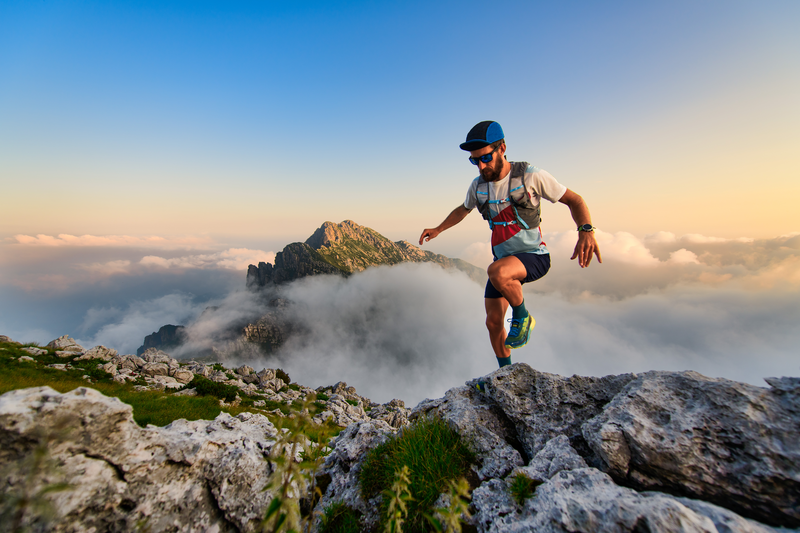
94% of researchers rate our articles as excellent or good
Learn more about the work of our research integrity team to safeguard the quality of each article we publish.
Find out more
ORIGINAL RESEARCH article
Front. Mater. , 18 March 2022
Sec. Structural Materials
Volume 9 - 2022 | https://doi.org/10.3389/fmats.2022.842887
Experimental study on one-dimensional consolidation and scanning electron microscope imaging of expansive soil improved by MICP method has been carried out, by using WG type consolidator and electron scanning microscope. Theoretical analysis on microstructure evolution process of improved expansive soil has been carried out based on fractal theory and damage theory. Through the research, the influence mechanism of cementation and filling effect of calcium carbonate precipitation on the microstructure of improved soil samples such as particle size and pore characteristics is revealed. Based on fractal theory, a porosity calculation model of improved expansive soil has been established considering microstructure damage of soil. Furthermore, a fractal calculation theory of consolidation deformation of improved expansive soil has been proposed. The relevant calculation parameters have also been determined. The rationality of this calculation theory is verified by comparing the calculated results with the tested results. With these research results, a theoretical foundation for further research on microstructure evolution of expansive soil improved by MICP method has been laid. A new train of thought for quantitative research on the water stability and swell–shrink characteristics as well as strength characteristics of improved expansive soil has been provided.
Expansive soil is a special kind of catastrophic clay contribute to its’ characteristics of swelling with water absorption and shrinking with water loss. The construction of projects in areas where expansive soils are distributed often poses a great potential hazard due to their poor engineering characteristics (Sun et al., 1995). Therefore, in the process of engineering construction, it is usually necessary to mix lime, cement or other inorganic materials with the expansive soil to improve and treat it. A large number of engineering practices have shown that these traditional improvement methods have achieved very good improvement effects (Intharasombat et al., 2007; Liu et al., 2011; Bian et al., 2013). However, these methods need to consume a lot of resources and are not economical. Besides they need to prolong the construction period as well as cause serious environmental pollution, which is not in line with the concept of green ecological construction. Therefore, it is very necessary to find a method of expansive soil improvement to meet the ecological and environmental protection requirements of China.
With the development of modern microbial technology, Microbially Induced Carbonate Precipitation (MICP) method is widely used in other engineering fields. Studies have shown that the presence of free metal ions in the soil (Bai et al., 2021a; Bai et al., 2019). MICP is a process whereby certain bacteria in nature produce urease, a natural enzyme that breaks down urea. The carbonate ions produced by the decomposition of urea combine with naturally free calcium metal ions to produce calcium carbonate colloids. The effect is to improve the engineering properties of the soil (Zhao, 2014; Shen et al., 2017).
In geotechnical engineering (Yao et al., 2022; Yao D. et al., 2021), the MICP method is mainly used to improve sandy soils in the early days and achieves very good results, which also greatly promotes the development of biogeotechnical technology (Bai et al., 2017; Bai et al., 2020). A large number of studies have shown that calcium carbonate colloid can greatly improve the cohesion between soil particles (Liu et al., 2018a; Tian Z. F. et al., 2020; Xiao et al., 2021; Dejong et al., 2006; Feng et al., 2017; Kwon et al., 2019; Wu et al., 2020; Jiang and Soga, 2017; Yao Y. et al., 2021; Han and Cheng, 2016). With the continuous development of this technology, scholars at home and abroad have applied the MICP method to the improve the Engneering properties of soft clay soils. Jiang et al. (2021) have identified the effect of the number of amendments on the expansion and contraction characteristics of expansive soils. Li et al. (2021) investigated the effect of microbial concentration on the compressive properties of expanded soils modified by the MICP method. It is found that the process of microbially induced calcium carbonate can also be accomplished in clayey soils under appropriate environmental conditions. This process, moreover, has a significant effect on improving the water stability and strength properties of soft clay soils (Shen et al., 2019; Wang et al., 2020; Kannan et al., 2020; Vail et al., 2019; Bai et al., 2021b). However, at this stage, as a new technology for improving soft soils, there are still two aspects of the research on the application of the MICP method by scholars at home and abroad that need to be addressed. One is that the current research only focuses on the improvement of sandy soils, while the research on the improvement of catastrophic soft clay soils, such as expansive soils, has not yet been reported in the literature. Secondly, the existing studies only focus on the study of the macroscopic properties of the improved soils, but lack the knowledge of the microscopic mechanism of the engineering properties of soft soils improved by the MICP method.
With the development of fractal theory, a brand new way has been proposed to solve complex scientific and technical problems (Liu and Li, 1997; Fenzhen et al., 2011; Sun et al., 2017; Liu et al., 2018b; Szeląg, 2019; Yu et al., 2019; Duan et al., 2021). In the past 20 years, scholars at home and abroad have applied fractal theory to carry out in-depth research on the mechanisms affecting damage cracks, pore evolution and other microstructural changes in geotechnical bodies caused by external loads. Many results have also been achieved (Peng et al., 2011; Gao et al., 2013; Xie and Wei, 2013; Xiao et al., 2014; Ju et al., 2018; Chen et al., 2019; Tian H. et al., 2020; Ge et al., 2021; Liu et al., 2021). It has been shown that the microscopic pore structure of soil has obvious fractal characteristics, and the microscopic structural damage evolution process of soil can be quantitatively analysed by using fractal theory. However, fractal models are currently mostly used for the analysis of unimproved soils and are rarely seen for the analysis of improved expansive soils. In addition, microstructural models for improved expansive soils using the MICP method have rarely been reported. Therefore, this paper investigates the influence of different consolidation pressures on the pore structure of the MICP method for improving expansive soils based on fractal theory through experimental studies such as one-dimensional consolidation and scanning electron microscope imaging. the microscopic mechanism of MICP on the engineering properties of improved expansive soils were revealed.
The expansive soil used in the experimental study comes from the Nanning Ring Road Project in Guangxi. According to the Standard for Geotechnical Test Methods (Ministry of Water Resources of the China, 2019) (GB/T 50123-2019, 2019), the basic physical property indexes of the soil samples obtained by the test, Natural density is 1.89 g/cm3, Maximum dry density is 1.75 g/cm3, Gs is 2.7, WL is 58.1%, Wp is 22.3%, Ip is 35.8%, OMC is 23.3%.
The cements are composed of urea, calcium chloride and water. The concentration of the cementing solution used in this paper is 1 mol/L according to the relevant studies in the literature (Jiang et al., 2021; Li et al., 2021). Based on the relative molecular masses, the content of the various components per 1 L of cementing solution are calculated. The content of urea, calcium and water is 60.06 g, 219.08 g and 891.88 ml respectively.
The microorganism used in the experimental study is Sporosarcina pasteurii, No. ATCC11859, acquired by the Chinese General Microbial Strain Collection Management Centre (CGMCC). The medium is prepared according to the cultivation requirements of the microorganism. The medium consists of 15 g casein peptone, 5 g soya peptone, 20 g urea, 1 L distilled water and 5 g sodium chloride, with a controlled pH of 7.3. The prepared medium is stored in conical flasks sealed with sealing film.
Before bacterial inoculation, the medium and the nozzle of the pipette gun were autoclaved. After autoclaving at 120°C for 20 min, the medium, bacterial solution and alcohol lamp are placed on a sterile bench and the hands, bench and walls of the cup containing the solution are wiped with alcohol. After sterilization, the seal was removed and two tubes of bacterial solution are pipetted into the medium solution, ensuring that the tip of the pipette does not touch the wall of the cup. All the above operations need to be carried out beside the lighted alcohol lamp, with the lighted alcohol lamps and the sterile operating table to ensure a good sterile environment. The culture medium inoculated with bacterial solution is placed in an intelligent shaker set at 30°C and 150 r/min for 36 h. After completion of the incubation, a portion of the solution is extracted and placed in a spectrophotometer and the OD600 value is measured to be stable at around 1.6 before the culture solution is used to improve the expansive soil.
For the modification process, 50 ml of bacterial solution and 125 ml of cementing solution were selected for the test, based on existing research from our group (Jiang et al., 2021; Li et al., 2021). The expansive soil was air-dried and sieved in accordance with the Standard for Geotechnical Test Methods (Ministry of Water Resources of the China, 2019). Then the bacteriological solution and the cementing solution were evenly mixed into the expansive soil. The mixed expansive soil was sealed with cling film, leaving some of the pores to ensure the oxygen required for bacterial metabolism. In addition, the soil samples are placed in a constant temperature and humidity biochemical incubator and maintain for 7 days. After completion of maintenance, the soil samples are air dried to an optimum moisture content of 23.3% required for maximum compaction. Once air-drying is complete, the soil samples are placed in a moisture vat and smothered for 24 h to obtain the improved expansive soil required for the test study. The last step is to prepare the ring knife sample with 90% compactness.
In order to investigate the effect of the MICP method on the microstructure of the improved expansive soils during consolidation, consolidation tests are carried out on the improved expansive soils using a single-lever consolidation apparatus of type WG. The consolidation pressures of 0, 50 kPa, 100 kPa, 200 kPa, 400 kPa and 800 kPa are selected for the one-dimensional consolidation tests on the MICP-amended and unamended expansive soils in accordance with the Geotechnical Test Methods Standard (Ministry of Water Resources of the China, 2019). Prior to the tests, short-necked specific gravity bottles are used to determine the specific gravity of the improved expansive soils and to calculate the initial pore ratio of the ring knife specimens in accordance with the test standard.
After the consolidation test, the specimens under various consolidation pressure conditions are taken out of the consolidation apparatus. Using the small piece sampling method, a small piece of each of the removed consolidation specimens is taken out and dried in an oven. A TESCAN MIRA 4 scanning electron microscope is selected to perform scanning electron microscope imaging tests on the dried specimens.
According to the test results, the e-p curves and ε-p curves for the improved expansive soil and the unimproved expansive soil are obtained respectively, as shown in Figure 1.
From Figure 1, it can be found that the initial pore space of the improved expansive soil is smaller its soil e-p curve is gentler than the data of the unimproved expansive soil, which also means that its compression coefficient is smaller and its compression modulus is larger. In contrast, the unimproved expansive soils have larger initial pores and steeper soil e-p curves, i.e., higher compression coefficients and lower compression moduli. This indicates that the expansive soils improved by the MICP method have become coarser due to the mutual cementation and coalescence of the soil particles as a result of the calcium carbonate precipitation. At the same time, the pore ratio of the soil has become smaller due to the partial filling of the pores by the calcium carbonate precipitation. As a result, the compressibility of the swelling is reduced after the modification and the resistance to external deformation is increased. It can also be found in Figure 1 that when p < 200 kPa, the pore ratio of the improved expanded soil is smaller than that of the unimproved expanded soil under the same consolidation pressure conditions; when p > 200 kPa, the pore ratio of the improved expanded soil is larger than that of the unimproved expanded soil under the same consolidation pressure conditions. This is because the initial pore ratio of the improved soil is reduced, the compressibility of the soil is reduced, and Δe is reduced under the same Δp action. Therefore, the two phenomena reflect the same essence. From Figure 1 it can also be found that the consolidation deformation of the soil samples all increase with the increase of consolidation pressure. Moreover, the consolidation deformation of the improved expansive soil is smaller than the data of the unimproved expansive soil under all levels of consolidation pressure. This result again proves that the microstructure of the expansive soil has been significantly improved and its compressibility has been significantly improved.
The microstructural features of the unimproved expansive soil and the improved expansive soil can be clearly seen by scanning electron microscope imaging tests at a magnification of 5,000. The SEM images of the unimproved expansive soil and improved expansive soil are obtained from the test and are shown in Figure 2, Figure 3, respectively.
As can be seen in Figures 2, 3, the microbially amended swollen soil has a significant presence of calcium carbonate crystals and acts as a cementing cohesion for the soil particles. It can also be found that the particles of the amended soil form cohesions and that the pores between the cohesions are significantly filled. This indicates that the crystallization of calcium carbonate has the effect of cementing for the soil particles and has the effect of filling on the soil pores. This process forms denser agglomerates in the amended soils and improves the stability of the microstructure of the soil particles. In contrast, in the SEM images of the unamended swollen soil, there are almost no calcium carbonate crystals present, and the soil particles have larger pores and many dispersed fine particles around the soil particles, which are extremely loose in microstructure. This finding is a strong evidence that microbially induced calcium carbonate precipitation has altered the microstructure of the expansive soil and improved its water stability and resistance to external deformation.
The microstructural changes of the MICP method improved expansive soils under different consolidation pressures can be investigated by SEM imaging analysis at a magnification of ×1,600. The SEM images of the improved expansive soils under different consolidation pressures are shown in Figure 4.
FIGURE 4. SEM imaging of improved expansive soil, 1,600x. (A) p = 0, (B) p = 50 kPa, (C) = 100 kPa, (D) p = 200 kPa, (E) p = 400 kPa, (F) p = 800 kPa.
From Figure 4, it can be found that the pore space between the soil particles decreases with increasing consolidation pressure and the connection between the particles becomes closer and closer. Similarly, as the consolidation pressure increases, the form of connection between the particles tends to be more and more similar to the face-to-face connection from point-to-point connection or point-to-surface connection. It can also be seen in Figure 4 that as the consolidation pressure increases, the precipitated calcium carbonate evolves from spherical colloids to flattened crystals interspersed between the particles. It is this “cementing” and “filling” effect of the calcium carbonate crystals that significantly increases the cohesion, internal friction angle and strength of the improved soil, and obviously reduces its compressive deformation. At the same time, this microstructural change significantly improves the water stability and expansion deformation characteristics of the expansive soil.
In order to investigate the microstructural changes of the MICP improved expansive soils under different consolidation pressures, SEM images of the soil samples with an area of 4,372 μm2 and a magnification of ×1,600 are quantitatively analysed. Firstly, the IPP software is applied to binarise the corresponding SEM images by manually adjusting the threshold values, and then the pore distribution data are collected. The microscopic pores of the MICP improved expansive soils are classified into six classes under different consolidation pressures according to the requirement of pore size intervals. The observed results of pore size, pore number and pore area of the various pore classes of MICP improved expansive soils under different consolidation pressures are shown in Table 1.
From Table 1, it can be found that with the increase of consolidation pressure, the area, number and pore size of different grades of pores do not change with the consolidation pressure in a clear pattern (Bai et al., 2021c). This is because under the action of consolidation pressure, the pores are not uniformly stressed resulting in the destruction of large pore size pores into different grades of small pores with different pore morphology. However, the total number of pores in the soil sample is constantly decreasing. This is due to the continuous squeezing and movement between soil particles under consolidation pressure, with the smaller pores being filled by the broken fine soil particles. As a result, the expanded soil improved by the MICP method becomes more compact as the consolidation pressure continues to increase. The relationship between the total number of pores (X) and the consolidation pressure, is shown in Figure 5.
FIGURE 5. Relation between pore number and consolidation pressure. (A) Columnar distribution of pores, (B) Relationship between X and P.
From Figure 5, it can be found that the number of pores gradually decreases as the consolidation pressure increases. However, when the consolidation pressure is greater than 400 kPa, the trend of pore number with consolidation pressure gradually slows down. This law coincides with the variation of pore volume e with consolidation pressure p in expansive soils represented in Figure 1, revealing the microscopic mechanism of the effect of the microbial mineralisation process on the pore ratio, compactness, water stability, deformation and strength properties of improved expansive soils.
The Sierpinski fractal model, used to study the microstructure of soil pores, is an effective analytical tool. The model is a fractal pattern similar to that of a hollow carpet, also known as a two-dimensional fractal model. Based on a regular fractal structure, the area at the hollow, can be expressed as
In Equation 1, the
A (δi) is the area of the hollow small square.
Ni is the number of small squares.
δi is the length of the sides of the small square.
i is the structural grade.
C is a constant.
D is the fractal dimension.
According to fractal theory, hollow small squares can be used to characterize the pores in the soil, and the number of pores N (r) at an observed scale L can be expressed as
In Equation 2, the
N (r) is the number of pores.
r is the pore size of the pore.
Therefore, the total area of N (r) pores can be expressed as
In Equation 3, the
A (r) is the total area of the pore space.
It is known from the theory of soil mechanics that the porosity of a soil is the ratio of the pore volume to the total volume of the soil. According to Sierpinski’s two-dimensional fractal model, if there are N (r) pores in the soil, the corresponding porosity can be expressed as
In Equation 4, the
ϕ is the porosity of the soil.
Cˊ is a constant.
L is the side length of the observation range.
Let X = CˊL−D. When r = L, the blank small square indicating the pore space occupies the whole observation range and the porosity of the soil is 1. Obviously, X = 1. Therefore, Equation 4 can be simplified as
Meanwhile, the total area in the pore space larger than a certain pore size can be expressed as that
In Equation 6, the
Ai is the total pore area in the pore space larger than a certain pore size.
Taking the logarithm of both sides of Eq. 6 yields that
Taking in [1-(Ai/A)] as the vertical coordinate and ln (r/L) as the horizontal coordinate, the relationship curve between the two can be obtained, and the slope of the curve can be obtained by linear fitting, that is, 2-D.
In this paper, A = 4372 μm2 and L = 65.82 μm. Using Origin software, the Sierpinski fractal dimension of the MICP improved expansive soils was obtained from the data in Table 1 and Eq. 7 as a function of different consolidation pressures. As shown in Figure 6.
From the fitting results in Figure 6, It can be found that the fractal dimension of the improved expansive soil is distributed between 1.9 and 2.0. It is known from fractal theory (Tao et al., 2010) that the fractal dimension in a two-dimensional model is between 0 and 2. This indicates that the expanded soils improved by the MICP method have good physical significance in the context of fractal theory, and also indicates that the microscopic pore characteristics of the expanded soils improved by the MICP method have good Sierpinski fractal properties. It can also be found in Figure 6 that the fractal dimension of the MICP-amended expansive soil increases with the increase of consolidation pressure. This indicates that as the external pressure increases, the pore structure in the soil becomes more complex, but its integrity is improved and the soil becomes more compact. This conclusion, which is consistent with the results reflected in Figure 4, also further reveals the microscopic mechanism of the changes in the e–p curves in Figure 1.
Using fractal theory, analysis of scanning electron microscopy images of soil samples reveals that the fractal dimension can well characterise the microscopic porosity of MICP improved expansive soils. It has been shown that the pore space of the geotechnical soil can be better calculated by substituting the fractal dimension into the porosity model (Tao et al., 2010). Thus, it is obtained that
Damage theory suggests that damage within a material accumulates gradually under external loading. Therefore, the use of a damage variable can be a good way to characterise the gradual deterioration of the mechanical properties of a material based on the change in one of the internal variables. The damage variable R is calculated by the equation that
In Equation 9.
A0 is the area of damage to the calcium carbonate.
A is the area of calcium carbonate in the cross section when the consolidation pressure is 0.
The SEM imaging of the MICP improved expansive soil with a magnification of 1,600x is selected for damage analysis. Applying the IPP software, the area of calcium carbonate in (A)the image can be extracted as shown in Table 2.
From Table 2 and Figure 4, it can be found that the area of calcium carbonate precipitation gradually decreases with increasing consolidation pressure. This indicates that during consolidation, the calcium carbonate precipitate is extruded and deformed, and acts as an adsorption of broken soil particles. Under the adsorption effect of calcium carbonate precipitation, the soil particles are gradually compacted, the integrity of the improved expansive soil is improved, and the fractal dimension is increasing. From Equation 9, Table 2 and Figure 5, the relationship between the damage variable R and the fractal dimension D of the MICP improved expansive soil can be obtained, as shown in Figure 7.
An analysis of Figure 7 shows that the effect of the damage variable R on the fractal dimension D of the MICP improved expansive soil can be expressed as
As the damage variable increases, the fractal dimension also increases, further proving that, the calcium carbonate precipitation within the soil plays a role in resisting the external load under the external force of the MICP method improved expansive soil, revealing the mechanism of the influence of calcium carbonate precipitation on the strength properties and deformation of the expansive soil.
According to equation 8–10, the relationship between porosity and pore diameter and damage variables of MICP improved expansive soil based on damage theory can be obtained as,
According to Equation 11, Figure 7 and Table 1, the calculation results of different porosity (n) models for MICP improved expansive soils under various levels of consolidation pressure can be obtained. A comparison of the calculated results with the measured results is shown in Figure 8.
From Figure 8, it can be found that the agreement between the calculated results and the measured results is high under the action of 0–800 kPa consolidation pressure. Therefore, it is verified that the porosity calculation model of the MICP method improved expansive soil based on the fractal theory is reliable and provides a new idea for the analysis of the pore characteristics of the improved expansive soil. The revised porosity calculation model based on damage theory can not only truly reflect the close relationship between calcium carbonate precipitation generated by microbial mineralization and soil deformation, but also take into account the influence of different consolidation pressures on soil microstructure damage degradation. It can also be found in Figure 8 that both the measured and calculated curves show that the porosity of the soil sample decreases rapidly as the consolidation pressure increases, but the rate of porosity reduction also becomes gradually smaller. This indicates that the pore volume in the soil is gradually reduced and the compactness of the soil is gradually increased. This results in a gradual increase in the strength of the soil and a gradual decrease in the compressive deformation of the soil. The analysis of the model calculation results reveals the microscopic mechanism of the effect of the MICP method on the strength and compression characteristics of the improved expansive soil.
According to the theory of soil mechanics, it is known that the pore ratio of soil is e = n/(1−n) and its compression deformation is Δs=(e1−e2) h/(1 + e1). According to Equation 11, the relationship between the compression deformation of soil based on damage theory and the pore diameter and damage variables can be obtained as that
In Equation 12, the
r1 is the diameter of the pore under initial conditions.
r1 is the damage variable under initial conditions.
ri is the diameter of the bore under the ith level of loading.
Ri is the damage variable under load level i.
h is the height of the soil sample.
By substituting the data in Table 1 and Table 2 into Equation 12, the compression deformation of MICP improved expansive soil under different consolidation pressure can be calculated. The comparison between the measured and calculated compressive deformation of the improved expansive soil is shown in Figure 9.
From Figure 9, it can be found that under the action of consolidation pressure from 0 to 800 kPa, the calculated results of compression deformation are closer to the measured results, and the predicted curve has the same development trend as the measured curve. As the consolidation pressure increases, the total compressive deformation of the soil sample increases, but the rate of growth of the compressive deformation gradually becomes smaller. This conclusion indicates that the macroscopic deformation of the soil sample is closely related to the changes in its microstructure. Therefore, the compression deformation calculation method based on fractal theory and damage theory can be used for the settlement analysis of MICP improved expansive soils.
An experimental study has carried out on Nanning expansive soils improved by the MICP method through compression consolidation and scanning electron microscope imaging. A theoretical analysis of the microstructure of the improved expansive soils has carried out based on fractal theory and damage theory. Through the study, the following conclusions are obtained.
1) Through experimental studies such as one-dimensional consolidation and scanning electron microscope imaging, it is found that the MICP method improved the expansive soil and significantly reduces the consolidation deformation of the soil. The calcium carbonate precipitation produced by microbial induction effectively fills the gaps between soil particles and cemented the soil particles. The microstructures of the soil sample were changed, which ultimately led to significant improvement in the water stability, expansion and contraction characteristics and strength properties of the expansive soil.
2) Based on the fractal theory, the theoretical analysis of the SEM imaging test results has carried out. Considering the microstructural damage of the soil, a model for calculating the porosity of the expanded soil improved by the MICP method was established and the model parameters were determined.
3) Through the analysis of the fractal dimension and damage variables of the model, the theory of calculating the compression consolidation deformation of the improved expansive soil is proposed. The calculation parameters are determined. The reasonableness of the calculation theory is verified by comparing the calculation results with the actual measurement results.
The above research results provide a theoretical basis for further research on the microstructural evolution of improved expansive soils using the MICP method. It also provides new ideas for quantitative research on the water stability, expansion and contraction characteristics and strength properties of improved expansive soils.
The original contributions presented in the study are included in the article/Supplementary Material, further inquiries can be directed to the corresponding author.
All authors listed have made a substantial, direct, and intellectual contribution to the work and approved it for publication.
The work described in this paper was supported by a grant from the National Natural Science Foundation of China (Project No. 50978097); the Research Foundation of Education Bureau of Hunan Province, China, Grant Number 19B581.
The authors declare that the research was conducted in the absence of any commercial or financial relationships that could be construed as a potential conflict of interest.
All claims expressed in this article are solely those of the authors and do not necessarily represent those of their affiliated organizations, or those of the publisher, the editors and the reviewers. Any product that may be evaluated in this article, or claim that may be made by its manufacturer, is not guaranteed or endorsed by the publisher.
Bai, B., Jiang, S., Liu, L., Li, X., and Wu, H. (2021a). The Transport of Silica Powders and lead Ions under Unsteady Flow and Variable Injection Concentrations. Powder Technol. 387, 22–30. doi:10.1016/J.POWTEC.2021.04.014
Bai, B., Long, F., Rao, D., and Xu, T. (2017). The Effect of Temperature on the Seepage Transport of Suspended Particles in a Porous Medium. Hydrol. Process. 31 (2), 382–393. doi:10.1002/hyp.11034
Bai, B., Nie, Q., Wu, H., and Hou, J. (2021b). The Attachment-Detachment Mechanism of Ionic/nanoscale/microscale Substances on Quartz Sand in Water. Powder Technol. 394, 1158–1168. doi:10.1016/J.POWTEC.2021.09.051
Bai, B., Nie, Q., Zhang, Y., Wang, X., and Hu, W. (2021c). Cotransport of Heavy Metals and SiO2 Particles at Different Temperatures by Seepage. J. Hydrol. 597, 125771. doi:10.1016/j.jhydrol.2020.125771
Bai, B., Rao, D., Chang, T., and Guo, Z. (2019). A Nonlinear Attachment-Detachment Model with Adsorption Hysteresis for Suspension-Colloidal Transport in Porous media. J. Hydrol. 578, 124080. doi:10.1016/j.jhydrol.2019.124080
Bai, B., Xu, T., Nie, Q., and Li, P. (2020). Temperature-driven Migration of Heavy Metal Pb2+ along with Moisture Movement in Unsaturated Soils. Int. J. Heat Mass Transfer 153, 119573. doi:10.1016/j.ijheatmasstransfer.2020.119573
Bian, J., Jiang, L., and Wang, B. (2013). Experiment on the Strength of Lime-Treated Expensive Soil. J. Chang'an University(Natural Sci. Edition) 33 (2), 38–43. doi:10.19721/j.cnki.1671-8879.2013.02.007
Chen, T., Chen, J., Sha, Y., Bai, W., Wu, Z., and Wang, T. (2019). Microstructure and Fractal Characteristics of Red clay Particles in Existing Foundation Based on SEM Image. Carsologica Sinica 38 (4), 635–642. doi:10.11932/karst20190424
Dejong, J. T., Fritzges, M. B., and Nüsslein, K. (2006). Microbially Induced Cementation to Control Sand Response to Undrained Shear. J. Geotech. Geoenviron. Eng. 132 (11), 1381–1392. doi:10.1061/(ASCE)1090-0241(2006)132:11(1381)
Duan, Q., An, J., Mao, H., Liang, D., Li, H., Wang, S., et al. (2021). Review about the Application of Fractal Theory in the Research of Packaging Materials. Materials 14 (4), 860. doi:10.3390/MA14040860
Feng, K., and Montoya, B. M. (2017). Quantifying Level of Microbial-Induced Cementation for Cyclically Loaded Sand. Geotech. Geoenviron. Eng. 143 (6), 1–4. doi:10.1061/(ASCE)GT.1943-5606.0001682
Gao, Z.-f., Li, L.-s., Wu, Z.-j., Shen, X.-m., Lü, H.-h., and Su, S.-h. (2013). Effects of Microwave Heating on Pore Fractal Properties of Blast Furnace Sludge. J. Iron Steel Res. Int. 20 (8), 27–33. doi:10.1016/S1006-706X(13)60137-5
Ge, M., Li, L., Sheng, D., Zhu, C., and Pineda, J. (2021). Experimental Investigation of Microscopic Deformation Mechanism of Unsaturated Compacted Loess under Hydraulic Coupling Conditions. Rock Soil Mech. 42 (9), 2437–2448. doi:10.16285/j.rsm.2020.1784
Han, Z., and Cheng, X. (2016). An Experimental Study of Microorganism’s Treatment on Liquefiable Sands. Ha'erbin gong ye da xue xue bao 48 (12), 103–107. doi:10.11918/j.issn.0367-6234.2016.12.014
Intharasombat, N., Puppala, A. J., Banavathu, N. G., Qasim, S. R., and Williammee, R. (2007). Experimental Investigations to Evaluate Benefits of Using Compost Amendments to Modify Expansive Soils. Ijewm 1 (2/3), 291. doi:10.1504/IJEWM.2007.013637
Jiang, N.-J., and Soga, K. (2017). The Applicability of Microbially Induced Calcite Precipitation (MICP) for Internal Erosion Control in Gravel-Sand Mixtures. Géotechnique 67 (1), 42–55. doi:10.1680/jgeot.15.P.182
Jiang, W., Zhang, C., Xiao, H., Luo, S., Li, Z., and Li, X. (2021). Preliminary Study on Microbially Improved Expansive Soil of Embankment. Geomechanics Eng. 26 (3), 301–310. doi:10.12989/GAE.2021.26.3.301
Ju, Y., Chen, J., Wang, Y., Gao, F., and Xie, H. (2018). Numerical Analysis of Hydrofracturing Behaviors and Mechanisms of Heterogeneous Reservoir Glutenite, Using the Continuum‐Based Discrete Element Method while Considering Hydromechanical Coupling and Leak‐Off Effects. J. Geophys. Res. Solid Earth 123 (5), 3621–3644. doi:10.1029/2017JB015092
Kannan, K., Bindu, J., and Vinod, P. (2020). Engineering Behaviour of MICP Treated marine Clays. Mar. Georesources Geotechnology 38 (1), 761–769. doi:10.1080/1064119X.2020.1728791
Kwon, Y. M., Chang, I., Lee, M., and Cho, G. C. (2019). Geotechnical Engineering Behavior of Biopolymer-Treated Soft marine Soil. Geomech. Eng. 17 (5), 453–464. doi:10.12989/gae.2019.17.5.453
Li, X., Zhang, C., Xiao, H., Jiang, W., Qian, J., and Li, Z. (2021). Reducing Compressibility of the Expansive Soil by Microbiological-Induced Calcium Carbonate Precipitation. Adv. Civil Eng. 2021 (1), 1–12. doi:10.1155/2021/8818771
Liu, H., Hou, B., Wang, G., and Ma, M. (2018a). Characteristics of Rock Damage Based on Acoustic Emission Parameters and Fractal Theory. China Sciencepaper 13 (22), 2605–2609. doi:10.3969/j.issn.2095-2783.2018.22.015
Liu, H., Xiao, P., Xiao, Y., Wang, J., Chen, Y., and Chu, J. (2018b). Dynamic Behaviors of MICP-Treated Calcareous Sand in Cyclic Tests. Chin. J. Geotechnical Eng. 40 (1), 38–45. doi:10.11779/CJGE201801002
Liu, L., and Liu, K. (2011). Study on the Modification Mechanism of Expansive Soil and Modifiers Contrast Test. Highw. Eng. 36 (1), 142–144. doi:10.3969/j.issn.1674-0610.2011.01.036
Liu, R., Guo, S., and Wang, J. (2021). Pore Characteristics and Evolution of Tight sandstone Reservoirs of Shanxi Formation of Taikang-Bengbu Uplift in Southern North China Basin. J. Yangtze University(Natural Sci. Edition) 18 (3), 1–13. doi:10.3969/j.issn.1673-1409.2021.03.002
Liu, Y., and Li, Y. (1997). Fractal and Multifractal Image Processing and Analysis. Beijing: Tsinghua University.
Peng, R., Yang, Y., Ju, Y., Mao, L., and Yang, Y. (2011). Computation of Fractal Dimension of Rock Pores Based on gray CT Images. Chin. Sci. Bull. 56 (31), 3346–3357. doi:10.1007/s11434-011-4683-9
Shen, D., Wang, Z., Mei, L., Li, F., Shao, G., and Liu, L. (2017). Review of Microbial Induced Calcite Precipitation Ground Improvement Technique. Jiangsu Ke Ji da Xue Xue Bao. J. Jiangsu Univ. Sci. Technol. Zi ran ke Xue ban. 31 (3), 390–398. doi:10.3969/j.issn.1673-4807.2017.03.024
Shen, T., Li, X., Wang, S., Xu, C., and Xue, L. (2019). Triaxial Shear Strength and Immersion Compressive Strength of sandy Clayey Purple Soil Treated by Microbial Induced Calcite Precipitation. Trans. Chin. Soc. Agric. Eng. (Transactions CSAE) 35 (21), 135–143. doi:10.11975/j.issn.1002-6819.2019.21.016
Su, F., Gao, Y., Zhou, C., Yang, X., and Fei, X. (2011). Scale Effects of the continental Coastline of China. J. Geogr. Sci. 21 (6), 1101–1111. doi:10.1007/s11442-011-0903-0
Sun, C., Ying, Z., Wang, F., and Liu, H. (1995). A Review of Research into the Properties of Expansive Soils. Adv. Sci. Technol. Water Resour. 6, 11–15.
Sun, H., Liu, B.-C., and Tian, Q. (2017). Polaron Effects in Cylindrical GaAs/Al X Ga 1- X as Core-Shell Nanowires *. Chin. Phys. B 26 (9), 097302–097420. doi:10.1088/1674-1056/26/9/097302
Szeląg, M. (2019). Properties of Cracking Patterns of Multi-Walled Carbon Nanotube-Reinforced Cement Matrix. Materials 12 (18), 2942–2963. doi:10.3390/ma12182942
Tao, G., Zhang, J., Hang, L., and Yuan, L. (2010). Description of Pore Property of Porous Materials Using Fractal Theory. J. Building Mater. 13 (5), 678–681. doi:10.3969/j.issn.1007-9629.2010.05.023
Tian, H., Li, L., Zhang, K., and Wang, P. (2020). Effects of Dry-Wet and Freeze-Thaw Cycles on Microstructure of Soil Based on SEM. J. Lanzhou Univ. Technol. 46 (4), 122–127. doi:10.3969/j.issn.1673-5196.2020.04.022
Tian, Z.-F., Tang, X., Xiu, Z.-L., and Xue, Z.-J. (2020). Effect of Different Biological Solutions on Microbially Induced Carbonate Precipitation and Reinforcement of Sand. Mar. Georesources Geotechnology 38 (4), 450–460. doi:10.1080/1064119X.2019.1595229
Vail, M., Zhu, C., Tang, C. S., Anderson, L., Moroski, M., and Montalbo-Lomboy, M. T. (2019). Desiccation Cracking Behavior of MICP-Treated Bentonite. Geosciences 9 (9), 385. doi:10.3390/geosciences9090385
Wang, Z., Wei, R., Cai, H., Xiao, J., and Wu, S. (2020). Effect of Nutrient Solution Concentration and Microbial Activity on MICP Solidified Strength of Soft clay. J. China Inst. Water Resour. Hydropower Res. 18 (6), 486–493. doi:10.13244/j.cnki.jiwhr.20190225
Wu, C., Zheng, J., Lai, H., Cui, M., and Song, Y. (2020). Experiental Study of the Strength Enhancing Mechanism of Bio-Cemented Sand and its Influential Factors. J. Civil Environ. Eng. 42 (1), 31–38. doi:10.11835/j.issn.2096-6717.2019.140
Xiao, L., Xue, S., Liu, G., and Zhang, C. (2014). Fractal Features of Soil Profiles under Different Land Use Patterns on the Loess Plateau, China. J. Arid Land 6 (5), 550–560. doi:10.1007/s40333-014-0023-7
Xiao, Y., Wang, Y., Wang, S., Evans, T. M., Stuedlein, A. W., Chu, J., et al. (2021). Homogeneity and Mechanical Behaviors of Sands Improved by a Temperature-Controlled One-phase MICP Method. Acta Geotech. 16 (5), 1417–1427. doi:10.1007/S11440-020-01122-4
Xie, X.-j., and Wei, F.-q. (2013). Soil Aggregates and Fractal Features under Different Land Use Types in a Frequent Debris Flow Area. J. Mt. Sci. 10 (3), 437–444. doi:10.1007/s11629-013-2500-3
Yao, D., Wu, J., Wang, G., Wang, P., Zheng, J.-J., Yan, J., et al. (2021). Effect of Wool Fiber Addition on the Reinforcement of Loose Sands by Microbially Induced Carbonate Precipitation (MICP): Mechanical Property and Underlying Mechanism. Acta Geotech. 16 (5), 1401–1416. doi:10.1007/s11440-020-01112-6
Yao, Y., Li, J., Ni, J., Liang, C., and Zhang, A. (2022). Effects of Gravel Content and Shape on Shear Behaviour of Soil-Rock Mixture: Experiment and DEM Modelling. Comput. Geotechnics 141, 104476. doi:10.1016/j.compgeo.2021.104476
Yao, Y., Ni, J., and Li, J. (2021). Stress-dependent Water Retention of Granite Residual Soil and its Implications for Ground Settlement. Comput. Geotechnics 129, 103835. doi:10.1016/j.compgeo.2020.103835
Yu, J., Jiang, S., and Qin, Y. (2019). Fractal Experimental Study on Crack Evolution of Recycled concrete Beams. Building Sci. 35 (9), 99–105. doi:10.13614/j.cnki.11-1962/tu.2019.09.016
Keywords: microbially induced carbonate precipitation, improved expansive soil, microstructure, fractal theory, damage variable
Citation: Su H, Xiao H, Li Z, Tian X, Luo S, Yu X and Ouyang Q (2022) Experimental Study on Microstructure Evolution and Fractal Features of Expansive Soil Improved by MICP Method. Front. Mater. 9:842887. doi: 10.3389/fmats.2022.842887
Received: 04 January 2022; Accepted: 28 February 2022;
Published: 18 March 2022.
Edited by:
Kequan Yu, Tongji University, ChinaCopyright © 2022 Su, Xiao, Li, Tian, Luo, Yu and Ouyang. This is an open-access article distributed under the terms of the Creative Commons Attribution License (CC BY). The use, distribution or reproduction in other forums is permitted, provided the original author(s) and the copyright owner(s) are credited and that the original publication in this journal is cited, in accordance with accepted academic practice. No use, distribution or reproduction is permitted which does not comply with these terms.
*Correspondence: Hongbin Xiao, dDIwMDkwMTY5QGNzdWZ0LmVkdS5jbg==
Disclaimer: All claims expressed in this article are solely those of the authors and do not necessarily represent those of their affiliated organizations, or those of the publisher, the editors and the reviewers. Any product that may be evaluated in this article or claim that may be made by its manufacturer is not guaranteed or endorsed by the publisher.
Research integrity at Frontiers
Learn more about the work of our research integrity team to safeguard the quality of each article we publish.