- 1Department of Pharmaceutics, Faculty of Pharmacy, Shiraz University of Medical Sciences, Shiraz, Iran
- 2Pharmaceutical Sciences Research Center, Shiraz University of Medical Sciences, Shiraz, Iran
- 3Department of Quality Control, Faculty of Pharmacy, Shiraz University of Medical Sciences, Shiraz, Iran
- 4Department of Clinical Pharmacy, Faculty of Pharmacy, Shiraz University of Medical Sciences, Shiraz, Iran
Among different topical drug delivery systems, nanoemulsions (NEs) are attractive due to enhanced skin permeation potential and minimum toxicity. Topical nanoemulgels (NEMGELs) contain both gelling agents and NEs and thus can enhance skin permeation with sustained effects at the site of application. Oxybutynin (OXB) is an anti-muscarinic agent that is administered orally to treat hyperhidrosis but its systemic side effects may decrease patient compliance. Therefore, it assumes that oxybutynin-nanoemulgel (OXB-NEMGEL) with sustained release properties and skin permeation ability may be a suitable alternative. Using Design Expert software®, 17 OXB-NE formulations were prepared and optimized based on particle size, zeta potential and physical stability. Three polymers were used to prepare and optimized OXB-NEMGEL based on spreadability and viscosity. In-vitro drug release and ex-vivo skin permeation were investigated for optimized formulation. The model suggested by Design-Expert was quadratic and there was significant association between response variables including particle size and zeta potential and independent variables (Oil:Surfactant ratio, Oil:Water ratio, and surfactants HLB) with P-values of 0.0334 and 0.0173, respectively. Also, the lack of fit was not significant (P-values 0.057 and 0.569, respectively) which confirms the fitness of this model. Average size and zeta potential of optimized nanoemulsions were 99 ± 0.1 nm and -57.3 ± 0.5 mv, respectively. Carboxymethyl cellulose-based nanoemulgels (CMC-NEMGEL) showed acceptable viscosity with a shear thinning rheological pattern and good spreadability which released OXB within 8 h. Ex-vivo OXB skin permeation revealed OXB flux of 1.5 ± 0.3 and 3.6 ± 0.2 μg/cm2/h after 12 and 24 h, respectively, which was equal to 5.3 ± 1.1% and 11.9 ± 0.7% of drug, while simple OXB gel did not permeate through skin layers. Thus, OXB-NEMGEL with effective skin permeation potential would be a novel promising drug delivery system for hyperhidrosis management which may lower systemic side effects.
Introduction
Hyperhidrosis is a disorder in which more sweat is produced than the physiologic amounts and could be annoying for many patients. Oxybutynin (OXB) is an anticholinergic drug that has been administered orally for management of hyperhidrosis. Oral administration of OXB as a conventional therapeutic option can induce anticholinergic adverse reactions including urinary retention, dry mouth, and blurred vision which may reduce patient compliance (Wolosker et al., 2012; Wolosker et al., 2014; Delort et al., 2017). Therefore, it would be highly desirable to design a topical formulation of OXB for the localized management of hyperhidrosis. Considering OXB lipophilicity (log P of 4.36) and low water solubility (about 10 μg/ml) (Aulton, 2008), conventional topical formulations are not adequate means to promote enough skin permeation and efficient clinical outcome.
Topical drug delivery is an appropriate non-invasive route of administration with localized drug delivery to skin organelles which leads to lower systemic absorption and adverse reactions. Transdermal delivery of active pharmaceuticals would be potentially considered as sustained drug delivery approaches and skin permeation enhancement which can increase patient compliance in dosage form consumption (Aalto-Korte and Turpeinen, 1993; Ghasemiyeh and Mohammadi-Samani, 2020). Different types of nanocarriers such as lipid nanoparticles (Wissing, 2003; Muller et al., 2007; Pardeike et al., 2009; Ghasemiyeh and Mohammadi-Samani, 2018), liposomes (Perez et al., 2016; Doppalapudi et al., 2017; Sakdiset et al., 2017; Yokota and Tokudome, 2017), niosomes (Muzzalupo et al., 2017; Tavano et al., 2017; Aziz et al., 2018), nanoemulsions (NEs) (Primo et al., 2008; Zhou et al., 2010; Thiagarajan, 2011; Abd et al., 2015), hydrogels (Şenyiğit et al., 2011; Yoncheva et al., 2012; Ghasemiyeh and Mohammadi-Samani, 2019), nanoemulgels (NEMGELs) (Panwar et al., 2011; Kaur et al., 2017), etc. have been proposed for topical drug delivery with different advantages and drawbacks that should be overcome (Ghasemiyeh and Mohammadi-Samani, 2018). The selection of suitable nanocarrier highly depends on the physicochemical characteristics of the drug and the therapeutic goals of the study. Among all aforementioned-nanocarriers, NEMGELs are highly favorable and have the advantages of both NEs and gels which could enhance skin permeation and provide longer drug deposition in the target area. Topical gels have the limited capability in efficient delivery of hydrophobic drug while NEMGELs may overcome this limitation, for instance, highly lipophilic drugs such as cyproterone acetate and piroxicam were encapsulated within the nanoemulsion to enhance transdermal delivery (Ghasemiyeh et al., 2017; Mohammadi-Samani et al., 2018; Ghasemiyeh et al., 2019). Further, the presence of gelling agents (polymers) in NEMGELs, with suitable pharmaceutical characteristics including good spreadability, skin softening, and moisturizing properties, not only can increase patient compliance, but also forms suitable formulation appearance, sustained release pattern, and drug protection against light for photosensitive agents (Panwar et al., 2011; Ashara et al., 2016; Kaur et al., 2017; Ghasemiyeh and Mohammadi-Samani, 2021).
Commercial topical creams containing OXB treatment of overactive bladder disease are available; however, their systemic side effects are considerable. So, the main purpose of this study was design, optimization, and characterization of a suitable topical NEMGEL formulation for transdermal delivery of OXB in order to manage hyperhidrosis.
The main limitation of this study was the specific experiment to assess the exact pathway of drug penetration through the skin layers and evaluate the capability of this novel NEMGEL formulation for targeted drug delivery to sweat glands.
Materials and Methods
Materials
OXB hydrochloride (OXB) was gifted by Exir Company. Sodium alginate (ALG), methanol and acetonitrile (HPLC grade), hydroxy propyl methyl cellulose (HPMC), and sodium carboxy methyl cellulose (CMC), were purchased from a domestic supplier of Samchun, South Korea. Ethanol, acetic acid, sodium chloride, potassium chloride, disodium hydrogen phosphate, potassium dihydrogen phosphate, Tween 80, and Span 20 were purchased from a domestic supplier of Merck, Germany. Tefose 63 was gifted by Gattefosse, France. All other chemicals were of analytical grade and were used without further purification.
OXB Analysis
An isocratic system with flow rate of 1 ml/min was eluted and the mobile phase consisted of water: acetonitrile (50:50 ratio) which was adjusted at pH = 4 using acetic acid. OXB analysis was performed using a developed high performance liquid chromatography (HPLC) method with ultraviolet (UV) detector (CECIL 4900, England) and C8 column (Shimadzu-C8, Japan). To obtain high precise results, an internal standard (IS) was used in the analysis method.
HPLC Method Validation
Standard calibration curve was constructed in the concentration range of 0.625–10 μg/ml which was tested in triplicate and analyzed on three different days. Validation parameters including linearity, intra-day and inter-day precision and accuracy, sensitivity, limit of detection (LOD), and limit of quantitation (LOQ) were assessed.
OXB-Nanoemulsions Preparation
Considering pre-formulation studies, to obtain the optimum NE formulation with the highest physicochemical stability, three different surfactants (Tween 80, Tefose 63, and Span 20) in oil phases (liquid paraffin or polyethyleneglycol) were used to prepare NEs using the spontaneous emulsification method. Briefly, the organic phase containing ethanolic OXB and hydrophobic surfactants in liquid paraffin were added dropwise at 70°C to the aqueous phase containing hydrophilic surfactants and mixed continuously until it was cooled to 25°C (top-down technique).
OXB-NEs Optimization by Design-Expert®
Design-Expert® software (version 7.1, Stat-Ease Inc., Minneapolis, MN) and the Box-Behnken model were used for the NE optimization process. Considering preliminary studies, three independent variables including Oil:Surfactant (Oil:Sur) ratio (0.6–1.2), Oil:Water (Oil:Wat) ratio (0.1–0.2), and surfactants HLB (10.5–12.5) were selected and 17 runs were suggested by the software.
OXB-NEs Characterization
Particle Size and Size Distribution
Particle size of NEs was determined using a particle size analyzer (Shimadzu, SALD-2101, Japan) with laser diffraction technique (Montaseri et al., 2017). Each sample was evaluated in triplicate and results were reported as mean ± SD.
Zeta Potential
Zeta potential of NEs was measured using Zeta Check (Microtract, ZC007, Germany) without further dilution (Montaseri et al., 2017). The test was repeated in triplicate for each sample and results were reported as mean ± SD.
Stability Assessment
The centrifugation test is a technique that is used to assess stability of NEs. In this regard, a fixed volume of NEs was centrifuged at 5000 rpm for 30 min at room temperature and phase separations were evaluated and reported as instability signs of NEs.
OXB-NEMGELs Preparation
To optimize the best gel base to prepare OXB-NEMGELs, three different polymers, including carboxymethyl cellulose (CMC), hydroxypropyl methyl cellulose (HPMC), and alginate (ALG), in three various concentrations were prepared and stored in a refrigerator for 24 h. Then, OXB-NEs were mixed with gels under stirring to form NEMGELs.
OXB-NEMGELs Characterization
To optimize OXB-NEMGELs, the viscosity and spreadability were determined. The best formulation was selected for further examinations.
Viscosity
The viscosity of OXB-NEMGELs was assessed using a cone and plate viscometer (R/S PLUS, Brookfield) at room temperature and shear rate of 0–100 s−1 (Parhizkar et al., 2017). All tests were repeated in triplicate and results were reported as mean ± SD.
Spreadability
Considering previous studies, a predetermined amount of OXB-NEMGELs was placed on the surface plate and covered with another plate with the weight of 42 g for 3 min. Then the spreadability was calculated based on the diameter and surface of the spread OXB-NEMGELs. All tests were repeated in triplicate and results were reported as mean ± SD.
pH Determination
In this regard, pH of the 1% w/w OXB-NEMGELs was assessed by a pH meter after dilution of the formulations.
Drug Content
A known amount of OXB-NEMGELs was diluted with deionized water, mixed with acetonitrile, and centrifuged at 10,000 rpm for 15 min. Then the OXB content in the supernatant was analyzed using the validated analysis method.
In-vitro Drug Release
OXB in-vitro release pattern from selected OXB-NEMGELs was determined using a dialysis membrane (dialysis tubing cellulose membrane, D9652-100FT, Sigma-Aldrich, cutoff 14,000 Da) in phosphate buffer saline (PBS, with pH equal to 7.4) as release medium at 37°C while the medium was continuously stirred at 150 rpm (Rahnama et al., 2021). The optimized OXB-NEMGEL formulation was poured in a dialysis bag membrane and floated in 200 ml of release medium to maintain sink condition. Samples were collected at 1, 2, 4, 6, and 8 h and OXB amounts were determined using the validated analysis method and OXB release patterns were assessed. For better comparisons, OXB containing simple gels of selected formulations (OXB-GEL-C4 and OXB-GEL-A3) release and free-OXB dissolution pattern were assessed. Each formulation was examined in triplicate and results were reported as mean ± SD.
Animal Studies
Male hamsters were obtained from the Animal House, Shiraz University of Medical Sciences (SUMS), Shiraz, Iran. The animals were maintained and handled according to ethical codes and guidelines of SUMS as well as The Codes of Ethics of the World Medical Association EC directive 86/609/EEC for animal handling and experiments. The protocols in the current study were approved by the Ethics Committee of SUMS (Ethics code No. IR.SUMS.REC.1400.056).
Ex-vivo Skin Permeation
Ex-vivo skin permeation assessments were performed using hamster flank organ skin. The shaved flank organ skins were cut and mounted on Franz diffusion cells (surface area of 3.46 cm2 and acceptor volume of 30 ml) while the stratum corneum of the skins was facing up to the donor compartment. The receptor compartment was PBS (pH, 7.4) containing 2.5% of Tween 80 to maintain sink condition. At the donor compartment, optimized OXB-NEMGEL formulation and simple gels were applied. For further comparisons, skin permeation of free-OXB solution and simple gel (OXB-GEL-C3) were also assessed. Receptor compartment temperature was fixed at 32°C and medium was completely mixed at 250 rpm. Sampling was done at 0, 0.25, 0.5, 1, 2, 4, 6, 8, 10, 12, and 24 h after formulation application on the donor compartment and immediately an aliquot volume of fresh receptor medium was replaced each time. Samples were analyzed using the developed analysis method and the amounts of drug flux through the skin were measured. Different solvents in various ratios were evaluated to extract OXB from the permeation medium.
Results
OXB Analysis
OXB assay method was developed using HPLC at UV wavelength of 240 nm. Methyl prednisolone (MP) was used as an internal standard (IS). As shown in Figure 1A, OXB and MP retention times were 4 and 6 min, respectively. To determine the specificity of analysis method, the permeation media chromatogram was obtained to which indicates the non-interfering peaks in retention times of OXB and IS (Figure 1B).
HPLC Method Validation
Results revealed that there was no interaction between OXB and MP peaks with a blank sample which confirmed that this method had enough specificity/selectivity for OXB detection as shown in Figure 1. OXB analysis method validation results showed that there was acceptable linearity (R2 = 0.9996) between OXB concentrations and peak area ratios of OXB to MP with an equation of y = 0.5648x-0.0776. The method accuracy, inter-day and intra-day precision were 97.6 ± 3.6%, 97.1 ± 2.9%, and 97.8 ± 1.7%, respectively. Sensitivity of method was assessed in terms of LOQ of 0.625 μg/ml and LOD of 0.208 μg/ml.
OXB-NEs Optimization by Design-Expert®
NEs optimization was done by Design-Expert® software. Three independent variables including Oil:Sur ratio, Oil:Wat ratio, and surfactant HLB were determined and the response variables were set to particle size and zeta potential. According to the results of 17 runs, which were suggested by the software (Table 1), the model was quadratic and there was significant association between independent variables and response variables (P-value of 0.0334 and 0.0173) while lack of fit was not significant (P-value of 0.057 and 0.569) which confirms the fitness of this model (Table 2). As shown in Figure 2, the 3D-model graphs revealed the binary relationship between independent variables and response variables. According to these results and the quadratic model, there is an optimum surface to achieve the targeted particle size and zeta potential in NE formulations.
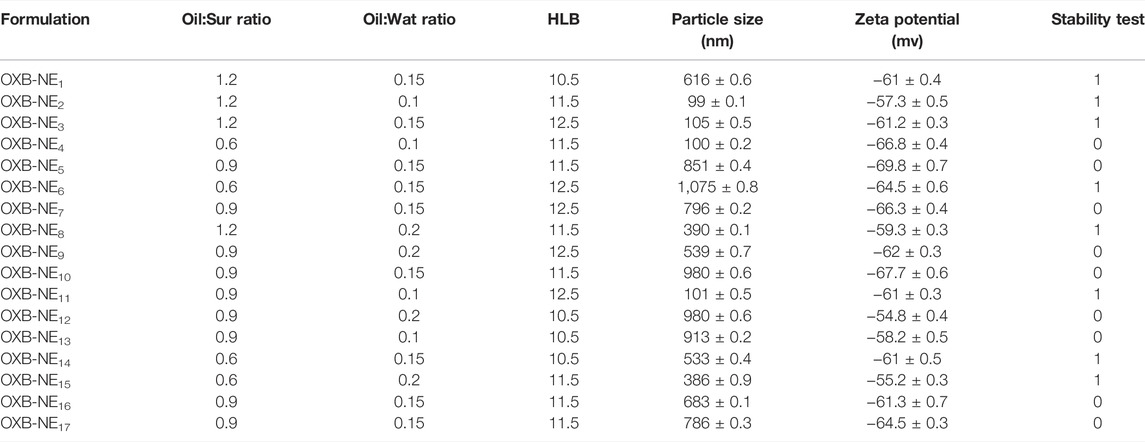
TABLE 1. Nanoemulsion ingredients, particle size, zeta potential, and stability study results (mean ± SD, n = 3).
OXB-NEs Characterization
Particle Size and Size Distribution
Mean particle size of different NE formulations is reported in Table 1 which showed the range of 99–1075 nm. A representative graph of particle size and size distribution of selected OXB-NE formulation (OXB-NE2) is shown in Figure 3, which revealed that the selected OXB-NE2 formulation had a mean particle size of 100 nm with a sharp distribution peak that confirmed the monodispersity in OXB-NE2.
Zeta Potential
Zeta potential of different OXB-NE formulations is listed in Table 1 which showed the range of −54 to −70 mv. Results revealed that the selected OXB-NE formulation (OXB-NE2) had a zeta potential of −57.3 ± 0.5 mv.
Stability Assessment
Centrifugation test was considered for stability assessment of different OXB-NE formulations. The results of the stability test were coded as 1 (for homogenous samples) and 0 (for two-phase samples) after centrifugation. Eight OXB-NEs formulations passed the centrifugation stability test. Stability results are shown in Table 1.
OXB-NEMGELs Preparation
Three different polymers including CMC, HPMC, and ALG in three various concentrations were used to prepare OXB-NEMGELs. All 9 polymeric solutions were prepared and stayed overnight. Freshly prepared NEs were mixed with polymeric solution in 1:1 ratio. The mixture was mixed slowly until homogenous NEMGEL formation. OXB-NEMGEL formulations are presented in Table 3.
OXB-NEMGELs Characterization
Viscosity
OXB-NEMGELs viscosity results are reported in Table 3. Based on the results, OXB-NEMGEL-C4 and OXB-NEMGEL-A3 had similar viscosity to the commercial piroxicam gel. Rheological pattern of OXB-NEMGEL-C4 and OXB-NEMGEL-A3 are depicted in Figure 4 as it has been shown results were compared with commercial piroxicam gel formulation that is available in the market.
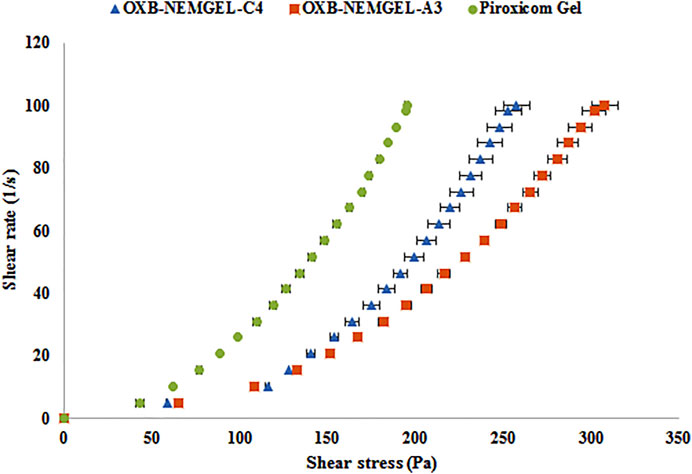
FIGURE 4. Shear stress vs. shear rate rheogram of CMC-EMGEL and ALG-EMGEL in comparison with piroxicam gel (n = 3).
Spreadability
OXB-NEMGELs spreadability results are presented in Table 3. Based on the results, OXB-NEMGEL-C4 and OXB-NEMGEL-A3 had similar spreadability to the commercial piroxicam gel.
According to the viscosity and spreadability results, further OXB-NEMGELs characterization tests were performed for the selected OXB-NEMGEL-C4 and OXB-NEMGEL-A3.
pH Determination
OXB-NEMGEL-C4 and OXB-NEMGEL-A3 pH were 5.84 ± 0.041 and 6.53 ± 0.047, respectively.
Drug Content
Drug content results of selected OXB-NEMGEL-C4 and OXB-NEMGEL-A3 were 103 ± 3% and 105 ± 2%, respectively.
In-vitro Drug Release
OXB release from selected OXB-NEMGEL-C4 and OXB-NEMGEL-A3 in comparison with their simple gel formulations (OXB-GEL-C4 and OXB-GEL-A3) and free-OXB dissolution pattern through dialysis membrane are depicted in Figure 5. As it is shown, OXB dissolution and release from OXB-NEMGEL-A3 were similarly fast; however, as it was predicted, both NEMGEL formulations release was slower than simple gels.
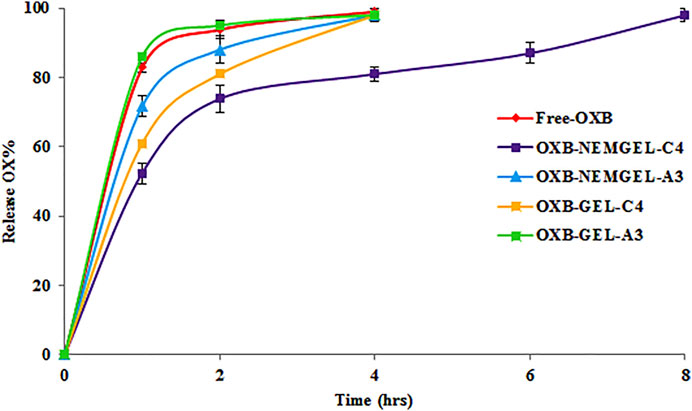
FIGURE 5. OXB release pattern from CMC-4-NEMGEL, ALG-3-NEMGEL, CMC-4-GEL, and ALG-3-GEL in comparison with free OXB permeation through a dialysis membrane (n = 3).
Animal Study
Ex-vivo Skin Permeation
According to the drug release results, OXB-NEMGEL-C4 was selected for further skin permeation assessments which was compared with simple CMC gel (OXB-GEL-C4). Results of the OXB flux through skin layers after 12 and 24 h are listed in Table 4.
Drug extraction process from release medium revealed that the best solvent for this purpose is acetonitrile:water (with ratio of 10:1) that results in OXB recovery of 87 ± 0.37% which was included in permeation assessment results.
Discussion
OXB Analysis
A simple and validated HPLC method was developed in this study to quantify OXB in different processes including drug content, drug release, and skin permeation assessments. Also, peak shapes revealed the acceptable symmetry and lack of tailing. The regression coefficient (R2) of calibration curve was 0.999, which confirms the linearity in the range of 0.625–10 μg/ml. Precision and accuracy results revealed that the analysis method had fulfilled the United States Pharmacopeia (USP) criteria of method validation (Health UDO and H Services, 2001; Ghasemiyeh et al., 2020). LOD and LOQ values confirmed the acceptable sensitivity of this method in comparison with previously reported studies (Zobrist et al., 2001; Varma et al., 2004; Nicoli et al., 2006; Ali et al., 2014).
OXB-NEs Optimization by Design-Expert®
OXB is a very slightly soluble drug (water solubility of about 0.01 mg/ml) with limited skin permeation from the conventional topical formulation (Nicoli et al., 2006). Therefore, in the present study, OXB-NEMGELs were prepared and evaluated in order to enhance skin permeation of OXB (USP C, 2008). During this study, a mixture of different non-ionic surfactants containing Tween 80, Tefose 63, and Span 20 were examined in a pre-formulation design study to determine the adequate range of surfactants. Liquid paraffin and polyethylene glycol 400 (PEG 400) were also evaluated as the organic phase. Different NE formulations were prepared and evaluated based on their particle size, zeta potential, and stability pattern. Finally, suitable surfactants (Tefose 63 and Tween 80) and organic phase (liquid paraffin) were selected for optimization.
Design-Expert® software with the Box-Behnken model was used for NEs optimization to achieve the smallest particle size with the lowest poly dispersity index, proper zeta potential, and physicochemical stability. Results revealed that the suggested quadratic model was significant while lack of fit was not significant, which confirm the fitness of the model considering other previous optimization studies (MHFS, 2011; Ghasemiyeh et al., 2017). All three independent variables had significant effect (with P-value of <0.05) on response variable (particle size and zeta potential). Considering Table 2 results, the Model F-value of for particle size and zeta potential was 4.31 and 5.53 that implied the model was significant. There is only a 3.34 and 1.73% chance that an F-value this large could occur due to noise. Values of “Prob > F″ less than 0.0500 indicate model terms are significant.
OXB-NEs Characterization
Surfactant mixtures with HLB ranges of 10–12 are a suitable emulsion stabilizer in formulation of oil in water NEs. Results of the present study revealed that increasing HLB values leads to NEs formation with smaller particle size and the smallest size was obtained by the HLB of 11.5 which was in agreement with previous reports (Min et al., 2018). Surfactants can facilitate NEs formation, therefore increasing surfactant concentration up to an optimum level could diminish NEs particle size and avoid unwanted particle aggregation (Ghasemiyeh et al., 2017), while enhancing over an optimum level, surfactants could induce micelle formation and aggregation would be predicted (Hasani et al., 2015). As it was predicted, decreasing Oil:Wat ratio diminished NE particle size due to the lower lipid amount (MHFS, 2011). For particle size Oil:Wat*HLB, Oil:Sur*HLB, (Oil:Wat) 2, and (Oil:Sur) 2 were significant model terms while for the zeta potential Oil:Wat* Oil:Sur, and (Oil:Wat) 2 were significant model terms.
As shown in Figure 2, 3D-model graphs revealed a binary relationship between independent variables and responses. The smallest NEs size was achieved with the lowest Oil:Wat ratio (1:10 ratio). Another parameter that was affecting the particle size was the Oil:Sur ratio. Decreasing the Oil:Sur ratio leads to an increase in the NEs particle size due to enhancement of surfactant concentration over the optimum level (Hasani et al., 2015). The smallest NEs were achieved with surfactant HLB value of 11.5, Oil:Wat ratio of 0.1, and Oil:Sur ratio of 1.2 (Kumar et al., 2014). Then, increasing the HLB value, decreasing Oil:Wat ratio, or increasing HLB value and Oil:Sur ratio leads to formation of smaller NEs. Increasing the Oil:Wat ratio, while decreasing the Oil:Sur ratio leads to a lower zeta potential and more stabilized NEs.
Previous studies reported that the suitable particle size ranges of NEs for topical drug delivery purposes is 125–148 nm (Deshmane, 2018). The negative zeta potential could obtain acceptable physical stability for this formulation by repulsion induction between nanoparticles and avoidance of particle aggregations (Patel et al., 2011). A centrifugation test was done as an indicator of stability of NEs (Shafiq et al., 2007). Results of the stability test were coded as 1 (for homogenous samples) and 0 (for two-phase samples) after centrifugation. In the present study, different prepared OXB-NEs particle sizes were in the range of 99–1057 nm and they showed negative zeta potential in the range of -54.8 to -69.8 mv. Considering software optimization, OXB-NE2 formulation with mean diameter of 99 nm and zeta potential of -57.3 mv was selected for further assessments. Eight OXB-NE formulations showed suitable stability during the test with no phase separation.
OXB-NEMGELs Characterization
Rheological test assessments were performed for all OXB-NEMGELs and compared with commercial piroxicam gel. As it was predictable, increasing the polymer concentration leads to higher gel viscosity. Formulations OXB-NEMGEL-C4 and OXB-NEMGEL-A3 containing CMC 4% and ALG 3% with the most similar viscosity to previous reports on NEMGEL and commercial topical gel as control were remarked (Deshmane, 2018).
Spreadability results were also similar to previous reports in the range of 5–11 g cm2/s (Bansal et al., 2015; Radhika and Guruprasad, 2016) which confirmed the acceptable NEMGELs spreadability. Comparing both viscosity and spreadability results of selected NEMGELs with commercial topical gel, OXB-NEMGEL-C4 and OXB-NEMGEL-A3 formulations were selected for further assessments.
OXB-NEMGEL-C4 and OXB-NEMGEL-A3 pH were 5.8 and 6.6, respectively, which were compatible with normal pH (5.5–7) ranges of skin (Kumar et al., 2014; Radhika and Guruprasad, 2016). The rheograms of selected NEMGELs showed a pseudoplastic shear thinning pattern which was similar to commercial control topical gel and is suitable for topical formulations.
The drug release pattern revealed a sustained release pattern for OXB from the NEMGELs in comparison with GEL-C4 and GEL-A3. Also, results showed that OXB-NEMGEL-C4 had slower release rate up to 8 h in comparison with OXB-NEMGEL-A3. Previous studies were reported that metronidazole, aceclofenac, mefenamic acid, and ofloxacin-loaded NEMGELs released 80, 55, 52, and 60% of loaded drugs within 6 h, respectively (Modi and Patel, 2011; Khullar et al., 2012; Rao et al., 2013; Jagdale and Pawar, 2017), therefore, the OXB-NEMGEL-C4 with slower drug release pattern was selected for skin permeation evaluation.
OXB-NEMGEL Ex-Vivo Skin Permeation
It was proved that NEs with average diameter of 100 nm permeate through the skin layers via the para-cellular pathway (Sharma et al., 2017). According to the previous study on transdermal OXB formulation, it had the advantage of targeted drug delivery and bypass hepatic first pass effect which reduces anticholinergic adverse effects related to OXB oral administration (Dmochowski et al., 2002). Although CYP450 enzyme in the skin could also exchange 5% of absorbed OXB to its metabolite with similar anticholinergic adverse effects, the presence of ethanol in this NEMGEL formulation could inhibit this enzyme and prevent the exchange of OXB to its metabolite (Jirschele and Sand, 2013). In addition, ethanol could act as a permeation enhancer and facilitate the dermal delivery of OXB. In the present study considering in-vitro results, the optimized OXB-NEMGEL-A3 was assessed for the ex-vivo skin permeation test. For better comparison, free-OXB and OXB-GEL-C4 were examined in the same condition. Free-OXB and simple gel (OXB-GEL-C4) formulation did not show detectable amounts of OXB permeation through skin layers while OXB-NEMGEL-C4 had 5% (36 μg/cm2) and 12% (80 μg/cm2) drug permeation after 12 and 24 h of formulation application, respectively. Previous study on skin permeation of OXB transdermal patch reported 11% drug permeation after 24 h (Jirschele and Sand, 2013). Another study on OXB transdermal spray showed threefold higher drug permeation than these results which may be attributed to the higher surface area (twofold) of used membrane compared to the present study (Bakshi et al., 2008).
Previous reports on topical drug delivery systems indicated that one of the most important parameters that could affect skin permeation is particle size (Ghasemiyeh et al., 2019). Overall, it seems that OXB-NEMGELs had good skin permeation and potential of deposition in skin organelles specifically sweat glands and the presence of the organic phase (oil) could enhance contact with the stratum corneum layer (Dev et al., 2015) and also the lipid nature of NEs could enhance skin permeation through para-cellular pathways (Sengupta and Chatterjee, 2017).
Conclusion
The aim of this study was to prepare a suitable pharmaceutical OXB-NEMGEL with sustained release pattern and permeation ability through the skin to decrease sweat glands secretion for hyperhidrosis management. Preliminary studies lead to select liquid paraffin as the organic phase and Tefose 63 and Tween 80 mixture as non-ionic surfactants. HLB value, Oil:Wat ratio, and Oil:Sur ratio were chosen variables to optimize the smallest OXB-NEs with a stable zeta potential. Three natural and semisynthetic common polymers (ALG, CMC, and HPMC) were used to prepare NEMGELs. According to the rheology results, CMC-based NEMGEL could release OXB in a sustained manner within 8 h. Skin permeation results revealed OXB flux of 1.5 ± 0.3 and 3.6 ± 0.2 μg/cm2/h after 12 and 24 h, respectively, which was equal to 5 and 12% of drug while simple OXB gel did not permeate OXB. Thus, OXB-NEMGEL with effective skin permeation potential would be a novel promising drug delivery system for hyperhidrosis management with advantages of minimizing systemic adverse drug reactions. In this regard, further ex-vivo and in-vivo studies are necessary to confirm the efficacy.
Data Availability Statement
The original contributions presented in the study are included in the article/Supplementary Material, further inquiries can be directed to the corresponding author.
Ethics Statement
The animal study and the protocols in the current study were reviewed and approved by the Ethics Committee of SUMS (Ethics code No. IR.SUMS.REC.1400.056).
Author Contributions
SM-S contributed to supervision, conceptualization, project administration, methodology, and writing–review and editing. PM contributed data curation, formal analysis, and writing–review and editing. PG contributed to formal analysis and writing–original draft, review and editing. SA contributed to supervision, conceptualization, project administration, methodology, and writing–review and editing. All authors have read and approved the manuscript.
Conflict of Interest
The authors declare that the research was conducted in the absence of any commercial or financial relationships that could be construed as a potential conflict of interest.
Publisher’s Note
All claims expressed in this article are solely those of the authors and do not necessarily represent those of their affiliated organizations, or those of the publisher, the editors, and the reviewers. Any product that may be evaluated in this article, or claim that may be made by its manufacturer, is not guaranteed or endorsed by the publisher.
Acknowledgments
This study was a part of Pharm. D. thesis of PM. The authors would like to thank Vice Chancellor for Research of Shiraz University of Medical Sciences [grant No. 98-01-36–21604] for financial support. Also, our thanks are due to Hassan Khajehei, for linguistic editing of the manuscript.
References
Aalto‐Korte, K., and Turpeinen, M. (1993). Transepidermal Water Loss and Absorption of Hydrocortisone in Widespread Dermatitis. Br. J. Dermatol. 128 (6), 633–635.
Abd, E., Namjoshi, S., Mohammed, Y. H., Roberts, M. S., and Grice, J. E. (2015). Synergistic Skin Penetration Enhancer and Nanoemulsion Formulations Promote the Human Epidermal Permeation of Caffeine and Naproxen. J. Pharm. Sci. doi:10.1002/jps.24699
Ali, I., Al-Othman, Z. A., Al-Warthan, A., Alam, S. D., and Farooqi, J. A. (2014). Enantiomeric Separation and Simulation Studies of Pheniramine, Oxybutynin, Cetirizine, and Brinzolamide Chiral Drugs on Amylose-Based Columns. Chirality 26 (3), 136–143. doi:10.1002/chir.22276
Aulton, M. E. (2008). Aulton’s Pharmaceutics: The Design and Manufacture of Medicines. Edinburgh, Churchill Livingstone: MLA (7th edition).
Ashara, K. C., Paun, J. S., Soniwala, M. M., Chavda, J. R., Mendapara, V. P., and Mori, N. M. (2016). Microemulgel: An Overwhelming Approach to Improve Therapeutic Action of Drug Moiety. Saudi Pharm. J. 24 (4), 452–457. doi:10.1016/j.jsps.2014.08.002
Aziz, D. E., Abdelbary, A. A., and Elassasy, A. I. (2018). Investigating Superiority of Novel Bilosomes over Niosomes in the Transdermal Delivery of Diacerein: In Vitro Characterization, Ex Vivo Permeation and In Vivo Skin Deposition Study. J. liposome Res. 29, 1–13. doi:10.1080/08982104.2018.1430831
Bakshi, A., Bajaj, A., Malhotra, G., Madan, M., and Amrutiya, N. (2008). A Novel Metered Dose Transdermal spray Formulation for Oxybutynin. Indian J. Pharm. Sci. 70 (6), 733–739. doi:10.4103/0250-474X.49094
Bansal, A., Singh, S., Saleem, M., and Imam, S. (2015). Preparation and Evaluation of Valdecoxib Emulgel Formulations. Biomed. Pharmacol. J. 1 (1), 131–138.
Delort, S., Marchi, E., and Corrêa, M. A. (2017). Oxybutynin as an Alternative Treatment for Hyperhidrosis. Bras. Dermatol. 92 (2), 217–220. doi:10.1590/abd1806-4841.201755126
Deshmane, S. V. (2018). Design and Development of Emulgel Preparation Containing Diclofenac Potassium. Asian J. Pharmaceutics (Ajp) Free full text articles Asian J Pharm. 11 (04). doi:10.22377/ajp.v11i04.1699
Dev, A., Chodankar, R., and Shelke, O. (2015). Emulgels: a Novel Topical Drug Delivery System. Pharm. Biol. evaluations 2 (4), 64–75.
Dmochowski, R. R., Davila, G. W., Zinner, N. R., Gittelman, M. C., Saltzstein, D. R., Lyttle, S., et al. (2002). Efficacy and Safety of Transdermal Oxybutynin in Patients with Urge and Mixed Urinary Incontinence. J. Urol. 168 (2), 580–586. doi:10.1097/00005392-200208000-00038
Doppalapudi, S., Jain, A., Chopra, D. K., and Khan, W. (2017). Psoralen Loaded Liposomal Nanocarriers for Improved Skin Penetration and Efficacy of Topical PUVA in Psoriasis. Eur. J. Pharm. Sci. 96, 515–529. doi:10.1016/j.ejps.2016.10.025
Ghasemiyeh, P., Azadi, A., Daneshamouz, S., and Mohammadi Samani, S. (2017). Cyproterone Acetate-Loaded Solid Lipid Nanoparticles (SLNs) and Nanostructured Lipid Carriers (NLCs): Preparation and Optimization. Trends Pharm. Sci. 3 (4), 275–286.
Ghasemiyeh, P., and Mohammadi-Samani, S. (2019). Hydrogels as Drug Delivery Systems; Pros and Cons. Trends Pharm. Sci. 5 (1), 7–24.
Ghasemiyeh, P., and Mohammadi-Samani, S. (2021). Polymers Blending as Release Modulating Tool in Drug Delivery. Front. Mater. 8 (490). doi:10.3389/fmats.2021.752813
Ghasemiyeh, P., and Mohammadi-Samani, S. (2018). Solid Lipid Nanoparticles and Nanostructured Lipid Carriers as Novel Drug Delivery Systems: Applications, Advantages and Disadvantages. Res. Pharm. Sci. 13 (4), 288–303. doi:10.4103/1735-5362.235156
Ghasemiyeh, P., Azadi, A., Daneshamouz, S., Heidari, R., Azarpira, N., and Mohammadi-Samani, S. (2019). Cyproterone Acetate-Loaded Nanostructured Lipid Carriers: Effect of Particle Size on Skin Penetration and Follicular Targeting. Pharm. Dev. Technol. 24 (7), 812–823. doi:10.1080/10837450.2019.1596133
Ghasemiyeh, P., and Mohammadi-Samani, S. (2020). Potential of Nanoparticles as Permeation Enhancers and Targeted Delivery Options for Skin: Advantages and Disadvantages. Dddt 14, 3271–3289. doi:10.2147/dddt.s264648
Ghasemiyeh, P., Vazin, A., Zand, F., Azadi, A., Karimzadeh, I., and Mohammadi-Samani, S. (2020). A Simple and Validated HPLC Method for Vancomycin Assay in Plasma Samples: the Necessity of TDM center Development in Southern Iran. Res. Pharma Sci. 15 (6), 529–540. doi:10.4103/1735-5362.301337
Hasani, F., Pezeshki, A., and Hamishehkar, H. (2015). Effect of Surfactant and Oil Type on Size Droplets of Betacarotene-Bearing Nanoemulsions. Int. J. Curr. Microbiol. App Sci. 4 (9), 146–155.
Health UDOH Services (2001). Bioanalytical Method Validation, Guidance for Industry. Available at: http://wwwfdagov/cder/guidance/4252fnlhtm.2001 (Accessed on: December, 2021).
Jagdale, S., and Pawar, S. (2017). Gellified Emulsion of Ofloxacin for Transdermal Drug Delivery System. Adv. Pharm. Bull. 7 (2), 229–239. doi:10.15171/apb.2017.028
Jirschele, K., and Sand, P. K. (2013). Oxybutynin: Past, Present, and Future. Int. Urogynecol. J. 24 (4), 595–604. doi:10.1007/s00192-012-1915-8
Kaur, G., Bedi, P., and Narang, J. K. (2017). Topical Nanoemulgel: A Novel Pathway for Investigating Alopecia. J. Nanomed Nanotechnol 8 (472), 2. doi:10.4172/2157-7439.1000472
Khullar, R., Kumar, D., Seth, N., and Saini, S. (2012). Formulation and Evaluation of Mefenamic Acid Emulgel for Topical Delivery. Saudi Pharm. J. 20 (1), 63–67. doi:10.1016/j.jsps.2011.08.001
Kumar, V., Mahant, S., Rao, R., and Nanda, S. (2014). Emulgel Based Topical Delivery System for Loratadine. ADMET and DMPK 2 (4), 254–271. doi:10.5599/admet.2.4.64
MHFS (2011). Effects of Oil and Drug Concentrations on Droplets Size of palm Oil Esters (POEs) Nanoemulsion. J. oleo Sci. 60 (4), 155–158. doi:10.5650/jos.60.155
Min, J.-Y., Ahn, S.-I., Lee, Y.-K., Kwak, H.-S., and Chang, Y. H. (2018). Optimized Conditions to Produce Water-In-Oil-In-Water Nanoemulsion and spray-dried Nanocapsule of Red Ginseng Extract. Food Sci. Technol. 38 (3), 485–492. doi:10.1590/fst.09517
Modi, J. D., and Patel, J. K. (2011). Nanoemulsion-based Gel Formulation of Aceclofenac for Topical Delivery. Int. J. Pharm. Pharm. Sci. Res. 1 (1), 6–12.
Mohammadi-Samani, S., Zojaji, S., and Entezar-Almahdi, E. (2018). Piroxicam Loaded Solid Lipid Nanoparticles for Topical Delivery: Preparation, Characterization and In Vitro Permeation Assessment. J. Drug Deliv. Sci. Tech. 47, 427–433. doi:10.1016/j.jddst.2018.07.015
Montaseri, H., Alipour, S., and Vakilinezhad, M. (2017). Development, Evaluation and Optimization of Superparamagnetite Nanoparticles Prepared by Co-precipitation Method. Res. Pharma Sci. 12 (4), 274–282. doi:10.4103/1735-5362.212044
Muller, R., Petersen, R., Hommoss, A., and Pardeike, J. (2007). Nanostructured Lipid Carriers (NLC) in Cosmetic Dermal Products☆. Adv. Drug Deliv. Rev. 59 (6), 522–530. doi:10.1016/j.addr.2007.04.012
Muzzalupo, R., Pérez, L., Pinazo, A., and Tavano, L. (2017). Pharmaceutical Versatility of Cationic Niosomes Derived from Amino Acid-Based Surfactants: Skin Penetration Behavior and Controlled Drug Release. Int. J. Pharm. 529 (1-2), 245–252. doi:10.1016/j.ijpharm.2017.06.083
Nicoli, S., Penna, E., Padula, C., Colombo, P., and Santi, P. (2006). New Transdermal Bioadhesive Film Containing Oxybutynin: In Vitro Permeation across Rabbit Ear Skin. Int. J. Pharm. 325 (1-2), 2–7. doi:10.1016/j.ijpharm.2006.06.010
Panwar, A., Upadhyay, N., Bairagi, M., Gujar, S., Darwhekar, G., and Jain, D. (2011). Emulgel: a Review. Asian J. Pharm. Life Sci. 2231, 4423.
Pardeike, J., Hommoss, A., and Müller, R. H. (2009). Lipid Nanoparticles (SLN, NLC) in Cosmetic and Pharmaceutical Dermal Products. Int. J. Pharm. 366 (1-2), 170–184. doi:10.1016/j.ijpharm.2008.10.003
Parhizkar, E., Emadi, L., and Alipour, S. (2017). Development and Evaluation of Midazolam In Situ Nasal Gel Properties in Presence of Solubility Enhancers at Cilia-Friendly pH. Macromol. Res. 25 (3), 255–261. doi:10.1007/s13233-017-5031-y
Patel, J., Patel, A., Raval, M., and Sheth, N. (2011). Formulation and Development of a Self-Nanoemulsifying Drug Delivery System of Irbesartan. J. Adv. Pharm. Tech. Res. 2 (1), 9. doi:10.4103/2231-4040.79799
Perez, A. P., Altube, M. J., Schilrreff, P., Apezteguia, G., Celes, F. S., Zacchino, S., et al. (2016). Topical Amphotericin B in Ultradeformable Liposomes: Formulation, Skin Penetration Study, Antifungal and Antileishmanial Activity In Vitro. Colloids Surf. B: Biointerfaces. 139, 190–198. doi:10.1016/j.colsurfb.2015.12.003
Primo, F. L., Rodrigues, M. M. A., Simioni, A. R., Bentley, M. V. L. B., Morais, P. C., and Tedesco, A. C. (2008). In Vitro studies of Cutaneous Retention of Magnetic Nanoemulsion Loaded with Zinc Phthalocyanine for Synergic Use in Skin Cancer Treatment. J. Magnetism Magn. Mater. 320 (14), e211–e214. doi:10.1016/j.jmmm.2008.02.050
Radhika, P. R., and Guruprasad, S. (2016). Nanoemulsion Based Emulgel Formulation of Lipophilic Drug for Topical Delivery. Int. J. Pharmtech Res. 9 (6), 210–223.
Rahnama, V., Motazedian, M. H., Mohammadi-Samani, S., Asgari, Q., Ghasemiyeh, P., and Khazaei, M. (2021). Artemether-loaded Nanostructured Lipid Carriers: Preparation, Characterization, and Evaluation of In Vitro Effect on Leishmania Major. Res. Pharm. Sci. 16 (6), 623. doi:10.4103/1735-5362.327508
Rao, M., Sukre, G., Aghav, S., and Kumar, M. (2013). Optimization of Metronidazole Emulgel. J. Pharm. (Cairo) 2013, 501082. doi:10.1155/2013/501082
Sakdiset, P., Kitao, Y., Todo, H., and Sugibayashi, K. (2017). High-Throughput Screening of Potential Skin Penetration-Enhancers Using Stratum Corneum Lipid Liposomes: Preliminary Evaluation for Different Concentrations of Ethanol. J. Pharm. (Cairo) 2017, 7409420. doi:10.1155/2017/7409420
Sengupta, P., and Chatterjee, B. (2017). Potential and Future Scope of Nanoemulgel Formulation for Topical Delivery of Lipophilic Drugs. Int. J. Pharm. 526 (1-2), 353–365. doi:10.1016/j.ijpharm.2017.04.068
Şenyiğit, T., Tekmen, I., Sönmez, Ü., Santi, P., and Özer, Ö. (2011). Deoxycholate Hydrogels of Betamethasone-17-Valerate Intended for Topical Use: In Vitro and In Vivo Evaluation. Int. J. pharmaceutics 403 (1-2), 123–129. doi:10.1016/j.ijpharm.2010.10.036
Shafiq, S., Shakeel, F., Talegaonkar, S., Ahmad, F. J., Khar, R. K., and Ali, M. (2007). Development and Bioavailability Assessment of Ramipril Nanoemulsion Formulation. Eur. J. Pharmaceutics Biopharmaceutics 66 (2), 227–243. doi:10.1016/j.ejpb.2006.10.014
Sharma, G., Thakur, K., Raza, K., Singh, B., and Katare, O. P. (2017). Nanostructured Lipid Carriers: a New Paradigm in Topical Delivery for Dermal and Transdermal Applications. Crit. Reviews™ Ther. Drug Carrier Syst. 34 (4), 355–386. doi:10.1615/critrevtherdrugcarriersyst.2017019047
Tavano, L., Picci, N., Ioele, G., and Muzzalupo, R. (2017). Tetracycline-niosomes versus Tetracycline Hydrochloride-Niosomes: How to Modulate Encapsulation and Percutaneous Permeation Properties. J. Drug 1 (2), 1–6. doi:10.24218/jod.2017.6
Thiagarajan, P. (2011). Nanoemulsions for Drug Delivery through Different Routes. Res. Biotechnol. 2 (3), 1–13.
Varma, M. V. S., Kaushal, A. M., and Garg, S. (2004). Rapid and Selective UV Spectrophotometric and RP-HPLC Methods for Dissolution Studies of Oxybutynin Immediate-Release and Controlled-Release Formulations. J. Pharm. Biomed. Anal. 36 (3), 669–674. doi:10.1016/j.jpba.2004.07.044
Wissing, S. (2003). Cosmetic Applications for Solid Lipid Nanoparticles (SLN). Int. J. Pharmaceutics 254 (1), 65–68. doi:10.1016/s0378-5173(02)00684-1
Wolosker, N., de Campos, J. R. M., Kauffman, P., and Puech-Leão, P. (2012). A Randomized Placebo-Controlled Trial of Oxybutynin for the Initial Treatment of Palmar and Axillary Hyperhidrosis. J. Vasc. Surg. 55 (6), 1696–1700. doi:10.1016/j.jvs.2011.12.039
Wolosker, N., Teivelis, M. P., Krutman, M., de Paula, R. P., Kauffman, P., de Campos, J. R. M., et al. (2014). Long-term Results of the Use of Oxybutynin for the Treatment of Axillary Hyperhidrosis. Ann. Vasc. Surg. 28 (5), 1106–1112. doi:10.1016/j.avsg.2013.12.024
Yokota, M., and Tokudome, Y. (2017). “Related Topic: Liposomes—Recent Advances and Future Perspective,” in Skin Permeation and Disposition of Therapeutic and Cosmeceutical Compounds. Japan: Springer, 137–142. doi:10.1007/978-4-431-56526-0_11
Yoncheva, K., Doytchinova, I., and Tankova, L. (2012). Preparation and Evaluation of Isosorbide Mononitrate Hydrogels for Topical Fissure Treatment. Cdd 9 (5), 452–458. doi:10.2174/156720112802650635
Zhou, H., Yue, Y., Liu, G., Li, Y., Zhang, J., Gong, Q., et al. (2010). Preparation and Characterization of a Lecithin Nanoemulsion as a Topical Delivery System. Nanoscale Res. Lett. 5 (1), 224. doi:10.1007/s11671-009-9469-5
Zobrist, R. H., Schmid, B., Feick, A., Quan, D., and Sanders, S. W. (2001). Pharmacokinetics of the R-And S-Enantiomers of Oxybutynin and N-Desethyloxybutynin Following Oral and Transdermal Administration of the Racemate in Healthy Volunteers. Pharm. Res. 18 (7), 1029–1034. doi:10.1023/a:1010956832113
Keywords: oxybutynin, nanoemulsion, nanoemulgels, skin permeation, hyperhydrosis
Citation: Mohammadi-Samani S, Masoumzadeh P, Ghasemiyeh P and Alipour S (2022) Oxybutynin-Nanoemulgel Formulation as a Successful Skin Permeation Strategy: In-vitro and ex-vivo Evaluation. Front. Mater. 9:848629. doi: 10.3389/fmats.2022.848629
Received: 04 January 2022; Accepted: 22 February 2022;
Published: 07 April 2022.
Edited by:
Lucia Baldino, University of Salerno, ItalyReviewed by:
Barkat Ali Khan, Gomal University, PakistanSatish Manchanda, Delhi Pharmaceutical Sciences and Research University, India
Copyright © 2022 Mohammadi-Samani, Masoumzadeh, Ghasemiyeh and Alipour. This is an open-access article distributed under the terms of the Creative Commons Attribution License (CC BY). The use, distribution or reproduction in other forums is permitted, provided the original author(s) and the copyright owner(s) are credited and that the original publication in this journal is cited, in accordance with accepted academic practice. No use, distribution or reproduction is permitted which does not comply with these terms.
*Correspondence: Shohreh Alipour, alipour_sh@sums.ac.ir