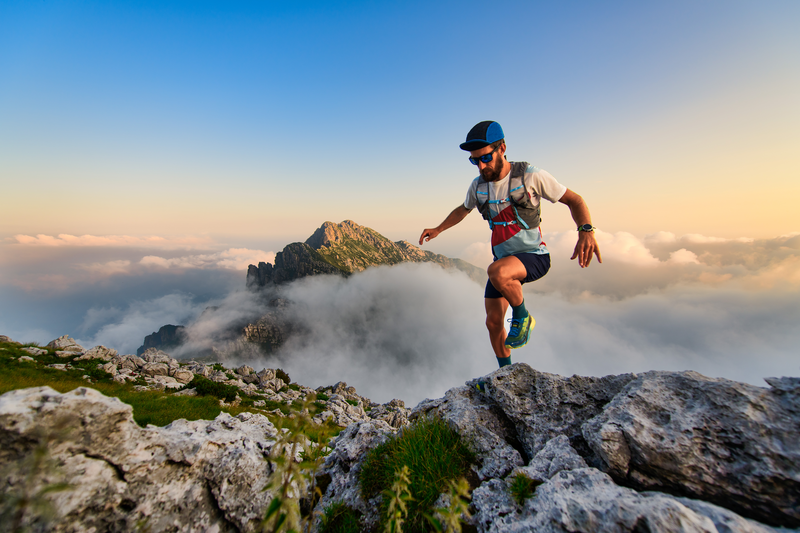
94% of researchers rate our articles as excellent or good
Learn more about the work of our research integrity team to safeguard the quality of each article we publish.
Find out more
ORIGINAL RESEARCH article
Front. Mater. , 23 May 2022
Sec. Structural Materials
Volume 9 - 2022 | https://doi.org/10.3389/fmats.2022.872381
This article is part of the Research Topic Application of Fiber and Composite Materials in Civil Engineering View all 4 articles
In order to study the tensile properties of a high-density polyethylene (HDPE) geonet for rainwater scour resistance and the micro properties of HDPE after tensile failure, a series of tensile tests on the HDPE geonet were carried out by the material testing system (MTS) tensile device. In this study, a correlation has been proposed to analyze the relationship between elongation and tensile strength of geonet material. The microscopic characteristics of the HDPE geonet were observed by using a scanning electron microscope SU8000. The high-density polyethylene geonet for rainwater scour resistance was evaluated based on mechanical performance and microstructure. The rainwater scour resistance properties of the high-density polyethylene geonet were estimated. It is determined that the geonet improved the rainwater scour resistance of the soil slope. The mechanical test results indicated that the longitudinal mechanical properties of the geonet are better than the transversal. The scanning electron microscope images of the geonet results confirmed that the polyethylene polymer produces fine and neat radial cracks during the tensile process of the geonet. The findings of this work are essential for the fundamental understanding, design, and application of the geonet.
Carbon dioxide peaking and carbon neutrality have become hot issues of political and economic activities in China and abroad. Due to rapid industrialization, large scale infrastructure development is taking place worldwide. This includes railways, high-speed highways, and elevated roads. Polyethylene is among the most widely used thermoplastics on the planet. Polyethylene is classified into three types (Khanam and AlMaadeed, 2015): high-density polyethylene (HDPE), low-density polyethylene (LDPE), and linear low-density polyethylene (LLDPE). Recently, with the rapid development of the industry, a number of high-density polyethylenes are generated every year in China (Mok et al., 2012). Because of its exceptional qualities such as toughness, near-zero moisture absorption, great chemical inertness, low coefficient of friction, simplicity of manufacturing, and remarkable electrical properties, HDPE is one of the most frequently used thermoplastics in the world. Geosynthetics, or factory-made polymer materials, have been employed successfully to tackle various geotechnical difficulties in civil engineering (Wei et al., 2020). The geonet composite is a geonet structure generally applied to the slopes of the highway. In addition, geonet materials are now commonly used in variable civil and material engineering. In general, their use has become a standard practice in constructing new disposal cells in many countries. Industry and academics have been interested in high-density polyethylene during the last few decades, particularly in automotive, aircraft, electronic systems, medical devices, civil construction, chemical industries, and other consumer uses (Khanam and AlMaadeed, 2015). The performance of the geonet has been used tremendously. To evaluate the advantages of the geonet, a series of tensile tests were conducted (Shi et al., 2020). Geonets are one of the most commonly used HDPE materials because of their limited space requirements, factory-controlled properties, generally lower cost, ease of transport and installation, and contributions to the bearing capacity of the soil.
At the moment, political debates throughout the world are centered on sustainable development. Demand for reducing energy consumption and emission of climate-related gases like CO2 and CH4 are big challenges for the construction industry. In China, many highway slopes are constructed between mountains that have extremely aggressive sloping sides (greater than 30°). Therefore, large quantities of soil are required to increase the stability of the side slopes, and as a result, the total volume available for cultivated soil is decreased. The use of geonets in the highway slope, which is made from high-density polyethylene, reduces the total cost of constructing highway slopes because they are convenient to produce (Wang et al., 2019). The geonet can be filled with sand, soil, or plant roots to fix them on the slopes of the highway. Disastrous heavy rains in the summer of 2017–2021 in China caused a lot of damage to property and human lives. The severity of damage to civil engineering structures in heavy rain areas draws the special attention of the research community. In the Chinese highway region, the challenges generated by the interaction between slopes and the hydrologic environment in traffic civil engineering are quite important (Chen et al., 2021). To meet environmentally safe slope requirements, it is important to maintain the slope system stability (the ultimate limit state) and integrity (the serviceability limit state) throughout the highway slope lifetime. Geonets are geocomposites of unique nature which combine the characteristics of the polymer (Pillai and Gali, 2021). When a geonet is used for rainwater, scour resistance material of a highway slope system, and alike, it is considered that this material will be charged with typically high pressure and tension. Moreover, the load in a highway slope on the geonet is sustained for a long time either until the soil may be consolidated or for the complete service life (Mok et al., 2012). The use of geonets allows good drainage performance, as well as increased stability of the slopes of the highway, because of their reinforcement effects (Jeon, 2016). This is to minimize the impact on human health and ensure environmental safety. A scanning electron microscope (SEM) has been employed in a diverse variety of polymer and composite investigations. Surface roughness, adhesive failure, fractured surfaces, net, and phase boundaries are examples of applications. High-density polyethylene geonet crack dispersion agglomeration and fiber pull-out can be observed by using a SEM. Geonets were composed of regenerated high-density polyethylene (HDPE), which was used to increase the stability and develop the hydraulic performance on the highway slope. Due to the importance of rainwater scour resistance in environmental and economic terms, the main objective of this study was to explore the tensile properties of high-density polyethylene geonet for rainwater scour resistance and the micro-properties of geonets.
The tensile properties and chemical resistance of the HDPE geonet were examined in accordance with geosynthetic geonets for highways (Ministry of Transport of the People’s Republic of China, 2004) (JT/T513-2004) and the test procedure for geosynthetic materials for highway engineering (JTG E50-2006) (Research Institute of Highway Ministry of Transport of the People’s Republic of China, 2006), respectively. In this study, two types of geonets were used to evaluate the tensile properties. Typical specifications for samples are provided in Table 1. These geonets are made of HDPE (high-density polyethylene). HDPE GN1 and GN2 geonets were produced by Shandong Hongxiang Material Company. The retention rate of UV strength was 97% longitudinally (1,500 h cycle) and 96% transversely (1,500 h cycle), and the black carbon content was 2.2%.
Photographs of these samples are shown in Figure 1.
The purpose of this study was to assess the impact of tensile strength on the high-density polyethylene geonet (El-Gayar, 1997). The effects of tensile strength on the high-density polyethylene geonet are investigated. Final test results, as well as the displacement, were reported. Factors influencing the mechanical capacity of the geonet are also examined (Dickinson et al., 2010). A schematic representation of the MTS-CMT tensile test device used in these experiments is presented in Figure 2. The MTS-CMT is a 0.7-m wide, 1.95-m tall, cylindrical steel pressure vessel capable of applying vertical stresses of up to 1,000 kPa using compressed air on a flexible rubber bladder.
All specimens were loaded continuously to rupture at a constant displacement rate of 20 mm/min using an MTS universal tensile testing machine (CMT4304). Mechanical properties of the test equipment are as follows: the test equipment of the HDPE geonet was the MTS-CMT tensile test device produced by Mechanical Testing Simulation Co. Figure 3 depicts the test instrument. The test apparatus has a minimum measurement value of 20 N and a maximum measurement value of 5000 kN. The test apparatus has an isometric tensile function; the loading rate of the test is controlled according to the computer, and the accuracy of the indicated value is no more than 0.5 N (Ni et al., 2019).
High-density polyethylene geonet materials were obtained from commercially produced lots. The mechanical properties of high-density polyethylene geonet materials were evaluated by using a tensile test. To verify that the test results are accurate, the geonet of the fixture is fixed by tightening the fixture bolts. The test results showed that the specimens are reliably clamped, and no slip occurs during the experiment, which meets the requirement of the “Test Methods of Geosynthetics for Highway Engineering” JTG E50-2006 and “Geosynthetics in Highway Engineerings Geonets and Geomatics” JT/T513-2004. According to the test methods of geosynthetics for highway engineering stipulated in the specification, tests were performed under the conditions of temperature 20 ± 2°C and humidity 65 ± 5%, for not less than 24 h before preparation. The specimens are considered to have been conditioned when the change in mass does not exceed 0.25% of the specimen mass during continuous weighing at an interval of at least 2 h. No less than five geonet specimens are used for each test of HDPE geonet in longitudinal and transversal directions. The longitudinal length and width of the specimen are 200 ± 1 mm, and the model’s transversal size and scope are 200 ± 1 mm, consistent with the international standard. It is like the actual condition that the high-density polyethylene geonet encounters during usage. The scanning electron microscope (SEM) is SU8000 type, HITACHI, Japan. The geonet started degradation in its mechanical properties after incubation and reached 50% of its initial tensile break strength and tear strength.
From the results of the GN1 test, linear regression curves were constructed (Wang et al., 2020). The performance limit pressures were evaluated as 401.33 and 202.56 N for longitudinal and transversal geonets, as shown in Figure 4. Under the best conditions, these values increased by 98.13% in tensile at the break. After the specimen reached the first peak turning point, the HDPE geonet specimen showed intermittent fiber-tearing sound. As the tensile force of the HDPE geonet grows, the elongation rate increases steadily, and the tensile force shows a linear relationship with the elongation rate. The result shows that the longitudinal geonet has a higher capacity than the transversal geonet. In the tensile test, an improvement of 198.77 N for the tensile strength was achieved. For the geonet samples selected in the test, the force curve of the HDPE geonet in two directions can be divided into three distinct phases. The first phase is a deformation phase. The strain is an elastic strain under tensile load conditions, which is approximately linear with the tensile strength. The second phase is a viscoelastic deformation phase, and the curve gradually transits from the straight line to the bending line, in which the strain growth rate gradually decreases. The third phase is a deformation stabilization phase; the curve further slows down, and the strain growth rate tends to be a constant value.
The slope of the linear relationship decreases substantially compared with the first peak turning point before, and the tensile force gradually tends to be smooth until it breaks. Table 2 shows the tensile properties of the high-density polyethylene geonet. GN 1 was considered as an example. The longitudinal tensile force of the geonet differs significantly from the transversal tensile force of the geonet. The longitudinal tensile force of the geonet is 401.33 N. The transversal breaking tensile force is 202.56 N. The difference between the breaking tensile forces is 198.77 N. The time taken to reach the maximum tensile force in the longitudinal direction of the HDPE geonet is 1490.25 s. The time taken to achieve the leading tensile force in the transversal direction is 1406.18 s. The longitudinal breaking elongation of the geonet is 49.02%. The transversal breaking elongation is 40.71%. The difference between breaking elongation is 8.31%. The difference between the time is 84.07 s. The tensile displacement at the maximum load in the longitudinal direction is 298.04 mm, and the tensile displacement at full load in the transversal direction is 281.41 mm. The difference between them is 16.63 mm. The measured tensile forces of the specimens at 2% elongation are 9.25 N in the longitudinal direction and 64.97 N in the transversal direction.
The curves of the load with displacement were obtained by using an experimental machine. Figure 5 shows the comparison between the numerical values of tensile forces in the longitudinal and transversal directions in the test data. The trend is approximately the same for longitudinal and transversal directions, with a gradual increase in the initial tensile force values. As the value approaches the maximum value, the growth rate slows down. When the tensile force values reached the maximum state, the corresponding values for longitudinal and transversal directions were 788.98 and 512.71 N. The corresponding elongation rates for longitudinal and transversal directions were 49.61 and 45.18%, respectively.
As shown in Figure 5, the tensile force values of the longitudinal direction grew with the increase in displacement, while they decreased with the changes in displacement from 25.17 mm. The tensile force values of the transversal direction grew with the increase in displacement. When the curve reached its peak, the curve began to decline. The tensile force values decreased with the changes in displacement from 24.86 mm. The tensile force had a significant influence on displacement, and the higher tensile force led to a bigger tensile displacement, indicating a lower property of geonets. Furthermore, it can be seen as obvious that the longitudinal tensile force was higher than the transverse. This demonstrated that longitudinal tensile properties were better than those of transverse. The uniaxial tensile test revealed that the tensile maximum of longitudinal properties was 53.88% higher than those of the transversal.
According to the entire tensile performance test results, the tensile properties of GN1 are relatively smaller than that of GN2. Figure 4 and Figure 5 show the tensile results. The experimental results had indicated 96.59% of tensile strength improvement. GN2 obtained the highest strength. The tensile strength dramatically influences the mechanical properties of HDPE geonets (Liu et al., 2021). Based on the test results, it can be determined that with the increase of the external load, the longitudinal and transversal tensile forces of both geonets first increase and then decrease. The peak values of longitudinal fracture tensile forces of GN1 are almost 49.13% smaller than those of GN2. The peak values of transversal fracture tensile forces of GN1 are almost 60.49% smaller than those of GN2. This is mainly due to the different laminated layers that constitute the geonet material. The number of laminated layers of GN2 is more than that of GN1, and the tensile force of GN2 is larger than that of GN1. The material concavity of GN2 and the bottom of the material are bonded and welded, and the peak values of the tensile force of GN2 are larger. The tensile force of GN1 increases uniformly with increasing elongation. The tensile force of GN2 fluctuates more as the elongation increases. Different structure types of HDPE geonet materials are susceptible to tensile strength, and the properties are greatly affected by the two directions. The increase in the tensile strength could be attributed to the improvement in the geonet structure that had contributed to the fiber load-bearing efficiency. The results indicated that the longitudinal direction is more likely to exert solid tensile properties under tensile test conditions. The main reason is that the longitudinal direction contains more geonet ribs than the transversal direction, which can effectively block the tensile.
High-density polyethylene geonet mechanical properties were characterized by using a universal testing machine. The cross-head speed was equal to 20 mm/min. The tensile tests were carried out at room temperature and humidity. The morphological properties of the fractured surfaces obtained from tensile tests of products were also examined by SEM analyses. Morphological analyses were performed using the Hitachi SU8000 cold field emission scanning electron microscope of the Tsinghua University Analysis Center. The energy range of SEM is about 0.2 eV, the electron source size is below 5 nm, and the probe current is about 2 nA, which can carry out ultra-high resolution observation.
The surface characterization of the morphology of the microstructure within the fracture and non-fracture regions of the investigated high-density polyethylene geonet is examined using SEM, and the obvious crack areas are the damaged regions. This is a result of the fact that geonet specimen types are made of HDPE. A scanning electron microscope was used to investigate the type one geonet. It is obvious that the surface line of the geonet presents non-linear, as shown in Figure 6. The non-linearity of the geonet fracture may relate to the disordered geometry of the fiber network, local mass non-uniformity, localization of existing inherent or newly generated damage inside the geonet sheets, and other factors that together result in a disorder of the stress field during fracture, which inevitably implies the randomness and complexity of the fracture process. In non-fracture regions, the obvious crack areas are damaged regions developed by increasing stress or the manufacturing and converting process.
A scanning electron microscope was employed to demonstrate the surface of high-density polyethylene geonet composites. Photos taken with a scanning electron microscope may be used to demonstrate fiber pull-out. The fracture of HDPE geonet material includes three processes: initial crack formation, gradual breaking of tiny fibers at the accumulated cracks by accelerated cracking, and fracturing of the geonet material. The surface morphology of the HDPE geonet before the beginning of stretching is delicate and smooth, as shown in Figure 6, with the presence of tiny fibers of varying thicknesses. HDPE polymer is internal to the external force stretching process; the inner polymer crystals under the tensile stress field produced the phenomenon of slippage. Under the external force, the molecular chains of the HDPE polymer can prevent the expansion of cracks, and the whole polymer section is relatively flat.
As the tensile test of the HDPE monolayer geonet proceeded, as shown in Figure 7, micro cracks began to form on the surface of the HDPE monolayer geonet, and the microfibers HDPE inside the specimen began to show signs of fracture with obvious plastic deformation. With the increase in tensile displacement, the number of cracks in the polymer pulled out from the polymer fracture surface increases. The magnitude of the gaps that were revealed grew larger. The cracks showed signs of gradual expansion. The cracks showed a radial pattern, which was analyzed due to the HDPE microfiber’s plastic deformation under external tensile forces.
The electron beam interacts with the surface region during the scanning process, generating secondary electrons from the composite. At the later stage of the tensile damage of the HDPE monolayer geonet, as shown in Figure 8, the microfiber fracture expands with the typical characteristics of ductile fracture. With the increase in force on the HDPE monolayer, stress concentration is easily generated at the cracks of polymer microfibers. As the tensile load on the HDPE monolayer geonet continues to increase, the microfibers fracture, and fracture voids can be seen. At the later stage of tensile fracture, the entire geonet specimen fails, and the tensile force no longer increases. It can be seen that the closer to the center point, the greater is the crack stretching width of the HDPE monolayer. The farther away from the central point, the smaller is the crack tensile width. The damage extends in the cracking region from the crack tip region to the crack boundary region.
Geonets are made of polymer materials and have a particular geometric shape. The HDPE monolayer geonet material has a more complicated structure, and the microstructure of high-density polyethylene has a particular influence on its tensile properties. For the problems existing in the quantitative study of the microstructure of geonets (Liu et al., 2013), a method was proposed in this work. The quantification of the crack image involves the following three steps: image segmentation, crack identification, and measurement. First, the image is converted to a binary image, noise in the binary image is removed, and crack spaces are fused. Then, the medial axis of the crack network is extracted from the binary image. Finally, the parameters of the crack area can be calculated. According to the calculation results, we can conclude that the crack area of the geonet increases from 541 pixels to 2619 pixels. The calculation results showed that the crack of the geonet increases gradually with the increase of tensile force. At the macroscopic level, the HDPE monolayer geonet shows uniform extension at the early force stage. The surface of the specimen is intact. The appearance of HDPE geonet cracks is microscopic, and there is even no visible deformation damage. With the increase of tensile force, the cracks of the HDPE geonet gradually extended, and tiny cracks began to emerge on the surface of the specimens, showing the characteristics of parallel-arranged vertical silver lines and linear cracks.
The microstructure of the geonet is a reflection of the macro-mechanics mechanism. At the microscopic level based on SEM, it can be concluded that the nature of deformation of geonets after being subjected to load is due to changes in the internal microstructure of HDPE. During the stretching process, the tensile force damaged the overall integrity of the polyethylene polymer, and cracks were generated within the polymer, which initially sprouted from the edges of the HDPE monolayer geonet and gradually expanded toward the middle part of the specimen along with the tensile test. It was found that the unstretched geonet, with smooth surface morphology, developed cracks on the fiber surface when the HDPE monolayer geonet was stretched to a certain length. The crack behavior of the geonet due to the complicated influences of the environment and stress will lead to a sustained increase in deformation, which seriously affects the service performance of the high-density polyethylene geonet for rainwater scour (Wei et al., 2020). When the tensile load was continued, the cracks developed gradually along the tip of the cracks in the direction perpendicular to the tensile axis, and the width of the cracks along the fiber axis increased accordingly. The test found that the material gradually deformed under the external tensile load, and with the expansion of the deformation, the material possessed a specific plastic deformation capacity. From the fractured picture of the stretched geomaterial, it can be seen that there is one crack in the specimen, which is surrounded by delicate and neat radial lines, and the fracture morphology of the stretched HDPE geonet specimen is abundant (Liu et al., 2021). It is seen that the longitudinal direction has a higher tensile strength, longer time, and a more significant tensile displacement than the transversal direction. This result is due to the low stress resistance of the transversal direction. Longitudinal was better than transversal of the high-density polyethylene geonet.
The rainfall scouring model test was carried out in the key laboratory of Tianjin transportation engineering green materials engineering technology center. The high-density polyethylene geonet properties when applied to the highway slopes were estimated by self-developed test equipment. The rainfall data of the study area and the Taihang Mountains in China were compiled, and the flow meter of the rainfall scour test was adjusted to 400 ml/min. Soil erosion analysis of rainfall scour was conducted based on the accumulated rainfall. The amount of soil erosion caused by rainfall scour was used to study the ability of the geonet to resist rainfall scour on the slope. The side slope grade was 1:1.25, according to the research statistics of the slope in the study area, and the soil material used in the rainwater scouring resistance test was powdered clay obtained from the Taihang Mountains high-speed area.
The test data were analyzed. Soil loss was used as resistance to rainwater washout. At the same rainfall volume, the loss value of GN1 was larger than that of GN2. At 6 L and 18 L, there are two obvious rainwater scour quantity change characteristic value points, corresponding to the accumulated soil loss values of 445.02 and 1792.32 g. The slope after comparing the two characteristic points knows that the slope of the soil loss relationship after 18 L rainwater is the largest. By analyzing the loss area of rainfall resistance, it was observed that it was mainly because the soil loss is larger due to the larger slumping of the slope surface of the tested side slope. The geotechnical network can significantly reduce the erosion of the slope surface by rainwater in the late stage of rainfall (Liu et al., 2022).
Geonets, made from high-density polyethylene materials, were designed for rainwater scour resistance. The unsaturated soil in the early stage of rainfall is dominated by water absorption. The soil slope is affected by the inflow of rainwater (Vianna et al., 2020). Geonets can improve the hydraulic performance on highway slopes. When the rainfall was 18 L, there was longer ditch runoff on the slope surface, and obvious surface erosion damage occurred. There was also obvious rainwater immersion at the bottom of the slope. There was minor surface erosion damage on the lower right side of the slope toe, and more soil in the middle of the slope was loose and floating. The original erosion area has developed to half of the slope after 24 L rainfall, showing apparent surface erosion. The trend growth of its loss increases slowly and then increases rapidly, as shown in Table 3. The geonet exhibits superior soil and water conservation properties. When the rainfall intensity was 6 L, the runoff and erosion of the slope and soil erosion were reduced than the bare area and the non-exposed slope. According to the test results, the GN1 treated slope was improved by 23.1%. The GN2 treated slope was improved by 29.5%. The multiple hindrances of the geonet play a leading role in regulating the hydrological effect of the slope (Aparicio Ardila et al., 2021). The high-density polyethylene geonet system affects the slope soil’s permeability and water storage capacity, improving soil slope erosion resistance. GN2 has better resistance to rainwater scouring than GN1. The reason for this is that GN2 is a stable tri-dimensional net structure, produced by multilayer plastic geonet welding together. The surface is uneven and crumbly, so it allows the soil to be filled in by 90%. The bottom geonet can avoid mass-wasting for its low elongation and high strength. The geonet and soil are firmly connected to protect the soil slope.
In recent years, great effort has been made toward establishing suitable roadway slopes, including material behavior, building phases, and improved slope models. In highway slope construction, high-density polyethylene geonet can serve a variety of roles such as draining, reinforcement, filtering, separation, protection, and proofing. The geonet has an important role in the hydrological effect. The high-density polyethylene geonet can stabilize rainwater infiltration and successfully inhibit surface runoff under continuous heavy rainfall. The geonet system significantly enhances the water storage capacity of the slope and greatly increases the permeability of the slope soil in the rainfall, which is totally different from that of the exposed slopes (Portelinha et al., 2021). The geonet leads to changes in the water flow due to its relative aperture. The geonet reinforced unsaturated layer conditions favored the development of a capillary break that also blocked the advancement of wetting for a period of the test. This interface hydraulic effect is observed to play an essential role in the stress transference from the geonet to soil. Rainfall infiltration is not constant, and it changes in four different stages. First, there is a progressive loss of soil suction due to infiltration. In the second stage, the proximity of the wetting front moves to the reinforcement layer. In the third phase, the sudden suction reduction due to the complete wetting at the geosynthetic soil interface affects the interaction and, consequently, reduces the rate of load increments. In particular, the water content increases due to the water pounded over the geonet interfaces, leading to higher increases in loads. In the fourth stage, the global suction is significantly reduced, and the shear strength of the soil is highly reduced. Analysis of the experimental data indicated that the geonets improved the performance of the reinforced slope compared with the exposed slope.
In this study, experimental tests were designed and conducted to study the tensile and microscopic properties of high-density polyethylene geonets for rainwater scour resistance. The results concluded from the study are as follows:
1) Experimental studies using the MTS-CMT apparatus yielded differences in the HDPE geonet material’s longitudinal and transversal tensile forces under the same loading conditions. The test results showed that the longitudinal tensile strength increased compared to the transversal tensile strength. The tensile experiments have been down for the GN1 and GN2 geonets. The test results showed that GN2 was better than GN1. In the process of actual use of civil engineering, high-density polyethylene geonet should be selected as much as possible to prevent the uneven bearing phenomenon of geonet material.
2) The microstructure morphology of the HDPE geonet polymer has a significant effect on the tensile properties of macro geonets. With the aid of scanning electron microscopy, it is concluded that the joint action between polymer molecular chains will break gradually in the tensile process, and the fibers at the edge of the geonet first show a 45° angle fracture. The middle part is inhibited and then gradually extends to the surrounding regions. This method can be used in further research analysis to compare unreinforced and reinforced high-density polyethylene geonet highway slopes.
3) The microstructural change of the HDPE geonet polymer will influence the macro mechanical properties of the geonet. The fracture surface morphology inside HDPE shows that the material fractures when the crack area exceeds 39%. In the preparation process of high-density polyethylene materials, more attention should be paid to considering the microstructural properties of molecular chains and the tensile mechanical properties of the materials to promote the application of geosynthetic materials in many fields.
4) In recent years, rainfall has been considered of great importance in many different civil projects. A laboratory test that simulates the mechanical of high-density polyethylene geonet reinforced slope soil layers was used to analyze the effect of moderate rainfall infiltration on slope tensile loads and rainwater resistance. High-density polyethylene geonets possess a combination of outstanding mechanical properties, resistant to rainwater washout properties attributed to them. The high-density polyethylene geonet is used as a reliable material for rainwater scour resistance. The results showed that on the whole, the GN2 geonet is more advantageous to the reinforcement of slopes under the conditions of rainwater erosion characteristic of slopes. In the research study, rainfall infiltration led to increased uploads mobilized by high-density polyethylene geonet reinforcements. This study provides a chance to acquire useful properties that will benefit the use of future geosynthetic materials.
The original contributions presented in the study are included in the article/Supplementary Material; further inquiries can be directed to the corresponding author.
Conceptualization: LL and YD; methodology: LL; validation: LL and YD; formal analysis: LL; investigation: LL and YD; data curation: LL; writing—original draft preparation: LL; and writing—review and editing: LL. All authors participated in discussions and contributed to this work.
The authors declare that the research was conducted in the absence of any commercial or financial relationships that could be construed as a potential conflict of interest.
All claims expressed in this article are solely those of the authors and do not necessarily represent those of their affiliated organizations, or those of the publisher, the editors, and the reviewers. Any product that may be evaluated in this article, or claim that may be made by its manufacturer, is not guaranteed or endorsed by the publisher.
The authors would like to express their gratitude to Jiangang Qiao and the Ministry of Transportation Science and Technology Project for their help during this research.
Aparicio Ardila, M., dos Santos Junior, R., Kobelnik, M., Aparecido Valentin, C., Silva Schliewe, M., Teixeira Coelho, A., et al. (2021). Semi-Rigid Erosion Control Techniques with Geotextiles Applied to Reservoir Margins in Hydroelectric Power Plants, Brazil. Water 13, 500. doi:10.3390/w13040500
Chen, J., Lei, X.-w., Zhang, H.-l., Lin, Z., Wang, H., and Hu, W. (2021). Laboratory Model Test Study of the Hydrological Effect on Granite Residual Soil Slopes Considering Different Vegetation Types. Sci. Rep. 11, 14668. doi:10.1038/s41598-021-94276-4
Dickinson, S., Brachman, R. W. I., and Rowe, R. K. (2010). Thickness and Hydraulic Performance of Geosynthetic Clay Liners Overlying a Geonet. J. Geotech. Geoenviron. Eng. 136, 552–561. doi:10.1061/(ASCE)gt.1943-5606.0000247
El‐Gayar, O. F. (1997). The Use of Information Technology in Aquaculture Management. Aquac. Econ. Manag. 1, 109–128. doi:10.1080/13657309709380207
Jeon, H.-Y. (2016). Analysis of Environmental Applicability of HDPE Geomembrane by Simulated Applicability Testing for Waste Containment Construction. Front. Mat. 3, 44. doi:10.3389/fmats.2016.00044
Khanam, P. N., and AlMaadeed, M. A. A. (2015). Processing and Characterization of Polyethylene-Based Composites. Adv. Manuf. Polym. Compos. Sci. 1, 63–79. doi:10.1179/2055035915y.0000000002
Liu, C., Tang, C.-S., Shi, B., and Suo, W.-B. (2013). Automatic Quantification of Crack Patterns by Image Processing. Comput. Geosciences 57, 77–80. doi:10.1016/j.cageo.2013.04.008
Liu, L., Dou, Y., and Qiao, J. (2022). Evaluation Method of Highway Plant Slope Based on Rough Set Theory and Analytic Hierarchy Process: A Case Study in Taihang Mountain, Hebei, China. Mathematics 10, 1264. doi:10.3390/math10081264
Liu, L., Qiao, J., and Dou, Y. (2021). Tensile Performance of PE-HD Vegetation Net and Single-Layer Geonet. Eng. Plast. Appl. 49, 110–114. doi:10.3969/j.issn.1001-3539.2021.10.020
Ministry of Transport of the People's Republic of China (2004). Geosynthetics in Highway Engineerings Geonets and Geomats: JT/T513-2004. Beijing, China: China Communications Press.
Mok, M. S., Blond, E., Mylnarek, J., and Jeon, H. Y. (2012). A New Approach to Evaluate the Long-Term Designing Normal Pressure of Geonets Using a Short-Term Accelerated Compressive Creep Test Method. Geotext. Geomembranes 30, 2–7. doi:10.1016/j.geotexmem.2011.01.002
Ni, T., Yang, Y., Gu, C., Liu, J., Chen, J., and Lou, X. (2019). Early-Age Tensile Basic Creep Behavioral Characteristics of High-Strength Concrete Containing Admixtures. Adv. Civ. Eng. 2019, 1–11. doi:10.1155/2019/9495231
Pillai, A. G., and Gali, M. L. (2022). Role of Particle Shape on the Shear Strength of Sand-GCL Interfaces under Dry and Wet Conditions. Geotext. Geomembranes 50, 262–281. doi:10.1016/j.geotexmem.2021.11.004
Portelinha, F. H. M., Santos, M. C., and Futai, M. M. (2021). A Laboratory Evaluation of Reinforcement Loads Induced by Rainfall Infiltration in Geosynthetic Mechanically Stabilized Earth Walls. Geotext. Geomembranes 49, 1427–1439. doi:10.1016/j.geotexmem.2021.05.006
Research Institute of Highway Ministry of Transport of the People's Republic of China (2006). Test Methods of Geosynthetics for Highway Engineering: JTG E50-2006. Beijing, China: China Communications Press.
Shi, X., Brescia-Norambuena, L., Tavares, C., and Grasley, Z. (2020). Semicircular Bending Fracture Test to Evaluate Fracture Properties and Ductility of Cement Mortar Reinforced by Scrap Tire Recycled Steel Fiber. Eng. Fract. Mech. 236, 107228. doi:10.1016/j.engfracmech.2020.107228
Vianna, V. F., Fleury, M. P., Menezes, G. B., Coelho, A. T., Bueno, C., Lins da Silva, J., et al. (2020). Bioengineering Techniques Adopted for Controlling Riverbanks' Superficial Erosion of the Simplício Hydroelectric Power Plant, Brazil. Sustainability 12, 7886. doi:10.3390/su12197886
Wang, J., Dai, Q., Si, R., and Guo, S. (2019). Mechanical, Durability, and Microstructural Properties of Macro Synthetic Polypropylene (PP) Fiber-Reinforced Rubber Concrete. J. Clean. Prod. 234, 1351–1364. doi:10.1016/j.jclepro.2019.06.272
Wang, J.-Q., Xu, L.-J., Lin, Z.-N., and Tang, Y. (2020). Study on Creep Characteristics of Geogrids Considered Sand-Geosynthetics Interaction under Different Loading Levels. J. Eng. Fibers Fabr. 15, 155892502095852. doi:10.1177/1558925020958520
Keywords: mechanical property, tensile test, scanning electron microscope, high-density polyethylene, geonet, rainwater scour, composite materials
Citation: Liu L and Dou Y (2022) Tensile and Microscopic Properties of High-Density Polyethylene Geonet for Rainwater Scour Resistance. Front. Mater. 9:872381. doi: 10.3389/fmats.2022.872381
Received: 09 February 2022; Accepted: 21 April 2022;
Published: 23 May 2022.
Edited by:
Jiaqing Wang, Nanjing Forestry University, ChinaReviewed by:
Maria Zielecka, Scientific and Research Centre for Fire Protection, PolandCopyright © 2022 Liu and Dou. This is an open-access article distributed under the terms of the Creative Commons Attribution License (CC BY). The use, distribution or reproduction in other forums is permitted, provided the original author(s) and the copyright owner(s) are credited and that the original publication in this journal is cited, in accordance with accepted academic practice. No use, distribution or reproduction is permitted which does not comply with these terms.
*Correspondence: Luliang Liu, MjAxNjExNjAxMDAxQHN0dS5oZWJ1dC5lZHUuY24=
Disclaimer: All claims expressed in this article are solely those of the authors and do not necessarily represent those of their affiliated organizations, or those of the publisher, the editors and the reviewers. Any product that may be evaluated in this article or claim that may be made by its manufacturer is not guaranteed or endorsed by the publisher.
Research integrity at Frontiers
Learn more about the work of our research integrity team to safeguard the quality of each article we publish.