- 1School of Stomatology, Southern Medical University, Guangzhou, China
- 2Stomatological Hospital, Southern Medical University, Guangzhou, China
- 3Xinjiang Medical University, Urumqi, China
- 4The First People’s Hospital of Kashi, Kashi, China
This study evaluated the influence of surface free energy (SFE) of titanium disks on the adsorption and conformation of fibronectin (FN) and the biological behavior of osteoblasts cultured on the FN-treated modified surfaces. High [H]-SFE titanium disks were irradiated by a 30 W UV light, while low (L)-SFE titanium disks received no treatment. The surface characteristics of the titanium disks were examined using scanning electron microscope, optical surface profilometer, X-ray photoelectron spectroscopy, and contact angle measurements. Adsorbed FN on different groups was investigated using attenuated total reflection-Fourier transform infrared spectroscopy. MG-63 cells were cultured on FN-treated titanium disks to evaluate the in vitro bioactivity. The experiment showed H-SFE titanium disks adsorbed more FN and acquired more ß-turn content than L-SFE group. MG-63 cells cultured on FN-treated H-SFE titanium disks showed better osteogenic responses, including adhesion, proliferation, alkaline phosphatase activity and mineralization than that on FN-treated L-SFE titanium disks. Compared to L-SFE titanium disks, integrin-β1, integrin-α5 and Rac-1 mRNA levels were significantly higher in MG-63 cells on FN-treated H-SFE after 3 h of culture. These findings suggest that the higher SFE of H-SFE compared to L-SFE titanium disks induced changes in the conformation of adsorbed FN that enhanced the osteogenic activity of MG-63 cells.
Introduction
Titanium and titanium-based alloys are widely used as orthopedic implant materials. Osteointegration is a major factor influencing the success rate of orthopedic implants. Osteointegration is related to implant macroscopic surface structure, surface topography, surface wettability, surface charge, surface free energy (SFE), surface hydrophilicity, pore structure, release of bioactive molecule (Chen et al., 2018), and coating (Smeets et al., 2016). Osteoblasts are not in direct contact with the implant surface immediately after implantation (Barberi and Spriano, 2021). Rather, implants adsorb a thin layer of proteins, including immunoglobulins, vitronectin, fibrinogen and fibronectin (FN), which modulate a pro-inflammatory response and clotting to create the correct microenvironment for osteointegration (Raphel et al., 2016; Barberi and Spriano, 2021). Recently, extensive research efforts have focused on the analysis of protein adsorption to synthetic surfaces (Keselowsky et al., 2003). Findings have showed that altering the surface of titanium-implant materials affects protein adsorption, cell–substrate interactions, and tissue integration (Rapuano et al., 2012; Isoshima et al., 2019; Yao et al., 2019; Zeng et al., 2019; Bayrak et al., 2020; Lin et al., 2020).
FN is a glycoprotein in the extracellular matrix that promotes osteoblast adhesion to a substrate. FN is a dimer of subunits joined by two disulfide bonds at their C-terminal ends. Each subunit has six domains, which perform different functions (Maurer et al., 2016). The arginine-glycine-aspartate (Arg-Gly-Asp, RGD) domain is a binding receptor for integrins, and directly associated with cell adhesion (Asghari Sana et al., 2017). The RGD domain is located in a ß-turn structure in FN, and increasing ß-turn content is associated with improved cell adhesion properties (Hasan et al., 2018). FN has a dynamic structure and must transition from a compact to an extended conformation to expose the RGD domain and mediate cell adhesion (Maurer et al., 2016).
Studies show that implant material and surface properties, including pH, temperature, surface polarity and surface charge, affect the adsorption and conformation of FN (Osterlund, 1988; Kowalczynska et al., 2009; Lv et al., 2017; Gossart et al., 2018; Hasan et al., 2018); however, the influence of the SFE of an implant on the adsorption and conformation of FN, and the biological behavior of osteoblasts cultured on the FN-treated surface, remains to be elucidated. SFE is of fundamental importance when characterizing interactions between liquids and solids as it relates to adhesion, binding affinity, adsorption and interfacial attractive forces. To date, the most widely used method of determining SFE is by measuring the contact angle between the liquid and solid (Zhang et al., 2019). In this study, we exposed titanium disks to ultraviolet-C (UVC) light to evaluate the effects of changes in the SFE of titanium implants on the adsorption and conformation of FN and the biological behavior of cultured osteoblasts.
Materials and Methods
Specimens
Titanium disks were15 mm in diameter and 1 mm thick. Titanium disks were abraded with a sequence of successively finer silicon carbide papers; washed with acetone, anhydrous ethanol and dH2O for 15 min each; dried for 1 h at room temperature; etched in mixed acid solution (water: H2SO4:HCl 2:1:1 v/v); and washed 3 times with water. Disks were divided into two groups. The SFE of one group of titanium disks (high [H]-SFE) was increased by exposing the disks to a 30 W light tube that emitted UV light at wavelengths of 200–275 nm (Philips, Holland) for 24 h. The disks in the low (L)-SFE group received no treatment.
The surface morphology of the titanium disks was observed with a scanning electron microscope (SEM, Regululs8239, Hitachi, Japan).
Surface roughness of the titanium disks was measured with an optical surface profilometer (BMT EXPERT, BMT, Germany). The arithmetic mean roughness (Sa), and average height over the measurement field (Sz) were calculated as amplitude parameters to characterize surface topography.
Contact angles were measured with a contact angle meter (OCA40 Micro, dataphysics, Germany).
SFE was calculated using contact angle data and the Owens-Wendt model:
The Owens- Wendt model (Zhang et al., 2019) considers dispersion and polar contributions (e.g.,
The chemical composition of the titanium disks was confirmed with X-ray photoelectron spectroscopy (XPS, ESCALAB 250, Thermo-VG, America), using C1s at 284.8 eV) as the charge reference and by comparing the amount of surface hydroxyl (OH) groups.
Protein Adsorption and Secondary Structure Analysis
Human plasma FN (Sigma-Aldrich, MO, United States) was adsorbed onto titanium disks from a 1 mg/ml FN solution at 37°C for 3, 6, and 24 h. FN was desorbed from the titanium disks by shaking with 5% SDS in PBS at 37°C for 1 h. Protein was estimated using a BCA Protein Assay kit (MA0082, United States).
The secondary structure of adsorbed FN was investigated using attenuated total reflection-Fourier transform infrared (ATR-FTIR) (Nicolet 6700-Contiuµm, Thermo, England) spectroscopy, particularly in the amide I band IR absorption range, 1,600–1700 cm−1 (Buchanan and El-Ghannam, 2009). After FN adsorption for 24 h, titanium disks were dried at 37°C and ATR-FTIR spectra were acquired. The secondary structure of FN was determined by evaluating the amide I band through second derivative/Gaussian curve fitting analysis.
Effect of SFE of Titanium Disks on Osteoblast Behavior
Human plasma FN (Sigma) was adsorbed onto titanium disks from a 1 mg/ml FN solution at 37°C for 24 h prior to investigating osteoblast behavior.
Cell Culture
Human osteosarcoma cells (MG-63) were provided by Procell Life Science and Technology Co., Ltd. Cells were cultured in α-Minimum Essential Medium (α-MEM, Gibco, United States) supplemented with 10% fetal bovine serum (FBS, Gibco, United States) and 1% P/S (Gibco, United States) at 37°C and 5% CO2. Culture media was replaced every 2 days, and cells were passaged by trypsinization at 80% confluence.
Cell Adhesion and Proliferation
MG-63 cells were seeded at 1 × 104 cells/titanium disk, incubated for 3, 6 and 24 h, and rinsed 3 times with PBS to remove nonadhered cells. Cells were permeabilized with DAPI (P0131, Beyotime) for 30 min and visualized with an inverted fluorescent microscope (X50; DMi8, Leica, Germany). 10 fields of view per disk were captured to determine the mean number of adhered cells. Images were analyzed with ImageJ software.
1 × 104 MG-63 cells were seeded at 1 × 104 cells/titanium disk, incubated for 1, 3, 5, and 7 days, and culture media was removed. MG-63 proliferation was evaluated with a CCK-8 assay (CA1210, Solarbio) using absorbance at 450 nm (SpectraMax Plus384, MD, United States), according to the manufacturer’s instructions.
Alkaline Phosphatase Assay
Differentiation of osteoblasts was investigated by evaluating alkaline phosphatase (ALP) activity. ALP is a mineralization-related protein and an important marker of early osteoblast differentiation (Xu et al., 2015). MG-63 cells were seeded at 1×104 cells/titanium disk and cultured for 7 and 14 days. Culture media was removed and disks were rinsed 3 times with PBS. Cells were lysed with 200 μl cell lysis buffer (P0013J, Beyotime, China), and centrifuged at 14,000 g at 4°C for 5 min. ALP activity in the supernatant was determined with an ALP assay kit (P0321S, Beyotime, China) using absorbance at 405 nm, according to the manufacturer’s instructions.
Mineralization
Mineralization of osteoblasts was investigated with alizarin red staining. MG-63 cells were seeded at 1 × 104 cells/titanium disk and incubated for 14 days. Disks were rinsed 3 times in PBS, incubated with 1 ml alizarin red solution (G1450, Solarbio) for 30 min, rinsed with ddH2O, and observed under a light microscope (S9i, Leica, Germany). Images were analysed with ImageJ software.
Real-Time Reverse Transcription Polymerase Chain Reaction
To evaluate MG-63 cell adhesion, integrin-β1, integrin-α5, FAK, Src, Rac-1, and RhoA mRNA levels were analyzed by RT-PCR. MG-63 cells were cultured at 2 × 105 cells/disk for 3 and 6 h on FN-treated H-SFE and L-SFE titanium disks. Total RNA was isolated with a SteadyPure Universal RNA Extraction Kit (Accurate Biotechnology Co.,Ltd., AG21017, China). cDNA was synthesized using an Evo M-MLV RT Mix Kit (Accurate Biotechnology Co., Ltd., AG11728, China), according to the manufacturer’s instructions. Gene expression analysis was performed using a SYBR Green Premix Pro Tap HS qPCR Kit (Accurate Biotechnology Co., Ltd., AG11701, China) on an Real-Time PCR system (Loghtcycler 96, Roche, Switzerland). Sequence specific primers (Table 1) were purchased from Telenbiotech DNA Technologies (China). Differential gene expression was calculated using mRNA levels from MG-63 cells cultured on L-SFE titanium disks as the reference.
Statistical Analysis
Statistical analyses were performed with SPSS 19.0 (SPSS Inc., United States). All experiments were repeated in triplicate. Data are reported as means and standard deviations. Comparisons between H-SFE and L-SFE disks were performed with the independent samples t-test and nonparametric Mann-Whitney U test. p ≤ 0.05 was considered statistically significant.
Results
Surface Characteristics of H-SFE and L-SFE Titanium Disks
The surface characteristics of the H-SFE and L-SFE titanium disks are shown in Figure 1, Tables 2, 3. L-SFE (Figures 1A,B) and H-SFE (Figures 1C,D) titanium disks had pore sizes of 2–3 µm. There were no significant differences in amplitude parameters (Figures 1E,F) Sa or Sz (Table 2) between H-SFE and L-SFE titanium disks. The water contact angle (Figures 1G,I; Table 3) at room temperature was significantly higher for L-SFE compared to H-SFE titanium disks; there was no significant difference in the diiodomethane contact angle (Figures 1H,J; Table 3) at room temperature.
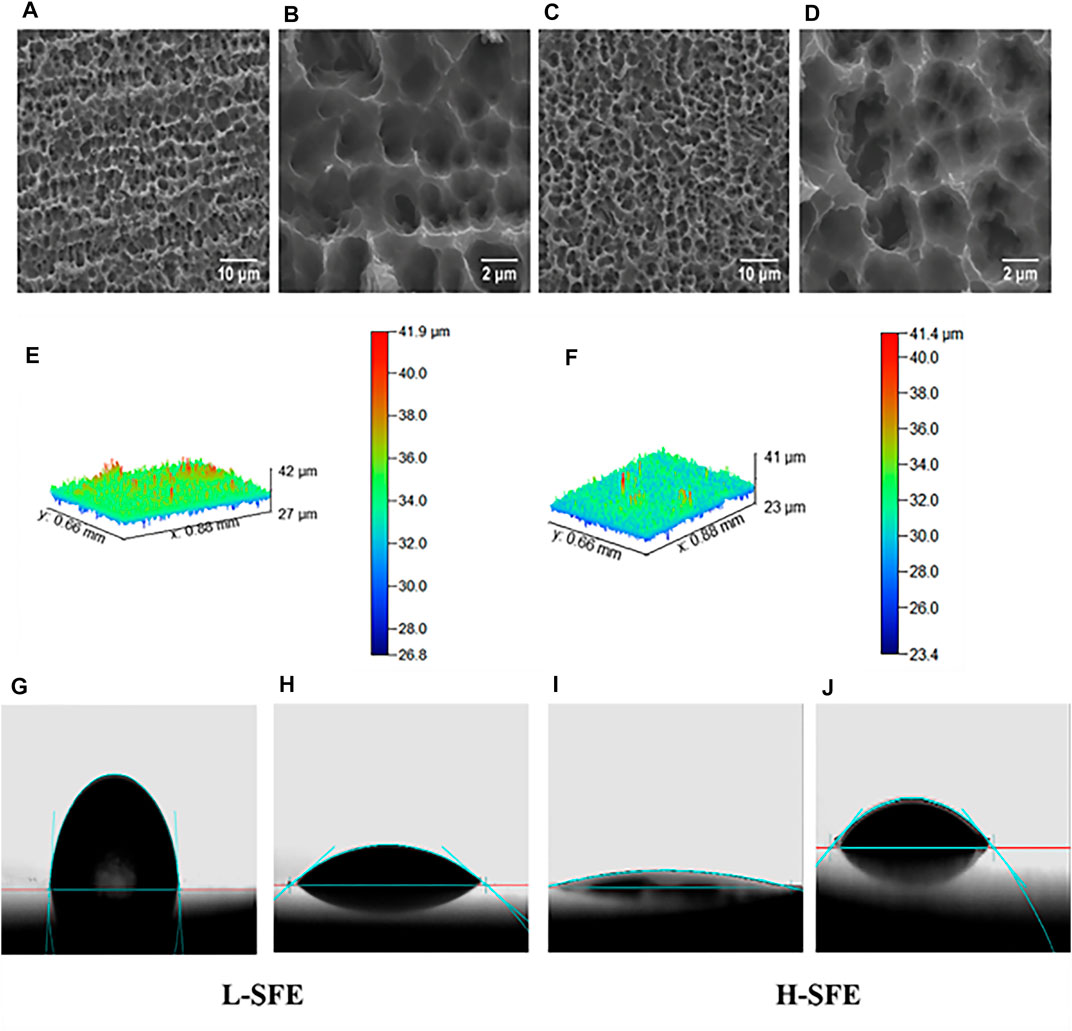
FIGURE 1. Surface characteristics of H-SFE and L-SFE titanium disks. Surface microstructures of L-SFE (A, B) and H-SFE (C, D) titanium disks; Optical Profiler images of the surface topography of L-SFE (E) and H-SFE (F) titanium disks; water (G, I) and CH2I2 (H, J) contact angles; (A, C) = ×2,000 magnification; (B, D) = ×10,000 magnification.
SFE was significantly higher for H-SFE compared to L-SFE titanium disks (p < 0.001; Table 3).
The surfaces of the L-SFE and H-SFE titanium disks had high percentages of C, O, N, Ti. The C1s and O1s XPS spectra for L-SFE and H-SFE titanium disks are shown in Figure 2.
Adsorbed FN
The FN adsorption capabilities of H-SFE and L-SFE titanium disks after immersion in a 1 mg/ml FN solution at 37°C for 3, 6, and 24 h are shown in Figure 3. FN adsorption levels were significantly increased on H-SFE compared to L-SFE titanium disks after immersion for 3 and 6 h, but there was no significant difference in FN adsorption level after immersion for 24 h (Figure 3A). ATR-FTIR spectra showed the ß-turn content in adsorbed FN was significantly increased on FN-treated H-SFE compared to L-SFE titanium disks (Figures 3B,C).
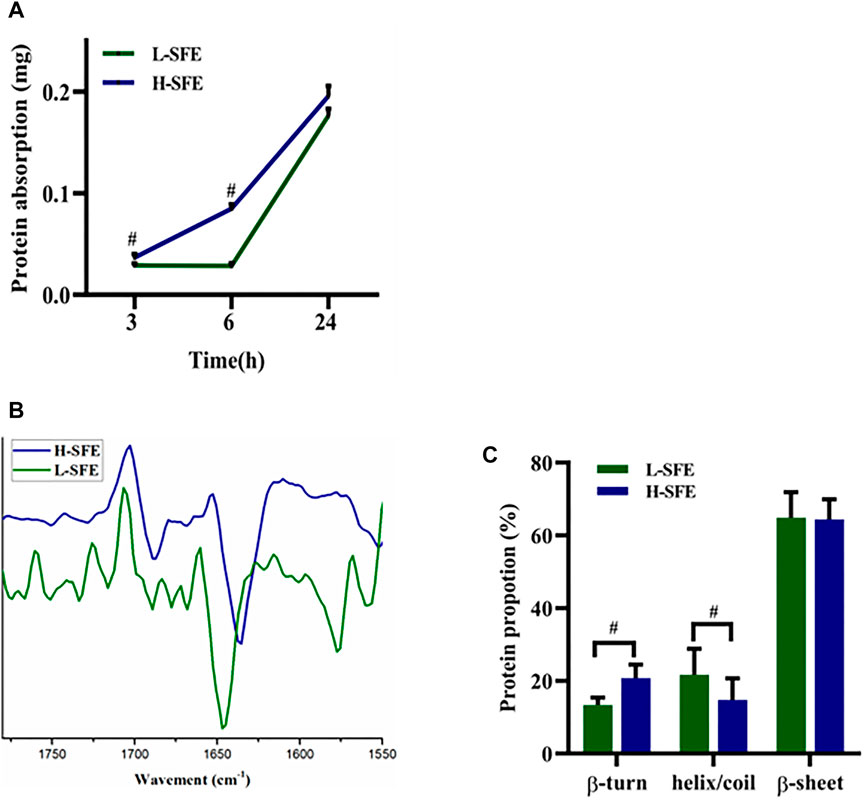
FIGURE 3. FN adsorption capabilities of H-SFE and L-SFE titanium disks. FN adsorption levels (A); ATR-FTIR spectra of the amide I band (1,600–1700 cm−1) (B); ß-turn content in adsorbed FN (C). #p ≤ 0.05.
Osteoblast Behavior
MG-63 cell adhesion on FN-treated H-SFE and L-SFE titanium disks after 3, 6, and 24 h of culture is shown in Figure 4A. The number of MG-63 cells adhered to FN-treated H-SFE and L-SFE titanium disks increased with time, with maximum MG-63 cell adhesion on FN-treated H-SFE titanium disks after 24 h of culture (Figure 4B).
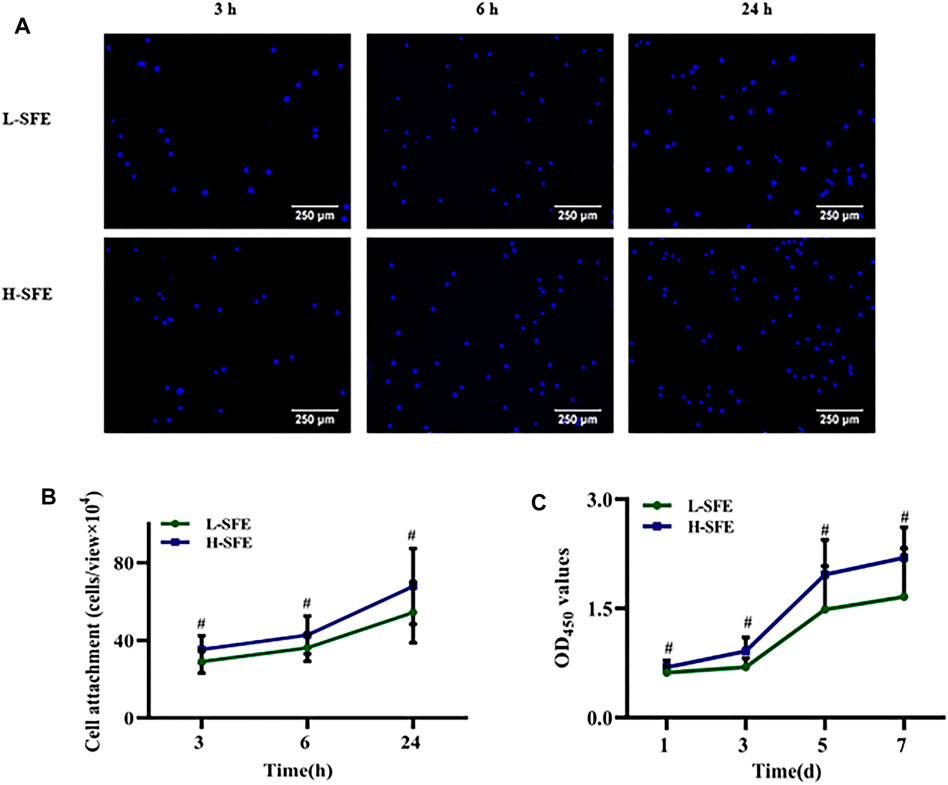
FIGURE 4. MG-63 cell adhesion (A, B) and proliferation (C) on FN-treated L-SFE and H-SFE titanium disks. #p ≤ 0.
MG-63 cell proliferation on FN-treated H-SFE and L-SFE titanium disks increased with time, with maximum MG-63 cell proliferation on FN-treated H-SFE titanium disks after 7 days of culture (Figure 4C).
MG-63 cell adhesion and proliferation were significantly greater in FN-treated H-SFE compared to L-SFE titanium disks at each time point.
ALP activity of MG-63 cells was significantly increased in FN-treated H-SFE compared to L-SFE titanium disks after 7 and 14 days of culture (Figure 5A). MG-63 cell mineralization was significantly increased in FN-treated H-SFE compared to L-SFE titanium disks after 14 days of culture (Figures 5B,C).
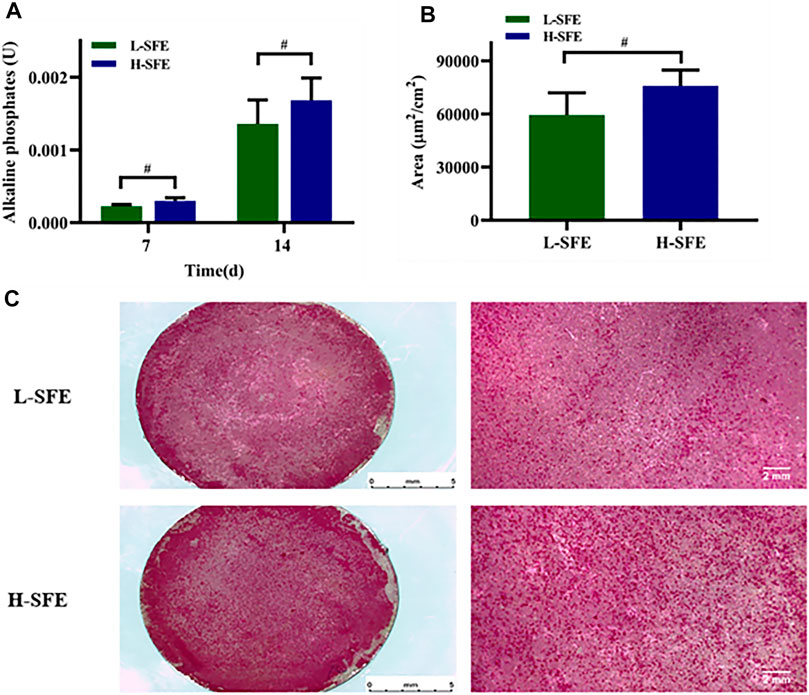
FIGURE 5. MG-63 cell ALP activity after 7 and 14 days in culture (A) and mineralization, depicted by alizarin red S stained calcium phosphate deposits, after 14 days in culture (B,C), on FN-treated L-SFE and H-SFE titanium disks. #p ≤ 0.05.
mRNA Levels of Cell Adhesion Molecules in Osteoblasts
Integrin-β1, integrin-α5, FAK, Src, Rac-1, and RhoA mRNA levels in MG-63 cells cultured on FN-treated H-SFE and L-SFE titanium disks are shown in Figure 6. Integrin-β1, integrin-α5, and Rac-1 mRNA levels were significantly higher in MG-63 cells on FN-treated H-SFE compared to L-SFE titanium disks after 3 h of culture, while there were no significant differences in FAK, Src, and RhoA mRNA levels (Figure 6A). Integrin-β1, integrin-α5, FAK, Src, and RhoA mRNA levels were significantly higher in MG-63 cells on FN-treated L-SFE compared to H-SFE titanium disks after 6 h of culture, while there was no significant difference in Rac-1 mRNA level (Figure 6B).
Discussion
In this study, we exposed titanium disks to UVC light to evaluate the effects of changes in the SFE of titanium implants on the adsorption and conformation of FN and the biological behavior of osteoblasts cultured on the FN-treated modified surfaces. Pure titanium quickly forms a nanometer thick layer of titanium oxide when exposed to air. Titanium oxide is a semiconductor and will undergo a photocatalytic reaction when irradiated with ultraviolet light (Sharmin and Ray, 2012; Khanna and Shetty, 2013; Assadi et al., 2014). This reaction will decompose hydrocarbons and water on the surface of H-SFE titanium disks to reduce C content and form a large number of hydroxyl groups (Diebold, 2003; Bikondoa et al., 2006; Sun et al., 2012). Therefore, our H-SFE titanium disks had a high SFE and hydrophilicity, consistent with the results of studies. Exposure to UV irradiation does not change the surface morphology and roughness of pure titanium, eliminating the influence of these surface characteristics on our findings.
As a component of the extracellular matrix, FN is involved in cell-implant interaction events. It can bind to integrins on the cell membrane, resulting in a conformational change in these proteins (Marconi et al., 2021). In our study, the FN adsorption capabilities of H-SFE and L-SFE titanium disks increased with time. FN adsorption levels were significantly increased on H-SFE compared to L-SFE titanium disks after titanium disks were immersed in a 1 mg/ml FN solution at 37°C for 3 and 6 h. This suggests high SFE can promote the early adsorption of FN on a pure titanium surface. Although there was no significant difference in FN adsorption level on H-SFE compared to L-SFE titanium disks after immersion for 24 h, the ß-turn content in FN was significantly increased on FN-treated H-SFE compared to L-SFE titanium disks. OH groups on titanium surfaces may react with the amino groups of an adsorbed protein through electrostatic interactions and cause a change in protein conformation (Hong et al., 2014). This may expose ß-turns. In the present study, XPS spectra showed the binding energy at 531.5 eV, the position expected for surface OH groups, was higher for H-SFE compared to L-SFE titanium disks, while ATR-FTIR spectra showed the ß-turn content in FN was significantly increased on FN-treated H-SFE compared to L-SFE titanium disks. These data suggest H-SFE titanium disks may adsorb FN faster than L-SFE titanium disks by optimizing ß-turn exposure.
FN interacts with integrins on the cell surface, arbitrates mechanical anchoring, and establishes focal adhesions between intracellular actin bundles and the extracellular matrix. Extracellular signals are translated into cellular responses at focal adhesions (Marconi et al., 2021). The biological behavior of osteoblasts in vitro can be used as a reference that reflects the biological activity of artificial materials. There was no significant difference in FN adsorption level on H-SFE compared to L-SFE titanium disks after immersion for 24 h, but osteogenic responses were significantly improved in MG-63 cells cultured on H-SFE compared to L-SFE titanium disks after 24 h FN adsorption. This implies that the osteogenic responses of MG-63 cells are related to protein adsorption level and the secondary structure of the adsorbed proteins. Protein adsorption is the first step in cell-biomaterial interactions (Hubbell et al., 2009; Petros and DeSimone, 2010; Scharnagl et al., 2010; Grafahrend et al., 2011). In vivo, proteins that are adsorbed onto an implant surface play a key role in cell/implant interactions (García et al., 1999; Hynes, 2002; Mao and Schwarzbauer, 2005; Roach et al., 2005; Vogel and Sheetz, 2006; Tsapikouni and Missirlis, 2008). We have shown that the physicochemical properties of the surface of a biomaterial impact the secondary structure of adsorbed proteins, and then impact cell adhesion and the behavior of attached cells (Toworfe et al., 2009; Kushiro et al., 2016). FN is a dimeric glycoprotein that promotes osteoblast adhesion, migration, and differentiation (Pankov and Yamada, 2002; Cantini et al., 2012). Unfolding of FN may expose binding sites (Felgueiras et al., 2014), such as the RGD sequence, which can be recognized by cell integrins (Shen et al., 2008). In FN, the RGD domain is located in a ß-turn structure, and increasing ß-turn content is associated with improved cell adhesion properties (Hasan et al., 2018). These data suggest that the higher SFE of H-SFE compared to L-SFE titanium disks may have induced changes in the conformation of adsorbed FN that enhanced the osteogenic activity of MG-63.
Integrins play key structural roles in cells as they are transmembrane proteins that engage with the extracellular matrix and regulate the organization of the actin cytoskeleton. In addition, a and ß integrins are important initiating and regulating factors in signal transduction (Juliano et al., 2004) and integrin-α5 and integrin-β1 recognize and connect to the RGD domain in FN, resulting in focal adhesions FAK and Src are key components of the signaling pathways controlling focal adhesions. Cytoplasmic tails (β1, β3, and β5) of ß integrin promote activation of FAK through an undefined mechanism (Toutant et al., 2002). Src can be activated by binding directly to the cytoplasmic domain of ß integrin (Arias-Salgado et al., 2003), and Src can also activate FAK through phosphorylation. Interactions between Src and FAK and integrin-mediated signaling pathways can activate the Rho family of small GTPases, which mediate the biological activities of cells (Turner, 2000; Wozniak et al., 2004; Nayal et al., 2006; Morgan et al., 2009; Tang et al., 2018; Rajah et al., 2019). Rac-1 regulates the formation of plate-shaped pseudopodia and promotes cell migration (Tang et al., 2018; Liu et al., 2020), while RhoA is involved in maintaining cell adhesions (Warner et al., 2019).
In the present study, the expression of some genes related to focal adhesions, and Rac-1, the gene related to cell migration, was significantly higher in MG-63 cells on FN-treated H-SFE compared to L-SFE titanium disks after 3 h of culture. After 6 h of culture, the expression of genes related to focal adhesions was significantly higher in MG-63 cells on FN-treated L-SFE compared to H-SFE titanium disks, while there was no significant difference in the expression of Rac-1. We speculate that MG-63 cells on FN-treated H-SFE titanium disks were migrating and developing focal adhesions after 3 h in culture, and proliferating and differentiating after 6 h in culture. In contrast, MG-63 cells on FN-treated L-SFE titanium disks were migrating and developing focal adhesions after 6 h in culture. These data suggest that increasing the SFE of titanium disks promotes conformational changes in FN and biological behavior in cultured osteoblasts.
Conclusion
In this study, titanium disks with high SFE were generated by UVC irradiation. There were no significant differences in surface characteristics such as pore size, Sa, or Sz between H-SFE and L-SFE titanium disks. MG-63 cells cultured on FN-treated H-SFE titanium disks showed better osteogenic responses, including adhesion, proliferation, ALP activity and mineralization, than MG-63 cells cultured on FN-treated L-SFE titanium disks. Although the underlying mechanisms remain to be elucidated, UVC irradiation may have increased the number of OH groups on the surface of FN-treated H-SFE titanium disks, which induced changes in the conformation of adsorbed FN, exposed RGD binding sites, and enhanced the biological activity of MG-63 cells.
Data Availability Statement
The raw data supporting the conclusions of this article will be made available by the authors, without undue reservation.
Author Contributions
JL and SL proposed and designed the experiments. JL and HD carried out the experiments with the help of YW and XZ. JL and HD drafted the article and interpreted the data. JL and SL revised the article. All the authors approved the final version of this article.
Funding
This work was financially supported by the National Natural Science Foundation of China (No. 81801008, Guangzhou, China), Natural Science Foundation of Xinjiang Uygur Autonomous Region (No. 2020D01C004, Xinjiang, China) and Foundation of Research and Cultivation Program of Stomatological Hospital of Southern Medical University (No. PY2021019, Guangzhou, China). Support was received from Medjaden Inc.
Conflict of Interest
The authors declare that the research was conducted in the absence of any commercial or financial relationships that could be construed as a potential conflict of interest.
Publisher’s Note
All claims expressed in this article are solely those of the authors and do not necessarily represent those of their affiliated organizations, or those of the publisher, the editors and the reviewers. Any product that may be evaluated in this article, or claim that may be made by its manufacturer, is not guaranteed or endorsed by the publisher.
Acknowledgments
The authors gratefully acknowledgment support from Medjaden Inc. for scientific editing. All the authors of this article report no financial relationships related to any companies or products mentioned in this study.
References
Arias-Salgado, E. G., Lizano, S., Sarkar, S., Brugge, J. S., Ginsberg, M. H., and Shattil, S. J. (2003). Src Kinase Activation by Direct Interaction with the Integrin Beta Cytoplasmic Domain. Proc. Natl. Acad. Sci. U S A. 100 (123), 13298–13302. doi:10.1073/pnas.2336149100
Asghari Sana, F., Çapkın Yurtsever, M., Kaynak Bayrak, G., Tunçay, E. Ö., Kiremitçi, A. S., and Gümüşderelioğlu, M. (2017). Spreading, Proliferation and Differentiation of Human Dental Pulp Stem Cells on Chitosan Scaffolds Immobilized with RGD or Fibronectin. Cytotechnology 69 (4), 617–630. doi:10.1007/s10616-017-0072-9
Assadi, A. A., Bouzaza, A., Wolbert, D., and Petit, P. (2014). Isovaleraldehyde Elimination by UV/TiO2 Photocatalysis: Comparative Study of the Process at Different Reactors Configurations and Scales. Environ. Sci. Pollut. Res. 21 (19), 11178–11188. doi:10.1007/s11356-014-2603-7
Barberi, J., and Spriano, S. (2021). Titanium and Protein Adsorption: An Overview of Mechanisms and Effects of Surface Features. Materials 14 (7), 1590. doi:10.3390/ma14071590
Bayrak, Ö., Ghahramanzadeh Asl, H., and Ak, A. (2020). Protein Adsorption, Cell Viability and Corrosion Properties of Ti6Al4V alloy Treated by Plasma Oxidation and Anodic Oxidation. Int. J. Miner Metall. Mater. 27 (9), 1269–1280. doi:10.1007/s12613-020-2020-5
Bikondoa, O., Pang, C. L., Ithnin, R., Muryn, C. A., Onishi, H., and Thornton, G. (2006). Direct Visualization of Defect-Mediated Dissociation of Water on TiO2(110). Nat. Mater 5 (3), 189–192. doi:10.1038/nmat1592
Buchanan, L. A., and El-Ghannam, A. (2009). Effect of Bioactive Glass Crystallization on the Conformation and Bioactivity of Adsorbed Proteins. J. Biomed. Mater. Res. 93, 537. doi:10.1002/jbm.a.32561
Cantini, M., Rico, P., Moratal, D., and Salmerón-Sánchez, M. (2012). Controlled Wettability, Same Chemistry: Biological Activity of Plasma-Polymerized Coatings. Soft Matter 8 (20), 5575. doi:10.1039/c2sm25413a
Chen, S., Guo, Y., Liu, R., Wu, S., Fang, J., Huang, B., et al. (2018). Tuning Surface Properties of Bone Biomaterials to Manipulate Osteoblastic Cell Adhesion and the Signaling Pathways for the Enhancement of Early Osseointegration. Colloids Surf. B Biointerfaces 164, 58–69. doi:10.1016/j.colsurfb.2018.01.022
Diebold, U. (2003). The Surface Science of Titanium Dioxide. Surf. Sci. Rep. 48 (5-8), 53–229. doi:10.1016/s0167-5729(02)00100-0
Felgueiras, H. P., Sommerfeld, S. D., Murthy, N. S., Kohn, J., and Migonney, V. (2014). Poly(NaSS) Functionalization Modulates the Conformation of Fibronectin and Collagen Type I to Enhance Osteoblastic Cell Attachment onto Ti6Al4V. Langmuir 30 (31), 9477–9483. doi:10.1021/la501862f
García, A. J., Vega, M. D., and Boettiger, D. (1999). Modulation of Cell Proliferation and Differentiation through Substrate-dependent Changes in Fibronectin Conformation. Mol. Biol. Cel 10 (3), 785–798.
Gossart, A., Battiston, K. G., Gand, A., Pauthe, E., and Santerre, J. P. (2018). Mono vs Multilayer Fibronectin Coatings on Polar/hydrophobic/ionic Polyurethanes: Altering Surface Interactions with Human Monocytes. Acta Biomater. 66, 129–140. doi:10.1016/j.actbio.2017.11.013
Grafahrend, D., Heffels, K.-H., Beer, M. V., Gasteier, P., Möller, M., Boehm, G., et al. (2011). Degradable Polyester Scaffolds with Controlled Surface Chemistry Combining Minimal Protein Adsorption with Specific Bioactivation. Nat. Mater 10 (1), 67–73. doi:10.1038/nmat2904
Hasan, A., Saxena, V., and Pandey, L. M. (2018). Surface Functionalization of Ti6Al4V via Self-Assembled Monolayers for Improved Protein Adsorption and Fibroblast Adhesion. Langmuir 34 (11), 3494–3506. doi:10.1021/acs.langmuir.7b03152
Hong, Y., Yu, M., Lin, J., Cheng, K., Weng, W., and Wang, H. (2014). Surface Hydroxyl Groups Direct Cellular Response on Amorphous and Anatase TiO2 Nanodots. Colloids Surf. B Biointerfaces 123, 68–74. doi:10.1016/j.colsurfb.2014.08.030
Hubbell, J. A., Thomas, S. N., and Swartz, M. A. (2009). Materials Engineering for Immunomodulation. Nature 462 (7272), 449–460. doi:10.1038/nature08604
Hynes, R. O. (2002). Integrins: Bidirectional, Allosteric Signaling Machines. Cell 110 (6), 673–687. doi:10.1016/s0092-8674(02)00971-6
Isoshima, K., Ueno, T., Arai, Y., Saito, H., Chen, P., Tsutsumi, Y., et al. (2019). The Change of Surface Charge by Lithium Ion Coating Enhances Protein Adsorption on Titanium. J. Mech. Behav. Biomed. Mater. 100, 103393. doi:10.1016/j.jmbbm.2019.103393
Juliano, R. L., Reddig, P., Alahari, S., Edin, M., Howe, A., and Aplin, A. (2004). Integrin Regulation of Cell Signalling and Motility. Biochem. Soc. Trans. 32 (33), 443–446. doi:10.1042/bst0320443
Keselowsky, B. G., Collard, D. M., and García, A. J. (2003). Surface Chemistry Modulates Fibronectin Conformation and Directs Integrin Binding and Specificity to Control Cell Adhesion. J. Biomed. Mater. Res. 66A (2), 247–259. doi:10.1002/jbm.a.10537
Khanna, A., and Shetty, K. V. (2013). Solar Photocatalysis for Treatment of Acid Yellow-17 (AY-17) Dye Contaminated Water Using Ag@TiO2 Core-Shell Structured Nanoparticles. Environ. Sci. Pollut. Res. 20 (8), 5692–5707. doi:10.1007/s11356-013-1582-4
Kowalczyńska, H. M., Kołos, R., Nowak-Wyrzykowska, M., Dobkowski, J., Elbaum, D., Szczepankiewicz, A., et al. (2009). Atomic Force Microscopy Evidence for Conformational Changes of Fibronectin Adsorbed on Unmodified and Sulfonated Polystyrene Surfaces. J. Biomed. Mater. Res. 91A (4), 1239–1251. doi:10.1002/jbm.a.32473
Kushiro, K., Lee, C.-H., and Takai, M. (2016). Simultaneous Characterization of Protein-Material and Cell-Protein Interactions Using Dynamic QCM-D Analysis on SAM Surfaces. Biomater. Sci. 4 (6), 989–997. doi:10.1039/c5bm00613a
Lin, D.-J., Fuh, L.-J., and Chen, W.-C. (2020). Nano-morphology, Crystallinity and Surface Potential of Anatase on Micro-arc Oxidized Titanium Affect its Protein Adsorption, Cell Proliferation and Cell Differentiation. Mater. Sci. Eng. C 107, 110204. doi:10.1016/j.msec.2019.110204
Liu, M., Banerjee, R., Rossa, C., and D’Silva, N. J. (2020). RAP1-RAC1 Signaling Has an Important Role in Adhesion and Migration in HNSCC. J. Dent. Res. 99 (8), 959–968. doi:10.1177/0022034520917058
Lv, L., Li, K., Xie, Y., Cao, Y., and Zheng, X. (2017). Enhanced Osteogenic Activity of Anatase TiO2 Film: Surface Hydroxyl Groups Induce Conformational Changes in Fibronectin. Mater. Sci. Eng. C 78, 96–104. doi:10.1016/j.msec.2017.04.056
Mao, Y., and Schwarzbauer, J. E. (2005). Fibronectin Fibrillogenesis, a Cell-Mediated Matrix Assembly Process. Matrix Biol. 24 (6), 389–399. doi:10.1016/j.matbio.2005.06.008
Marconi, G. D., Fonticoli, L., Della Rocca, Y., Oliva, S., Rajan, T. S., Trubiani, O., et al. (2021). Enhanced Extracellular Matrix Deposition on Titanium Implant Surfaces: Cellular and Molecular Evidences. Biomedicines 9 (11), 1710. doi:10.3390/biomedicines9111710
Maurer, L. M., Ma, W., and Mosher, D. F. (2016). Dynamic Structure of Plasma Fibronectin. Crit. Rev. Biochem. Mol. Biol. 51 (4), 213–227. doi:10.1080/10409238.2016.1184224
Morgan, M. R., Byron, A., Humphries, M. J., and Bass, M. D. (2009). Giving off Mixed Signals-Distinct Functions of α5β1and αvβ3integrins in Regulating Cell Behaviour. IUBMB Life 61 (7), 731–738. doi:10.1002/iub.200
Nayal, A., Webb, D. J., Brown, C. M., Schaefer, E. M., Vicente-Manzanares, M., and Horwitz, A. R. (2006). Paxillin Phosphorylation at Ser273 Localizes a GIT1-PIX-PAK Complex and Regulates Adhesion and Protrusion Dynamics. J. Cel Biol. 173 (4), 587–589. doi:10.1083/jcb.200509075
Osterlund, E. (1988). The Secondary Structure of Human Plasma Fibronectin: Conformational Changes Induced by Acidic pH and Elevated Temperatures; a Circular Dichroic Study. Biochim. Biophys. Acta 955 (3), 330–336.
Pankov, R., and Yamada, K. M. (2002). Fibronectin at a Glance. J. Cel Sci. 115 (Pt 20), 3861–3863. doi:10.1242/jcs.00059
Petros, R. A., and DeSimone, J. M. (2010). Strategies in the Design of Nanoparticles for Therapeutic Applications. Nat. Rev. Drug Discov. 9 (8), 615–627. doi:10.1038/nrd2591
Rajah, A., Boudreau, C. G., Ilie, A., Wee, T.-L., Tang, K., Borisov, A. Z., et al. (2019). Paxillin S273 Phosphorylation Regulates Adhesion Dynamics and Cell Migration through a Common Protein Complex with PAK1 and βPIX. Sci. Rep. 9 (1), 11430. doi:10.1038/s41598-019-47722-3
Raphel, J., Karlsson, J., Galli, S., Wennerberg, A., Lindsay, C., Haugh, M. G., et al. (2016). Engineered Protein Coatings to Improve the Osseointegration of Dental and Orthopaedic Implants. Biomaterials 83, 269–282. doi:10.1016/j.biomaterials.2015.12.030
Rapuano, B. E., Lee, J. J. E., and MacDonald, D. E. (2012). Titanium alloy Surface Oxide Modulates the Conformation of Adsorbed Fibronectin to Enhance its Binding to α5β1 Integrins in Osteoblasts. Eur. J. Oral Sci. 120 (3), 185–194. doi:10.1111/j.1600-0722.2012.954.x
Roach, P., Farrar, D., and Perry, C. C. (2005). Interpretation of Protein Adsorption: Surface-Induced Conformational Changes. J. Am. Chem. Soc. 127 (22), 8168–8173. doi:10.1021/ja042898o
Rupp, F., Haupt, M., Klostermann, H., Kim, H. S., Eichler, M., Peetsch, A., et al. (2010). Multifunctional Nature of UV-Irradiated Nanocrystalline Anatase Thin Films for Biomedical Applications. Acta Biomater. 6 (12), 4566–4577. doi:10.1016/j.actbio.2010.06.021
Scharnagl, N., Lee, S., Hiebl, B., Sisson, A., and Lendlein, A. (2010). Design Principles for Polymers as Substratum for Adherent Cells. J. Mater. Chem. 20 (40), 8789. doi:10.1039/c0jm00997k
Sharmin, R., and Ray, M. B. (2012). Application of Ultraviolet Light-Emitting Diode Photocatalysis to Remove Volatile Organic Compounds from Indoor Air. J. Air Waste Manage. Assoc. 62 (9), 1032–1039. doi:10.1080/10962247.2012.695760
Shen, J.-W., Wu, T., Wang, Q., and Pan, H.-H. (2008). Molecular Simulation of Protein Adsorption and Desorption on Hydroxyapatite Surfaces. Biomaterials 29 (5), 513–532. doi:10.1016/j.biomaterials.2007.10.016
Smeets, R., Stadlinger, B., Schwarz, F., Beck-Broichsitter, B., Jung, O., Precht, C., et al. (2016). Impact of Dental Implant Surface Modifications on Osseointegration. Biomed. Res. Int. 2016, 1–16. doi:10.1155/2016/6285620
Sun, C., Liao, T., Lu, G. Q., and Smith, S. C. (2012). The Role of Atomic Vacancy on Water Dissociation over Titanium Dioxide Nanosheet: A Density Functional Theory Study. J. Phys. Chem. C 116 (3), 2477–2482. doi:10.1021/jp208951p
Tang, K., Boudreau, C. G., Brown, C. M., and Khadra, A. (2018). Paxillin Phosphorylation at Serine 273 and its Effects on Rac, Rho and Adhesion Dynamics. Plos Comput. Biol. 14 (7), e1006303. doi:10.1371/journal.pcbi.1006303
Toutant, M., Costa, A., Studler, J.-M., Kadaré, G., Carnaud, M., and Girault, J.-A. (2002). Alternative Splicing Controls the Mechanisms of FAK Autophosphorylation. Mol. Cel Biol. 22 (22), 7731–7743. doi:10.1128/MCB.22.22.7731-7743.2002
Toworfe, G. K., Bhattacharyya, S., Composto, R. J., Adams, C. S., Shapiro, I. M., and Ducheyne, P. (2009). Effect of Functional End Groups of Silane Self-Assembled Monolayer Surfaces on Apatite Formation, Fibronectin Adsorption and Osteoblast Cell Function. J. Tissue Eng. Regen. Med. 3 (1), 26–36. doi:10.1002/term.131
Tsapikouni, T. S., and Missirlis, Y. F. (2008). Protein-material Interactions: From Micro-to-nano Scale. Mater. Sci. Eng. B 152 (1-3), 2–7. doi:10.1016/j.mseb.2008.06.007
Turner, C. E. (2000). Paxillin and Focal Adhesion Signalling. Nat. Cel Biol. 2 (12), E231–E236. doi:10.1038/35046659
Vogel, V., and Sheetz, M. (2006). Local Force and Geometry Sensing Regulate Cell Functions. Nat. Rev. Mol. Cel Biol. 7 (4), 265–275. doi:10.1038/nrm1890
Warner, H., Wilson, B. J., and Caswell, P. T. (2019). Control of Adhesion and Protrusion in Cell Migration by Rho GTPases. Curr. Opin. Cel Biol. 56, 64–70. doi:10.1016/j.ceb.2018.09.003
Wozniak, M. A., Modzelewska, K., Kwong, L., and Keely, P. J. (2004). Focal Adhesion Regulation of Cell Behavior. Biochim. Biophys. Acta Mol. Cel Res. 1692 (2-3), 103–119. doi:10.1016/j.bbamcr.2004.04.007
Xu, G., Shen, X., Hu, Y., Ma, P., and Cai, K. (2015). Fabrication of Tantalum Oxide Layers onto Titanium Substrates for Improved Corrosion Resistance and Cytocompatibility. Surf. Coat. Techn. 272, 58–65. doi:10.1016/j.surfcoat.2015.04.024
Yao, Y.-t., Liu, S., Swain, M. V., Zhang, X.-p., Zhao, K., and Jian, Y.-t. (2019). Effects of Acid-Alkali Treatment on Bioactivity and Osteoinduction of Porous Titanium: An In Vitro Study. Mater. Sci. Eng. C 94, 200–210. doi:10.1016/j.msec.2018.08.056
Zeng, Y., Yang, Y., Chen, L., Yin, D., Zhang, H., Tashiro, Y., et al. (2019). Optimized Surface Characteristics and Enhanced In Vivo Osseointegration of Alkali-Treated Titanium with Nanonetwork Structures. Int. J. Mol. Sci. 20 (5), 1127. doi:10.3390/ijms20051127
Keywords: conformation, fibronectin, osteoblast, titanium, surface free energy
Citation: Lin J, Dong H, Wen Y, Zhuang X and Li S (2022) Surface Free Energy of Titanium Disks Enhances Osteoblast Activity by Affecting the Conformation of Adsorbed Fibronectin. Front. Mater. 9:840813. doi: 10.3389/fmats.2022.840813
Received: 21 December 2021; Accepted: 08 February 2022;
Published: 07 March 2022.
Edited by:
Michel Assad, Charles River Laboratories, CanadaReviewed by:
Takao Hanawa, Tokyo Medical and Dental University, JapanGuya Diletta Marconi, University of Studies G. d’Annunzio Chieti and Pescara, Italy
Wei-Jen Chang, Taipei Medical University, Taiwan
Copyright © 2022 Lin, Dong, Wen, Zhuang and Li. This is an open-access article distributed under the terms of the Creative Commons Attribution License (CC BY). The use, distribution or reproduction in other forums is permitted, provided the original author(s) and the copyright owner(s) are credited and that the original publication in this journal is cited, in accordance with accepted academic practice. No use, distribution or reproduction is permitted which does not comply with these terms.
*Correspondence: Shaobing Li, issaclee@163.com