- Peter Grünberg Research Centre, College of Telecommunications and Information Engineering, Nanjing University of Posts and Telecommunications, Nanjing, China
Whispering gallery mode (WGM) cavities provide resonance configurations for light propagation through internal reflection, achieving high Q factors, low thresholds, and small mode volumes. GaN-based materials exhibit high freedom in band engineering and are highly compatible with contemporary semiconductor processing technology. Recently, lasers from artificial GaN microdisks, obtained by combining the excellent material properties of GaN with the advantages of WGM, have attracted considerable research attention. These have a wide application scope in optical communication, display, and optoelectronic integration. In this review, we summarize the recent advances in GaN-based WGM microlasers, including the fabrication methods for GaN microcavities, observations of optical pumped GaN microdisk lasing, lasing mechanisms, comparison of Q factors, lasing modes, and threshold properties, commonly used light field control techniques, and mode clipping methods. Furthermore, we introduce the recent advances in electrically driven GaN-based laser diodes, followed by research challenges and strategies for promising applications, such as electrically pumped lasers and optoelectronic chip integration.
Introduction
An optical microcavity confines light propagation through resonant recirculation. In the past few decades, there has been extensive research on the applications and challenges of this technology (Chen Y et al., 2021; Rupprecht et al., 2021; Tian et al., 2021). Based on the structure of a microcavity, lasing can be classified into three categories: The first is random lasing that is typically observed in semiconductor nanopowders or -films, where light is amplified along closed loop feedback paths resulting from recurrent scattering at crystal boundaries. The second is F-P lasing observed in nanorods, where light is amplified along the two end planes of the nanorod perpendicular to the nanorod axis. For random lasing observed in semiconductor nanopowders or -films, the scattering loss of crystal particles is large, and the lasing mode is difficult to control. For F-P lasing observed in nanorods, the transmission losses of the end planes are very large, and it is not easy to obtain high-quality low-threshold lasing. Compared to this, whispering gallery mode (WGM) lasing exhibits a significantly higher quality factor (Q), smaller mode volume, and lower lasing threshold because of the extremely weak optical loss of the total internal reflection (TIR) at the cavity boundary. The research on WGM lasing started in the 1970s, and early WGM lasing was realized on GaInP/InP (λ = 650 nm), ZnSe/CdS (λ = 510 nm), ZnO/SiO2 (λ = 390 nm), and InGaN/GaN (λ = 370 nm) substrates. In recent years, WGM lasing has promoted the development of several applications, such as thermal sensing and aerial mapping (Xu et al., 2018), photonic gyroscope (Xia et al., 2019), and biological imaging (Knapper et al., 2016).
With advances in materials science, there has been considerable research interest in nitrides. GaN, a representative third-generation semiconductor material, has a high refractive index and a direct band gap of 3.4 eV, and it is compatible with existing semiconductor processing technologies (Chung et al., 2010; Hill and Gather, 2014). There has been extensive research on the laser characteristics of GaN microcavities with different structures, such as hexagonal prisms, spheres, and strips (Feng et al., 2018; Shi et al., 2021). Prof. K.W. Choi of the University of Hong Kong’s research group (Li K. H et al., 2015; To et al., 2020) fabricated suspended circular and hemispherical microcavities supported by silicon columns using silica spheres as masks and obtained blue light lasers. Prof. Feng Yun’s research group of Xi’an Jiaotong University (Li et al., 2017; Li et al., 2018) prepared 3D microdisk cavities by material crimping and obtained low-threshold ultraviolet (UV) lasers. Prof. Sun Qian’s research group at the Institute of Semiconductors, Chinese Academy of Sciences (Wang et al., 2019), fabricated GaN microdisks using standard semiconductor processes, such as photolithography and reactive ion etching, and achieved electrically driven UV lasers. The above GaN microcavity lasers are based on WGM lasing and are widely used in quantum technology, UV spectrum manipulation, microdisplay, and visible light communication (Miao et al., 2016; Yang et al., 2019).
In this review, we summarize the recent advances in GaN microlasers. In Introduction, the classification of microcavites, advantage and typical reported of GaN microdisk laser was presented. The fabrication methods of GaN microdisk was then introduced in Design and Fabrication of III-N Micro- and Nanoresonantors. In Optically Pumped GaN Microcavity Lasing, the lasing mechanisms, including the mode evolution, Q factor, and threshold characteristics, are reviewed. The commonly used light field control techniques and mode clipping methods are introduced in Tailoring the Lasing Mode of GaN Microcavities. Then, recent advances in GaN-based microlasers, such as vertical laser diodes and microdisk laser diodes, are introduced in Electrically Driven GaN Laser Diodes. Finally, Optoelectronic Chip Integration presents further research challenges of the potential applications of large-scale on-chip integration.
Design and Fabrication of III-N Micro- and Nanoresonators
The idea of WGMs was presented several hundred years ago when the phenomenon of acoustic propagation was observed in the dome of St Paul’s Cathedral in 1912 (Yang et al., 2015). Winters et al. observed that if they were all standing near a wall (Winters and Coburn, 1979; Figure 1A), people could hear murmurs from anywhere in the gallery. This phenomenon also applies to light; that is, light can be reflected and confined to an infinite number of microsphere or microdisk cavities to enhance light–matter interactions (Kang et al., 1998; Rahmani and Jagadish, 2018). Since the 20th century, this phenomenon has been widely implemented in applications such as biosensors, optical communications, displays, and light sources (Skromme et al., 1999; Ferreira et al., 2016; Irmer et al., 2016; Zhang et al., 2016; Guo et al., 2019; Jiang et al., 2019; Toropov et al., 2021). To adapt to different applications and obtain high-quality and low-threshold lasers, microcavities of various shapes have been designed and fabricated (Figures 1B–G). The device architectures have smooth faces that ensure excellent optical properties.
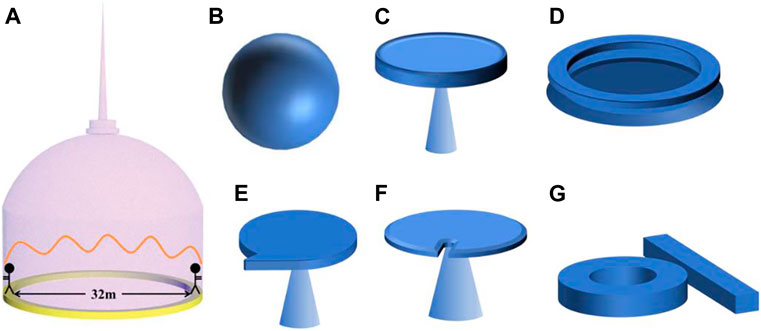
FIGURE 1. Schematic of various WGM resonators with different structures: (A) dome of St Paul’s Cathedral in London (Yang et al., 2015), reprinted with permission© WILEY‐VCH Verlag GmbH & Co. KGaA, Weinheim (Yang et al., 2015); (B) microsphere; (C) microdisk; (D) microring; (E) microdisk with chamfering; (F) microdisk with slit; (G) hybrid microcavities.
Some typical methods used to achieve GaN microstructures are molecular beam epitaxy (MBE) (Li and Waag, 2012; Higashiwaki et al., 2014), hydride vapor phase epitaxy (HVPE) (Lai et al., 2021; Seredin et al., 2021), and metal-organic chemical vapor deposition (MOCVD) (Peng et al., 2021; Wang et al., 2021). The resulting GaN structures have atomic-level smooth surfaces and low density of dislocations; moreover, the lasing thresholds are typically low, and the Q factors are high. However, these cavities have several obvious limitations. For example, the cavity structures are relatively fixed, such as hexagonal disks, microrods, or pyramidal cavities. With the development of top-down micro- and nanofabrication technologies, microcavities with controllable structures can be fabricated, resulting in chip-integrated semiconductor devices (Borselli et al., 2005; Xiao et al., 2008; Tamboli et al., 2009; Jiang et al., 2016; Sellés et al., 2016; Yonkee et al., 2016; Tabataba-Vakili et al., 2018; Yao and Yang, 2020). Our group introduced an experimental etching process to develop GaN-based microdisks (Zhu et al., 2018) (schematic illustration in Figure 2A). GaN microdisks in a grid pattern can be fabricated with Ni hard masks on a commercial GaN-on-silicon substrate with photolithography followed by dry and isotropic wet etching of silicon. As opposed to GaN microdisks manufactured using photoresist masks, this process can also be conducted using SiO2 and Al microsphere masks (Figure 2C). Zhang Y et al. (2014) fabricated GaN microdisks using microsphere lithography followed by dry and wet etching (Figure 2B). In this design, Al microspheres (diameter = ∼2 μm) were used as hard masks, and thus, the fabricated microdisks had diameters <2 μm. As illustrated in Figure 2D, the microdisk is much smaller than those reported in studies of Vicknesh et al. (2007) or Woolf et al. (2014).
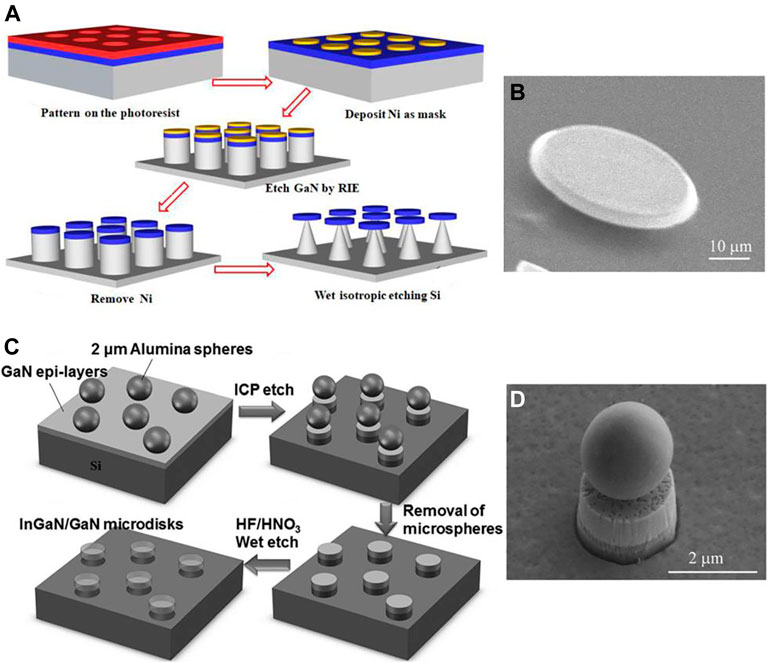
FIGURE 2. Schematic of fabrication of GaN microdisks with (A) an Ni hard mask and (C) a sphere metal mask. SEM images of the (B) floated GaN microdisk fabricated by the Ni hard mask (Zhu et al., 2018), reprinted with permission© Optical Society of America (Zhu et al., 2018), and (D) GaN microdisk fabricated with the sphere mask (Zhang Y. et al., 2014), reprinted with permission© AIP Publishing LLC (Zhang Y. et al., 2014).
Optically Pumped GaN Microcavity Lasing
Resonant Mode Calculation and Main Parameters
Compared with other types of lasing cavities such as F-P, WGM lasing has a higher quality factor (Q) and lower laser threshold owing to the small optical loss on cavity boundaries (Khurgin and Noginov, 2021). However, the nature of resonance is the same for all these laser structures. In theory, the resonance mode of a cavity depends on its optical length (L), and the mode is fitted using the following equation:
For F-P mode cavities,
The typical parameters used to characterize the lasing properties of microdisks are as follows: quality factor (Q), mode volume (V), free spectral range (FSR), and threshold value (Pth).
where
FSR is the resonant wavelength or frequency interval of two adjacent modes (Chen H et al., 2021). If we ignore refractive index dispersion, FSR is calculated using the following equation:
Threshold value (Pth) is another important parameter of lasing properties. Exploring the limits of low-threshold lasing is one of the key goals in the development of nanocavity lasers (Streiff et al., 2003). Most GaN microdisk lasers are fabricated using GaN quantum well (QW) materials. For GaN microdisks, Q is in the range 650–5,500 and Pth is < 270 kW/cm2. Microdisk cavities based on GaN materials have the advantages of high Q factors, small mode volumes, and low thresholds (Michler et al., 2000; Simeonov et al., 2008; Aharonovich et al., 2013; Wang et al., 2018).
WGM Lasing in GaN Microdisk Cavities
WGM lasing has a higher Q, lower lasing threshold, and smaller mode volume than other types of lasers. This is because the TIR on cavity boundaries can ensure weak optical loss. Owing to its excellent performance, WGM microcavity lasing has attracted considerable attention for several applications such as single-particle label-free sensing, microdisplays, imaging, and scanning (Vahala, 2003; Miller, 2009; Stock et al., 2013); GaN UV lasing has attracted considerable attention in optics research (Choi et al., 2011; Tabataba-Vakili et al., 2020). Typical results are presented in Figure 3, and several resonator structures (Figures 3A–D), such as spherical, disk, toroidal, and microbubble, have been demonstrated in recent years (Seo et al., 2003; Kwon et al., 2008; Sumetsky, 2010; Ward et al., 2014; Dong et al., 2017; Wang et al., 2017). As seen in Figure 3E, light is well confined in the x–y plane of GaN microdisks. The low- and high-order modes are confined in the thickness of the microcavity along the z-axis. Typical GaN lasing has a symmetric resonance spectrum, such as lasing spectra in Figure 3F reported by Zhu et al. (2017). Optically pumped lasing at room temperature has excitation energy densities in the range 188–298 kW/cm2. Optical resonances in multiple modes were observed in the gain range of 376–380 nm. Similar results are presented in Figure 3G, obtained by Zhang X et al. (2014). Optically pumped lasing was achieved at excitation energy densities of 9.06 mJ/cm2, and the obtained values of Q were approximately 770 at a lasing peak value of ∼430.2 nm. Although GaN microdisk lasers have been widely studied, owing to the rotational symmetry of these WGM cavity structures, they typically generate planar isotropic laser emission, resulting in extremely low collection efficiency in free space. Future studies should attempt to address this drawback (Yan et al., 2009; Bogusławski et al., 2018; Liu et al., 2019).
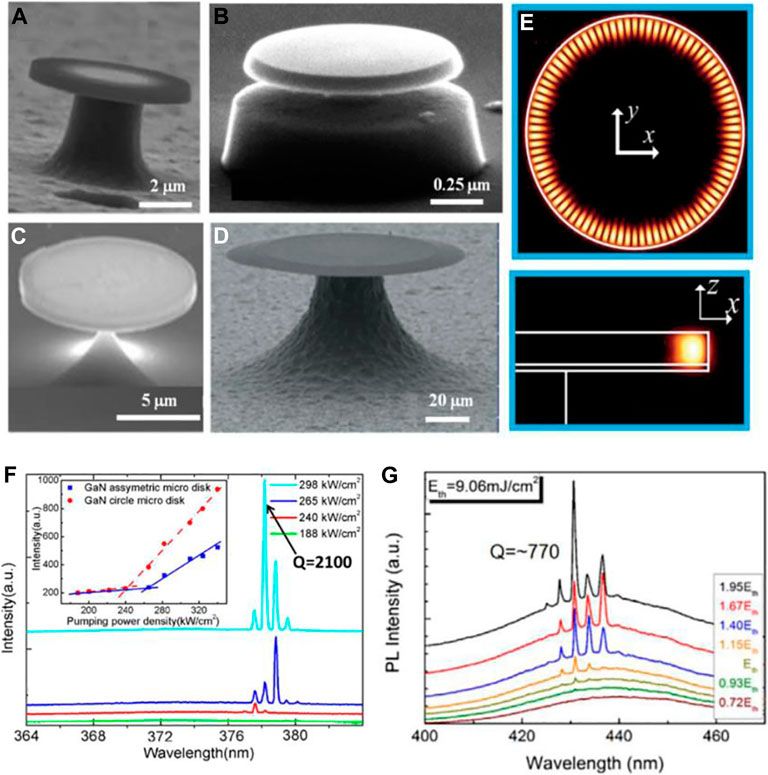
FIGURE 3. (A–D) GaN microdisk cavity lasers with various morphologies (Yan et al., 2009; Zhang X et al., 2014; Bogusławski et al., 2018; Liu et al., 2019); (E) WGM lasing simulation results of microdisk cavities; (F) lasing spectra of the GaN microdisk in the UV region; (G) lasing spectra of the GaN microdisk in the blue region (Zhang X et al., 2014; Zhu et al., 2017). Reprinted with permission© AIP Publishing (Yan et al., 2009; Zhu et al., 2018).
First, the smoothness of the optical cavities should be improved, as roughness and thickness of the cavity considerably influence its lasing properties. According to Alexander’s results, finite-thickness microdisks cannot address the degeneracy of the WGM doublets; however, these can limit their accessible Q factors to some extent (still very high) (Nosich et al., 2007). Based on this idea, Simeonov et al. (2008) demonstrated selective wet chemical etching for an AlInN sacrificial layer lattice-matched to GaN for the fabrication of air-gap photonic structures (Figures 4A–G). Optically pumped lasing at a wavelength of 408.8 and 471.1 nm was achieved under continuous wave (CW) laser pumping. Woolf et al. (2014) fabricated low-threshold lasers with high-quality optical cavities and gain materials of InGaN quantum dots or QWs. GaN microdisks with diameter = 1.2 μm and thickness = 200 nm were set as the resonant cavities (Figures 4H,I). Lasing oscillation with a main wavelength of approximately 450 nm and Q value of approximately 5,500 was realized with a threshold value of 184 μW (Figure 4J). Tamboli et al. (2007) realized lasing in microdisk arrays with diameter = 1.2 μm under continuous lasing operation at room temperature; the threshold was approximately 270 W/cm2, and Q was approximately 3,700. This research aimed to reduce the thickness of microdisks to the thickness of the quantum well layer.
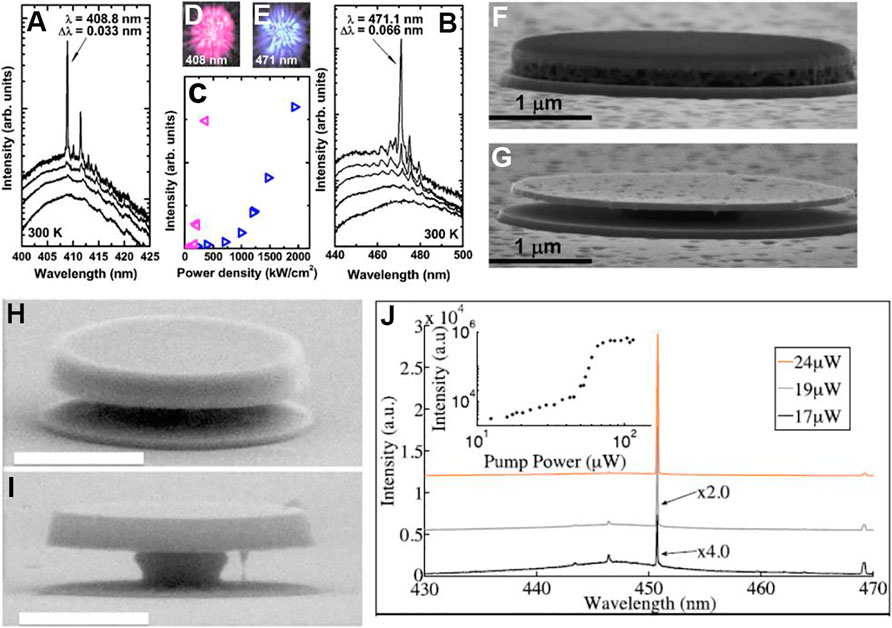
FIGURE 4. Lasing properties of GaN microcavities (Simeonov et al., 2008), reprinted with permission© AIP Publishing (Simeonov et al., 2008). Lasing spectra of GaN microcavities with (A) low and (B) high In content. (C) Threshold curves of GaN microcavities. Optical microscopy images with false color (D,E). SEM images of AlGaN (F) before and (G) after removal of the oxidation sacrificial layer. Lasing properties of floating GaN microstructures with a quantum well layer (Woolf et al., 2014): (H,I) SEM images; (J) lasing spectra with the threshold curve as an inset image, reprinted with permission© PNAS Publishing (Woolf et al., 2014).
Second, the cavity shape should be designed appropriately. Typically, the μ-PL system, which can provide uniform pump-laser beams with diameter = 20–50 μm, is used to measure the PL properties of GaN microcavities. Light in WGMs is usually limited to the periphery of the microdisks; therefore, the internal volume of the microdisk structure only slightly affects the laser properties. However, it also leads to energy loss due to light absorption in the cavity. For cavities with gain properties, gain, γ, should be introduced in the active region and a continuous condition should be added for the tangential component of the field on the boundary of this region (Smotrova et al., 2005). Reducing the disk size and thickness of the cavity is a useful strategy for achieving high-quality lasing. A ring-shaped active region can be as narrow as 0.2 μm and still provide the same value of the material gain threshold with the same mode as that in the uniformly active disk (Rex et al., 2001). In 1999, Zeng et al. (1999) studied the optical resonance modes in InGaN/GaN multiple-quantum-well microring cavities. Zhu et al. (2020a) obtained floating GaN microring lasing using the Burstein–Moss effect at room temperature. Zhang et al. (2020) designed and fabricated an asymmetric microring cavity by introducing a GaN-based eccentric microring with an inner hole located off the center; they achieved low threshold values and unidirectional lasing emission.
Third, realization of multi-functional microcavities such as single-mode or directional radiation is a popular research topic. A serious drawback of microdisk lasers is the low directionality of light emission inherent circular cavities. The isotropic lasing emission of WGM lasing typically limits their applications in several fields. To overcome this drawback, rotational symmetry of the microcavity should be broken by introducing defects in the mode field region or by forming an asymmetric/deformed cavity structure (Wang et al., 2010; Liu et al., 2012; Zhan et al., 2015). To control the emission direction of GaN microdisks, Zhang et al. (2021) fabricated a microring with diameter = 40 μm (Figure 5A) and an off-centered embedded hole and warped structure of strained III-nitride quantum well multilayers. In a similar previous study (Zhang et al., 2020) (Figure 5I), unidirectional and single-mode lasing was achieved. Compared with other studies, this study could more conveniently realize mode control of lasing properties (Zhizhchenko et al., 2019). Zhu et al. designed microdisks with corners (Figure 5H) (Zhu et al., 2017) and self-focusing structures (Figure 5D) (Zhu et al., 2019) to engineer the mode number and emission direction of the cavity. Li et al. (2018) presented a three-dimensional (3D) WGM with a self-bending microdisk, which comprised strain-released AlGaN/GaN bilayer films (Figure 5B); it provided more WGM photon degrees-of-freedom in the vertical direction compared to the two-dimensional WGM distributed in the horizontal direction. Xiao et al. (2017) demonstrated circular-side hexagonal resonator (CSHR) microstructures (Figure 5G) to realize unidirectional emission single-mode microlasers. Spiral microcolumns, slits, gratings, and notched elliptical structures were also introduced to the microcavities (Figures 5C,E,F) (Ben-Messaoud and Zyss, 2005; Wang et al., 2010; Cai et al., 2012), realizing lasing resonant. All these structures ensure that light radiates at a specific angle.
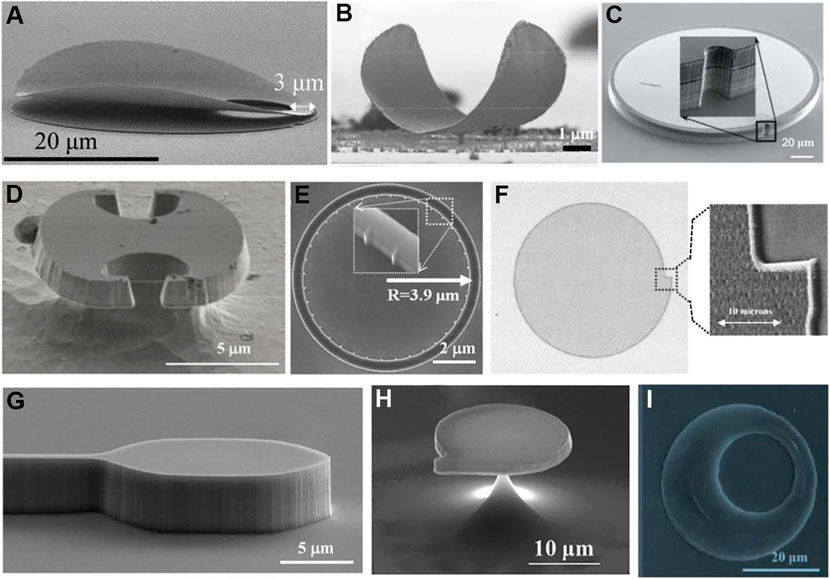
FIGURE 5. (A,B) Microring with an off-centered embedded hole and warped structure (Li et al., 2018; Zhang et al., 2021). (C) Notched elliptical microcavity quantum cascade lasers (Wang et al., 2010). (D) Microdisk with a self-focusing structure (Zhu et al., 2019). (E) Microdisk with grating structures (Cai et al., 2012). (F) Spiral microcolumn cavity (Ben-Messaoud and Zyss, 2005). (G) Circular-side hexagonal resonator (CSHR) microlasers (Xiao et al., 2017). (H) Microdisk with a corner (Zhu et al., 2017). (I) Microdisk with a hole (Zhang et al., 2020). Reprinted with permission© PNAS Publishing (Wang et al., 2010),© IOP Publishing (Zhu et al., 2019),© American Association for the Advancement of Science (Cai et al., 2012),© AIP Publishing (Ben-Messaoud and Zyss, 2005; Zhu et al., 2018),© OSA Publishing (Xiao et al., 2017; Zhang et al., 2020).
FDTD simulation (Chen and Wang, 2007; Jiang et al., 2012) is a conventional method to characterize the direction and mode characteristics of GaN lasing. Due to the isotropy of a cavity with a circular structure, the light field is uniformly circular, and it has an obvious standing wave shape. As shown in Figure 6A, Tamboli’s work indicated a first-order mode visible at wavelength = 418 nm with a distinct number of standing wave modes (Tamboli et al., 2007). Puchtler et al. (2015) presented high-quality factor devices comprising nitrides and simulated the optical field distribution. Wiersig and Hentschel (2006) demonstrated a microdisk with a hole, shown in Figures 6B,C; it showed a faint resemblance to a WGM, but it implied a clear directed emission due to refractive escape. The directional emission is clearer in Zhu et al.’s work (Zhu et al., 2017); it had a chamfer in the disk (SEM image in Figure 5H). Figure 6D shows light emitted from the corner of the disk. It is more interesting in their other work, in which GaN microdisks with focus effect were designed (SEM image in Figure 5D) (Zhu et al., 2019). The simulation results of two lasing peaks at wavelengths of 375.3 and 377.3 nm were consistent with the experimental lasing spectra (Figures 6E,F). These modes are focused on the two sides of the cavity. Lasing of warped microring in Zhang’s work (Zhang et al., 2021) presented clear anisotropic characteristics (Figures 6G,I). Light is efficiently collected and plotted in Figure 6H. The far-field of the warped microring illustrated in Figure 6J had the same anisotropic characteristics (0–30°). This indicates that the warped microring has a small far-field angle.
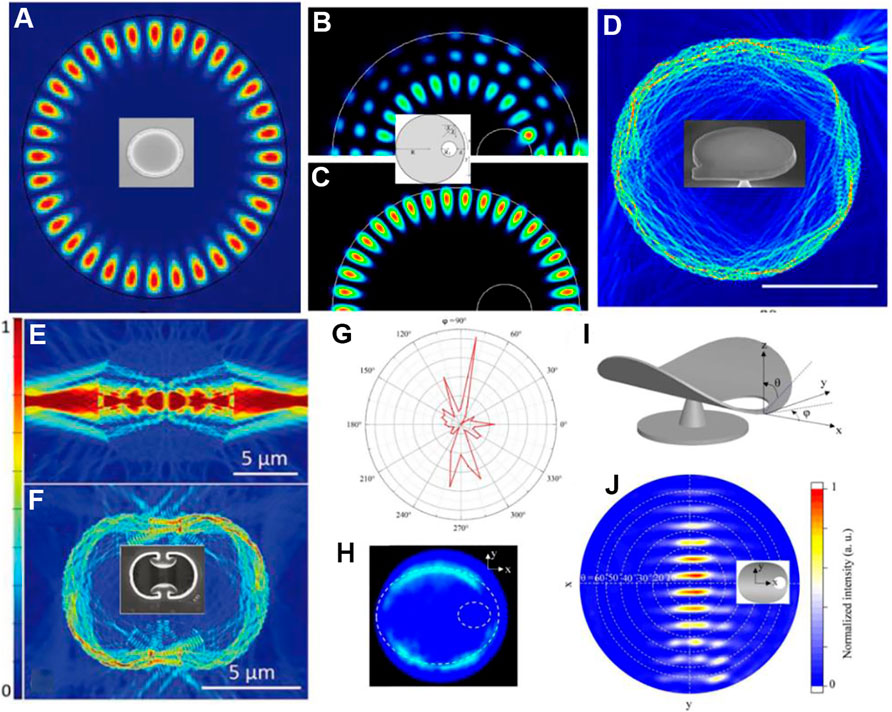
FIGURE 6. FDTD simulation results of a (A) microdisk (Tamboli et al., 2007), (B,C) microdisk with a hole (Wiersig and Hentschel, 2006), (D) GaN microdisk with a corner (Zhu et al., 2017), and (E,F) GaN Penrose microcavity (Zhu et al., 2019). (G) PL intensity of the laser mode at 441.3 nm in various detection angles. (I) Illustration of warped microdisks in a 3D spherical coordinate system. (H) CCD image of the warped microring. (J) Far-field pattern of the warped microring calculated by FDTD (Zhang et al., 2021). Reprinted with permission© Nature Publishing Group (Tamboli et al., 2007),© American Physical Society (Wiersig and Hentschel, 2006),© AIP Publishing (Zhu et al., 2018),© IOP Publishing (Zhu et al., 2019),© 2021 Chinese Laser Press (Zhang et al., 2021).
Tailoring the Lasing Mode of GaN Microcavities
Compared with multi-mode lasers, output lasing with short modes, even a single mode, is valuable for practical applications (Xu et al., 2012; Feng et al., 2014; Nakajima et al., 2019). Conventional experimental schemes, such as reducing the cavity size and introducing structures such as gratings, slots, or nano-antennas, have been widely utilized to achieve single-mode lasers. Fujita and Baba (2002) introduced saw tooth structures for GaInAsP–InP microcavities (Figure 7A). Moiseev et al. (2017) introduced antenna structures on the side of InAs microdisks (Figure 7E). Bogdanov et al. (2015) etched slits on the surface of InAs microdisk cavities using focused ion beam technology (Figure 7D). All above research has observed laser mode regulation in microdisk cavities. Our group (Zhu G. Y. et al., 2020) realized quasi-single-mode ultraviolet WGM lasing from microchimney cavities under optical pumping (Figure 7F). Lasing spectra in Figure 7G imply single-mode resonance of approximately 372 nm. Compared to other works, the lasing of microchimney cavities directs light along the cavity and obtains a spiral path of light (Figures 7H–J). In our other works (Zhu et al., 2018), a grating structure was introduced to floating GaN microdisks. Single-mode lasing (Figures 7B,C,K) was realized in this structure. With increasing pumping power, lasing resonance appeared at approximately 379.25 nm. Based on electrical field distribution, WGM lasing was confirmed; this study is similar to Wang’s work (Wang et al., 2018). Although laser mode regulation can be achieved, the above methods may introduce damage to the microcavity. It may reduce the quality of the microcavity laser and increase the laser threshold. The effect of large-scale changes in alignment indexing caused by small changes in the measurement value is defined as the Vernier effect (Wang Y. Y et al., 2016; Liu et al., 2021). It is also applied in optical systems. The FSR can be controlled by adjusting the size of microcavity. A common mode can be selected by using two devices with similar spectra. With the Vernier effect, the lasing can maintain low laser threshold and high Q value. By designing the coupling cavity and using the Vernier effect, mode selection can be realized while improving laser quality. Xu et al. (2012) achieved a single-mode laser by coupling two GaN microrods near each other. A single microcavity produced a multi-mode laser, while the coupling of two cavities produced a single-mode laser. This lasing mode engineering can even be generalized to dynamic mode regulation (Yang et al., 2018; Peng et al., 2019; Qin et al., 2021b).
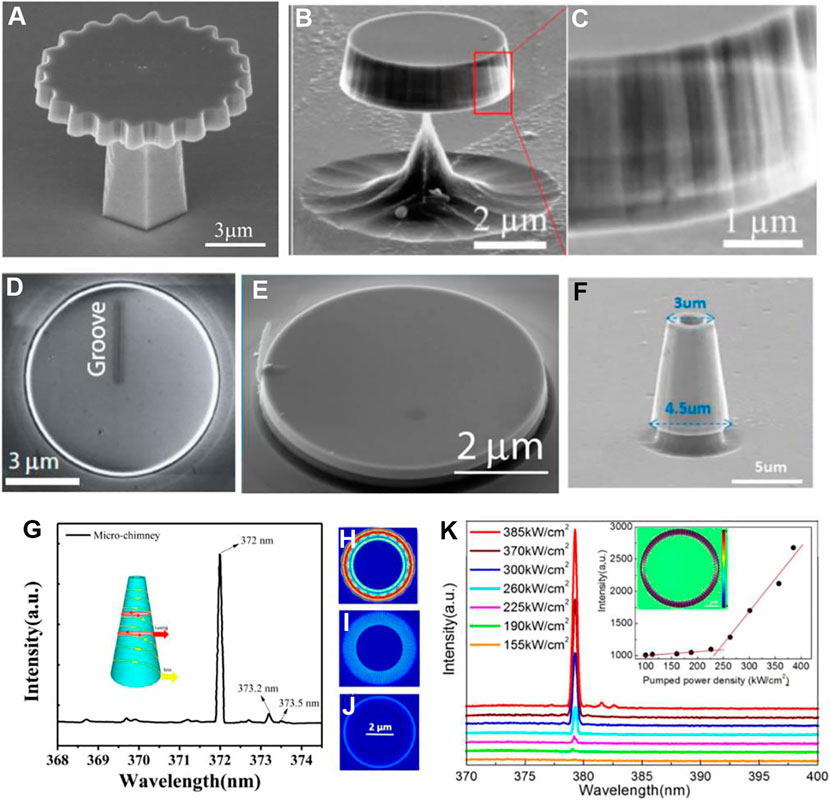
FIGURE 7. (A) Microdisk with a saw tooth structure for a GaInAsP–InP microdisk (Fujita and Baba, 2002). SEM images of the GaN microdisk with a (B,C) grating along the side (Zhu et al., 2018), (D) slit (Bogdanov et al., 2015), and (E) Pt antenna structure (Moiseev et al., 2017). (F) SEM images, (G) lasing spectra, and (H–J) optical field distribution of the microchimney cavity (Zhu G. Y. et al., 2020). (K) Power-dependent lasing spectra of the GaN microdisk (Zhu et al., 2018). Reprinted with permission© AIP Publishing (Fujita and Baba, 2002),© Optical Society of America (Bogdanov et al., 2015; Zhu et al., 2018),© ACS Publishing (Moiseev al., 2017),© Elsevier Publishing (Zhu G. Y. et al., 2020).
Electrically Driven GaN Laser Diodes
Owing to their advantages in wide-emission ranging from UV to near-infrared (IR) and direct band gap, GaN materials have been widely used for high-efficiency light-emitting diodes (LEDs) and LDs (Strawbridge et al., 2011; Lu et al., 2014; Ding et al., 2021; Jmerik et al., 2021; Yulianto et al., 2021). III-Nitride LDs have been widely used in displays, lighting, and optical storage and have shown considerable potential for applications in monolithic integration, visible light communication, optical clocks, material processing, quantum technology, and medical instruments. GaN lasers can be fabricated on GaN, sapphire, SiC, or Si substrates (Lee et al., 2017; Sun et al., 2018). Most contemporary GaN-based LDs are produced on two-inch free-standing (FS) GaN substrates (∼$4,000/pc), because of which the LD chip costs 2–3 orders of magnitude higher than LEDs grown on Si or sapphire substrates. The most recent research results indicate that the III-nitride semiconductor laser directly grown on Si is a potential on-chip light source for Si photonics. Moreover, it may greatly lower the manufacture cost of laser diodes and further expand their applications. Due to its low cost, large volume, low resistivity, and high thermal conductivity, the GaN-on-Si substrate has become a popular research topic in recent years (Bao, 2017; Jiang et al., 2017).
A typical layer structure of GaN-based LDs on Si substrates is seen in Figure 8A (Feng et al., 2021). It presents InGaN/GaN MQWs and optical cladding layers. For poor cavity quality, it presented normal spontaneous radiation (Figure 8D) (Mei et al., 2021). Violet, blue, and near-UV LDs were realized in structures with better-quality cavities (Zhang et al., 2003; Christ et al., 2004; Roehrens et al., 2010). Popular research groups in this field are Sun Qian’s research group of the Semiconductor Institute of Chinese Academy of Sciences and the SINANO research group. Feng et al. (2021) used a “sandwich-like” architecture with upper and lower AlGaN cladding layers to design lasing structures (Figure 8B). They demonstrated confinement of the optical field in InGaN-based microdisk lasers grown on Si substrates. Lasing resonance was observed under a 250 mA-driven current threshold (Figures 9C,D). To further reduce the optical loss, Zhu et al. (2020b) designed and fabricated a perovskite-coated GaN microwheel structure (Figure 8C). This device exhibited two emission peaks near 438 and 512 nm (Figures 9A,B). However, only spontaneous radiation in the blue range was observed. Recently, Wang et al. (2020) fabricated GaN microdisks with diameter = 10 μm. Lasing resonance with a high Q factor was realized under current driven <18 mA (Figures 8E,F).
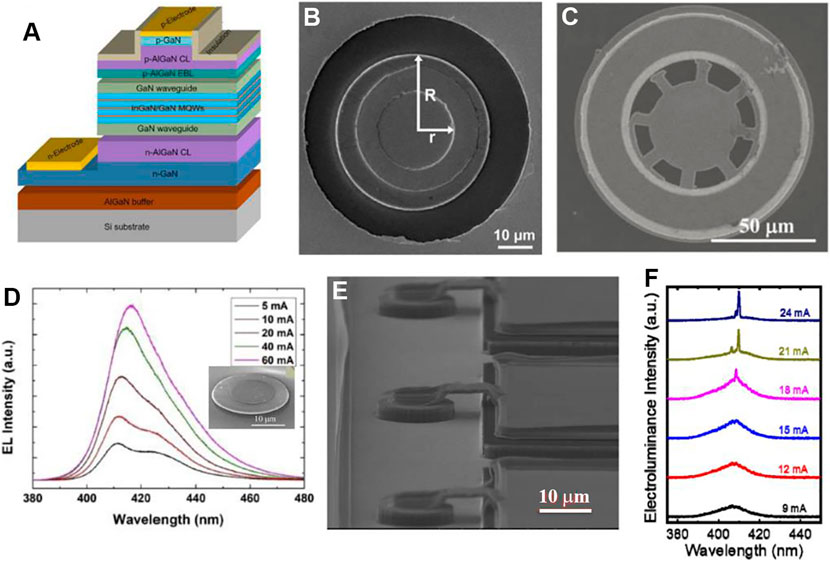
FIGURE 8. (A) Typical layer structure of the GaN-based laser diode (LD) on an Si substrate. (B,E) SEM images of the GaN microdisk for electrically driven lasing (Wang et al., 2020; Feng et al., 2021) and (C) GaN microwheel for LED (Zhu et al., 2020b). EL spectra of the (D) GaN microdisk (Mei et al., 2021) and (F) microdisk laser (Wang et al., 2020). Reprinted with permission© Elsevier Publishing (Feng et al., 2021),© IOP Publishing (Zhu et al., 2019).
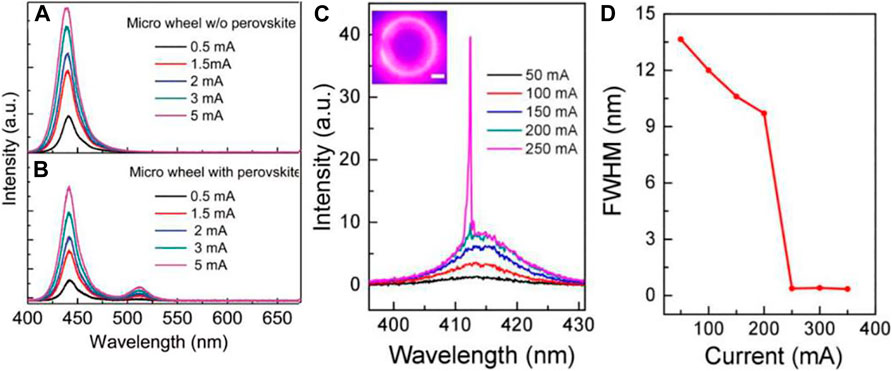
FIGURE 9. EL spectra of a GaN microwheel (A) without and (B) with CH3NH3PbBr3 at varying currents (Zhu et al., 2020b). (C) EL spectra of an as-fabricated InGaN-based microring laser grown on an Si substrate (Feng et al., 2021). (D) Relationship between the FWHM of EL spectra and the pulsed injection current in Figure 9C. Reprinted with permission© Elsevier Publishing (Feng et al., 2021),© IOP Publishing (Zhu et al., 2019).
Optoelectronic Chip Integration
Optoelectronic integration technologies compatible with large-scale low-cost silicon electronics are considered promising approaches to overcome the speed and bandwidth limitations of communication and computing technologies (Moerman et al., 1997; Hao et al., 2021). However, the drawbacks of Si-based materials, such as narrow and indirect band gaps, are not conducive to optoelectronic devices with gain. To overcome this, silicon-based GaN has been used to fabricate integrated devices (Shih et al., 2005; Ogihara et al., 2008; Pham et al., 2013). Compared with Si, nitride compound semiconductors (AlGaN or InGaN) have tunable and direct optical band gaps, and these are compatible with traditional micromachining processes. Hence, coupling WGM microdisk lasers with waveguides can enable the monolithic integration of GaN microdisks or microrings with other structures (Koseki et al., 2009). Witzens et al. (2005) realized the monolithic integration of vertical-cavity surface-emitting lasers with in-plane waveguides. Tabataba-Vakili et al. (2019) demonstrated a critical coupling structure on an active GaN microdisk laser with bus waveguide on an Si substrate (Figures 10A,B). Lasing parameters, such as thresholds and Q factor, can be controlled by varying the coupling distance. As shown in Figure 10B, the resonance mode in the range of 410–450 nm was obtained. The evanescent tail of resonance in GaN microdisks is coupled by the bus waveguide on the side, and transmission through the waveguide can be detected in the back of the waveguide. According to Rasoloniaina’s reports (Rasoloniaina et al., 2014) and (Spillane et al., 2003) shown in Figures 10C,D, the resonance mode is coupled with different coupling distances and can be quantized. The transmission through the waveguide can be explained based on the gap between the microdisk and the waveguide.
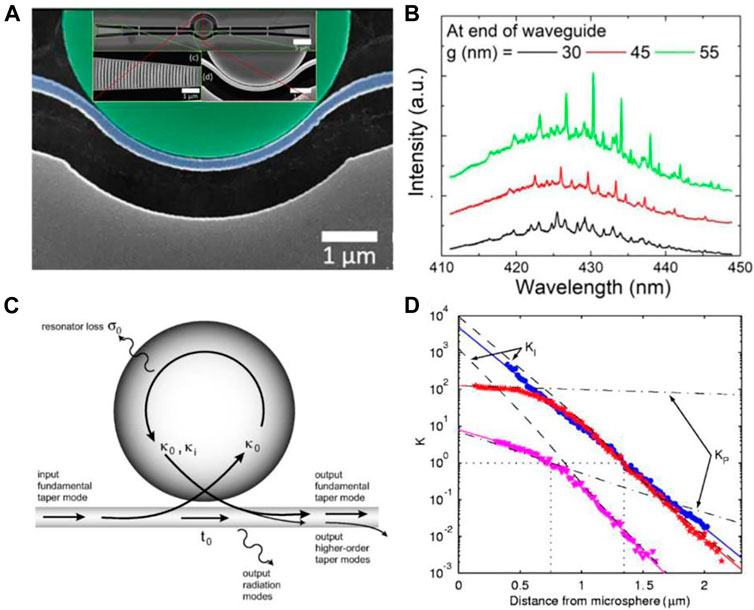
FIGURE 10. (A) Coupling structure in an active GaN microdisk laser with bus waveguide on an Si substrate. (B) Lasing spectra of coupled structures with different coupling distances (Tabataba-Vakili et al., 2019). (C) Schematic diagram of the waveguide coupled with a microcavity. (D) Relationship between the coupling distance and the coupling coefficient (Spillane et al., 2003). Reprinted with permission© APS Physic Publishing (Spillane et al., 2003).
By combining optically pumped GaN laser structures and chip-integrated LED or LD structures, waveguide and photoelectric detectors on the Si substrate have been studied and used in high-speed communication (Schinkel et al., 2009; Li X et al., 2015; Wang Y et al., 2016; Tanaka et al., 2017). As shown in Figure 11, Feng et al. (2018) fabricated on-chip-integrated GaN-based lasers, modulators, and photodetectors grown on Si substrates (Figures 11A,B). A multi-quantum well structure with 290 μm (LD region), 190 μm (modulation region), and 790 μm (PD region) was designed and fabricated. EL with an FWHM of 1 nm and peak position of 412.8 nm was realized in this study. The photocurrent in the PD is presented in Figure 11C and modulated basis the bias voltage.
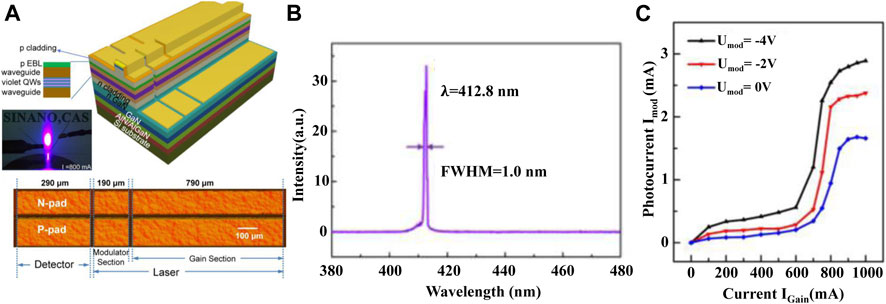
FIGURE 11. (A) Schematic diagram of integrated devices; (B) lasing spectra of GaN LDs; (C) photocurrent response curves at different bias voltages (Feng et al., 2018). Reprinted with permission© IEEE Xplore Publishing (Feng et al., 2018).
Summary and Prospect
In this study, we review recent advances in the realization of GaN microstructures, observations of WGM lasing, and the corresponding lasing mode engineering. The WGM lasing mechanism is summarized as the total reflection of the inner wall. The Q factor, mode volume, FSR, and threshold value are important parameters to evaluate the quality of microcavity. GaN microdisks are prepared using two types of methods, namely, direct growth using CVD or MBE and fabrication using photolithography followed by wet and dry etching. Optically pumped lasing has been obtained in GaN microstructures such as microdisks or microrings. Engineering of lasing properties such as mode number tailoring or emission direction controlling is well studied. Methods such as introduction of holes, corners, metal antennas, and slits are used to control the emission direction, and special structures such as grating or saw tooth are introduced to tailor the lasing mode. Decreasing the thickness of the cavity and improving the sidewall roughness are key issues to optimize the Q factor or threshold value of the cavity. The aim of studying optically pumped WGM lasing is to obtain electrically injected lasing. By decreasing the gain region, floating the cavity, or improving the surface condition, an electrically injected WGM laser can be realized for a cavity with diameter ≤10 μm. Further research challenges in GaN microdisk lasing are the fabrication of microstructures with smooth surfaces and steep side walls. In addition, the design and preparation of special microstructures for achieving high-performance electrically pumped lasers and integrated device design are also popular topics for future research.
Author Contributions
GZ gave the article structure and organized the manuscript. FG, YS, MT, and BJ summarized the work. FQ edits the manuscript. XL and YW gave some comments on the content and structure of the manuscript.
Funding
This work was supported by the Research Start-Up fund (NY219147, NY220181), the Foundation of Jiangsu Provincial Double-Innovation Doctor Program grant (CZ002SC20013), the Jiangsu Youth Fund (BK20210593), the National Natural Science Foundation of China (61704024), and the project supported by the State Key Laboratory of Luminescence and Applications (SKLA-2021-04).
Conflict of Interest
The authors declare that the research was conducted in the absence of any commercial or financial relationships that could be construed as a potential conflict of interest.
Publisher’s Note
All claims expressed in this article are solely those of the authors and do not necessarily represent those of their affiliated organizations, or those of the publisher, the editors, and the reviewers. Any product that may be evaluated in this article, or claim that may be made by its manufacturer, is not guaranteed or endorsed by the publisher.
Acknowledgments
The authors would like to thank Doctor X.X.Wang and R. Wang of Southeast University for their help in literature search.
References
Aharonovich, I., Woolf, A., Russell, K. J., Zhu, T., Niu, N., Kappers, M. J., et al. (2013). Low Threshold, Room-Temperature Microdisk Lasers in the Blue Spectral Range. Appl. Phys. Lett. 103, 021112–021113. doi:10.1063/1.4813471
Bao, A. (2017). Group III-Nitride Nanowires. Mater. Sci. Technol. 33, 765–776. doi:10.1080/02670836.2016.1192765
Ben-Messaoud, T., and Zyss, J. (2005). Unidirectional Laser Emission from Polymer-Based Spiral Microdisks. Appl. Phys. Lett. 86, 241110. doi:10.1063/1.1949708
Bogdanov, A. A., Mukhin, I. S., Kryzhanovskaya, N. V., Maximov, M. V., Sadrieva, Z. F., Kulagina, M. M., et al. (2015). Mode Selection in InAs Quantum Dot Microdisk Lasers Using Focused Ion Beam Technique. Opt. Lett. 40, 4022–4025. doi:10.1364/ol.40.004022
Bogusławski, J., Wang, Y., Xue, H., Yang, X., Mao, D., Gan, X., et al. (2018). Graphene Actively Mode-Locked Lasers. Adv. Fun. Mater. 28, 1801539. doi:10.1002/adfm.201801539
Borselli, M., Johnson, T. J., and Painter, O. (2005). Beyond the Rayleigh Scattering Limit in High-Q Silicon Microdisks: Theory and experiment. Opt. Express 13, 1515–1530. doi:10.1364/opex.13.001515
Cai, X., Wang, J., Strain, M. J., Johnson-Morris, B., Zhu, J., Sorel, M., et al. (2012). Integrated Compact Optical Vortex Beam Emitters. Science 338, 363–366. doi:10.1126/science.1226528
Chen, J., and Wang, J. (2007). Comparison between HIE-FDTD Method and ADI-FDTD Method. Microw. Opt. Technol. Lett. 49, 1001–1005. doi:10.1002/mop.22340
Chen, Y., Yin, Y., Ma, L., and Schmidt, O. G. (2021). Recent Progress on Optoplasmonic Whispering‐Gallery‐Mode Microcavities. Adv. Opt. Mater. 9, 2100143–2100158. doi:10.1002/adom.202100143
Chen, H., Guo, K., Yin, J., He, S., Qiu, G., Zhang, M., et al. (2021). Photoluminescence-Induced Four-Wave Mixing Generation in a Monolayer-MoS2-Cladded GaN Microdisk Resonator. Laserphotonics Rev. 15, 2000459. doi:10.1002/lpor.202000459
Choi, J. H., Zoulkarneev, A., Kim, S. I., Baik, C. W., Yang, M. H., Park, S. S., et al. (2011). Nearly Single-Crystalline GaN Light-Emitting Diodes on Amorphous Glass Substrates. Nat. Photon 5, 763–769. doi:10.1038/nphoton.2011.253
Christ, A., Zentgraf, T., Kuhl, J., Tikhodeev, S. G., Gippius, N. A., and Giessen, H. (2004). Optical Properties of Planar Metallic Photonic crystal Structures: Experiment and Theory. Phys. Rev. B 70, 1–5. doi:10.1103/physrevb.70.125113
Chung, K., Lee, C.-H., and Yi, G.-C. (2010). Transferable GaN Layers Grown on ZnO-Coated Graphene Layers for Optoelectronic Devices. Science 330, 655–657. doi:10.1126/science.1195403
Ding, B., Frentrup, M., Fairclough, S. M., Kusch, G., Kappers, M. J., Wallis, D. J., et al. (2021). Multimicroscopy of Cross-Section Zincblende GaN LED Heterostructure. J. Appl. Phys. 130, 115705. doi:10.1063/5.0058429
Dong, H., Zhang, C., Lin, X., Zhou, Z., Yao, J., and Zhao, Y. S. (2017). Dual-Wavelength Switchable Vibronic Lasing in Single-Crystal Organic Microdisks. Nano Lett. 17, 91–96. doi:10.1021/acs.nanolett.6b03499
Feng, L., Wong, Z. J., Ma, R.-M., Wang, Y., and Zhang, X. (2014). Single-Mode Laser by Parity-Time Symmetry Breaking. Science 346, 972–975. doi:10.1126/science.1258479
Feng, M., Wang, J., Zhou, R., Sun, Q., Gao, H., Zhou, Y., et al. (2018). On-Chip Integration of GaN-Based Laser, Modulator, and Photodetector Grown on Si. IEEE J. Select. Top. Quan. Electron. 24, 1–5. doi:10.1109/jstqe.2018.2815906
Feng, M., Liu, J., Sun, Q., and Yang, H. (2021). III-Nitride Semiconductor Lasers Grown on Si. Prog. Quan. Electron. 77, 100323. doi:10.1016/j.pquantelec.2021.100323
Ferreira, R. X. G., Xie, E., McKendry, J. J. D., Rajbhandari, S., Chun, H., Faulkner, G., et al. (2016). High Bandwidth GaN-Based Micro-LEDs for Multi-Gb/s Visible Light Communications. IEEE Photon. Technol. Lett. 28, 2023–2026. doi:10.1109/lpt.2016.2581318
Fujita, M., and Baba, T. (2002). Microgear Laser. Appl. Phys. Lett. 80, 2051–2053. doi:10.1063/1.1462867
Guo, B., Xiao, Q. l., Wang, S. h., and Zhang, H. (2019). 2D Layered Materials: Synthesis, Nonlinear Optical Properties, and Device Applications. Laser Photon. Rev. 13, 1800327. doi:10.1002/lpor.201800327
Hao, Y., Xiang, S., Han, G., Zhang, J., Ma, X., Zhu, Z., et al. (2021). Recent Progress of Integrated Circuits and Optoelectronic Chips. Sci. China Inf. Sci. 64, 201401–201433. doi:10.1007/s11432-021-3235-7
Higashiwaki, M., Sasaki, K., Kuramata, A., Masui, T., and Yamakoshi, S. (2014). Development of Gallium Oxide Power Devices. Phys. Status Solidi A. 211, 21–26. doi:10.1002/pssa.201330197
Hill, M. T., and Gather, M. C. (2014). Advances in Small Lasers. Nat. Photon 8, 908–918. doi:10.1038/nphoton.2014.239
Irmer, G., Röder, C., Himcinschi, C., and Kortus, J. (2016). Nonlinear Optical Coefficients of Wurtzite-Typeα-GaN Determined by Raman Spectroscopy. Phys. Rev. B 94, 195201–195209. doi:10.1103/physrevb.94.195201
Jiang, X.-F., Xiao, Y.-F., Zou, C.-L., He, L., Dong, C.-H., Li, B.-B., et al. (2012). Highly Unidirectional Emission and Ultralow-Threshold Lasing from On-Chip Ultrahigh-Q Microcavities. Adv. Mater. 24, OP260–OP264. doi:10.1002/adma.201201229
Jiang, X.-F., Zou, C.-L., Wang, L., Gong, Q., and Xiao, Y.-F. (2016). Whispering-Gallery Microcavities with Unidirectional Laser Emission. Laser Photon. Rev. 10, 40–61. doi:10.1002/lpor.201500163
Jiang, M., He, G., Chen, H., Zhang, Z., Zheng, L., Shan, C., et al. (2017). Wavelength-Tunable Electroluminescent Light Sources from Individual Ga-Doped ZnO Microwires. Small 13 (19), 70105. doi:10.1002/smll.201770105
Jiang, J. A., Xu, H., Sheikhi, M., Li, L., Yang, Z., Hoo, J., et al. (2019). Omnidirectional Whispering-Gallery-Mode Lasing in GaN Microdisk Obtained by Selective Area Growth on Sapphire Substrate. Opt. Express 27, 16195–16205. doi:10.1364/oe.27.016195
Jiang, X., Qavi, A. J., Huang, S. H., and Yang, L. (2020). Whispering-Gallery Sensors. Matter 3, 371–392. doi:10.1016/j.matt.2020.07.008
Jmerik, V., Toropov, A., Davydov, V., and Ivanov, S. (2021). Monolayer‐Thick GaN/AlN Multilayer Heterostructures for Deep‐Ultraviolet Optoelectronics. Physica Status Solidi (RRL) – Rapid Res. Lett. 15, 2100242. doi:10.1002/pssr.202100242
Kang, T. W., Park, S. H., Song, H., Kim, T. W., Yoon, G. S., and Kim, C. O. (1998). Mechanism of Donor-Acceptor Pair Recombination in Mg-Doped GaN Epilayers Grown on Sapphire Substrates. J. Appl. Phys. 84, 2082–2085. doi:10.1063/1.368269
Khurgin, J. B., and Noginov, M. A. (2021). How Do the Purcell Factor, the Q ‐Factor, and the Beta Factor Affect the Laser Threshold? Laser Photon. Rev. 15, 2000250. doi:10.1002/lpor.202000250
Knapper, K. A., Heylman, K. D., Horak, E. H., and Goldsmith, R. H. (2016). Chip-Scale Fabrication of High- Q All-Glass Toroidal Microresonators for Single-Particle Label-Free Imaging. Adv. Mater. 28, 2945–2950. doi:10.1002/adma.201504976
Koseki, S., Zhang, B., De Greve, K., and Yamamoto, Y. (2009). Monolithic Integration of Quantum Dot Containing Microdisk Microcavities Coupled to Air-Suspended Waveguides. Appl. Phys. Lett. 94, 051110. doi:10.1063/1.3078522
Kwon, O. D., Yoon, J. H., Kim, D. K., Kim, Y. C., Lee, S. E., and Kim, S. S. (2008). “Mega-pixel PQR Laser Chips for Interconnect, Display ITS, and Biocell-Tweezers OEIC,” in Proceedings of the SPIE, San Jose, CA, February 12, 2008.
Lai, Y., Wang, D., Kong, Q., Luo, X., Tang, J., Liu, R., et al. (2021). Free-Standing Carbon-Doped Semi-Insulating GaN Wafer Grown by HVPE. J. Cryst. Growth 573, 126216. doi:10.1016/j.jcrysgro.2021.126216
Lee, M., Mikulik, D., Yang, M., and Park, S. (2017). The Investigation of Stress in Freestanding GaN Crystals Grown from Si Substrates by HVPE. Sci. Rep. 7, 8587. doi:10.1038/s41598-017-08905-y
Li, S., and Waag, A. (2012). GaN Based Nanorods for Solid State Lighting. J. Appl. Phys. 111, 071101. doi:10.1063/1.3694674
Li, K. H., Cheung, Y. F., and Choi, H. W. (2015). Whispering Gallery Mode Lasing in Optically Isolated III-Nitride Nanorings. Opt. Lett. 40, 2564–2567. doi:10.1364/ol.40.002564
Li, X., Zhu, G., Gao, X., Bai, D., Huang, X., Cao, X., et al. (2015). Suspended P-N junction InGaN/GaN Multiple-Quantum-Well Device with Selectable Functionality. IEEE Photonic. J. 7, 2701407–2701415. doi:10.1109/jphot.2015.2499544
Li, Y., Feng, L., Su, X., Li, Q., Yun, F., Yuan, G., et al. (2017). Whispering Gallery Mode Lasing from InGaN/GaN Quantum Well Microtube. Opt. Express 25, 18072–18080. doi:10.1364/oe.25.018072
Li, Y., Feng, L., Li, F., Hu, P., Du, M., Su, X., et al. (2018). Three-Dimensional Anisotropic Microlaser from GaN-Based Self-Bent-Up Microdisk. Acs Photon. 5, 4259–4264. doi:10.1021/acsphotonics.8b01061
Liu, Y. C., Xiao, Y. F., Jiang, X.-F., Li, B. B., Li, Y., and Gong, Q. (2012). Cavity-QED Treatment of Scattering-Induced Free-Space Excitation and Collection in High-Q Whispering-Gallery Microcavities. Phys. Rev. A. 85, 013843. doi:10.1103/physreva.85.013843
Liu, X., Bruch, A. W., Lu, J., Gong, Z., Surya, J. B., Zhang, L., et al. (2019). Beyond 100 THz-Spanning Ultraviolet Frequency combs in a Non-Centrosymmetric Crystalline Waveguide. Nat. Commun. 10, 2971. doi:10.1038/s41467-019-11034-x
Liu, Y., Li, X., Zhang, Y.-N., and Zhao, Y. (2021). Fiber-Optic Sensors Based on Vernier Effect. Measurement 167, 108451. doi:10.1016/j.measurement.2020.108451
Lu, J., Xu, C., Dai, J., Li, J., Wang, Y., Lin, Y., et al. (2014). Plasmon-Enhanced Whispering Gallery Mode Lasing from Hexagonal Al/ZnO Microcavity. ACS Photon. 2, 73–77. doi:10.1021/ph5002582
Luo, Y., Luo, X.-Q., Yi, J., Ou, J., Zhu, W., Chen, Z., et al. (2021). Whispering-Gallery Mode Resonance-Assisted Plasmonic Sensing and Switching in Subwavelength Nanostructures. J. Mater. Sci. 56, 4716–4726. doi:10.1007/s10853-020-05581-8
Matsko, A. B., and Ilchenko, V. S. (2006). Optical Resonators with Whispering-Gallery Modes-Part I: Basics. IEEE J. Select. Top. Quan. Electron. 12, 3–14. doi:10.1109/jstqe.2005.862952
Mei, Y., Xie, M., Xu, H., Long, H., Ying, L., and Zhang, B. (2021). Electrically Injected GaN-Based Microdisk towards an Efficient Whispering Gallery Mode Laser. Opt. Express 29, 5598–5606. doi:10.1364/oe.416873
Miao, P., Zhang, Z., Sun, J., Walasik, W., Longhi, S., Litchinitser, N. M., et al. (2016). Orbital Angular Momentum Microlaser. Science 353, 464–467. doi:10.1126/science.aaf8533
Michler, P., Kiraz, A., Zhang, L., Becher, C., Hu, E., and Imamoglu, A. (2000). Laser Emission from Quantum Dots in Microdisk Structures. Appl. Phys. Lett. 77, 184–186. doi:10.1063/1.126918
Miller, D. (2009). Device Requirements for Optical Interconnects to Silicon Chips. Proc. IEEE 97, 1166–1185. doi:10.1109/jproc.2009.2014298
Moerman, I., Van Daele, P. P., and Demeester, P. M. (1997). A Review on Fabrication Technologies for the Monolithic Integration of Tapers with III-V Semiconductor Devices. IEEE J. Select. Top. Quan. Electron. 3, 1308–1320. doi:10.1109/2944.658785
Moiseev, E. I., Kryzhanovskaya, N., Polubavkina, Y. S., Maximov, M. V., Kulagina, M. M., Zadiranov, Y. M., et al. (2017). Light Outcoupling from Quantum Dot-Based Microdisk Laser via Plasmonic Nanoantenna. Acs Photon. 4, 275–281. doi:10.1021/acsphotonics.6b00552
Nakajima, H., Hamaguchi, T., Tanaka, M., Ito, M., Jyokawa, T., Matou, T., et al. (2019). Single Transverse Mode Operation of GaN-based Vertical-Cavity Surface-Emitting Laser with Monolithically Incorporated Curved Mirror. Appl. Phys. Express 12, 084003. doi:10.7567/1882-0786/ab3106
Nosich, A. I., Smotrova, E. I., Boriskina, S. V., Benson, T. M., and Sewell, P. (2007). Trends in Microdisk Laser Research and Linear Optical Modelling. Opt. Quant Electron. 39, 1253–1272. doi:10.1007/s11082-008-9203-z
Ogihara, M., Sagimori, T., Mutoh, M., Furuta, H., Suzuki, T., Fujiwara, H., et al. (2008). “Single-Crystal Thin-Film Bonding on Diamond-Like Carbon Film by Intermolecular Force for Super High-Density Integration of High-Power LEDs,” in IEEE International Electron Devices Meeting, San Francisco, CA, December 15–17, 2008 (IEEE), 477–480.
Peng, Y., Lu, J., Peng, D., Ma, W., Li, F., Chen, Q., et al. (2019). Dynamically Modulated GaN Whispering Gallery Lasing Mode for Strain Sensor. Adv. Funct. Mater. 29, 1905051–1905059. doi:10.1002/adfm.201905051
Peng, L., Liu, S., Yang, J., Zhao, D., Liang, F., Chen, P., et al. (2021). The Influence of Residual GaN on Two-Step-Grown GaN on Sapphire. Mater. Sci. Semiconductor Process. 135, 105903. doi:10.1016/j.mssp.2021.105903
Pham, N. P., Rosmeulen, M., Li, Z., Sabuncuoglu, D., and Osman, H. (2013). “Substrate transfer for GaN-based LEDs on 200 mm Si,” in 2013 IEEE 15th Electronics Packaging Technology Conference (EPTC 2013), Singapore, December 11-13, 2013 (IEEE), 324–328.
Puchtler, T. J., Woolf, A., Zhu, T., Gachet, D., Hu, E. L., and Oliver, R. A. (2015). Effect of Threading Dislocations on the Quality Factor of InGaN/GaN Microdisk Cavities. ACS Photon. 2, 137–143. doi:10.1021/ph500426g
Qin, F., Zhu, Q., Zhang, Y., Wang, R., Wang, X., Zhou, M., et al. (2021). Effect of Substrates on Lasing Properties of GaN Transferable Membranes. Opt. Mater. 122, 111663. doi:10.1016/j.optmat.2021.111663
Qin, F., Zhu, G., Wang, R., Wang, X., Lu, J., Yang, Y., et al. (2021). Thermal Effect Induced Dynamically Lasing Mode Tuning in GaN Whispering Gallery Microcavities. J. Phys. D: Appl. Phys. 54, 255103–325512. doi:10.1088/1361-6463/abefbb
Rahmani, M., and Jagadish, C. (2018). Light-Matter Interactions on the Nanoscale. Beilstein J. Nanotechnol. 9, 2125–2127. doi:10.3762/bjnano.9.201
Rasoloniaina, A., Huet, V., Nguyên, T. K. N., Le Cren, E., Mortier, M., Michely, L., et al. (2014). Controling the Coupling Properties of Active Ultrahigh-Q WGM Microcavities from Undercoupling to Selective Amplification. Sci. Rep. 4, 4023. doi:10.1038/srep04023
Rex, N. B., Chang, R. K., and Guido, L. J. (2001). Threshold Lowering in GaN Micropillar Lasers by Means of Spatially Selective Optical Pumping. IEEE Photon. Technol. Lett. 13, 1–3. doi:10.1109/68.903201
Robinson, J. T., Manolatou, C., Chen, L., and Lipson, M. (2005). Ultrasmall Mode Volumes in Dielectric Optical Microcavities. Phys. Rev. Lett. 95, 143901–214905. doi:10.1103/physrevlett.95.143901
Roehrens, D., Brendt, J., Samuelis, D., and Martin, M. (2010). On the Ammonolysis of Ga2O3: An XRD, Neutron Diffraction and XAS Investigation of the Oxygen-Rich Part of the System Ga2O3-GaN. J. Solid State. Chem. 183, 532–541. doi:10.1016/j.jssc.2009.12.024
Rupprecht, C., Lundt, N., Wurdack, M., Stepanov, P., Estrecho, E., Richard, M., et al. (2021). Micro-Mechanical Assembly and Characterization of High-Quality Fabry-Pérot Microcavities for the Integration of Two-Dimensional Materials. Appl. Phys. Lett. 118, 103103. doi:10.1063/5.0034851
Schinkel, D., Mensink, E., Klumperink, E., van Tuijl, E., and Nauta, B. (2009). Low-Power, High-Speed Transceivers for Network-On-Chip Communication. IEEE Trans. VLSI Syst. 17, 12–21. doi:10.1109/tvlsi.2008.2001949
Sellés, J., Brimont, C., Cassabois, G., Valvin, P., Guillet, T., Roland, I., et al. (2016). Deep-UV Nitride-On-Silicon Microdisk Lasers. Sci. Rep. 6, 21650. doi:10.1038/srep21650
Seo, S. S. A., Kim, M. W., Lee, Y. S., Noh, T. W., Park, Y. D., Thaler, G. T., et al. (2003). Observation of Sphere Resonance Peak in Ferromagnetic GaN:Mn. Appl. Phys. Lett. 82, 4749–4751. doi:10.1063/1.1588741
Seredin, P. V., Goloshchapov, D. L., Arsentyev, I. N., Sharofidinov, S., Kasatkin, I. A., and Prutskij, T. (2021). HVPE Fabrication of GaN Sub-Micro Pillars on Preliminarily Treated Si(001) Substrate. Opt. Mater. 117, 111130. doi:10.1016/j.optmat.2021.111130
Shi, L., Wang, Q., Jiang, L., Gao, Q., and Zhu, T. (2021). In-Fiber Butt-Coupled Spherical Microcavity with Whispering Gallery Mode and Fabry-Perot Resonances. IEEE Photon. Technol. Lett. 33, 553–556. doi:10.1109/lpt.2021.3075970
Shih, C.-F., Chen, N.-C., Chang, C.-A., and Liu, K.-S. (2005). Blue, Green and White InGaN Light-Emitting Diodes Grown on Si. Jpn. J. Appl. Phys. 44, L140–L143. doi:10.1143/jjap.44.l140
Simeonov, D., Feltin, E., Altoukhov, A., Castiglia, A., Carlin, J.-F., Butté, R., et al. (2008). High Quality Nitride Based Microdisks Obtained via Selective Wet Etching of AlInN Sacrificial Layers. Appl. Phys. Lett. 92, 171102–171106. doi:10.1063/1.2917452
Skromme, B. J., Jayapalan, J., Vaudo, R. P., and Phanse, V. M. (1999). Low-Temperature Luminescence of Exciton and Defect States in Heteroepitaxial GaN Grown by Hydride Vapor Phase Epitaxy. Appl. Phys. Lett. 74, 2358–2360. doi:10.1063/1.123850
Smotrova, E. I., Nosich, A. I., Benson, T. M., and Sewell, P. (2005). Cold-Cavity Thresholds of Microdisks with Uniform and Nonuniform Gain: Quasi-3-D Modeling with Accurate 2-D Analysis. IEEE J. Select. Top. Quan. Electron. 11, 1135–1142. doi:10.1109/jstqe.2005.853848
Spillane, S. M., Kippenberg, T. J., Painter, O. J., and Vahala, K. J. (2003). Ideality in a Fiber-Taper-Coupled Microresonator System for Application to Cavity Quantum Electrodynamics. Phys. Rev. Lett. 91, 043902. doi:10.1103/PhysRevLett.91.043902
Srinivasan, K., Borselli, M., Painter, O., Stintz, A., and Krishna, S. (2006). Cavity Q, Mode Volume, and Lasing Threshold in Small Diameter AlGaAs Microdisks with Embedded Quantum Dots. Opt. Express 14, 1094–1105. doi:10.1364/oe.14.001094
Stock, E., Albert, F., Hopfmann, C., Lermer, M., Schneider, C., Höfling, S., et al. (2013). On-Chip Quantum Optics with Quantum Dot Microcavities. Adv. Mater. 25, 707–710. doi:10.1002/adma.201202778
Strawbridge, B., Cernetic, N., Chapley, J., Singh, R. K., Mahajan, S., and Newman, N. (2011). Influence of Surface Topography Onin Situreflection Electron Energy Loss Spectroscopy Plasmon Spectra of AlN, GaN, and InN Semiconductors. J.Vac. Sci.Technol. A. 29, 041602. doi:10.1116/1.3584775
Streiff, M., Witzig, A., Pfeiffer, M., Royo, P., and Fichtner, W. (2003). A Comprehensive VCSEL Device Simulator. IEEE J. Select. Top. Quan. Electron. 9, 879–891. doi:10.1109/jstqe.2003.818858
Sumetsky, M. (2010). Mode Localization and the Q-Factor of a Cylindrical Microresonator. Opt. Lett. 35, 2385–2387. doi:10.1364/ol.35.002385
Sun, Y., Zhou, K., Feng, M., Li, Z., Zhou, Y., Sun, Q., et al. (2018). Room-Temperature Continuous-Wave Electrically Pumped InGaN/GaN Quantum Well Blue Laser Diode Directly Grown on Si. Light. Sci. Appl. 7, 13. doi:10.1038/s41377-018-0008-y
Tabataba-Vakili, F., Doyennette, L., Brimont, C., Guillet, T., Rennesson, S., Frayssinet, E., et al. (2018). Blue Microlasers Integrated on a Photonic Platform on Silicon. ACS Photon. 5, 3643–3648. doi:10.1021/acsphotonics.8b00542
Tabataba-Vakili, F., Doyennette, L., Brimont, C., Guillet, T., Rennesson, S., Damilano, B., et al. (2019). Demonstration of Critical Coupling in an Active III-Nitride Microdisk Photonic Circuit on Silicon. Sci. Rep. 9, 18095. doi:10.1038/s41598-019-54416-3
Tabataba-Vakili, F., Brimont, C., Alloing, B., Damilano, B., Doyennette, L., Guillet, T., et al. (2020). Analysis of Low-Threshold Optically Pumped III-Nitride Microdisk Lasers. Appl.Phys. Lett. 117, 1–11. doi:10.1063/5.0015252
Tamboli, A. C., Haberer, E. D., Sharma, R., Lee, K. H., Nakamura, S., and Hu, E. L. (2007). Room-Temperature Continuous-Wave Lasing in GaN/InGaN Microdisks. Nat. Photon 1, 61–64. doi:10.1038/nphoton.2006.52
Tamboli, A. C., Schmidt, M. C., Hirai, A., DenBaars, S. P., and Hu, E. L. (2009). Observation of Whispering Gallery Modes in Nonpolar M-Plane GaN Microdisks. Appl. Phys. Lett. 94, 251116. doi:10.1063/1.3160550
Tanaka, A., Choi, W., Chen, R., and Dayeh, S. A. (2017). Si Complies with GaN to Overcome Thermal Mismatches for the Heteroepitaxy of Thick GaN on Si. Adv. Mater. 29, 1702557. doi:10.1002/adma.201702557
Tian, X., Wang, L., Li, W., Lin, Q., and Cao, Q. (2021). Whispering Gallery Mode Lasing from Perovskite Polygonal Microcavities via Femtosecond Laser Direct Writing. ACS Appl. Mater. Inter. 13, 16952–16958. doi:10.1021/acsami.0c21824
To, C. H., Fu, W. Y., Li, K. H., Cheung, Y. F., and Choi, H. W. (2020). GaN Microdisk with Direct Coupled Waveguide for Unidirectional Whispering-Gallery Mode Emission. Opt. Lett. 45, 791–794. doi:10.1364/ol.381767
Toropov, N., Cabello, G., Serrano, M. P., Gutha, R. R., Rafti, M., and Vollmer, F. (2021). Review of Biosensing with Whispering-Gallery Mode Lasers. Light Sci. Appl. 10, 42–60. doi:10.1038/s41377-021-00471-3
Vicknesh, S., Tripathy, S., Lin, V. K. X., Wang, L. S., and Chua, S. J. (2007). Fabrication of Deeply Undercut GaN-Based Microdisk Structures on Silicon Platforms. Appl. Phys. Lett. 90, 071906. doi:10.1063/1.2472558
Wang, Q. J., Yan, C., Yu, N., Unterhinninghofen, J., Wiersig, J., Pflügl, C., et al. (2010). Whispering-Gallery Mode Resonators for Highly Unidirectional Laser Action. Proc. Natl. Acad. Sci. U.S.A. 107, 22407–22412. doi:10.1073/pnas.1015386107
Wang, Y. Y., Xu, C. X., Jiang, M. M., Li, J. T., Dai, J., Lu, J. F., et al. (2016). Lasing Mode Regulation and Single-Mode Realization in ZnO Whispering Gallery Microcavities by the Vernier Effect. Nanoscale 8, 16631–16639. doi:10.1039/c6nr04943e
Wang, Y., Zhu, G., Cai, W., Gao, X., Yang, Y., Yuan, J., et al. (2016). On-Chip Photonic System Using Suspended P-N junction InGaN/GaN Multiple Quantum Wells Device and Multiple Waveguides. Appl. Phys. Lett. 108, 162102–162107. doi:10.1063/1.4947280
Wang, Y., Ta, V. D., Leck, K. S., Tan, B. H. I., Wang, Z., He, T., et al. (2017). Robust Whispering-Gallery-Mode Microbubble Lasers from Colloidal Quantum Dots. Nano Lett. 17, 2640–2646. doi:10.1021/acs.nanolett.7b00447
Wang, D., Zhu, T., Oliver, R. A., and Hu, E. L. (2018). Ultra-Low-Threshold InGaN/GaN Quantum Dot Micro-ring Lasers. Opt. Lett. 43, 799–802. doi:10.1364/ol.43.000799
Wang, J., Feng, M., Zhou, R., Sun, Q., Liu, J., Huang, Y., et al. (2019). GaN-Based Ultraviolet Microdisk Laser Diode Grown on Si. Photon. Res. 7, B32–B36. doi:10.1364/prj.7.000b32
Wang, J., Feng, M., Zhou, R., Sun, Q., Liu, J., Sun, X., et al. (2020). Continuous-Wave Electrically Injected GaN-on-Si Microdisk Laser Diodes. Opt. Express 28, 12201–12208. doi:10.1364/oe.391851
Wang, Z., Zhu, S., Shan, X., Yuan, Z., Cui, X., and Tian, P. (2021). Full-Color Micro-LED Display Based on a Single Chip with Two Types of InGaN/GaN MQWs. Opt. Lett. 46, 4358–4361. doi:10.1364/ol.436317
Ward, J. M., Dhasmana, N., and Nic Chormaic, S. (2014). Hollow Core, Whispering Gallery Resonator Sensors. Eur. Phys. J. Spec. Top. 223, 1917–1935. doi:10.1140/epjst/e2014-02236-5
Wiersig, J., and Hentschel, M. (2006). Unidirectional Light Emission from High-Q Modes in Optical Microcavities. Phys. Revi. A. 73, 031802. doi:10.1103/physreva.73.031802
Winters, H. F., and Coburn, J. W. (1979). The Etching of Silicon with XeF2vapor. Appl. Phys. Lett. 34, 70–73. doi:10.1063/1.90562
Witzens, J., Scherer, A., Pickrell, G., Louderback, D., and Guilfoyle, P. (2005). Monolithic Integration of Vertical-Cavity Surface-Emitting Lasers with In-Plane Waveguides. Appl. Phys. Lett. 86, 101105. doi:10.1063/1.1880440
Woolf, A., Puchtler, T., Aharonovich, I., Zhu, T., Niu, N., Wang, D., et al. (2014). Distinctive Signature of Indium Gallium Nitride Quantum Dot Lasing in Microdisk Cavities. Proc. Natl. Acad. Sci. USA 111, 14042–14046. doi:10.1073/pnas.1415464111
Xia, D., Huang, L., and Zhao, L. (2019). A New Design of an MOEMS Gyroscope Based on a WGM Microdisk Resonator. Sensors 19, 2798. doi:10.3390/s19122798
Xiao, H., Liu, R., Ma, H., Lin, Z., Ma, J., Zong, F., et al. (2008). Thermal Stability of GaN Powders Investigated by XRD, XPS, PL, TEM, and FT-IR. J. Alloys Compd. 465, 340–343. doi:10.1016/j.jallcom.2007.10.084
Xiao, Z.-X., Huang, Y.-Z., Yang, Y.-D., Xiao, J.-L., and Ma, X.-W. (2017). Single-Mode Unidirectional-Emission Circular-Side Hexagonal Resonator Microlasers. Opt. Lett. 42, 1309–1312. doi:10.1364/ol.42.001309
Xu, H., Wright, J. B., Luk, T.-S., Figiel, J. J., Cross, K., Lester, L. F., et al. (2012). Single-mode Lasing of GaN Nanowire-Pairs. Appl. Phys. Lett. 101, 113106. doi:10.1063/1.4751862
Xu, X., Chen, W., Zhao, G., Li, Y., Lu, C., and Yang, L. (2018). Wireless Whispering-Gallery-Mode Sensor for thermal Sensing and Aerial Mapping. Light Sci. Appl. 7, 62. doi:10.1038/s41377-018-0063-4
Yan, C., Wang, Q. J., Diehl, L., Hentschel, M., Wiersig, J., Yu, N., et al. (2009). Directional Emission and Universal Far-Field Behavior from Semiconductor Lasers with Limaçon-Shaped Microcavity. Appl. Phys. Lett. 94, 251101. doi:10.1063/1.3153276
Yang, S., Wang, Y., and Sun, H. (2015). Advances and Prospects for Whispering Gallery Mode Microcavities. Adv. Opt. Mater. 3, 1136–1162. doi:10.1002/adom.201500232
Yang, Y., Wei, T., Zhu, R., Zong, H., Lu, J., Li, J., et al. (2018). Tunable Single-Mode Lasing in a Single Semiconductor Microrod. Opt. Express 26, 30021–30029. doi:10.1364/oe.26.030021
Yang, Y.-D., Tang, M., Wang, F.-L., Xiao, Z.-X., Xiao, J.-L., and Huang, Y.-Z. (2019). Whispering-Gallery Mode Hexagonal Micro-/Nanocavity Lasers [Invited]. Photon. Res. 7, 594–607. doi:10.1364/prj.7.000594
Yao, J., and Yang, G. (2020). 2D Material Broadband Photodetectors. Nanoscale 12, 454–476. doi:10.1039/c9nr09070c
Yonkee, B. P., Young, E. C., Lee, C., Leonard, J. T., DenBaars, S. P., Speck, J. S., et al. (2016). Demonstration of a III-Nitride Edge-Emitting Laser Diode Utilizing a GaN Tunnel junction Contact. Opt. Express 24, 7816–7822. doi:10.1364/oe.24.007816
Yulianto, N., Kadja, G. T. M., Bornemann, S., Gahlawat, S., Majid, N., Triyana, K., et al. (2021). Ultrashort Pulse Laser Lift-Off Processing of InGaN/GaN Light-Emitting Diode Chips. ACS Appl. Electron. Mater. 3, 778–788. doi:10.1021/acsaelm.0c00913
Zeng, K. C., Dai, L., Lin, J. Y., and Jiang, H. X. (1999). Optical Resonance Modes in InGaN/GaN Multiple-Quantum-Well Microring Cavities. Appl. Phys. Lett. 75, 2563–2565. doi:10.1063/1.125078
Zhan, X.-P., Ku, J.-F., Xu, Y.-X., Zhang, X.-L., Zhao, J., Fang, W., et al. (2015). Unidirectional Lasing From a Spiral-Shaped Microcavity of Dye-Doped Polymers. IEEE Photon. Technol. Lett. 27, 311–314. doi:10.1109/lpt.2014.2370641
Zhang, G. Y., Yang, Z. J., Tong, Y. Z., Qin, Z. X., Hu, X. D., Chen, Z. Z., et al. (2003). InGaN/GaN MQW High Brightness LED Grown by MOCVD. Opt. Mater. 23, 183–186. doi:10.1016/s0925-3467(03)00081-8
Zhang, Y., Ma, Z., Zhang, X., Wang, T., and Choi, H. W. (2014). Optically Pumped Whispering-Gallery Mode Lasing from 2-μm GaN Micro-disks Pivoted on Si. Appl. Phys. Lett. 104, 221106. doi:10.1063/1.4881183
Zhang, X., Cheung, Y. F., Zhang, Y., and Choi, H. W. (2014). Whispering-Gallery Mode Lasing from Optically Free-Standing InGaN Microdisks. Opt. Lett. 39, 5614–5617. doi:10.1364/ol.39.005614
Zhang, Y., Huang, J.-A., Li, K. H., Bai, D., Wang, Y., Wang, T., et al. (2016). Influence of Strain on Emission from GaN-on-Si Microdisks. J. Phys. D: Appl. Phys. 49, 375103. doi:10.1088/0022-3727/49/37/375103
Zhang, S., Li, Y., Hu, P., Li, A., Zhang, Y., Du, W., et al. (2020). Unidirectional Emission of GaN-Based Eccentric Microring Laser with Low Threshold. Opt. Express 28, 6443–6451. doi:10.1364/oe.386453
Zhang, S., Li, Y., Hu, P., Tian, Z., Li, Q., Li, A., et al. (2021). Realization of Directional Single-Mode Lasing by a GaN-based Warped Microring. Photon. Res. 9, 432–438. doi:10.1364/prj.413796
Zhizhchenko, A., Syubaev, S., Berestennikov, A., Yulin, A. V., Porfirev, A., Pushkarev, A., et al. (2019). Single-Mode Lasing from Imprinted Halide-Perovskite Microdisks. Acs Nano 13, 4140–4147. doi:10.1021/acsnano.8b08948
Zhu, G. Y., Qin, F. F., Guo, J. Y., Xu, C. X., and Wang, Y. J. (2017). Unidirectional Ultraviolet Whispering Gallery Mode Lasing from Floating Asymmetric circle GaN Microdisk. Appl. Phys. Lett. 111, 1–7. doi:10.1063/1.4991570
Zhu, G., Li, J., Li, J., Guo, J., Dai, J., Xu, C., et al. (2018). Single-Mode Ultraviolet Whispering Gallery Mode Lasing from a Floating GaN Microdisk. Opt. Lett. 43, 647–650. doi:10.1364/ol.43.000647
Zhu, G., He, S., Zhou, M., Qiu, G., Fang, M., Yuan, J., et al. (2019). Converging Lasing from Floating GaN Penrose Microcavity. EPL 127, 24001–24008. doi:10.1209/0295-5075/127/24001
Zhu, G. Y., He, S. Q., Li, J. P., Yuan, J. L., Qin, F. F., Li, J. T., et al. (2020). GaN Micro-Chimney Cavity Laser. Opt. Commun. 474 (2), 126054. doi:10.1016/j.optcom.2020.126054
Zhu, G., Fang, M., He, S., Qin, F., Yang, X., Wang, Y., et al. (2020a). Floating GaN Whispering Gallery Mode Micro-Ring Lasing with Burstein-Moss Effect. Aip Adv. 10, 105023. doi:10.1063/5.0015222
Keywords: GaN, microcavity, lasing properties, laser diode, whispering gallery mode (WGM)
Citation: Zhu G, Qin F, Li X, Sun Y, Gao F, Tian M, Ji B and Wang Y (2022) Research Progress of Gallium Nitride Microdisk Cavity Laser. Front. Mater. 9:845885. doi: 10.3389/fmats.2022.845885
Received: 30 December 2021; Accepted: 02 March 2022;
Published: 06 April 2022.
Edited by:
Mingming Jiang, Nanjing University of Aeronautics and Astronautics, ChinaReviewed by:
Hongyu Chen, South China Normal University, ChinaChangzheng Sun, Tsinghua University, China
Binghui Li, Changchun Institute of Optics, Fine Mechanics and Physics (CAS), China
Copyright © 2022 Zhu, Qin, Li, Sun, Gao, Tian, Ji and Wang. This is an open-access article distributed under the terms of the Creative Commons Attribution License (CC BY). The use, distribution or reproduction in other forums is permitted, provided the original author(s) and the copyright owner(s) are credited and that the original publication in this journal is cited, in accordance with accepted academic practice. No use, distribution or reproduction is permitted which does not comply with these terms.
*Correspondence: Gangyi Zhu, emh1Z2FuZ3lpQG5qdXB0LmVkdS5jbg==