- 1School of Civil Engineering, Chongqing University, Chongqing, China
- 2The National Centre for International Research of Low-Carbon and Green Buildings, Ministry of Science and Technology, and the Joint International Research Laboratory of Green Buildings and Built Environments, Ministry of Education, Chongqing, China
- 3Key Laboratory of New Technology for Construction of Cities in Mountain Area, Chongqing University, Chongqing, China
- 4School of Arts, Guizhou Normal University, Guiyang, China
The benefits of using wood in tall and commercial construction are undisputed, namely reducing the carbon footprint, shortening construction times, and enhancing seismic and building physics performance. The international market for wood as a structural material in tall and non-residential construction, however, is still relatively untapped. China is home to the world’s largest population and the largest construction sector worldwide, yet wood products are only used in a small fraction of buildings. The main reasons for this situation are the fire regulations and lack of guidelines for novel wood-based structural systems. This paper describes the design of a 10-storey timber-concrete business hotel which will be erected in the Guizhou province of China. The foundation design, gravity system design, lateral load resisting system design, seismic analysis and the fire resistance design were conducted, and the procedure provided appropriate information to the technological feasibility to promote the development of timber-based hybrid high-rise construction systems in China.
Introduction
Background
Wood, as one of the most attractive construction materials due to its renewability and carbon sequestration, has always been an essential part of the built environment (Foliente, 2000). Multiple studies (Buchanan et al., 2012; De Wolf et al., 2017; Andersen et al., 2021; Ryberg et al., 2021) demonstrated when compared to concrete or steel, using wood or its engineered wood product derivatives, e.g. Glued-Laminated Timber (GLT), Cross Laminated Timber (CLT), Laminated Veneer Lumber (LVL) and so on, as building materials present a favourable environmental balance. Wood contains a lot of solar energy, only a small amount of fossil fuel is needed in the production process, that is generally pollution-free in the production process. Modern wood structures produce little construction waste, which can improve the utilization efficiency of materials. Moreover, wood waste can be burned as renewable energy instead of fossil fuel to reduce energy consumption. The carbon dioxide released by wood combustion is the solar energy stored in the wood itself, which is part of the natural cycle and will not lead to the increase of greenhouse gases (Buchanan, 2007). According to Ritter et al., the proper installation and use of wood products in houses often reduce the greenhouse gases produced by products, and the environmental burden is lower than other building materials with the same function (Ritter et al., 2011). According to the case of tall wooden buildings, it is found that the cost of a 20-storey CLT building with the same function is roughly the same as that of a concrete building (Robertson et al., 2012). With the proposal of sustainable development goals and the increasing awareness of environmental protection, the positive attributes of wood are gradually highlighted, making it become the preferred green building material to replace steel and concrete.
Although the application of wood in building systems has a good performance record, the international market for wood as a structural material in tall and commercial construction is still relatively small and the building regulations all over the world limit the building height and area of wood in high-rise buildings, especially in China, where the relative research on the timber-based structure is backward.
Tall and Hybrid Timber Construction
With the rapidly increasing human population and the corresponding pressures on land resources, it has been argued that it is a matter of urgency to find a way to expand the possibilities of using wood as an efficient solution for large-scale tall buildings (Green and Karsh, 2012). Therefore, timber high-rise or timber-hybrid buildings are attracting more and more attention worldwide. Many studies have confirmed that timber and timber-hybrid buildings have enormous potential to reach higher heights with desired structural and environmental performance (Foster et al., 2016; Ramage et al., 2017; Wimmers, 2017; Zhang et al., 2021). Over the last decade, North America, Europe and other countries have begun to study the new system of building multi-storey and high-rise timber hybrid structures with other materials, for example, the concrete or the steel (He et al., 2016; Zhang et al., 2017; Zhang et al., 2018). Several feasibility studies for innovative tall timber-based hybrid structural systems were carried out, and many prototype buildings were tested (Kuilen et al., 2011; Hristovski et al., 2013; Zhang et al., 2016; Izzi et al., 2018). Meanwhile, several tall timber and timber-hybrid buildings, e.g., the 18-story Brock Commons in Canada (Tannert and Moudgil, 2017) and the 18-story Mjøstårnet in Norway (Abrahamsen, 2017), have been successfully built and are operating well, which demonstrates great competitiveness in the aspects of structural performance, environmental friendliness, and construction speed.
Timber-concrete hybrid building refers to the structure form dominated by wood structure and concrete structure. The most common type of tall wood hybrid structures is combining a case-in-place concrete core that resists the lateral loads, with the timber structure carrying the reminder of the gravity load and diaphragm loads. According to relevant research, timber concrete hybrid structure gives full play to the best performance of wood and concrete, and has higher bearing capacity, fire resistance and seismic performance compared with pure wood structure (Poirier et al., 2016).
Timber Construction in China
Building tall structures with wood in China is not new - pagodas built hundreds of years ago are still standing despite exposure to seismic events, strong winds, and high-moisture environments (Lam et al., 2008). However, due to the shortage of wood resources and fire safety considerations, the government set strict rules to limit the use of timber and more attention was paid on the study and construction of steel and concrete structures (Li et al., 2016; Karol et al., 2018; Li and Wang, 2021). Thus, the timber construction decreased rapidly and there have height and area limitations on it (timber structures were once limited to no more than three storeys in China, and the height was limited to no more than 10 m) (GB50016-2014: Code for Building Ddesign on Ffire protection, 2018).
Thanks to the advances in fire separation/fire-stopping system technology and the production of engineered wood products in worldwide, especially the global integration of low-carbon economy, increasing attention has been paid to using and researching structural timber in China in the past 2 decades. Imported dimension timber, glued-laminated timber and cross-laminated timber have recently been used to build homes, schools, shopping malls and stadiums, and substantial research has been undertaken on novel timber-based hybrid structures (Li et al., 2018).
Since the long construction periods for reinforced concrete buildings cause serious on-site noise and dust pollution, the Chinese government recently proposed a reform of the national approach to building systems and an implementation of the industrialization of buildings. Efforts are made to promote and develop prefabricated buildings which also include wood constructions. With the growing interest in using timber for structures and in expanding the tall wood building sector, there is a desire to develop design guidance for timber high-rises under consideration of the very strict fire resistance requirements in China. One approach, and currently the most feasible for China, to overcome the height and scale limitations in building codes is to use wood in combination with non-combustible materials. The timber-concrete hybrid building is one of the good options to provide a good balance for people’s living quality, environmental conservation, and fire protection.
Objective
The Objective of this study is to provide appropriate information on developing a timber concrete hybrid high rise building in China. Case studies of a 10-storey glulam timber frame with the concrete core business hotel are provided to present its structural design details and seismic performance.
Structural Design of the Timber- Steel Hybrid Building
Overview
A 10-storey timber-concrete hybrid building, proposed by the Guizhou Jianhe Yuanfang Forestry Investment & Explore Co., Ltd. (YF for short). was designed by Equilibrium Consulting Inc. The building was proposed for the location Jianhe County, Guizhou province of China and is designed for occupation as a luxury business hotel.
The building consists of a concrete core, concrete base and a Glulam frame system, shown in Figure 1, the first concrete floor is the parking lot, top nine stories of mass-timber superstructure, carrying all gravity loads, rest on the concrete core that act as both the wind and seismic lateral load-resisting systems. As shown in Figure 2, The 10-storey building has a typical inter-storey height of 3.9 m, a total building height of 46.43 m to the top of the timber roof, a typical storey floor area of 924 m2 (23.1 × 40 m), a total 10,000 m2 building area and provides lobbies, hotel rooms, and recreation clubs on floors 2–10. As it will be erected in China, the whole structure was designed mostly in accordance with the current structural design codes and technical standards of the People’s Republic of China. For some key connections or components design which lacks of Chinese code, like the timber - concrete composite (TCC) beam design, Eurocode 5 (Steer, 2001) will be used as a reference.
Foundation
Foundation design depends on the several factors like site location and soil conditions. Guizhou is a mountainous province with higher altitudes at the eastern end of the Yungui Plateau. According to Geotechnical Engineering Investigation Code of China (GB50021-2001 Code for Geotechnical engineering Investigation, 2002), the subsurface soil conditions at the proposed development site consisted of miscellaneous filling soil (Q4ml) and moderately weathered argillaceous-siliceous rock, which are selected as the foundation bearing layer based on the geological investigation report.
Shallow foundations are recommended for most concrete columns as they located on rocks. The columns in the southwest corner adopt traditional manual hole digging pile because of the soft fill dirt. Raft footing are utilized for foundation of cores on the base and rock bolts are applied at critical locations to provide adequate anti-overturning moment. The reinforced cast-in-place concrete sheets are placed on top of layered compacted soil, and sufficient soft fill dirt is substituted in the southwest corner in order to reduce the uneven settlement.
Gravity Load Resisting System
Glulam Beam and Timber-Concrete Composite Beam
The timber-concrete hybrid building was designed for seismic precautionary intensity 7 with a basic acceleration of ground motion 0.1 g according to the GB50011-2010 (GB50011-2010 Code for Seismic Design of Buildings, 2016) for a site class II in Guizhou, China. The dead load for typical timber floor was 2.5 kPa, for concrete floor was 5.0 kPa and for timber roof was 1.5 kPa respectively. On the roof, the live load was considered as 0.5 kPa. In the typical floors, the live load for the guest rooms was 2.0 kPa, for public space (including balcony, corridors, lobby, tea room and private room) was 2.5 kPa, for stairs was 3.5 kPa, for mechanical/electrical space was 7 kPa, and for partition was 1.0 kPa respectively. The reference snow pressure and reference wind pressure with a 50-year return period was 0.2 and 0.3 kPa respectively. All the load values were obtained based on GB 50009-2012 (GB 50009-2012 Loadcodeforthedesignofbuildingstructures, 2012) and they are typical for Chinese hotels.
The gravity system for this 10-storey timber-concrete hybrid structure adopts Glulam frame system as shown in Figure 1. The material properties of the glulam timber in this research are based on the products supplied by YF, the structural member specifications and material parameters are shown in Table 1.

TABLE 1. Structural member specifications and material parameters (GB T 50708, 2012) (GB 50010-2012: Code for design of concrete structures, 2015).
The glulam girders are the main framing beams which play a role in overall stability of the structure frame. They span between 9 and 11.1 m in north-south direction with secondary glulam beams placed on top in east-west direction to support the OSB floor slabs and the depth of girders is considered as 580 mm in order to satisfy the structural clearance requirements.
According to the design loads and the tributary-area design methods, the 290×2-290 mm cross section was selected for the glulam beam girders and 240 × 400 mm cross section was selected for secondary glulam beams. Table 2 summarizes the glulam beam sizes with corresponding utilizations as ratio of resistance vs. demand.
For some main girders which support high design loads with the span-depth ratio of 19 exceeds the common usage of glulam beams (normally round 12∼14), the timber (glulam)—concrete composite (TCC) beams are used to meet the structural strength, stiffness, and vibration performance requirements.
The applied TCC beams focus on optimizing performance and material requirements by engineering a structural connection between glulam and concrete components. Structural efficiency is gained by creating composite action between these two materials. The efficiency of TCC beams depends strongly on the shear connection employed and can be expressed as a coefficient between 0 and 1. If the two materials work together perfectly as one beam and no slip between the contacting surfaces, the coefficient can be assumed to be 1, in this case, composite sections can be evaluated based on Euler- Bernouli beam theory that plane sections remain plane, as shown in Figure 3A; if the two materials work separately and there is no composite action, the coefficient is assumed to be 0, as shown in Figure 3C. For most engineered TCC beams, partial composite action can be achieved, as shown in Figure 3B, with more rigid shear connections, leading to higher coefficients closer to 1. To guarantee sufficient stiffness and strength of the glulam-concrete composite beam, the glued-in Holz-Beton-Verbund (HBV) shear connector (TiComTec Technical dossier HBV-systems, 2011), as shown in Figure 4, is used.
The HBV system consists of an expanded steel mesh with 2.5 mm thickness that is glued into a saw cut in the timber member and then concrete is cast on top. The adhesive and the concrete fill the voids in the mesh creating a mechanical “adhesive dowel” connection, which, if designed properly, will lead to the steel mesh yielding before adhesive failure (Gerber and Tannert, 2015). Glued in rebar, both angled and straight, can help push the failure into the timber beam after full plasticization of the connectors (Ceccotti et al., 2007). The HBV has been successfully tested and approved and maintains a high degree of stiffness and load bearing capacity even through an unsupported separation layer (Lehringer and Gabriel, 2014). The composite coefficient for HBV system is as high as 0.85 and the stiffness of the TCC beams using this type of connector can reach more than three times of traditional glulam beam. The design of the timber-concrete composite beam is based on Eurocode 5 (Steer, 2001), the capacity of the concrete, timber and the HBV connector components that constitute the TCC beam all need to be checked. As shown in Table 3, there are four different types of the TCC beam that are marked as TCC1 to TCC4, the location of the TCC beams in the floor framing and their design details are presented in Figure 5.
HSK Composite Glulam Columns
Similar to the glulam beams, the size selection for glulam columns are also based on the Chinese timber building designing codes (GB 50009-2012 Loadcodeforthedesignofbuildingstructures, 2012) (GB5005-2017: Standard for design of timber structures, 2017; GB 50206-2012 Code for construction quality acceptance of timber structures, 2012; GB T 50329 2002, 2002). Based on the vertical load take-off, the design loads for the glulam column on the bottom floor are shown in Figure 6, typical column size is designed accordingly as 580 × 580 mm, the ratio of compression resistance versus Demand in the ultimate limit state is 63.3%, 71.6% in 1-hour fire resistance and 99.5% in 2-hour fire resistance respectively.
Considering the limitation of manufacturing technology, two 290 × 580 mm glulam columns are designed and combined using Holz-Stahl-Komposit (HSK) connector as one single column. The HSK connector is based on adhesively bonded perforated steel plates. The holes in the plate are filled by the adhesive after inserting it into the wood, forming so-called “Adhesive Dowels”. The “bond capacity” is based on the sum of the individual adhesive dowel capacities which has been proved to equal 0.8kN (Bathon et al., 2014). As long as enough adhesive dowels are provided, the connection can be very stiff and the two glulam columns can perfectly work together as a whole.
As shown in Figure 7, the manufacturing process includes three steps. Firstly, providing slot at the composite face of each segment column; secondly, injecting glue in segment 1 slots and inserting HSK plats; thirdly, combing segment 1 and 2 to form a composite column, injecting glue via holes in segment 2 until glue comes out from vent holes. Figure 7 shows the connection detail for the HSK composite glulam column to the concrete base.
Aside from the calculation of axial compression capacity and stability, the shortening of the glulam column under the compression force should also be taken into account, because the differential shortening between columns and concrete core walls will cause additional stresses on the members.
Figure 9 presents the detailed shortening calculation of the glulam column which carries the largest compression force. The results shows that the maximum axial shortening of the column under long term loading is only around 5 mm. As the concrete core walls are very stiff, the long-term deformation can be ignored. The distance between them is around 8 m, that results in the slop changes of the floor is only around 0.6% and the stress influence on the floor slab can be ignored.
Lateral Load Resisting System Design and Seismic Analysis
The concrete core walls for this 10-storey business hotel act as the Lateral Load Resisting System (LLRS) to resist the seismic and wind loads. In seismic design, the core together with the fundamental concrete walls are modelled in ETABS and verified with the hand calculation based on the Equivalent Static Method (ESM).
The calculation of ESM for seismic design is simple, while it is only suitable for building less than 40 m based on seismic design code (GB50011-2010 Code for Seismic Design of Buildings, 2016). As the height of this 10-storey business hotel (47 m) exceeds the limitation, and the mode analysis in ETABS is needed. The mode-superposition method is a linear dynamic-response procedure which evaluates and superimposes free-vibration mode shapes to characterize displacement patterns. Mode shapes describe the configurations into which a structure will naturally displace. The obtained base shears for these two methods were compared in Table 4.

TABLE 4. Comparison of base shear under equivalent static method (ESM) and mode-superposition method in ETABS.
The linear dynamic analysis in ETABS considered twelve vibration modes, the model mass participation ratio reaches 95% which meets the code requirements. The dynamic analysis shows that the fundamental vibration mode contributes the majority of the base shear and the higher-mode effect on the seismic responses can be ignored for this timber-based hybrid tall building.
The first three vibration modes are shown in Figure 10. The fundamental and second vibration modes are translational motion and the third one is torsion. As the torsional stiffness of the concrete core is large, the torsional deformation is controllable. Also, the calculated torsional sensitivity ratio equals 1.1 which is smaller than 1.7 limitation in the seismic design code, that means torsional effects can be ignored for this structure.
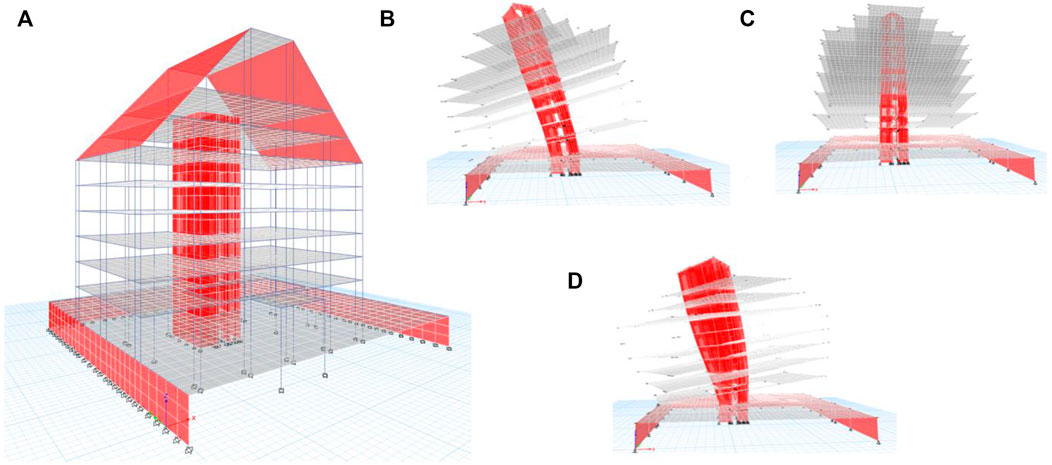
FIGURE 10. Model Shapes of the LLRS: (A) ETABS Mode of the LLRS; (B) First Mode: Translation in East-West; (C) Second Mode: Translation in South-North; (D) Third Mode: Torsion.
A total of seven ground motion records, including five historical strong earthquake records and two artificial records, were selected for the analyses. According to the GB50011-2010 (GB50011-2010 Code for Seismic Design of Buildings, 2016), for the Jianhe County in Guizhou with 7 seismic fortification and peak ground acceleration of 0.1 g, the maximum seismic influence coefficient αmax is set as 0.08 and the corresponding targe spectrum is plotted in Figure 11. Those five earthquake records were selected from the Pacific Earthquake Engineering Research (PEER) database (PEER, 2013), as summarized in Table 5. All the selected records were linearly scaled so that their response spectra matched to the target design spectrum of the building site, as shown in Figure 11.
Figure 12 shows the mean seismic response results where the blue line represents the translation response in East-West (E-W) direction (X) and red line represents the North-South (N-S) direction (Y). Overall the building exhibited adequate seismic resistance that the maximum inter-story drifts were 0.024 and 0.020% for both directions, which were below the drift limit of 1/1000. The building had a maximum roof displacement of 6.8 and 5.4 mm in the E-W and NS directions, respectively, with a base shear of 2,480 and 2070 kN.
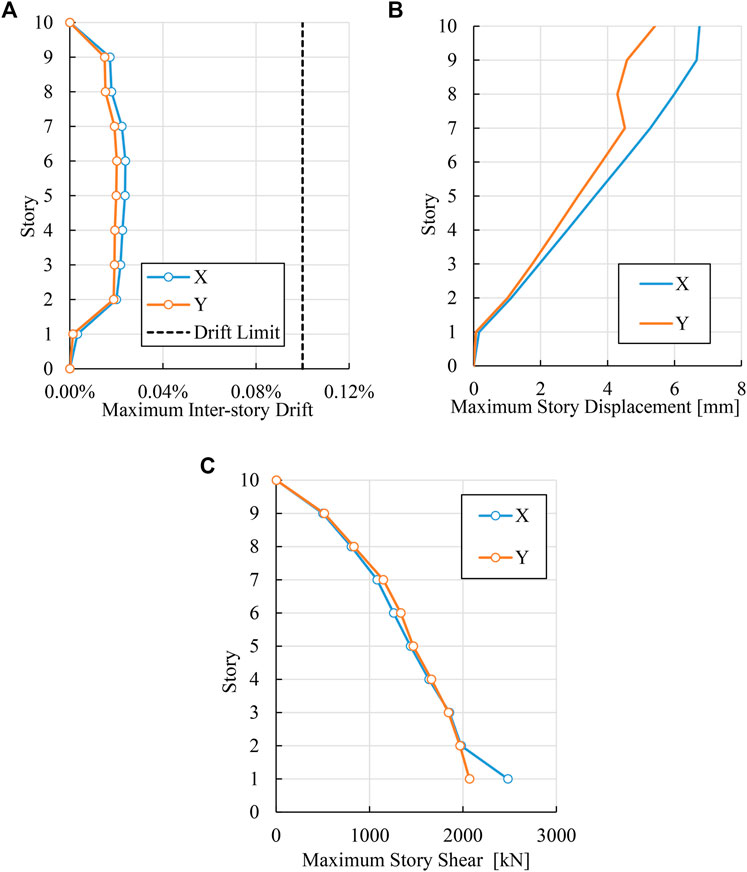
FIGURE 12. Seismic Response, (A) maximum inter-story drift; (B) maximum story displacement; (C) maximum story shear.
With regard to wind resisting analysis, the calculated maximum wind design force is in the south-west direction and equals 1758kN, which is only 71% of seismic design load in that direction. Therefore, the seismic load governs the design of the LLRS. Besides, as the fundamental period is less than 1s, the structural lateral response (displacement and acceleration) resulting from the wind almost has no effect to the comfort of living.
Fire Design
The fire design is of great importance for this project as the fire resistance requirements for timber buildings in China are very strict. To meet these requirements, the following strategy was chosen. First, the concrete topping on the floor slabs is an effective fire and smoke barrier. The 30 mm thick OSB floor slab belongs to category of heavy timber which also has some extent of fire protection. Even it burns, the 75 mm thick reinforced concrete topping can provide additional protection during a fire. Besides, as the main components of the gravity system, the glulam columns are designed for 2-hour fire-resistance, and the glulam girders and secondary glulam beams are designed for 1-hour fire resistance. The charring rate is considered 0.6 mm/min and 10 mm addition char layer is reduced to obtain the residual cross section for load capacity calculation (Karacabeyli and Desjardins, 2011). The design illustration of fire-damaged glulam members is shown in Figure 13.
Another fire protection consideration is using concealed connections, RICON and MEGANT connections. The RICON connector is a pre-engineered post-to-beam connector, a system manufactured from mild steel with a welded collar bolt. It consists of two identical parts, one installed in the primary timber member and the other in the secondary member. The MEGANT connector is also a pre-engineered post-to-beam connector, a system manufactured from aluminum with connector plates and threaded rod to secure the connection. These two types of connections have been tested for the North American mass timber market and are applied in the girder to secondary beam joints and girder to column joints in this project, as shown in Figure 14.
Figure 14 gives the connection application detail in glulam beam to column joints. The RICON and MEGANT, as the pre-engineered connections, can be installed fully concealed or visible and provide large shear capacity, not only suitable for timber to timber, but also can be used for connecting the timber element to the concrete core walls.
Conclusion
This paper presented the design detail of a 10-storey timber-concrete hybrid building in China. The procedure includes foundation design, gravity system design, lateral load resisting system design, seismic analysis and the fire resistance design. It meets the requirements of Chinese building code with the advanced timber design technology and the structural analysis results proved the accuracy of the design, it can serve as guidance for subsequent timber-based hybrid high-rise structures and will promote the development of novel construction systems in China.
Data Availability Statement
The original contributions presented in the study are included in the article/supplementary material, further inquiries can be directed to the corresponding author.
Author Contributions
XZ: conceptualization, methodology, validation, analysis, writing-original draft, LX: validation, analysis, WH: validation, investigation, YL: conceptualization, validation, PL: conceptualization, investigation, writing-review and editing.
Funding
The authors would like to thank the National Key R&D Program of China (Grant Nos. 2021YFF0500804), the National Natural Science Foundation of China (Grant Nos. 52108192); the OEICDI fund (Grant Nos. B13041); the Entrepreneurship and Innovation Support Program for Overseas-educated student in Chongqing China (Grant Nos. CX2021085); This study also received funding from MITCAS Canada through an Elevate grant with Equilibrium Consulting Inc.
Conflict of Interest
This study received funding from Equilibrium Consulting Inc. The funder had the following involvement with the study: the gravity system design, lateral load resisting system design and the fire design.
Publisher’s Note
All claims expressed in this article are solely those of the authors and do not necessarily represent those of their affiliated organizations, or those of the publisher, the editors and the reviewers. Any product that may be evaluated in this article, or claim that may be made by its manufacturer, is not guaranteed or endorsed by the publisher.
References
Abrahamsen, R. (2017). “Mjøstårnet-Construction of an 81 M Tall Timber Building,” in Internationales Holzbau-Forum IHF, 1–12.
Andersen, J. H., Rasmussen, N. L., and Ryberg, M. W. (2021). Comparative Life Cycle Assessment of Cross Laminated Timber Building and Concrete Building with Special Focus on Biogenic Carbon. Energy and Buildings, 111604.
Bathon, L., Bletz-Mühldorfer, O., Schmidt, J., and Diehl, F. (2014). Fatigue Design of Adhesive Connections Using Perforated Steel Plates. Quebec, CA: World Conference for Timber Engineering.
Buchanan, A. (2007). Energy and CO2 Advantages of Wood for Sustainable Buildings. New Zealand Timber Des. J. 15 (1), 11. doi:10.1016/j.soilbio.2014.05.017
Buchanan, A. H., John, S., and Love, S. (2012). LCA and Carbon Footprint of Multi-Storey Timber Buildings Compared with Steel and concrete Buildings. World 15, 19
Ceccotti, A., Fragiacomo, M., and Giordano, S. (2007). Long-term and Collapse Tests on a Timber-concrete Composite Beam with Glued-In Connection. Mater. Struct. 40 (1), 15–25. doi:10.1617/s11527-006-9094-z
De Wolf, C., Pomponi, F., and Moncaster, A. (2017). Measuring Embodied Carbon Dioxide Equivalent of Buildings: A Review and Critique of Current Industry Practice. Energy and Buildings 140, 68–80. doi:10.1016/j.enbuild.2017.01.075
Foliente, G. C. (2000). “History of Timber Construction,” in Wood Structures: A Global Forum on the Treatment, Conservation, and Repair of Cultural Heritage (ASTM International).
Foster, R. M., Reynolds, T. P. S., and Ramage, M. H. (2016). Proposal for Defining a Tall Timber Building. J. Struct. Eng. 142 (12), 02516001. doi:10.1061/(asce)st.1943-541x.0001615
Gb 50009-2012 Loadcodeforthedesignofbuildingstructures, (2012). National Standards ofthePeople’s Republic ofChina ,Ministry ofConstruction ,PRC. Beijing: China Building Industry Press.
GB 50010-2012: Code for design of concrete structures, (2015). National Standards of the People’s Republic of China, Ministry of Construction, PRC. Beijing: China Building Industry Press.
Gb 50206-2012 Code for construction quality acceptance of timber structures, (2012). National Standards of the People’s Republic of China, Ministry of Construction, PRC. Beijing: China Building Industry Press.
Gb T 50329 2002, (2002). Technical Standard for Methods Testing of Timber Structures. Beijing: China Building Industry Press.
Gb T 50708, (2012).Technical Standard for glued laminated timber structures. Beijing: China Building Industry Press.
Gb50011-2010 Code for Seismic Design of Buildings, (2016). National Standards of the People’s Republic of China, Ministry of Construction, PRC. Beijing: China Building Industry Press.
GB50016-2014: Code for Building Design on Fire protection, (2018). National Standards of the People’s Republic of China, Ministry of Construction, PRC. China Building Industry Press, Beijing.
Gb50021-2001 Code for Geotechnical engineering Investigation, (2002). National Standards of the People’s Republic of China, Ministry of Construction, PRC. Beijing: China Building Industry Press.
Gb5005-2017: Standard for design of timber structures, (2017). National Standards of the People’s Republic of China, Ministry of Construction, PRC. Beijing: China Building Industry Press.
Gerber, A., and Tannert, T. (2015). “Timber-concrete Composites Using Flat-Plate Engineered wood Products,” in ASCE Structures Congress (USA: Portland). doi:10.1061/9780784479117.201
Green, M., and Karsh, J. E. (2012). Tall wood -The Case for Tall wood Buildings. Vancouver: Wood Enterprise CoalitionCanada.
He, M., Tao, D., and Li, Z. (2016). Research Progress of Multi-story and High-Rise Timber and Timber Hybrid Structures. J. Building Structures 37 (10), 1–9. doi:10.14006/j.jzjgxb.2016.10.001
Hristovski, V., Dujic, B., Stojmanovska, M., and Mircevska, V. (2013). Full-scale Shaking-Table Tests of XLam Panel Systems and Numerical Verification: Specimen 1. J. Struct. Eng. 139 (11), 2010–2018. doi:10.1061/(asce)st.1943-541x.0000754
Izzi, M., Casagrande, D., Bezzi, S., Pasca, D., Follesa, M., and Tomasi, R. (2018). Seismic Behaviour of Cross-Laminated Timber Structures: A State-Of-The-Art Review. Eng. Structures 170, 42–52. doi:10.1016/j.engstruct.2018.05.060
Karol, S., Jianli, H., Isaac, G., Weiqi, X., Subo, W., and Zitong, C. (2018). July)Feasibility Study on Further Utilization of Timber in ChinaIOP Conference Series: Materials Science and Engineering. IOP Conf. Ser. Mater. Sci. Eng. 383 (No. 1), 012029. doi:10.1088/1757-899x/383/1/012029
Kuilen, J. W. G. V. D., Ceccotti, A., Xia, Z., and He, M. (2011). Very Tall Wooden Buildings with Cross Laminated Timber. Proced. Eng. 14, 1621–1628. doi:10.1016/j.proeng.2011.07.204
Lam, F., He, M., and Yao, C. Example of traditional tall timber buildings in China the Yingxian pagoda. Struct. Eng. Int. 18, 126–129. doi:10.2749/101686608784218743
Lehringer, C., and Gabriel, J. (2014). Review of Recent Research Activities on One-Component PUR-Adhesives for Engineered wood Products. Mater. joints timber structures, 405–420. doi:10.1007/978-94-007-7811-5_37
Li, B., Cai, L., Wang, K., and Zhang, Y. (2016). Prediction of the Residual Strength for Durability Failure of concrete Structure in Acidic Environments. J. Wuhan Univ. Technol.-Mat. Sci. Edit. 31 (2), 340–344. doi:10.1007/s11595-016-1373-0
Li, P., and Wang, H. (2021). A Novel Strategy for the Crossarm Length Optimization of PSSCs Based on Multi-Dimensional Global Optimization Algorithms. Eng. Structures 238, 112238. doi:10.1016/j.engstruct.2021.112238
Li, Z., Zhou, R., He, M., and Sun, X. (2018). August)Modern Timber Construction Technology and Engineering Applications in ChinaProceedings of the Institution of Civil Engineers-Civil Engineering. Thomas Telford Ltd 172 (5), 17. doi:10.1680/jcien.18.00024
Peer, (2013). Pacific Earthquake Engineering Research (PEER) NGA-West 2 Database. Berkeley, CA, USA.
Poirier, E., Moudgil, M., Fallahi, A., Staub-French, S., and Tannert, T. (2016). Design and Construction of a 53-Meter-Tall Timber Building at the university of British Columbia. Vienna, Austria: Proceedings of WCTE.
Ramage, M., Foster, R., Smith, S., Flanagan, K., and Bakker, R. (2017). Super Tall Timber: Design Research for the Next Generation of Natural Structure. The J. architecture 22 (1), 104–122. doi:10.1080/13602365.2016.1276094
Ritter, M., Skog, K., and Bergman, R. (2011). Science Supporting the Economic and Environmental Benefits of Using wood and wood Products in green Building constructionGeneral Technic Report FPL-GTR-206. Washington, D.C.: Unites States Department of Agriculture, Forest Service, Forest Products Laboratory.
Robertson, A., Lam, F., and Cole, R. (2012). A Comparative Cradle-To-Gate Life Cycle Assessment of Mid-rise Office Building Construction Alternatives: Laminated Timber or Reinforced Concrete. Buildings 2012 (2), 245–270. doi:10.3390/buildings2030245
Ryberg, M. W., Ohms, P. K., Møller, E., and Lading, T. (2021). Comparative Life Cycle Assessment of Four Buildings in Greenland. Building Environ. 204, 108130. doi:10.1016/j.buildenv.2021.108130
Steer, P. J. (2001). EN1995 Eurocode 5: Design of Timber structuresProceedings of the Institution of Civil Engineers-Civil Engineering. Proc. Inst. Civil Eng. - Civil Eng. 144 (6), 39–43. doi:10.1680/cien.2001.144.6.39
Tannert, T., and Moudgil, M. (2017). Structural Design, Approval, and Monitoring of a UBC Tall wood Building. Structures Congress, 541–547. doi:10.1061/9780784480410.045
TiComTec Technical dossier Hbv-systems, (2011). Retrieved from https://ticomtec.de/en/hbvsystems/.
Wimmers, G. (2017). Wood: a Construction Material for Tall Buildings. Nat. Rev. Mater. 2 (12), 1–2. doi:10.1038/natrevmats.2017.51
Zhang, X., Azim, M. R., Bhat, P., Popovski, M., and Tannert, T. (2017). Seismic Performance of Embedded Steel Beam Connection in Cross-Laminated Timber Panels for Tall-wood Hybrid System. Can. J. Civ. Eng. 44 (8), 611–618. doi:10.1139/cjce-2016-0386
Zhang, X., Fairhurst, M., and Tannert, T. (2016). Ductility Estimation for a Novel Timber–Steel Hybrid System. J. Struct. Eng. 142 (4), E4015001. doi:10.1061/(asce)st.1943-541x.0001296
Zhang, X., Pan, Y., and Tannert, T. (2021). The Influence of Connection Stiffness on the Dynamic Properties and Seismic Performance of Tall Cross-Laminated Timber Buildings. Eng. Structures 238, 112261. doi:10.1016/j.engstruct.2021.112261
Keywords: tall timber-concrete hybrid building, glulam column, structural design, ground motion, seismic analysis
Citation: Zhang X, Xuan L, Huang W, Yuan L and Li P (2022) Structural Design and Analysis for a Timber-Concrete Hybrid Building. Front. Mater. 9:844398. doi: 10.3389/fmats.2022.844398
Received: 28 December 2021; Accepted: 24 January 2022;
Published: 25 February 2022.
Edited by:
Qian Zhang, FAMU-FSU College of Engineering, United StatesReviewed by:
Zhibin Ling, Suzhou University of Science and Technology, ChinaYingyang Liu, Zhengzhou University, China
Copyright © 2022 Zhang, Xuan, Huang, Yuan and Li. This is an open-access article distributed under the terms of the Creative Commons Attribution License (CC BY). The use, distribution or reproduction in other forums is permitted, provided the original author(s) and the copyright owner(s) are credited and that the original publication in this journal is cited, in accordance with accepted academic practice. No use, distribution or reproduction is permitted which does not comply with these terms.
*Correspondence: Pengcheng Li, bGlwZW5nY2hlbmdAY3F1LmVkdS5jbg==