated to form amalgamated CuO-GO-Ag nanocomposite (NCs). The anti-inflammatory and antioxidant activity of nanocomposite were examined. The anti-inflammatory and antioxidant ability of the nanocomposite were evaluated by UV-Visible spectroscopy. Results reveal that biologically synthesized CuO-GO-Ag NCs have high scavenging and inflammation reduction potential as compared to standards (ascorbic acid, diclofenac sodium). CuO-GO-Ag showed that scavenging efficiency for 0.3 mg/ml is 57.40% and for 0.5 mg/ml is 74.24% while scavenging efficiency for standard (ascorbic acid) is 44.22% for 0.3 mg/ml and 58.42% for 0.5 mg/ml. Likewise, CuO-GO-Ag nanocomposite showed a % inhibition value of 46.59 and 87.80 at 0.1 mg/ml and 0.5 mg/ml while diclofenac sodium showed a % inhibition value of 10.98 and 35.37 a similar concentration. Fagonia arabica seems to be a good source for acclimating the potential of NCs and nanoparticles toward different increased biological activities, according to the findings.
1 Introduction
Inflammation is a complex series of biological responses that results in protective and reparative responses to tissues (Schmid-Schönbein, 2006). Inflammation is of two types may be acute or chronic. In the acute phase, an injury or illness lasts for a short duration that’s why it is also termed as short-term inflammation (Murakami and Hirano, 2012). Some of the warning signs of acute inflammation are swelling, pain, redness, loss of function, and warmth in the affected area (Doyle et al., 2020). If acute inflammation is left untreated, the acute responses progressed to chronic disorders like cancer, arthritis, heart diseases, osteoporosis, and atherosclerosis (Nielsen and Ainsworth, 2013; McInnes and Schett, 2017; Schett and Neurath, 2018). Anti-inflammatory drugs are classified into two types steroidal and non-steroidal anti-inflammatory drugs (Juthani et al., 2017). Steroidal anti-inflammatory drugs are fast in action and were applied firstly to reduce inflammation (Rhen and Cidlowski, 2005; Ayroldi et al., 2012; Yatoo et al., 2018). Creating non-steroidal anti-inflammatory medications aimed to eliminate steroidal anti-inflammatory drugs’ adverse effects (SAIDs) (Celotti and Laufer, 2001; Rainsford, 2007). Some of the reported anti-inflammatory drugs are aspirin, ibuprofen, naproxen, diclofenac, diflunisal, etodolac, indomethacin, flubiprofen, and oxaprozin. Long-term use of NSAIDs increases the risk of stomach disorders, heart problems, and ulcers. The purpose of this research work is to check the efficiency of biologically synthesized CuO-GO-Ag nanocomposite to reduce inflammation. The phytoconstituent present in the plant (Fagonia arabica) used in this work can reduce the undesirable inflammatory response and exhibit anti-inflammatory activity (Ali and Khan, 2021).
Oxygen is a reactive element and plays a vital role in oxidative processes occurring in the living organism. Some naturally occurring free radicals include superoxide. NO•, OH•, and Reactive Oxygen Species (ROS) are involved in many physiological pathways (Kontoghiorghes and Kontoghiorghe, 2019). Some of the enzymes like glutathione peroxidase and superoxide dismutase are involved in the regulation of free radicals and ROS exhibiting activities essential for life. Besides these enzymes, some natural antioxidants like glutathione and some vitamins like A, C, and vitamin E also regulate antioxidant mechanisms (Denisov and Afanas’ ev, 2005; Ďuračková, 2010; Forman, 2016). Oxidation is a chain reaction for the production of free radicals. Toxic free radicals produced in a continuous chain reaction may lead to cell, toxicity, and other diseases like rheumatism, arthritis, and cancer (Matés et al., 2000; Calabrese et al., 2008; Singh et al., 2015). To reduce the risk of these diseases various antioxidant drugs are already reported such as ascorbic acid, stannic acid, lycopene, masoprocol, lipoic acid, and tocopherol (Meena et al., 2012; Mei et al., 2015; Müller et al., 2016). Researchers are now finding the biologically synthesized medicine to cure the diseases to avoid side effects and limitations reported by the use of antioxidant drugs.
Fagonia arabica is a medicinal plant that is often used to treat conditions such as liver cancer, high blood pressure, leprosy, inflammation, smallpox, and skin problems (Satpute et al., 2012). The twigs of the plants can be used as a paste on tumors, act as a natural remedy for snakebite, and the treatment of swallowed neck (Rizvi et al., 1996; SAEED and SABIR, 1999; Edeoga et al., 2005). Fagonia arabica has several biologically active phytoconstituents including flavonoids, alkaloids, saponins, tannins, coumarins, and phenolic compounds (Satpute et al., 2009) which exhibit antioxidant, anti-inflammatory, anticancer, antidiabetic, and analgesic activities (Alqasoumi et al., 2011; Chourasia et al., 2014; Al-Ziaydi, 2015; Iftikhar et al., 2021).
Nowadays metal oxide nanoparticles and their composites are of considerable importance because of their unique characteristics like surface-to-volume ratio, uniform morphology, light-absorbing potential, and small size. Their applications are increasing day by day in the fields of biomedicine, sensors, and bio-imaging. Metal and metal oxide NPs possess anti-inflammatory, antibacterial, anticancer, antioxidant, antimitotic, and anti-diabetic activities (Ijaz et al., 2017; Khan et al., 2018a; Iqbal et al., 2019). The biological synthesis of NPs and NCs has gained more importance in the recent era to overcome the hazardous effect and limitations of the chemical and physical approach. The main advantages of green synthesis are that it is cost-effective, nontoxic, robust, and easy to manage (Hussain et al., 2016; Parveen et al., 2016; Gour and Jain, 2019). Both physical and chemical approaches take a lot of energy to reduce and cap because they employ dangerous chemicals. The downside of these methods is that the harmful chemicals employed to cap and reduce nanoparticles remain on their surfaces even after repeated washings. Hence, due to toxicity risk, they cannot be used in biomedicine (Khan et al., 2018b; Haq et al., 2020). In contrast to these methods, the plant-based approach is environmentally friendly, economically feasible, and easily scalable. In the plant-based method plant’s biological molecules served as a capping agent and reducing. That’s why we have synthesized CuO-GO-Ag nanocomposite by using green synthesis and further evaluating their anti-inflammatory and antioxidant potential.
In this work, we have synthesized CuO-GO-Ag NCs by using biological molecules of Fagonia arabica. CuO-GO-Ag NCs were produced utilizing Fagonia arabica as a reducing and stabilizing agent in the current study. The antioxidant and anti-inflammatory properties of the biologically produced NCs were explored further.
2 Material and Methods
2.1 Chemicals
All the chemicals (CuSO4.5H2O, NaOH, AgNO3, H2SO4, K2S2O8, KMnO4, HCl) are of analytical grade and were purchased from Sigma-Aldrich Darmstadt, Germany.
2.2 Collection of Plant Material
Fagonia arabica leaves were obtained from a location near Nankana Sahib, Pakistan. To eliminate any impurities, it was then rinsed with distilled water. The washed leaves were then kept in shade for two to 3 weeks to dry them completely. When the leaves were completely dried, they were ground in a powder form to be used for extraction.
2.3 Preparation of Plant Extract
In a 500 ml beaker filled with distilled water, 50 g of Fagonia arabica powder was used to make a plant extract. After that, it was stirred for 30 min on a hot plate. The solution was then removed from the hot plate and permitted to cool down to 26°C. The extract was then filtered using Whatman No. 1 filter paper. CuO NPs, Ag NPs, CuO-GO, and CuO-GO-Ag nanocomposite were all synthesized using the obtained extract.
2.4 Green Synthesis of CuO Nanoparticles
CuSO4.5H2O solution (24.96 g of CuSO4.5H2O dissolved in 100 ml water) was prepared in a 100 ml conical flask. In a 500 ml beaker, 160 ml plant extract, 40 ml distilled water, and 100 ml copper sulfate pentahydrate solution were combined to make copper oxide nanoparticles. By placing the beaker on a magnetic stirrer for 15 min, the homogeneous mixture was achieved. The homogenized mixture was then allowed to heat in a hot air oven to 112°C and held there for 30 min. It was then taken out of the oven and left to cool for 3 h at room temperature to enable the precipitates to settle before being filtered. To eliminate the moisture content, the precipitates were dried in an oven at 110°C. A spatula was used to remove the precipitates from the filter paper. CuO NPs were then produced and kept in a vial until they were needed again.
2.5 Green Synthesis of Silver Nanoparticles
Aqueous silver nitrate solution of 0.25 molarity was prepared and covered with aluminum foil and placed in a darker area as the reaction is light sensitive. 100 ml leaf extract was added into 200 ml silver nitrate solution in a 500 ml beaker and then covered with aluminum foil to avoid light interaction. Notice the color change. The color of the solution changes from light green to reddish which indicates the formation of silver nanoparticles. The AgNPs were centrifuged at 4000 rpm for about 30 min. The Ag nanoparticles landed at the bottom of the centrifuged tube, where they were rinsed with distilled water to eliminate any unattached phytoconstituents before being filtered. The moisture content was then fully removed by heating the precipitates in a hot air oven. The Ag nanoparticles were produced and stored in a vial at 4°C until they were needed.
2.6 Synthesis of Graphene Oxide
2.6.1 Hydrothermal Synthesis of Graphene Oxide
Hydrothermal synthesis of graphene oxide involved the use of Teflon lined autoclave and graphene oxide was synthesized by the Tang Lau method. 0.5 M sugar solution was prepared by adding 42.75 g of sucrose in 100 ml distilled water. An autoclave with a capacity of 100 ml was used, and roughly half of its capacity was filled with sugar solution. After that, the autoclave was sealed and held at 180°C for about 80 min. After that, the oven was switched off and the autoclave was allowed to cool for about 4–5 h at room temperature. When the autoclave was opened after cooling, a brown-colored fluid containing graphene hanging at the bottom was produced. After that, the solution was filtered using Whatman filter paper. After obtaining the product, it was rinsed with distilled water. The remnants were washed thoroughly and then dried in the oven to eliminate any remaining moisture. The dry product was scooped out with a spatula and placed in a vial to be used and characterized later.
2.6.2 Synthesis of GO Using Modified Hummer’s Method
Graphene oxide was also synthesized by using the modified hummers method with some modifications. The second method performed to obtain graphene oxide is the modified hummers method to obtain graphene oxide. This method involves two steps. In the first step, 10 ml concentrated H2SO4 was taken in a 100 ml beaker followed by the addition of 3 g of potassium per Sulphate (K2S2O8). Then add carefully about 1.6 ml of phosphoric acid. Initially, a bright yellow color is observed. The solution was then heated to 80°C and allowed to be stirred for 3 h. In this solution carefully add 5 g of graphite powder during stirring. A dark blue color mixture is then cooled at room temperature. This dark blue color solution mixture is then diluted with distilled water, filtered, and washed several times. Washing must be continued until the waste solution has a neutral pH. The collected powder is pre-oxidized graphite powder which is then dried overnight in the air at room temperature to make it moisture free. In the second step, graphene oxide is synthesized from pre-oxidized graphene powder obtained in step 1.
In the second step firstly set up an ice bath. Add 23 ml of concentrated H2SO4 by maintaining the temperature to 0°C. 1 g of pre-oxidized graphite powder is then added to the beaker already having concentrated H2SO4 and was stirred vigorously to obtain homogenous dispersion. 3.0 g of KMnO4 was then added very carefully under slow stirring and the temperature of the resultant solution would not exceed 20°C. After that resulting solution was stirred for about 20 h at 35°C temperature. After 2 h dilute the solution by adding 46 ml of distilling water and again stirred for 15 min. For the termination of reaction 140 ml of distilling water and 2.5 ml of H2O2 (concentration 30%) was added to the reaction mixture. The appearance of bright yellow color indicates the termination of reaction as well as the formation of graphene oxide.
To remove metal ions from the resulting residue, the mixture was filtered and washed multiple times with 250 ml of dilute aqueous HCl solution with a ratio of 1:10 v/v. The graphene oxide was then dispersed in 300 ml water and centrifuged for 40 min at 8,000 rpm. After filtering, the supernatant was carefully collected. This graphene oxide dispersion was then placed in a filter membrane and ultra-filtered for 6 days to eliminate the remaining salts and acids. After that, the filtered graphene oxide was placed onto a petri dish and baked at 70°C to eliminate moisture. After removing the moisture, the graphene oxide was scooped and stored for later use (Figure 1).
2.7 Green Synthesis of CuO-GO Nanocomposites
1 M solution of copper sulfate pentahydrate (CuSO4.5H2O) was prepared in a 100 ml volumetric flask. Then, in a 500-milliliter beaker, 160 ml of plant extract, 40 ml of distilled water, 50 mg of graphene oxide, and CuSO4.5H2O solution were added, stirred, and baked for 30 min at 112 degrees Celsius. It was then taken out of the oven and set aside to cool to room temperature. After filtering the solution, the precipitates were dried in a 110°C oven to eliminate moisture. With the help of a spatula, the precipitates were removed from the filter paper and placed in a vial.
2.8 Green Synthesis of CuO-GO-Ag Nanocomposites
1 M solution of copper sulfate pentahydrate (CuSO4.5H2O) was prepared in a 100 ml volumetric flask. 500 ml beaker was taken and 160 ml of plant extract, 40 ml distilled water, 50 mg of graphene oxide, 100 mg of Ag NPs, and 100 ml CuSO4.5H2O solution were added, mixed, and sonicated for 1 h. The solution was then heated for 30 min and then allowed to cool for 3 h at room temperature, allowing the precipitates to settle to the bottom of the beaker (Figure 2). After filtering the solution, the precipitates were dried in a 110°C oven to eliminate moisture. With the use of a spatula, these precipitates were removed from the filter paper and placed in a vial for further use and analysis.
2.9 Characterization
Different spectroscopic techniques were used to characterize biologically synthesized CuO-GO-Ag nanocomposite as well as graphene oxide formed by hydrothermal approach and hummer’s method. The crystallinity of synthesized composite was examined by X-Ray diffraction (XRD) analysis. SEM images provide information about morphology. The elements in the sample are confirmed by energy-dispersive X-ray spectroscopy (EDX) analysis. The anti-inflammatory and antioxidant properties of CuO-GO-Ag nanocomposite were investigated using UV-Visible spectroscopy.
2.10 Anti-Inflammatory Activity
Nanotechnology has become known in a past few decades for its huge applications in almost every field. Nanoparticles exhibit efficient properties then bulk materials. In medical and therapeutic fields nanotechnology plays a vital role for the diagnosis and treatment of inflammatory disorders. Agerwal et al., reported the formation of zinc oxide nanoparticles and then evaluate their anti-inflammatory response (Agarwal et al., 2019; Agarwal and Shanmugam, 2020). The efficiency of the biologically produced nanocomposite was tested using anti-inflammatory activities. The findings were then compared to diclofenac sodium, which is a common pain reliever. Our body has a normal defense mechanism but sometimes it results in various disorders. The inflammatory disorders can be treated by using anti-inflammatory drugs. In the present work standard anti-inflammatory drug (diclofenac sodium) and biologically synthesized (CuO-GO-Ag NCs) were checked for the reduction of inflammation. For that purpose, two dilutions of various concentrations (0.1 and 0.5 mg/ml) for the sample (CuO-GO-Ag) and the Standard (Diclofenac Sodium) were made. The aforesaid dilution of sample and standard was supplemented with 0.3 ml egg albumin and 2.9 ml phosphate buffer saline with a pH of 6.4. The mixture was heated for 6 min at 70 degrees Celsius after being incubated at 37 degrees Celsius. With DMSO (Dimethyl sulfoxide) as a blank solution, absorbance was measured at 660 nm after cooling. % Inhibition was calculated by the formula given below (Das et al., 2019; Shahid et al., 2019).
Here V represent absorbance.
2.10.1 Preparation of Standard
Diclofenac sodium solutions of 100 and 500 ppm were produced. Then 0.3 ml egg albumin and 2.9 ml of pH 6.4 phosphate-buffered saline were added. After 20 min of incubation at 370°C, the mixture is ready to use. It was cooked for 6 min at 700°C. After cooling, the absorbance was determined at 660 nm using Dimethyl sulfoxide (DMSO) as a blank. The formula was used to compute the % inhibition.
2.10.2 Preparation of Phosphate Buffer Saline
The components of phosphate buffer saline are Potassium chloride (KCl), sodium chloride (NaCl), monopotassium phosphate (KH2PO4), and disodium phosphate (Na2HPO4). To 250 ml of distilled water add 2 g NaCl, 50 mg KCl, 0.36 g Na2HPO4, and 61.25 mg KH2PO4. The pH of the solution was adjusted at 6.4. The distilled water was then added to make a volume of 1 liter.
2.11 Antioxidant Activity
Antioxidant activity was performed by using the DPPH method and the Phosphomolybdenum method.
2.11.1 DPPH Method
The elimination of damaging free radicals that cause injury to the body is known as free radical scavenging. A conventional ascorbic acid DPPH experiment was used to determine the antioxidant capability of our biologically produced CuO-GO-Ag nanocomposite (Figure 3). Both the sample (CuO-GO-Ag nanocomposite) and standard were synthesized in four dilutions of various concentrations (0.05, 0.125, 0.25, and 0.5 mg/ml) (ascorbic acid). To the aforesaid dilutions, 4 ml of 0.1 mM methanolic DPPH solution was added and the mixture was shaken. After that, the solution was let to cool for approximately 25 min at room temperature. 517 nm was used to determine absorbance. As a blank solution, methanol was utilized. The method was done three times to get the mean value. % scavenging of both the sample and standard was calculated by using the formula given below
A control is the absorbance of control which in this method is Methanolic DPPH.
IC50 is half-maximal inhibitory concentration was calculated for both the sample and standard.
2.11.2 Phosphomolybdenum Method
The Phosphomolybdenum technique was used to determine the free radical scavenging potential. Both the sample and standard were produced with two dilutions of around 0.3 and 0.5 mg/ml concentrations. 5.3 g sodium phosphate, 16.7 ml sulfuric acid, and 2.5 g ammonium Molybdate were used to make Phosphomolybdenum reagent. To the sample and standard dilutions, 4 ml of reagent solution was added. These solutions, together with the reagent solution in a separate vial, were then stored in a water bath for cooling. For roughly 1 h and 30 min, the vials were held at 95°C. Using a reagent as a blank solution, the absorbance was measured at 695 nm. Both the sample (CuO-GO-Ag nanocomposite) and standard inhibitory concentrations were computed (ascorbic acid). Percentage scavenging was estimated by the formula given below
3 Result and Discussion
3.1 Scanning Electron Microscopy Analysis
3.1.1 SEM Analysis of CuO-GO-Ag Nanocomposite
To obtain the morphology of the material, a scanning electron microscope scans a focused stream of electrons. The sample’s interaction with the electrons in the beam produces a variety of signals that offer information about the surface topography. SEM has been used to determine surface morphology and composition. The system’s voltage was varied between 10 and 30 kV. The sample’s average size was 50–100 nm, which corresponds to the nanoparticle size range. Figure 4 shows SEM images of CuO NPs, CuO-GO, and CuO-GO-Ag. In comparison to CuO nanoparticles, the NCs produced exhibited reduced aggregation. The aggregation of CuO nanoparticles in powdered form is reduced by silver and graphene oxide doped NCs.
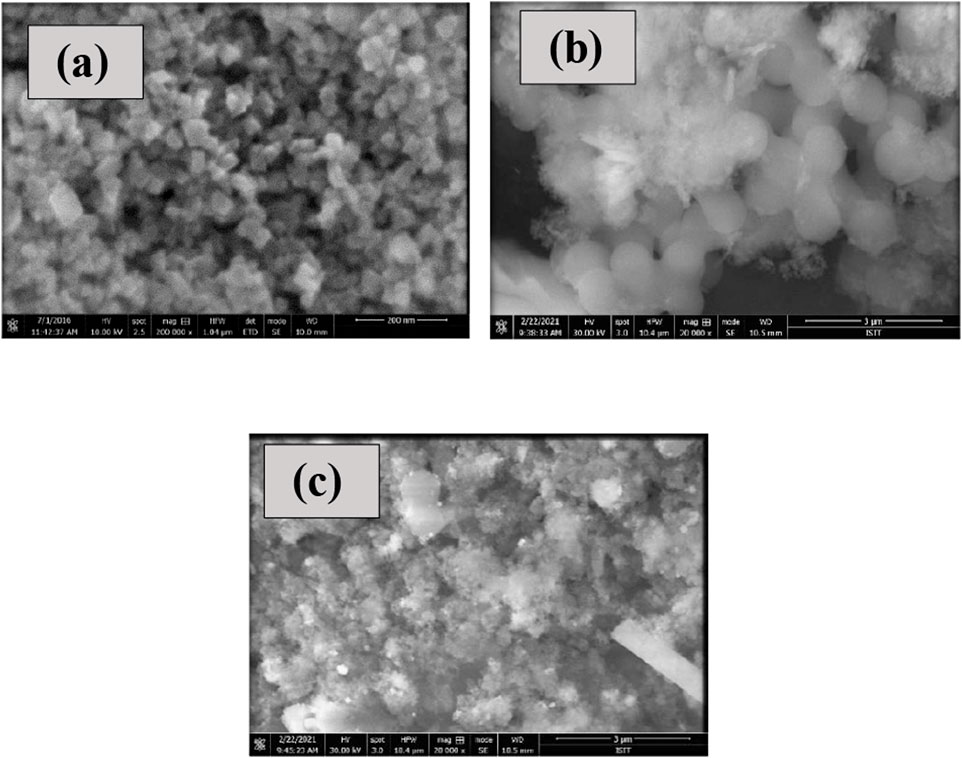
FIGURE 4. (A) SEM image of CuO NPs (B) SEM Images of CuO-GO (C) SEM Image of CuO-GO-Ag nanocomposite.
3.1.2 SEM Analysis of Graphene Oxide
SEM was used to examine the particle size and shape of graphene oxide produced using two techniques. Figure 5A displays SEM images of graphene oxide created using Hummer’s technique, while 5(b) shows GO synthesized using the hydrothermal method. The GO synthesized from the hydrothermal method showed particle nature and spherical morphology while GO synthesized from Hummer’s method showed flaky texture with interspaces of the layer. The size range of synthesized GO is in the nano-range.
3.2 X-Ray Diffraction Analysis
3.2.1 XRD Analysis of CuO, CuO-Ag, CuO-GO-Ag
The material’s structure was determined using X-ray diffractometry, which is an analytical approach. It was used to provide one-cell dimension information. Purity and particle size were determined using XRD. JCPDS card numbers 48–1,548 revealed the existence of CuO nanoparticles. Bragg’s equation is obeyed by the peaks in the picture. The intensity of some of the peaks is higher, while others are lower. The orderly arrangement of crystals and electron density determine the peak intensity. The peak intensity of a sample increases with the amount of periodicity in the crystal structure. The diffraction peaks were found to be smaller at higher temperatures. Between 2 and intensity is shown in the graph. In the XRD graph, 2 denotes the angle between the transmitted and reflected beams, and d represents the distance between them. In the case of CuO-GO, graphene oxide is incorporated in CuO to form CuO-GO NCs. Different diffraction peaks are demonstrated by XRD spectra. In the fabrication of CuO-GO-Ag nanocomposite Ag and GO are incorporated in CuO to form the desired product. Figure 6 shows the XRD spectra of CuO, CuO-Ag, CuO-GO-Ag.
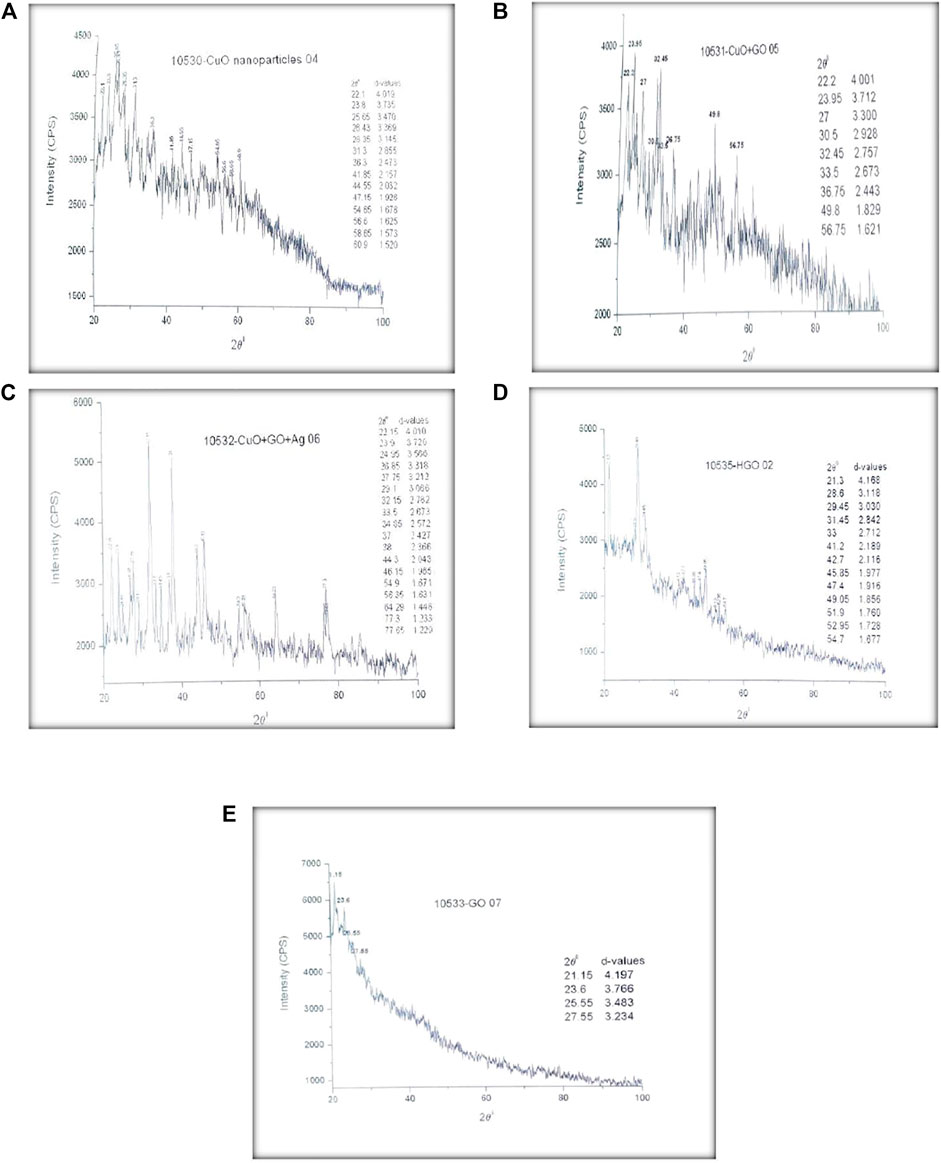
FIGURE 6. (A) XRD Results of CuO NPs (B) XRD Results of CuO-GO (C) XRD results of CuO-GO-Ag nanocomposite (D) XRD Results of GO synthesized by Hummers method (E) XRD Results of GO synthesized by Hydrothermal approach.
3.2.2 XRD Analysis of Graphene Oxide
GO synthesized by hummer’s method and Hydrothermal approach is also analyzed by XRD. XRD spectrum with different diffraction peaks at 2θ values is provided in Figure 5 (d, e). These diffraction peaks confirmed the synthesis of graphene oxide in the form of sheets as displayed in SEM images.
3.3 Energy Dispersive X-Rays Analysis
The elemental composition is determined via an EDX assessment. The elemental composition of synthesized CuO NPs, CuO-GO, CuO-GO-Ag, and GO was confirmed by EDX analysis. Figure 7 shows the EDX spectra of biologically synthesized CuO NPs, CuO-GO, CuO-GO-Ag, and GO. The presence of Cu, O, C, Ag peaks in the spectra’s confirmed the formation of our desired product.
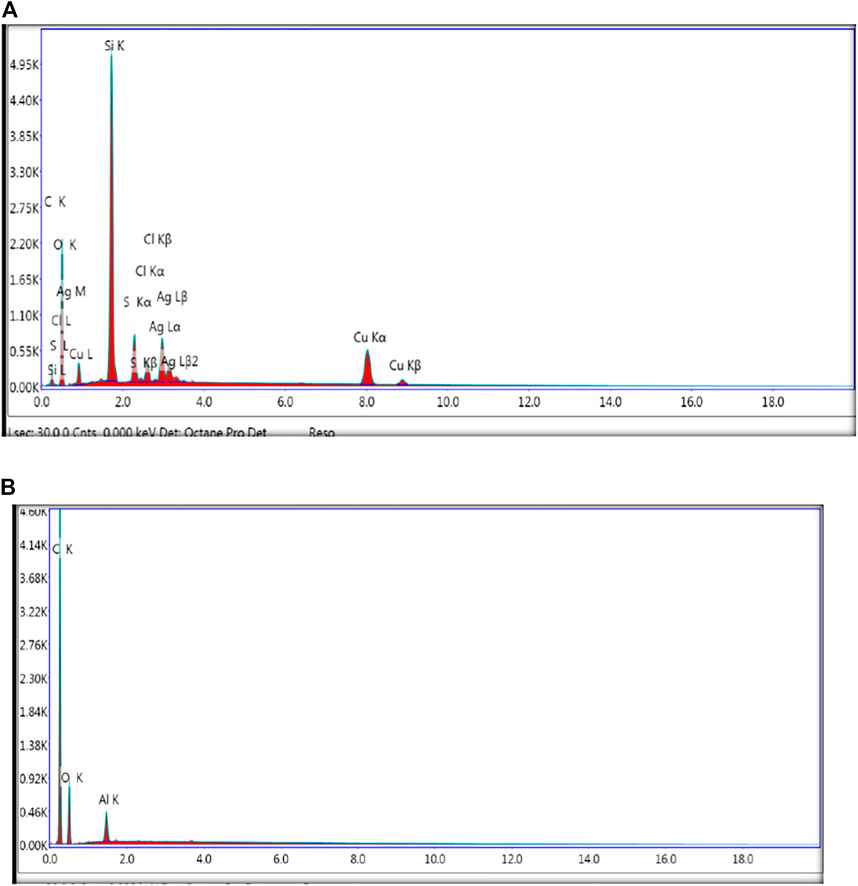
FIGURE 7. (A) EDX spectra of biologically synthesized CuO-GO-Ag Nanocomposite (B) EDX spectra of graphene oxide.
3.4 Anti-Inflammatory Activity
Inflammation is the first response to injury and wounds inside or outside the body. Anti-inflammatory drugs are used to reduce pain, inflammation, cold, and flu. Several drugs are reported for anti-inflammatory activity. In this, we have synthesized CuO-GO-Ag nanocomposite by biological approach and checked out its anti-inflammatory activity. The purpose of plant-mediated synthesis was to eliminate the side effects caused by chemically synthesized drugs. The results were then compared with the already reported anti-inflammatory drug (diclofenac Sodium). Results showed that nanocomposite synthesized by the green approach has a greater potential to reduce inflammation as compared to standard. Tables 1 and 2 indicate the concentration, absorbance, percent protein denaturation, and IC50 of the standard (Diclofenac Sodium) and sample (CuO-GO-Ag nanocomposite).
Diclofenac Sodium inhibited protein denaturation at 0.1 mg/ml concentration up to 10.98% and at 0.5 mg/ml concentration 35.37% protein denaturation was observed. Value of IC50 of Diclofenac Sodium at 0.1 mg/ml concentration was 0.46 while at 0.5 mg/ml concentration it was up to 0.74.
At 0.1 mg/ml concentration CuO-GO-Ag nanocomposite inhibited protein denaturation up-to 46.59% and at 0.5 mg/ml concentration it was 87.80%. Value of IC50 of CuO-GO-Ag nanocomposite at 0.1 mg/ml concentration was 0.11 while at 0.5 mg/ml concentration it was 0.13. Comparative study reveals that biologically fabricated CuO-GO-Ag nanocomposite showed high % inhibition and lower IC50 value as compared to standard diclofenac sodium. The lower the value of IC50 higher is the efficiency of the product. Figure 7 showed the comparative analysis of both standard and sample. The comparison graph indicates that CuO-GO-Ag nanocomposite showed less IC50 value thus a better choice to reduce inflammation as compared to standard.
3.5 Antioxidant Assessment
The production of free radicals is a chain reaction that may lead to several diseases like arthritis, skin disorder, tumors, and ulcers. To stop the activity of free radicals some molecules go and donate their electrons to make an unstable molecule highly stable and do not cause any damage. Those molecules which donate electrons and control these free radicals are known as antioxidants. These antioxidants scavenge the free radicals and reduce the damage caused by these radicals. Plants are rich in antioxidants, but some plants show high antioxidant potential while some have low. In this work, we have checked the antioxidant potential of our synthesized composite by DPPH assay and the Phosphomolybdenum method. Obtained results were then compared with standard ascorbic acid.
3.5.1 DPPH (2,2-Diphenyl-1-picrylhydrazyl) Assay
Furthermore, for contents of 0.05, 0.125, 0.25, and 0.5 mg/ml, the percent scavenging values for standard (Diclofenac sodium) were 12.77, 28.94, 36.49, and 46.70 percent. For concentrations of 0.05, 0.125, 0.25, and 0.5 mg/ml, the IC50 values of the standard were 0.20, 0.24, 0.37, and 0.51 mg/ml, respectively. Table 3 displays the scavenging % as well as the standard’s IC50 value (ascorbic acid).
The scavenging capacity of the sample was also evaluated by using UV-visible spectroscopy. % Scavenging for CuO-GO-Ag Nanocomposite at the concentration of 0.05, 0.125, 0.25, and 0.5 mg/ml was 15.96, 32.98, 47.87, and 59.57%. IC50 values for CuO-GO-Ag nanocomposite at concentration 0.05, 0.125, 0.25, and 0.5 mg/ml were 0.16, 0.20, 0.26, and 0.35 mg/ml % Inhibition and IC50 value of CuO-GO-Ag nanocomposite are shown in Table 4. CuO-GO-Ag nanocomposite shows high % scavenging and less value of IC50 as shown in Figure 8.
3.5.2 Phosphomolybdenum Method
Table 5 shows the standard (Ascorbic Acid) scavenging % and IC50 value. % At 0.3 mg/ml and 0.5 mg/ml, scavenging for Ascorbic Acid (Standard) was 44.22 percent and 58.42 percent, respectively. IC50 value at 0.3 mg/ml was 0.34 while at 0.5 mg/ml it was 0.38. % Scavenging for CuO-GO-Ag nanocomposite at concentration of 0.3 mg/ml and 0.5 mg/ml was 57.40 and 74.24% (Table 6). IC50 value at 0.3 mg/ml was 0.26 while at 0.5 mg/ml it was 0.21 IC50 values of CuO-GO-Ag nanocomposite were compared with Ascorbic Acid (Standard) as shown in Figures 9 and 10. It can be seen that better scavenging potential was shown by CuO-GO-Ag nanocomposite than Ascorbic Acid (Standard) as its IC50 was less.
4 Conclusion
Using a plant extract from Fagonia arabica, copper oxide nanoparticles were created. To create a CuO-GO nanocomposite, CuO nanoparticles were doped with graphene oxide. CuO nanoparticles were doped with graphene oxide and silver to create the CuO-GO-Ag nanocomposite. CuO nanoparticles, CuO-GO, and CuO-GO-Ag nanocomposite were utilized to study antioxidant and anti-inflammatory properties. The antioxidant and anti-inflammatory effects of the nanocomposite were explored using UV-visible spectroscopy. The NCs were studied using SEM, UV-Vis, EDX, XRD, TEM, and FTIR. The purity and particle size of the nanocomposite were investigated using XRD. JCPDS card number 48–1,548 verified the XRD study of CuO nanoparticles. SEM analyses were performed to check the shape and size distribution of nanoparticles. Graphene was synthesized by two methods including the hydrothermal method and the hummer’s method. SEM images of the hydrothermal method showed the particle nature while sheet-like structure observed in hummers method. GO was confirmed by JCPDS card number 04–0,836. Dilutions of CuO nanoparticles and composite were prepared for the conformation of antioxidant and anti-inflammatory activity. Antioxidant activity was checked by the DPPH method and the Phosphomolybdenum method. The value of IC50 was calculated for sample and standard. IC50 values were compared with sample and standard to check efficiency. Low IC50 showed that the efficiency of nanocomposite was high that was compared with standard.
Data Availability Statement
The original contributions presented in the study are included in the article/Supplementary Material, further inquiries can be directed to the corresponding author.
Author Contributions
SS: Conducted XRD analysis, performed some antibacterial experiments, acquisition of data, writing-original draft preparation. SM: Design of study, performed major experimental works, writing-original draft preparation. MJ: Material synthesis, visualization of data, writing reviewing and editing. SI: Conception, design of study, writing-original draft preparation and critical revision, supervision. UY: Performed FTIR analysis, reviewed revised manuscript and critical revision. HA: Analysis and/or interpretation of data, financial funding. NA: Conception, visualization of data, writing reviewing and editing. HI: Drafting the revised manuscript and critical revision. RA: Interpret the data, and critical revision. MA: Reviewed original manuscript, and critical revision. NT: Visualization of data, and critical revision. EE: Reviewing of data and financial support. SZ: Reviewed revised manuscript and critical revision. MS: Reviewed revised manuscript and critical revision. TR: Drafting the revised manuscript and critical revision.
Conflict of Interest
The authors declare that the research was conducted in the absence of any commercial or financial relationships that could be construed as a potential conflict of interest.
Publisher’s Note
All claims expressed in this article are solely those of the authors and do not necessarily represent those of their affiliated organizations, or those of the publisher, the editors and the reviewers. Any product that may be evaluated in this article, or claim that may be made by its manufacturer, is not guaranteed or endorsed by the publisher.
Acknowledgments
The authors extend their appreciation to the Deanship of Scientific Research at King Khalid University for supporting this work through research groups program under Grant number R.G.P.2/107/42. RA would like to acknowledge Taif University Researchers Supporting Project Number (TURSP-2020/209), Taif University, Taif, Saudi Arabia. The authors extend their appreciation to the Research Center at AIMaarefa University for funding this work.
References
Agarwal, H., Nakara, A., and Shanmugam, V. K. (2019). Anti-inflammatory Mechanism of Various Metal and Metal Oxide Nanoparticles Synthesized Using Plant Extracts: A Review. Biomed. Pharmacother. 109, 2561–2572. doi:10.1016/j.biopha.2018.11.116
Agarwal, H., and Shanmugam, V. (2020). A Review on Anti-inflammatory Activity of green Synthesized Zinc Oxide Nanoparticle: Mechanism-Based Approach. Bioorg. Chem. 94, 103423. doi:10.1016/j.bioorg.2019.103423
Al-Ziaydi, A. G. T. (2015). Protective Effect of Fagonia Arabica (L.) against Alloxan Monohydrate-Induced Diabetes in Albino Wistar Rats. Doctoral dissertation. Hyderabad: Kerbala University.
Ali, K., and Khan, H. (2021). Fagonia Indica; A Review on Chemical Constituents, Traditional Uses and Pharmacological Activities. Cpd 27 (22), 2648–2660. doi:10.2174/1381612826666201210105941
Alqasoumi, S. I., Yusufoglu, H. S., and Alam, A. (2011). Anti-inflammatory and Wound Healing Activity of Fagonia Schweinfurthii Alcoholic Extract Herbal Gel on Albino Rats. Afr. J. Pharm. Pharmacol. 5 (17), 1996–2001. doi:10.5897/ajpp11.190
Ayroldi, E., Cannarile, L., Migliorati, G., Nocentini, G., Delfino, D. V., and Riccardi, C. (2012). Mechanisms of the Anti-inflammatory Effects of Glucocorticoids: Genomic and Nongenomic Interference with MAPK Signaling Pathways. FASEB j. 26 (12), 4805–4820. doi:10.1096/fj.12-216382
Calabrese, V., Bates, T. E., Mancuso, C., Cornelius, C., Ventimiglia, B., Cambria, M. T., et al. (2008). Curcumin and the Cellular Stress Response in Free Radical-Related Diseases. Mol. Nutr. Food Res. 52 (9), 1062–1073. doi:10.1002/mnfr.200700316
Celotti, F., and Laufer, S. (2001). Anti-inflammatory Drugs: New Multitarget Compounds to Face an Old Problem. The Dual Inhibition Concept. Pharmacol. Res. 43 (5), 429–436. doi:10.1006/phrs.2000.0784
Chourasia, S. R., Kashyap, R. S., Deopujari, J. Y., Purohit, H. J., Taori, G. M., and Daginawala, H. F. (2014). Effect of Aqueous Extract and Fractions of Fagonia Arabica on In Vitro Anticoagulant Activity. Clin. Appl. Thromb. Hemost. 20 (8), 844–850. doi:10.1177/1076029613491458
Das, S. K., Behera, S., Patra, J. K., and Thatoi, H. (2019). Green Synthesis of Sliver Nanoparticles Using Avicennia officinalis and Xylocarpus Granatum Extracts and In Vitro Evaluation of Antioxidant, Antidiabetic and Anti-inflammatory Activities. J. Clust Sci. 30 (4), 1103–1113. doi:10.1007/s10876-019-01571-2
Denisov, E. T., and Afanas' ev, I. B. (2005). Oxidation and Antioxidants in Organic Chemistry and Biology. Boca Raton: CRC press.
Doyle, D. J., Goyal, A., Bansal, P., and Garmon, E. H. (2020). American Society of Anesthesiologists Classification. USA: ASA Class.) StatPearls Publishing. Treasure Island, FL.
Ďuračková, Z. (2010). Some Current Insights into Oxidative Stress. Physiol. Res. 59 (4).459–469. doi:10.33549/physiolres.931844
Edeoga, H. O., Okwu, D. E., Okwu, D. E., and Mbaebie, B. O. (2005). Phytochemical Constituents of Some Nigerian Medicinal Plants. Afr. J. Biotechnol. 4 (7), 685–688. doi:10.5897/ajb2005.000-3127
Forman, H. J. (2016). Redox Signaling: an Evolution from Free Radicals to Aging. Free Radic. Biol. Med. 97, 398–407. doi:10.1016/j.freeradbiomed.2016.07.003
Gour, A., and Jain, N. K. (2019). Advances in green Synthesis of Nanoparticles. Artif. Cell nanomedicine, Biotechnol. 47 (1), 844–851. doi:10.1080/21691401.2019.1577878
Haq, S., Rehman, W., Waseem, M., Shah, A., Khan, A. R., Rehman, M. U., et al. (2020). Green Synthesis and Characterization of Tin Dioxide Nanoparticles for Photocatalytic and Antimicrobial Studies. Mater. Res. Express 7 (2), 025012. doi:10.1088/2053-1591/ab6fa1
Hussain, I., Singh, N. B., Singh, A., Singh, H., and Singh, S. C. (2016). Green Synthesis of Nanoparticles and its Potential Application. Biotechnol. Lett. 38 (4), 545–560. doi:10.1007/s10529-015-2026-7
Iftikhar, N., Chatha, S. A. S., Ahmad, T., Ali, Q., Hussain, A. I., and Rathore, H. A. (2021). Combinatorial Chemistry & High Throughput Screening. Fagonia Arabica L. A. Review Its Phytochemistry, Pharmacology Traditional Uses 25 14 doi:10.2174/1386207325666210923120957
Ijaz, F., Shahid, S., Khan, S. A., Ahmad, W., and Zaman, S. (2017). Green Synthesis of Copper Oxide Nanoparticles Using Abutilon Indicum Leaf Extract: Antimicrobial, Antioxidant and Photocatalytic Dye Degradation Activitie. Trop. J. Pharm. Res. 16 (4), 743–753. doi:10.4314/tjpr.v16i4.2
Iqbal, J., Abbasi, B. A., Mahmood, T., Hameed, S., Munir, A., and Kanwal, S. (2019). Green Synthesis and Characterizations of Nickel Oxide Nanoparticles Using Leaf Extract of Rhamnus Virgata and Their Potential Biological Applications. Appl. Organomet. Chem. 33 (8), e4950. doi:10.1002/aoc.4950
Juthani, V. V., Clearfield, E., and Chuck, R. S. (2017). Non-steroidal Anti-inflammatory Drugs versus Corticosteroids for Controlling Inflammation after Uncomplicated Cataract Surgery. Cochrane Database Syst. Rev. 7 (7), CD010516. doi:10.1002/14651858.CD010516.pub2
Khan, S. A., Kanwal, S., Rizwan, K., and Shahid, S. (2018). Enhanced Antimicrobial, Antioxidant, In Vivo Antitumor and In Vitro Anticancer Effects against Breast Cancer Cell Line by green Synthesized Un-doped SnO2 and Co-doped SnO2 Nanoparticles from Clerodendrum Inerme. Microb. pathogenesis 125, 366–384. doi:10.1016/j.micpath.2018.09.041
Khan, S. A., Noreen, F., Kanwal, S., Iqbal, A., and Hussain, G. (2018). Green Synthesis of ZnO and Cu-Doped ZnO Nanoparticles from Leaf Extracts of Abutilon Indicum, Clerodendrum Infortunatum, Clerodendrum Inerme and Investigation of Their Biological and Photocatalytic Activities. Mater. Sci. Eng. C 82, 46–59. doi:10.1016/j.msec.2017.08.071
Kontoghiorghes, G. J., and Kontoghiorghe, C. N. (2019). Prospects for the Introduction of Targeted Antioxidant Drugs for the Prevention and Treatment of Diseases Related to Free Radical Pathology. Expert Opin. Investig. Drugs 28 (7), 593–603. doi:10.1080/13543784.2019.1631284
Matés, J. M., Perez-Gomez, C., and Blanca, M. (2000). Chemical and Biological Activity of Free Radical ‘scavengers’ in Allergic Diseases. Clinica Chim. Acta 296 (1-2), 1–15.doi:10.1016/s0009-8981(00)00215-1
McInnes, I. B., and Schett, G. (2017). Pathogenetic Insights from the Treatment of Rheumatoid Arthritis. The Lancet 389 (10086), 2328–2337. doi:10.1016/s0140-6736(17)31472-1
Meena, H., Pandey, H., Pandey, P., Arya, M., and Ahmed, Z. (2012). Evaluation of Antioxidant Activity of Two Important Memory Enhancing Medicinal Plants Baccopa Monnieri and Centella asiatica. Indian J. Pharmacol. 44 (1), 114. doi:10.4103/0253-7613.91880
Mei, Q., Li, Y., Li, B. N., and Zhang, Y. (2015). Oxidative Cleavage-Based Upconversional Nanosensor for Visual Evaluation of Antioxidant Activity of Drugs. Biosens. Bioelectron. 64, 88–93. doi:10.1016/j.bios.2014.08.061
Müller, L., Caris-Veyrat, C., Lowe, G., and Böhm, V. (2016). Lycopene and its Antioxidant Role in the Prevention of Cardiovascular Diseases—A Critical Review. Crit. Rev. Food Sci. Nutr. 56 (11), 1868–1879.doi:10.1016/s0009-8981(00)00215-1
Murakami, M., and Hirano, T. (2012). The Molecular Mechanisms of Chronic Inflammation Development. Front. Immun. 3, 323. doi:10.3389/fimmu.2012.00323
Nielsen, O. H., and Ainsworth, M. A. (2013). Tumor Necrosis Factor Inhibitors for Inflammatory Bowel Disease. N. Engl. J. Med. 369 (8), 754–762. doi:10.1056/nejmct1209614
Parveen, K., Banse, V., and Ledwani, L. (2016). April. Green Synthesis of Nanoparticles: Their Advantages and Disadvantages. AIP Conf. Proc. 1724, 020048. doi:10.1063/1.4945168
Rainsford, K. D. (2007). Anti-inflammatory Drugs in the 21st century, Inflammation in the Pathogenesis of Chronic Diseases, 3–27. doi:10.1007/1-4020-5688-5_1
Rhen, T., and Cidlowski, J. A. (2005). Antiinflammatory Action of Glucocorticoids - New Mechanisms for Old Drugs. N. Engl. J. Med. 353 (16), 1711–1723. doi:10.1056/nejmra050541
Rizvi, M. A., Ahmad, L., and Sarwar, G. R. (1996). Wild Medicinal Plants of Madinat Al-Hikmah and its Adjacent Areas. Hamd. Med. 39 (4), 8–10.
Saeed, M. A., and Sabir, A. (1999). Effects of Fagonia Cretica L. Constituents on Various Endocrinological Parameters in Rabbits. Turkish J. Biol. 23 (2), 187–198.doi:10.1016/s0378-8741(02)00365-3
Satpute, R., Bhattacharya, R., S Kashyap, R., J Purohit, H., Y Deopujari, J., M Taori, G., et al. (2012). Antioxidant Potential of Fagonia Arabica against the Chemical Ischemia-Induced in PC12 Cells. Iran J. Pharm. Res. 11 (1), 303–313.
Satpute, R. M., Kashyap, R. S., Deopujari, J. Y., Purohit, H. J., Taori, G. M., and Daginawala, H. F. (2009). Protection of PC12 Cells from Chemical Ischemia Induced Oxidative Stress by Fagonia Arabica. Food Chem. Toxicol. 47 (11), 2689–2695. doi:10.1016/j.fct.2009.06.007
Schett, G., and Neurath, M. F. (2018). Resolution of Chronic Inflammatory Disease: Universal and Tissue-specific Concepts. Nat. Commun. 9 (1), 3261–3268. doi:10.1038/s41467-018-05800-6
Schmid-Schönbein, G. W. (2006). Analysis of Inflammation. Annu. Rev. Biomed. Eng. 8, 93–151.doi:10.1146/annurev.bioeng.8.061505.095708
Shahid, S., Fatima, U., Sajjad, R., and Khan, S. A. (2019). Bioinspired Nano Theranostics Agent: Zinc Oxide; green Synthesis and Biomedical Potential. Dig. J. Nanomater. Biostruct 14, 1023–1031. https://chalcogen.ro/1023_ShahidS.pdf
Singh, R., Devi, S., and Gollen, R. (2015). Role of Free Radical in Atherosclerosis, Diabetes and Dyslipidaemia: larger-Than-life. Diabetes Metab. Res. Rev. 31 (2), 113–126. doi:10.1002/dmrr.2558
Yatoo, M. I., Gopalakrishnan, A., Saxena, A., Parray, O. R., Tufani, N. A., Chakraborty, S., Tiwari, R., Dhama, K., and Iqbal, H. M. N. (2018). Anti-Inflammatory Drugs and Herbs with Special Emphasis on Herbal Medicines for Countering Inflammatory Diseases and Disorders - A Review. Iad 12 (1), 39–58. doi:10.2174/1872213x12666180115153635
Keywords: bioinspired, nanocomposite, Fagonia arabica, inhibition, scavenging efficiency
Citation: Shahid S, Mansoor S, Javed M, Iqbal S, Yousaf U, Alsaab HO, Awwad NS, Ibrahium HA, Alzhrani RM, Alqahtani MD, Tamam N, Elkaeed EB, Zaman S, Sarwar MN and Riaz T (2022) CuO-GO-Ag; Green Synthesis With Fagonia Arabica and Biomedical Potential is a Bioinspired Nano Theranostics Composite. Front. Mater. 9:875148. doi: 10.3389/fmats.2022.875148
Received: 13 February 2022; Accepted: 15 March 2022;
Published: 01 April 2022.
Edited by:
Muhammad Idrees, Shenzhen University, ChinaReviewed by:
Pamphile Ndagijimana, Institute of Urban Environment (CAS), ChinaAzeem Ullah, Northwestern Polytechnical University, China
Copyright © 2022 Shahid, Mansoor, Javed, Iqbal, Yousaf, Alsaab, Awwad, Ibrahium, Alzhrani, Alqahtani, Tamam, Elkaeed, Zaman, Sarwar and Riaz. This is an open-access article distributed under the terms of the Creative Commons Attribution License (CC BY). The use, distribution or reproduction in other forums is permitted, provided the original author(s) and the copyright owner(s) are credited and that the original publication in this journal is cited, in accordance with accepted academic practice. No use, distribution or reproduction is permitted which does not comply with these terms.
*Correspondence: Shahid Iqbal, shahidgcs10@yahoo.com