- 1School of Science, Mae Fah Luang University, Chiang Rai, Thailand
- 2National Nanotechnology Center, National Science and Technology Development Agency, Pathum Thani, Thailand
- 3Center of Chemical Innovation for Sustainability, Mae Fah Luang University, Chiang Rai, Thailand
The potential of a drug delivery system of the longan seed extract (LSE) incorporated in the alginate/chitosan (Alg/CS) beads has been studied. The LSE-loaded Alg/CS beads were prepared using the ionic gelation method via the interaction between protonated amino groups of CS and negatively charged carboxylic groups of Alg. Properties of the LSE-loaded Alg/CS beads were investigated including the morphology of the beads, particle sizes, encapsulation efficiency (%EE), controlled release profile, cytotoxicity, and biocompatibility. From the results, the amount of gallic acid, ellagic acid, and corilagin found in LSE was 25.61 ± 0.48,18.83 ± 3.75, and 21.92 ± 1.42 mg/g (based on the weight of LSE), respectively. The half-maximum inhibitory concentration (IC50) of LSE was 24.29 ± 1.08 μg/ml. SEM images of the LSE-loaded Alg/CS beads showed spherical shapes and rough surfaces with some aggregation. The particle sizes were between 1.9 and 2.5 µm with the PDI values of 0.1503–0.3183. Encapsulation efficiencies were between 11 and 18%. The released amount of LSE from the LSE-loaded Alg/CS beads was ranging between 68 and 93%. Moreover, cytotoxicity and biocompatibility tests showed that the beads were non-toxic to both NCTC clone 929 and NHDF cells and promoted the attachment of NHDF cells. Thus, these beads could be used as polymeric drug carriers.
Introduction
Polymeric carriers have been developed to control drug levels within the desired range and prolong the contact time to the specific body site. They play an important role in controlling drug delivery systems for pharmaceutical and medical applications because of their efficient carrier characteristics such as sustained, controlled, and prolonged release and reduced drug toxicity (Gagliardi et al., 2012; George et al., 2019; Venditti, 2019). Moreover, they have the ability to protect the active compound or drug from degradation.
The main properties of polymeric carriers suitable for drug delivery systems are biocompatibility, biodegradability, and non-toxicity. For that reason, alginate (Alg) and chitosan (CS) are popular materials (Kumari et al., 2010; Sun et al., 2009). Alg has numerous uses in pharmaceutical and medical applications due to its non-immunogenicity, affordability, and absorption of wound exudate (Lee and Mooney 2012; Sarei et al., 2013). CS is a cationic polysaccharide that is derived from the deacetylation of chitin. It is composed of N-acetyl-d-glucosamine linked by β-(1-4) glycosidic bonds. Many research studies illustrated CS as polymeric carriers and reported that CS has the ability to control the release of active agents with antibacterial and hemostatic properties (Bernkop-Schnürch and Dünnhaupt 2012; Ji et al., 2011; Nguyen et al., 2017; Sinha et al., 2004).
Alg and CS can be formulated into the form of beads, which provide more benefits to controlled drug delivery systems. The Alg/CS beads could prevent rapid diffusion of the encapsulated drug in acid conditions and delay the degradation of drugs from oxidation, enzymatic degradation, and hydrolysis (Morsi et al., 2015; Nalini et al., 2019). Moreover, they could improve the stability, drug encapsulation efficiency, and the release of drug (Rajendran and Basu, 2009; Nagarwal et al., 2012). Alg and CS were used to fabricate the polymeric carriers using an ionic gelation method. This technique is a simple and mild process without the need for high temperature (De Pinho Neves et al., 2014). The attraction between protonated amino groups of CS and the negatively charged carboxylic groups of Alg creates the electrostatic interaction in the Alg/CS beads (Ahdyani et al., 2019; Nagarwal et al., 2012; Rahaiee et al., 2015; Rajendran and Basu, 2009). Rajendran and Basu prepared the nimodipine-loaded Alg/CS beads using the ionic gelation method for sustained drug release. The results showed that the nimodipine-loaded Alg/CS beads exhibited sustained drug release (Rajendran and Basu, 2009). Rahaiee et al. improved the stability of crocin by encapsulating in Alg/CS beads using a modified ionic gelation method. The results showed that Alg and CS biopolymers were the highly promising carriers for the delivery of crocin (Rahaiee et al., 2015). Moreover, Song et al. prepared Alg and CS nanoparticles loaded with curcumin. The results showed that Alg and CS nanoparticles exhibited the sustained release profiles and enhanced the uptake efficiency and cytotoxicity to cancer cells (Song et al., 2018). Thus, the Alg/CS beads have the potential for use as drug carriers.
Longan (Dimocarpus longan Lour.) is widely grown in Southeast Asia, China, and Taiwan. Chinese medicinal formulation uses longan for medication as an agent in the relief of neural pain (Yang et al., 2011). Due to the high content of polyphenolic compounds mainly corilagin, gallic acid, and ellagic acid (Worasuttayangkurn et al., 2012; Zhang et al., 2020), the longan seed extract (LSE) has several biological activities such as antioxidant ability, anti-tyrosinase, and anticancer activities (Lim 2013). Corilagin showed good antimicrobial, antitumor, and antioxidant activities. Gallic acid and ellagic acid were reported to be potent antioxidant and anticarcinogenic agents (Li et al., 2018; Tang et al., 2019).
This study aimed to fabricate the LSE-loaded Alg/CS beads for use as the drug delivery system. The LSE-loaded Alg/CS beads were prepared using the ionic gelation method. Various stirring methods, including the magnetic stirrer, homogenizer, and ultrasonicator, were employed for preparing the beads. High-performance liquid chromatography (HPLC) was used to determine the chemical compounds in LSE (i.e., gallic acid, ellagic acid, and corilagin). The antioxidant activity of LSE was investigated by 2,2-diphenyl-1-picrylhydrazyl (DPPH) assay. The LSE-loaded Alg/CS beads were characterized for their morphology, particle size, polydispersity index (PDI), and encapsulation efficiency (%EE). The release profiles of LSE from the LSE-loaded Alg/CS beads, cytotoxicity, and biocompatibility were also investigated.
Materials and Methods
Materials
Materials for the polymeric carrier, alginate acid sodium salt (viscosity: 15-25 cP), and chitosan (Mw = 20–30 kDa, degree of deacetylation ≥95%) were obtained from Sigma-Aldrich (United States) and Bio 21 Co., Ltd. (Thailand), respectively. 2,2-Diphenyl-1-picrylhydrazyl (DPPH) and Span 80 (viscosity: 1,000–200 mPa s) were purchased from Sigma-Aldrich (United States). l-Ascorbic acid was bought from Chem-Supply Pty Ltd. (Australia). Glacial acetic acid was bought from Merck KGaA (Germany). Ethanol, methanol, propan-2-ol, and dimethyl sulfoxide were purchased from RCI LabScan Limited (Bangkok, Thailand). Anhydrous sodium carbonate (Na2CO3), sodium chloride (NaCl), anhydrous disodium hydrogen orthophosphate (Na2HPO4), and paraffin oil were purchased from Ajax Finechem (Australia).
Methods
Extraction of Longan Seed
Edor cultivar longan seeds from a farm in Lamphun Province, Thailand, were selected for this study. The extraction procedures were carried out following Pankongadisak and Suwantong’s method with slight modification (Pankongadisak and Suwantong 2018). The dried longan seeds (approximately 10 g) were ground using mortar and pestle and then soaked in 600 ml of distilled water. The extraction process was carried out at room temperature for 72 h. After that, the mixture was filtered using a filter paper (Whatman No. 1). The extracted solution was then dried using lyophilization. Finally, the longan seed extract (LSE) was obtained and stored in a desiccator before use.
High-Performance Liquid Chromatography (HPLC) Analysis
The amounts of gallic acid, corilagin, and ellagic acid in LSE were analyzed using the HPLC technique. HPLC analysis was performed using a Waters Acquity Arc HPLC System. The chromatographic conditions were as follows: a column (Cortecs®C18 2.7 µm 4.6 × 50 mm, Waters); 0.1% v/v formic acid in Milli-Q water (solvent A), and acetonitrile (solvent B) for gradient elution were used as a mobile phase; and a total run time is 10 min. The sample injection volume was 1 µl/injection at a flow rate of 1.0 ml/min with a PDA detector-type HPLC 2998 detector used at a wavelength of 270 nm.
LSE solutions were prepared by dissolving LSE powder in Milli-Q water. The sample was filtered using a 0.22-µm membrane filter before injection. The standard solutions with concentrations varied as 25, 50, 100, 150, 200, 250, and 300 ppm were used to evaluate the amounts of gallic acid, ellagic acid, and corilagin in LSE. Quantitative analysis of polyphenolic compounds of gallic acid, ellagic acid, and corilagin was conducted by evaluating the peak area based on a standard curve.
Antioxidant Activity of Longan Seed Extract
The antioxidant activity of LSE was analyzed by DPPH assay according to the method of Blois with some modifications (Blois 1958). First, the test solutions were prepared by dissolving LSE powder in distilled water to make various concentrations as 3.125, 6.25, 12.5, 25, and 50 μg/ml. l-Ascorbic acid was used as a standard compound, and the solutions were prepared by using distilled water to make the same concentrations as LSE test solutions. Next, 50 µl of each solution was mixed with 150 µl of 100 µM DPPH solution. After that, the reaction mixture was kept in the dark at room temperature for 30 min. The antioxidant activity of LSE and l-ascorbic acid against DPPH radicals was determined by measuring the absorbance at 517 nm using a microplate reader (BioTek Instruments, United States). The percentage antioxidant activity (%AA) was calculated according to the following equation:
where Acontrol and Asample are the absorbance values of the testing solution without and with LSE or l-ascorbic acid, respectively.
Indirect Cytotoxicity of Longan Seed Extract
The indirect cytotoxicity of LSE was investigated using NCTC clone 929 and normal human dermal fibroblast (NHDF) cells. NCTC clone 929 (16th passage) and NHDF (16th passage) cells were cultured at 37°C in a humidified atmosphere of 95% air and 5% CO2 using Dulbecco’s modified Eagle medium (DMEM; GIBCO, United States). The DMEM contains 10% fetal bovine serum (FBS; GIBCO, United States) and 1% antibiotic and antimycotic formulation (GIBCO, United States). LSE powder was dissolved in a serum-free medium (SFM; containing DMEM and 1% antibiotic agent) to produce various concentration solutions as 5, 2.5, 1.25, 0.625, 0.313, 0.156, 0.078, 0.039, and 0.02 mg/ml. The LSE solutions were sterilized before testing by 0.22-μm Minisart syringe filters (Sartorius, Germany). Four replicates were performed in 96-well tissue culture plates (TCPS; SPL Life Science, Korea). The plates were seeded with cell suspension at 8,000 cells/well and incubated for 24 h at a 37°C humidified incubator with 5% CO2. Then, the cells were starved with SFM for 24 h. After 24 h, the medium was changed to LSE solution, and the cells were re-incubated for 24 h. Then 3-(4,5-dimethylthiazol-2-yl)-2,5-diphenyltetrazolium bromide (MTT; AMRESCO, United States) assay was used to determine the viability of cells cultured with LSE solution. The assay was carried out in triplicate (n = 3) with an Epoch Microplate Spectrophotometer (BioTek Instruments, United States) at the wavelength of 570 nm.
Preparation of LSE-Loaded Alg/CS Beads
The LSE-loaded Alg/CS beads were prepared by using the ionic gelation method. First, 4.5% w/v of Alg solution was prepared by dissolving 0.225 g of Alg in 5 ml of distilled water at room temperature. After that, 0.2 g (2% w/w, based on the weight of Alg and CS powder) of LSE was added to the Alg solution with stirring. The mixture (aqueous phase) was added dropwise into an oil phase that contained 25 ml of paraffin oil and 1.25 ml of Span 80 with a stirring speed of 1,200 rpm for 30 min. Then, 1% w/v of chitosan solution was prepared by dissolving chitosan in 1% v/v of acetic acid at room temperature for 24 h. After that, the LSE-loaded Alg/CS beads were obtained by adding 1% w/v CS solution (pH of 4) drop by drop to the mixture at different stirring conditions (see Table 1). A total of 10% w/v of CaCl2 solution was then added to the mixture with stirring at room temperature for 2 h. Then 10 ml of propan-2-ol was added to harden the beads. The LSE-loaded Alg/CS beads were collected by centrifugation at room temperature and subsequently washed several times with propan-2-ol and distilled water, respectively. The LSE-loaded Alg/CS beads were lyophilized for 1 day. Finally, the LSE-loaded Alg/CS beads were collected and stored in a desiccator before use.
Morphology and Particle Size of LSE-Loaded Alg/CS Beads
Morphology characterization of the LSE-loaded Alg/CS beads was carried out using an LEO 1450 VP Scanning Electron Microscope (SEM) with an accelerating voltage of 20 kV. The dry beads were deposited on a thin aluminum plate and then coated with a thin layer of gold. The SEM images of the LSE-loaded Alg/CS beads were obtained.
The particle size and PDI of the LSE-loaded Alg/CS beads were determined by dynamic light scattering (DLS) using a particle size analyzer (Zetasizer nano series, Malvern instrument, United Kingdom). The LSE-loaded Alg/CS bead diameter was determined after the dispersion of beads with distilled water in the ultrasonic bath for 2 min. The results were reported as the mean value and performed in triplicate.
Fourier Transform Infrared Spectroscopy (FTIR)
The LSE-loaded Alg/CS beads were mixed with KBr and prepared as a pellet to identify the structure of the LSE-loaded Alg/CS beads by FTIR (PerkinElmer, United States) in the range of 4,000–400 cm−1, with a resolution of 32 cm−1. The spectra are shown in Figure 4.
Encapsulation Efficiency of LSE-Loaded Alg/CS Beads
The LSE-loaded Alg/CS beads were first dissolved in phosphate-buffered solution (PBS) at 37°C for 1 day. The amount of LSE in PBS was determined by a UV–Vis spectrophotometer at the wavelength of 277 nm. The amounts of LSE in the beads were calculated using a calibration curve. The encapsulation efficiency (%EE) was calculated following Eq. 2.
Release Study
The LSE release profiles from the LSE-loaded Alg/CS beads were analyzed by using the total immersion method at 37°C for two days. Using a dialysis bag (molecular weight cut-off 12,000–14,000), the LSE/Alg/CS beads were loaded in a bag followed by 10 ml of PBS. The dialysis bag was then immersed in 20 ml of PBS. The release profiles were performed by collecting the sample solution at a specified time. Once the sample solution was collected, the same amount of fresh PBS was added. The UV–Vis spectrophotometer (Perkin-Elmer, United States) was used to analyze the amount of LSE released. The released amount of LSE was calculated using the calibration curve of LSE at 277 nm.
Indirect Cytotoxicity of LSE-Loaded Alg/CS Beads
NCTC clone 929 (14th passage) and NHDF (13th passage) cells were cultured at 8,000 cells/well in DMEM containing 10% FBS and 1% antibiotic and antimycotic formulation at 37°C in a humidified atmosphere of 95% air and 5% CO2 for 24 h to enable cell attachment. The LSE-loaded Alg/CS beads were immersed in SFM at 37°C for 24 h to produce various concentrations of the extraction media (i.e., 0.5, 5, and 10 mg/ml). This assay was performed in four replicate wells in 96-well plates. Before investigation, the extraction media were sterilized using 0.22-µm Minisart syringe filters. After that, the cultured cells were then starved with the serum-free medium (SFM) for 24 h. The medium was then replaced with the extraction media, and the cells were re-incubated for 24 h. Finally, the cell viability was determined by MTT assay. The viability of cells cultured with fresh SFM was used as a control.
Biocompatibility Evaluation of LSE-Loaded Alg/CS Beads
The biocompatibility evaluation of the LSE-loaded Alg/CS beads was performed with NHDF cells. First, NHDF cells (density of 25,000 cells/well) were allowed to grow in 24-well tissue culture polystyrene plates (TCPS; SPL Life Science, Korea) for 24 h. The LSE-loaded Alg/CS beads (2.5 mg) were sterilized under UV for 1 h and then added into each well. NHDF cells cultured with the LSE-loaded Alg/CS beads were allowed to grow for 2, 24, and 72 h. At each time point, the cell viability was investigated by MTT assay.
The morphology of cells cultured with the LSE-loaded Alg/CS beads was observed by using a SEM. NHDF cells (cell density of 25,000 cells/well) were seeded on a cover glass in 24-well plates and then cultured for 24 h to allow cell attachment. After that, the sterilized LSE-loaded Alg/CS beads were added to each well of 24-well plates. After 24 h, the culture medium in each well was removed and washed with PBS. The cells cultured with the beads were then fixed with 3% v/v glutaraldehyde for 30 min. After that, the cells were dehydrated with different concentrations (30, 50, 70, 90, and 100% v/v) of ethanol solution for two min each. The cells were immersed with 500 μl of 100% hexamethyldisilazane for five min and then left to dry in a desiccator at room temperature for 24 h. Finally, the cells were coated with gold before SEM observation.
Statistical Analysis
The experiments were represented as means ± standard derivation (SD). Analysis of variance (one-way ANOVA) and Tukey’s post hoc test in SPSS were carried out (IBM SPSS statistics 24, United States). The statistical significance was accepted at p < 0.05.
Results and Discussion
Extraction of Longan Seed Extract
The bioactive compounds in longan seeds such as phenolic acid, flavonoids, and polysaccharides showed antimicrobial, antioxidant, and anti-inflammatory activities (Rangkadilok et al., 2007). In this study, the ground longan seeds were extracted with distilled water. The percent yield of LSE was 7.46 ± 0.17%. The amount of gallic acid, ellagic acid, and corilagin, which are the major polyphenolic compounds found in the longan seed, was 25.61 ± 0.48, 18.83 ± 3.75, and 21.92 ± 1.42 mg/g (based on the weight of LSE), respectively. These values are in the same order with the results reported by Pankongadisak and Suwantong (Pankongadisak and Suwantong 2018).
Antioxidant Activity of Longan Seed Extract
The antioxidant activity of LSE as compared with l-ascorbic acid was determined by DPPH assay. In Figure 1, the antioxidant activity of LSE and l-ascorbic acid increased with increasing concentration. The maximum antioxidant activity of LSE and l-ascorbic acid at a concentration of 50 μg/ml was 89.10 and 92.71%, respectively. The half-maximum inhibitory concentration (IC50) of LSE was 24.63 ± 0.71 μg/ml, which is close to the standard drug (l-ascorbic acid) of 15.12 ± 0.09 μg/ml. Thus, the polyphenolic compounds such as gallic acid, ellagic acid, and corilagin in LSE showed strong antioxidant activity.
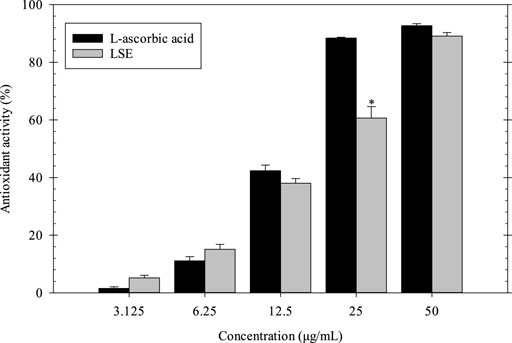
FIGURE 1. Antioxidant activity of LSE and L-ascorbic acid. *p < 0.05 compared with l-ascorbic acid at each concentration.
Indirect Cytotoxicity of Longan Seed Extract
The indirect cytotoxicity of LSE was carried out by MTT assay using NCTC clone 929 and NHDF cells. The cell viability was measured after 24 h of incubation (Figure 2). The results showed that the viability of NCTC clone 929 cells cultured with LSE ranged between ∼86 and ∼103%, while that of NHDF cells cultured with LSE ranged between ∼93 and ∼128% at a concentration of 0.02–0.625 mg/ml. These results confirmed that LSE at concentrations of 0.02–0.625 mg/ml was non-toxic to the cells and had potential use for further study.
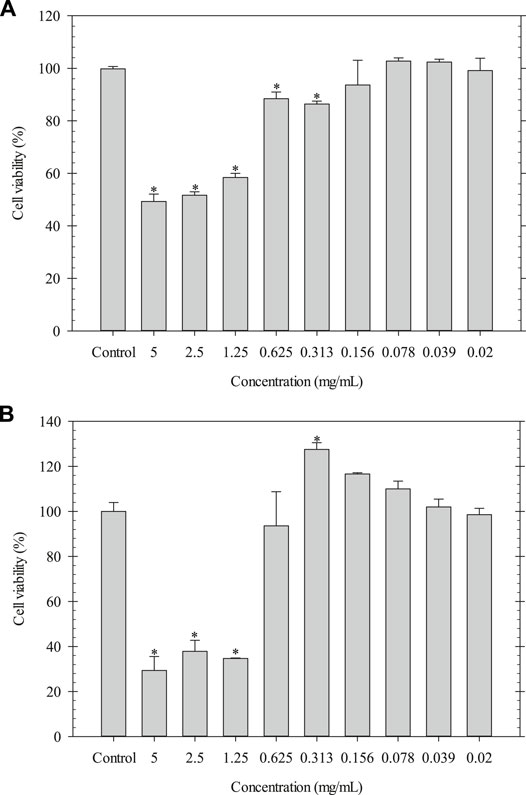
FIGURE 2. Indirect cytotoxicity of LSE cultured with (A) NCTC clone 929 and (B) NHDF cells. *p < 0.05 compared with the fresh culture medium.
Morphology, Size, and PDI of LSE-Loaded Alg/CS Beads
The LSE-loaded Alg/CS beads were prepared by using the ionic gelation method. The negatively charged carboxylic groups of Alg interacted with the protonated amino groups of CS to form the Alg/CS beads (Nagarwal et al., 2012; Rahaiee et al., 2015). Figure 3 shows that LSE/Alg/CS-S and LSE/Alg/CS-H exhibited more spherical shapes and dispersed particles. In contrast, LSE/Alg/CS-U showed an aggregation between particles because the shear force and time from the ultrasonicator were not enough to separate the particles completely. Besides, the merging of the polymer network during freeze-drying caused the aggregation of particles (Rahaiee et al., 2015). Moreover, the sizes of LSE/Alg/CS-S, LSE/Alg/CS-H, and LSE/Alg/CS-U were 1.88 ± 0.40, 2.41 ± 0.21, and 2.54 ± 0.15 μm, respectively. The PDIs of LSE/Alg/CS-S, LSE/Alg/CS-H, and LSE/Alg/CS-U were 0.198 ± 0.142, 0.150 ± 0.104, and 0.318 ± 0.048, respectively, as shown in Table 2.
FTIR Analysis
Figure 4 shows the FTIR spectra for LSE, Alg, CS, and LSE-loaded Alg/Cs beads. FTIR spectra of all samples between 3,000 and 3,600 cm−1 showed broad absorption bands indicating the O‒H stretching and N‒H stretching frequencies in the structure. For the spectrum of LSE, the peaks at 1,706 and 1,609 cm−1 were attributed to C‒H aromatic and C=C alkene stretching, respectively. The peaks at 1,522 cm−1 represented N‒O stretching. The peak at 1,050 cm−1 showed the strong intensity of C‒O stretching of ether (Aziz et al., 2018; Chollakup et al., 2021). For Alg, the FTIR spectrum represented the carboxyl anion stretching vibrations (asymmetric and symmetric) at 1,610 and 1,423 cm−1. The FTIR spectrum of CS showed the peak at 1,663 attributing to C=O stretching in amide I. The peaks at 1,592 cm−1 were assigned to N–H bending in amide II. In addition, the spectrum of CS showed the peak at 1,323 cm−1 which was assigned to N–H stretching in amide III (Bajpai 2019; Butt et al., 2019; Pereira et al., 2019). The LSE-loaded Alg/CS beads showed the shifted band intensity of 3,382 cm−1 (O–H, N–H) and 2,924 cm−1 (C–H). Moreover, the peak at 1,610 cm−1 of Alg was shifted to 1,606 cm−1. The peaks at 1,422 cm−1 were observed. This might be due to the shifting of asymmetrical and symmetrical stretching of -COO- of Alg. The peak at 1,530 cm−1 due to the protonation of amine groups of CS was not observed. This might be due to the overlap with the peak at 1,606 cm−1. From these results, it could be proved that the carboxylate groups of Alg reacted with the protonated amino groups of CS by intermolecular or electrostatic interactions to form the LSE-loaded Alg/CS beads (Butt et al., 2019; Rahaiee et al., 2015). Moreover, the absorption band of the O‒H stretching and N‒H stretching was shifted to 3,382 cm−1 due to the interaction of the hydroxyl group of LSE with Alg and CS. The disappearance of the peaks at 1,706, 1,609, and 1,522 cm−1 of LSE was due to the interaction of LSE with Alg and CS. The results confirmed that LSE was loaded in the Alg/CS beads successfully. In addition, the %EE of the LSE-loaded Alg/CS beads was another investigation to confirm that LSE was successfully loaded into the beads.
Encapsulation Efficiency
The % EE is the percentage of LSE that successfully entrapped into the Alg/CS beads. The %EE was quantified by the UV–Vis spectrophotometer at a wavelength of 277 nm. The %EE of the LSE-loaded Alg/CS beads is shown in Table 1. The %EE of LSE/Alg/CS-S, LSE/Alg/CS-H, and LSE/Alg/CS-U was 11.97 ± 0.91, 13.80 ± 2.99, and 18.42 ± 0.79%, respectively. The LSE/Alg/CS-U showed the highest value of %EE. This result showed that the large particle size of LSE/Alg/CS-U increased the ability to entrap LSE in high amounts (Lecaroz et al., 2006). Moreover, after freeze-drying, LSE/Alg/CS-U showed the merging of the polymer network caused by the formation of irregular shapes and particle aggregates.
Release Study
The release characteristics of LSE from the LSE-loaded Alg/CS beads were investigated by using the total immersion method for two days, and the results are shown in Figure 5. All samples showed similar profiles of the cumulative released amount. The release rate was fastest between 0 and 120 min. After that the cumulative released amounts were gradually increased and reached a plateau at the longest immersion time. The maximum cumulative released amount of LSE from LSE/Alg/CS-S, LSE/Alg/CS-H, and LSE/Alg/CS-U was 93, 86, and 68%, respectively. LSE/Alg/CS-U showed the lowest released amount of LSE, whereas LSE/Alg/CS-S and LSE/Alg/CS-H showed similar values. The low released amount of LSE observed from LSE/Alg/CS-U could be the merging of the beads that caused the formation of irregular shapes of the beads after the freeze-drying method. The merging of the beads makes the beads have large size with the low surface area. Thus, this can cause the lower released amount of LSE from the LSE/Alg/CS-U than the others. While, LSE/Alg/CS-S and LSE/Alg/CS-H showed the small spherical shape without the merging of the beads, resulting in the higher released amount of LSE from LSE/Alg/CS-S and LSE/Alg/CS-H.
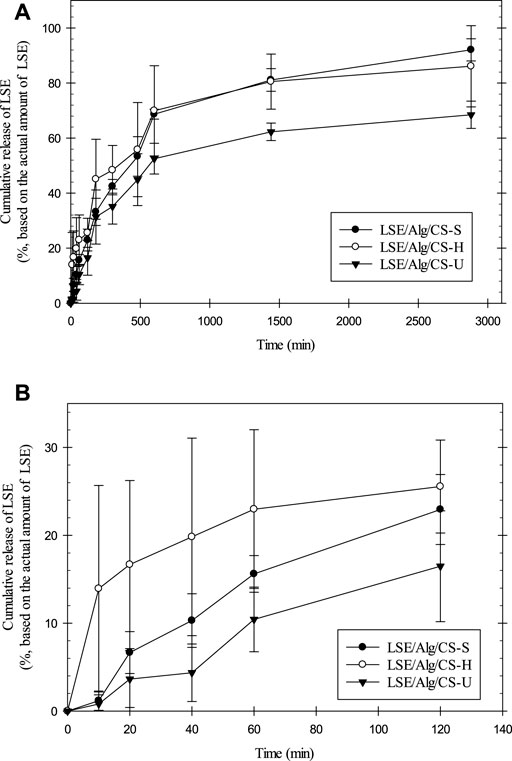
FIGURE 5. (A) Release profiles of LSE from LSE-loaded Alg/CS beads and (B) expanded figure of the release profile at first 120 min.
Indirect Cytotoxicity of LSE-Loaded Alg/CS Beads
The indirect cytotoxicity of the LSE-loaded Alg/CS beads was carried out by MTT assay using NCTC clone 929 cells and NHDF cells. The viability of cells was measured after 24 h incubation with different concentrations (0.5, 5, and 10 mg/ml) of extraction media from the LSE-loaded Alg/CS beads (Figure 6). The results showed that the viability of NCTC clone 929 cells cultured with the extraction media from LSE/Alg/CS-S, LSE/Alg/CS-H, and LSE/Alg/CS-U ranged between 74 and 95%, while the viability of NHDF cells ranged between 86 and 101%. According to the ISO 10993-5 cytotoxicity standard, if the percent cell viability is increased to >70%, it has a non-cytotoxic potential. Thus, these beads were non-toxic to both NCTC clone 929 cells and NHDF cells and had the potential for use in biomedical applications.
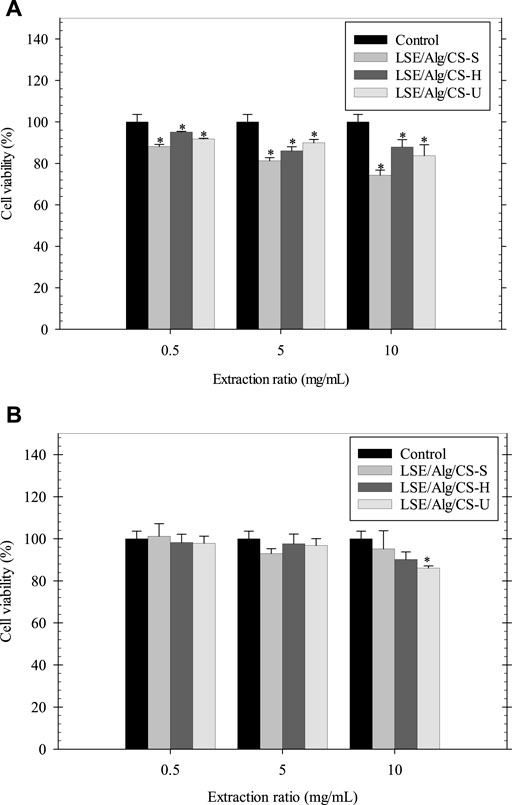
FIGURE 6. Indirect cytotoxicity of LSE-loaded Alg/CS beads cultured with (A) NCTC clone 929 and (B) NHDF cells (n = 3). *p < 0.05 compared with the fresh culture medium.
Biocompatibility
For use in biomedical applications, materials must exhibit good biocompatibility, non-toxic, and support cell growth (Kim et al., 2008). Thus, the biocompatibility of cells cultured with the LSE-loaded Alg/CS beads was carried out. The viability of NHDF cells cultured with the fresh culture medium was the control. The Alg/CS beads without the loading of LSE were designated as Alg/CS. Figure 7 demonstrates the viability of NHDF cells cultured with samples at different incubation times (2, 24, and 72 h). After incubation, the viability of cells was determined by MTT assay. The viability of NHDF cells cultured with all samples was between 97 and 126%. The viability of NHDF cells was more than 70% after treatment with samples at different time points, indicating these beads were non-toxic and compatible with the cells.
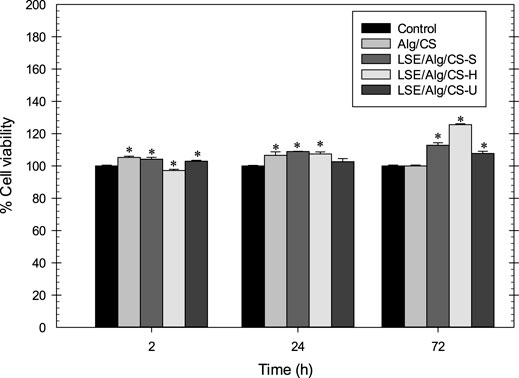
FIGURE 7. Cell biocompatibility of LSE-loaded Alg/CS beads with NHDF cells. *p < 0.05 compared with the fresh culture medium.
The morphology of NHDF cells cultured with LSE-loaded Alg/CS beads was observed by using a SEM (see Table 3). From the results, the NHDF cells cultured with all samples showed good attachment on the cover glass and exhibited a spindle shape on the surface. Therefore, these LSE-loaded Alg/CS beads had the potential for use in biomedical applications.
Conclusion
In this study, the LSE-loaded Alg/CS beads were successfully prepared. The polymeric carriers were made from Alg and CS using the ionic gelation method. The LSE was added as an active ingredient. The LSE-loaded Alg/CS beads showed the spherical shape with some aggregation. The particle sizes of the LSE-loaded Alg/CS beads were ranging between ∼1.9 and ∼2.5 μm. PDI ranged between 0.150 and 0.318. LSE/Alg/CS-S had the smallest sizes, while LSE/Alg/CS-U showed the highest %EE. The cumulative released amount of LSE from LSE/Alg/CS-S had the highest value. Finally, these beads were non-toxic to both NCTC clone 929 and NHDF cells and promoted the attachment of NHDF cells. These results suggested that these beads can be used as drug carriers for biomedical applications.
Data Availability Statement
The raw data supporting the conclusion of this article will be made available by the authors, without undue reservation.
Author Contributions
TB contributed to methodology, acquisition of data, analysis and interpretation of data, and drafting the manuscript; PK contributed to reviewing and editing the manuscript; SS helped with methodology and reviewing and editing the manuscript; CT assisted with reviewing and editing the manuscript; and OS involved in conceptualization, methodology, funding acquisition, analysis and interpretation of data, and reviewing and editing the manuscript.
Conflict of Interest
The authors declare that the research was conducted in the absence of any commercial or financial relationships that could be construed as a potential conflict of interest.
Publisher’s Note
All claims expressed in this article are solely those of the authors and do not necessarily represent those of their affiliated organizations, or those of the publisher, the editors, and the reviewers. Any product that may be evaluated in this article, or claim that may be made by its manufacturer, is not guaranteed or endorsed by the publisher.
Acknowledgments
The authors would like to acknowledge Mae Fah Luang University for funding. The authors gratefully acknowledge the National Nanotechnology Center (NANOTEC), the National Science and Technology Development Agency (NSTDA), Scientific and Technological Instruments Center (STIC), and Mae Fah Luang University for laboratory facilities.
References
Ahdyani, R., Novitasari, L., Martien, R., and Danarti, R. E. T. N. O. (2019). Formulation and Characterization of Timolol Maleate-Loaded Nanoparticles Gel by Ionic Gelation Method Using Chitosan and Sodium Alginate. Int. J. App Pharm. 11, 48–54. doi:10.22159/ijap.2019v11i6.34983
Aziz, H., Rahim, N., Ramli, S., Alazaiza, M., Omar, F., and Hung, Y.-T. (2018). Potential Use of Dimocarpus Longan Seeds as a Flocculant in Landfill Leachate Treatment. Water 10, 1672. doi:10.3390/w10111672
Bajpai, A. K. (2019). Facile Preparation of Ionotropically Crosslinked Chitosan-Alginate Nanosorbents by Water-In-Oil (W/O) Microemulsion Technique: Optimization and Study of Arsenic (V) Removal. J. Water Process Eng. 32, 100920. doi:10.1016/j.jwpe.2019.100920
Bernkop-Schnürch, A., and Dünnhaupt, S. (2012). Chitosan-based Drug Delivery Systems. Eur. J. Pharmaceutics Biopharmaceutics 81, 463–469. doi:10.1016/j.ejpb.2012.04.007
Blois, M. S. (1958). Antioxidant Determinations by the Use of a Stable Free Radical. Nature 181, 1199–1200. doi:10.1038/1811199a0
Butt, A., Jabeen, S., Nisar, N., Islam, A., Gull, N., Iqbal, S. S., et al. (2019). Controlled Release of Cephradine by Biopolymers Based Target Specific Crosslinked Hydrogels. Int. J. Biol. Macromolecules 121, 104–112. doi:10.1016/j.ijbiomac.2018.10.018
Chollakup, R., Kongtud, W., Sukatta, U., Premchookiat, M., Piriyasatits, K., Nimitkeatkai, H., et al. (2021). Eco-friendly rice Straw Paper Coated with Longan (Dimocarpus Longan) Peel Extract as Bio-Based and Antibacterial Packaging. Polymers 13, 3096. doi:10.3390/polym13183096
De Pinho Neves, A. L., Milioli, C. C., Müller, L., Riella, H. G., Kuhnen, N. C., and Stulzer, H. K. (2014). Factorial Design as Tool in Chitosan Nanoparticles Development by Ionic Gelation Technique. Colloids Surf. A: Physicochemical Eng. Aspects 445, 34–39. doi:10.1016/j.colsurfa.2013.12.058
Gagliardi, M., Bardi, G., and Bifone, A. (2012). Polymeric Nanocarriers for Controlled and Enhanced Delivery of Therapeutic Agents to the CNS. Ther. Deliv. 3, 875–887. doi:10.4155/tde.12.55
George, A., Shah, P. A., and Shrivastav, P. S. (2019). Natural Biodegradable Polymers Based Nano-Formulations for Drug Delivery: A Review. Int. J. Pharmaceutics 561, 244–264. doi:10.1016/j.ijpharm.2019.03.011
Ji, J., Hao, S., Wu, D., Huang, R., and Xu, Y. (2011). Preparation, Characterization and In Vitro Release of Chitosan Nanoparticles Loaded with Gentamicin and Salicylic Acid. Carbohydr. Polym. 85, 803–808. doi:10.1016/j.carbpol.2011.03.051
Kim, I.-Y., Seo, S.-J., Moon, H.-S., Yoo, M.-K., Park, I.-Y., Kim, B.-C., et al. (2008). Chitosan and its Derivatives for Tissue Engineering Applications. Biotechnol. Adv. 26, 1–21. doi:10.1016/j.biotechadv.2007.07.009
Kumari, A., Yadav, S. K., and Yadav, S. C. (2010). Biodegradable Polymeric Nanoparticles Based Drug Delivery Systems. Colloids Surf. B: Biointerfaces 75, 1–18. doi:10.1016/j.colsurfb.2009.09.001
Lecaroz, C., Gamazo, C., Renedo, M. J., and Blanco-Prieto, M. J. (2006). Biodegradable Micro- and Nanoparticles as Long-Term Delivery Vehicles for Gentamicin. J. Microencapsulation 23, 782–792. doi:10.1080/02652040600946886
Lee, K. Y., and Mooney, D. J. (2012). Alginate: Properties and Biomedical Applications. Prog. Polym. Sci. 37, 106–126. doi:10.1016/j.progpolymsci.2011.06.003
Li, X., Deng, Y., Zheng, Z., Huang, W., Chen, L., Tong, Q., et al. (2018). Corilagin, a Promising Medicinal Herbal Agent. Biomed. Pharmacother. 99, 43–50. doi:10.1016/j.biopha.2018.01.030
Lim, T. K. (2013). “Dimocarpus Longan Subsp. Malesianus Var. Malesianus,” in Edible Medicinal and Non-medicinal Plants (Springer), 33–38. doi:10.1007/978-94-007-5628-1_6
Morsi, N., Ghorab, D., Refai, H., and Teba, H. (2015). Preparation and Evaluation of Alginate/chitosan Nanodispersions for Ocular Delivery. Int. J. Pharm. Pharm. Sci. 7, 234–240.
Nagarwal, R. C., Kumar, R., and Pandit, J. K. (2012). Chitosan Coated Sodium Alginate-Chitosan Nanoparticles Loaded with 5-FU for Ocular Delivery: In Vitro Characterization and In Vivo Study in Rabbit Eye. Eur. J. Pharm. Sci. 47, 678–685. doi:10.1016/j.ejps.2012.08.008
Nalini, T., Basha, S. K., Mohamed Sadiq, A. M., Kumari, V. S., and Kaviyarasu, K. (2019). Development and Characterization of Alginate/Chitosan Nanoparticulate System for Hydrophobic Drug Encapsulation. J. Drug Deliv. Sci. Tech. 52, 65–72. doi:10.1016/j.jddst.2019.04.002
Nguyen, T. V., Nguyen, T. T. H., Wang, S.-L., Vo, T. P. K., and Nguyen, A. D. (2017). Preparation of Chitosan Nanoparticles by TPP Ionic Gelation Combined with spray Drying, and the Antibacterial Activity of Chitosan Nanoparticles and a Chitosan Nanoparticle-Amoxicillin Complex. Res. Chem. Intermed. 43, 3527–3537. doi:10.1007/s11164-016-2428-8
Pankongadisak, P., and Suwantong, O. (2018). The Potential Use of Thermosensitive Chitosan/silk Sericin Hydrogels Loaded with Longan Seed Extract for Bone Tissue Engineering. RSC Adv. 8, 40219–40231. doi:10.1039/C8RA07255H
Pereira, A. D. S., Diniz, M. M., De Jong, G., Gama Filho, H. S., Dos Anjos, M. J., Finotelli, P. V., et al. (2019). Chitosan-alginate Beads as Encapsulating Agents for Yarrowia Lipolytica Lipase: Morphological, Physico-Chemical and Kinetic Characteristics. Int. J. Biol. Macromolecules 139, 621–630. doi:10.1016/j.ijbiomac.2019.08.009
Rahaiee, S., Shojaosadati, S. A., Hashemi, M., Moini, S., and Razavi, S. H. (2015). Improvement of Crocin Stability by Biodegradeble Nanoparticles of Chitosan-Alginate. Int. J. Biol. Macromolecules 79, 423–432. doi:10.1016/j.ijbiomac.2015.04.041
Rajendran, A., and Basu, S. (2009). Alginate-chitosan Particulate System for Sustained Release of Nimodipine. Trop. J. Pharm. Res. 8, 433–440. doi:10.4314/tjpr.v8i5.48087
Rangkadilok, N., Sitthimonchai, S., Worasuttayangkurn, L., Mahidol, C., Ruchirawat, M., and Satayavivad, J. (2007). Evaluation of Free Radical Scavenging and Antityrosinase Activities of Standardized Longan Fruit Extract. Food Chem. Toxicol. 45, 328–336. doi:10.1016/j.fct.2006.08.022
Sarei, F., Zolfagharian, H., Khaki, P., Bidhendi, S., and Dounighi, N. (2013). Alginate Nanoparticles as a Promising Adjuvant and Vaccine Delivery System. Indian J. Pharm. Sci. 75, 442–449. doi:10.4103/0250-474X.119829
Sinha, V. R., Singla, A. K., Wadhawan, S., Kaushik, R., Kumria, R., Bansal, K., et al. (2004). Chitosan Microspheres as a Potential Carrier for Drugs. Int. J. Pharmaceutics 274, 1–33. doi:10.1016/j.ijpharm.2003.12.026
Song, W., Su, X., Gregory, D., Li, W., Cai, Z., and Zhao, X. (2018). Magnetic Alginate/chitosan Nanoparticles for Targeted Delivery of Curcumin into Human Breast Cancer Cells. Nanomaterials 8, 907. doi:10.3390/nano8110907
Sun, L., Zhou, S., Wang, W., Li, X., Wang, J., and Weng, J. (2009). Preparation and Characterization of Porous Biodegradable Microspheres Used for Controlled Protein Delivery. Colloids Surf. A: Physicochemical Eng. Aspects 345, 173–181. doi:10.1016/j.colsurfa.2009.04.053
Tang, Y.-Y., He, X.-M., Sun, J., Li, C.-B., Li, L., Sheng, J.-F., et al. (2019). Polyphenols and Alkaloids in Byproducts of Longan Fruits (Dimocarpus Longan Lour.) and Their Bioactivities. Molecules 24 (6), 1186. doi:10.3390/molecules24061186
Venditti, I. (2019). Morphologies and Functionalities of Polymeric Nanocarriers as Chemical Tools for Drug Delivery: A Review. J. King Saud Univ. - Sci. 31, 398–411. doi:10.1016/j.jksus.2017.10.004
Worasuttayangkurn, L., Watcharasit, P., Rangkadilok, N., Suntararuks, S., Khamkong, P., and Satayavivad, J. (2012). Safety Evaluation of Longan Seed Extract: Acute and Repeated Oral Administration. Food Chem. Toxicol. 50, 3949–3955. doi:10.1016/j.fct.2012.07.068
Yang, B., Jiang, Y., Shi, J., Chen, F., and Ashraf, M. (2011). Extraction and Pharmacological Properties of Bioactive Compounds from Longan (Dimocarpus Longan Lour.) Fruit - A Review. Food Res. Int. 44, 1837–1842. doi:10.1016/j.foodres.2010.10.019
Keywords: longan seed extract, chitosan, alginate, ionic gelation, drug delivery system
Citation: Buntum T, Kakumyan P, Surassmo S, Thanomsilp C and Suwantong O (2022) Potential of Longan Seed Extract–Loaded Alginate–Chitosan Beads as Drug Delivery System. Front. Mater. 9:818595. doi: 10.3389/fmats.2022.818595
Received: 19 November 2021; Accepted: 04 January 2022;
Published: 01 February 2022.
Edited by:
Nafisa Gull, University of the Punjab, PakistanReviewed by:
Mohammad Alnaief, German jordanian university, JordanDhony Hermanto, University of Mataram, Indonesia
Copyright © 2022 Buntum, Kakumyan, Surassmo, Thanomsilp and Suwantong. This is an open-access article distributed under the terms of the Creative Commons Attribution License (CC BY). The use, distribution or reproduction in other forums is permitted, provided the original author(s) and the copyright owner(s) are credited and that the original publication in this journal is cited, in accordance with accepted academic practice. No use, distribution or reproduction is permitted which does not comply with these terms.
*Correspondence: Orawan Suwantong, by5zdXdhbnRvbmdAZ21haWwuY29tL29yYXdhbi5zdXdAbWZ1LmFjLnRo