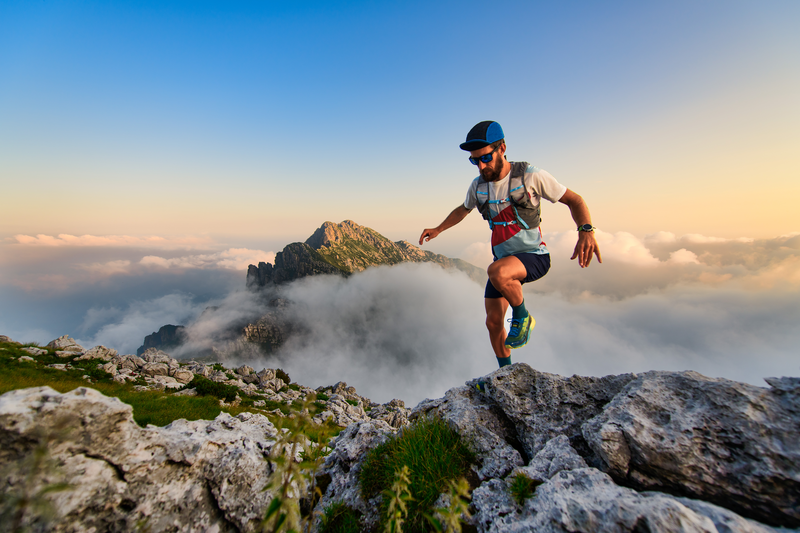
95% of researchers rate our articles as excellent or good
Learn more about the work of our research integrity team to safeguard the quality of each article we publish.
Find out more
ORIGINAL RESEARCH article
Front. Mater. , 27 April 2022
Sec. Polymeric and Composite Materials
Volume 9 - 2022 | https://doi.org/10.3389/fmats.2022.814551
In this study, a ternary blend modifier (TBM) was prepared with RPE, RPP, and various dosages of PP-g-MAH (i.e., 0%, 1%, 3%, 5%, 7%, and 9% by weight of RPE and RPP) by a twin-screw extruder. The performance of TBM (4% by weight of asphalt) modified asphalt was studied, with the increase of PP-g-MAH content, the penetration decreases gradually, but softening point and viscosity increases and ductility exists minimum value at 7% content, with the increasing of PP-g-MAH percentage, the dynamic rheological properties show that |G*| increases, while the δ and |G*|cosδ decrease, and the fatigue performance (|G*|sinδ) of TBM modified asphalt is worse than virgin asphalt. Dynamic stability test shows that TBM shows the prominent high-temperature properties of a modified (asphalt mixture. BBR test indicates that the m-value of asphalt modified with TBM does not fulfill the requirements (m-value>0.3) except at 5% and 7%, but the S meets the standard requirements (S < 300 MPa), and increases from 0% to 7%, then decreases slightly. In order to illuminate possible reasons, a chemical reaction (ester compounds generated) and physical twines of concerned polymer molecules were put forward, and the results were confirmed by infrared analysis.
Polyethylene and polypropylene are two major raw materials of plastic products, and recycled polyethylene (RPE) and recycled polypropylene (RPP) are still the main types of plastic waste in 2020 (Colantonio et al., 2019). Compared to energy utilization and landfill, recycling plastic waste is the best way to dispose of it (Ragaert et al., 2017; He et al., 2019). Therefore, a lot of reuses have been found by researchers for recycled plastics, such as utilizing them for infrastructure (Keskisaari and Kärki, 2018). The performance of asphalt modified by recycled plastics is comparable to virgin asphalt, utilization of recycled plastic shows a great potentiality on reducing construction costs and the impacts on the environment (Kalantar et al., 2012; Ragaert et al., 2017; Costa et al., 2019).
With the increase of RPE content, the softening point of RPE modified asphalt increases, while its penetration decreases; the asphalt mixture modified by RPE exhibits better strength and better rutting resistance as compared to unmodified one, especially when it is used in high-temperature conditions (Polacco et al., 2005; Ahmedzade et al., 2014; Gibreil and Feng, 2017). The rheological tests show that the modified asphalt with RPE increases the complex modulus of asphalt, reduces the phase angle, and improves the rheological properties of asphalt binder (Mohd Hasan et al., 2016; Ameri et al., 2020). Nevertheless, the storage stability of modified asphalt by RPE is not good due to crystallization (Liang et al., 2020). The utilization of RPE may also potentially reduce the low-temperature properties of modified asphalt (Othman, 2010).
The addition of RPP significantly reduces penetration and increases the softening point of asphalt binder; under the same proportion of RPP addition, the reduction percentages are greater than the RPE modified asphalt. In addition, if RPP degrades at high temperatures or reacts with maleic anhydride as additives, the results display similar change trends (Casey et al., 2008; Ahmedzade et al., 2015). Tapkın et al. (2010) utilized the artificial network model to simulate the variations of Marshall stability and flow value of asphalt modified with RPP, the simulation values are consistent with the experimental ones, both show improvement. Regarding the low-temperature performance, the ductility of asphalt binder modified with 5% RPP reduces about 20%, when the RPP is grafted with maleic anhydride, the ductility of asphalt with the same addition content reduces only about 5% (Al-Hadidy and Yi-qiu, 2009; Ahmedzade et al., 2015). The complex modulus, phase angle, and rutting factor (|G*|/sinδ) of asphalt also greatly improve when the asphalt is modified by RPP and other additives, especially with 5% chlorinated polypropylene (26% of Cl) and isotactic polypropylene (Yeh et al., 2005; Nien et al., 2008).
Although modification of asphalt with single RPE or RPP has been extensively studied, the combined utilization of RPE and RPP is rarely reported. Many recycled polymer combinations have been reported in the literature, but most of them were made from elastomers and plastomers, such as RPE + GTR (ground tire rubber) (Navarro et al., 2010; Zhang and Hu, 2015), RPP + GTR (Zhang and Hu, 2015), and REVA (recycled ethyl vinyl acetate) + RPE (Brovelli et al., 2015) etc. This possible reason is that the ingredients of recycled plastic blends are more complex, and their effects on asphalt and asphalt mixture are hard to characterize. The waste plastics are a mixture of various polymers (especially RPE and RPP), and numerous studies have shown that it is usually difficult to use a single modifier to improve the performance of modified asphalt (Casey et al., 2008; Jahromi and Khodaii, 2009; Zhu et al., 2014). It is thus significant to investigate a blended modifier prepared with RPE and RPP for asphalt binder (Yin et al., 2015).
In this study, a ternary blend modifier (TBM) was prepared with a twin-screw extruder. Chemical reactions appeared between the ring-opening of the maleic anhydride group and the -OH from RPE and RPP to generate ester compounds in the high temperature, synchronously, physical entangling of the polymer chains (such as PP molecule chain) raises. The influence of different contents of PP-g-MAH on the performance of TBM modified asphalt and its mixture was experimentally studied. This study provided a theoretical basis for industrial production and engineering application involving TBM.
RPE was collected from Dongguan Zhongmin New Material Technology Co. Ltd, its physical properties are 0.4522 g/10 min MFI (MFI test condition: 190°C and 2.16 kg), 7.423 wt% ash content, and 0.994 g/cm3 density. RPP was supplied by the Shanghai Jinfa Technology Development Co. Ltd., which has 11.486 g/10 min MFI (MFI test condition: 190°C and 2.16 kg), 2.337 wt% ash content, and 0.816 g/cm3 density. RPE and RPP were washed and then dried in an oven at 30°C for 24 h. PP-g-MAH was obtained from Xiamen Keisi Plastic Technology Co. Ltd., whose technical properties are 2.5 g/10 min MFI (ASTM D1238 ISO 1133), 0.95 g/cm3 density, 1.1% grafting ratio, 130°C melting point, and white particles. The virgin asphalt was produced by Shandong Yurun Road Material Co. Ltd., and its physical properties are >100 cm ductility (25°C), 54°C softening point (R&B), 89 mm Penetration (25°C), and 243°C flashpoint, its chemical composition is 23.64% resins, 10.84% saturates, 13.15% asphaltenes and 52.37% aromatics.
The preparation of TBM is illustrated in Figure 1. RPP and RPE were dried in an oven with a temperature of 80°C for 2h, PP-g-MAH was dried at 60°C for 2 h, and then they were cooled to room temperature. The feeding speed and material quantity information of hopper A and hopper B (RPP) are displayed in Table 1. The RPE/PP-g-MAH blends were premixed for 15 min at a speed of 2000 rpm before being fed into hopper A. The RPP was fed into hopper B. In this study, 0%, 1%, 3%, 5%, 7, and 9% PP-g-MAH dosage was added into TBM by the weight of RPP/RPE blends. Temperature controllers of the twin-screw extruder (from the first zone to the ninth zone) were set from 170 to 190°C, and the temperature of the nose was controlled at 185°C. After cooling and air blow-drying, the extruded strip materials were pelleted by the granulator. Then TBM modifier was obtained for virgin asphalt and its mixture.
The virgin asphalt (six samples, each sample 500 g) was heated to 170°C, then six different TBM modifiers (20 g, i.e., 4% by the weight of asphalt) were gradually added into the preheated asphalt. They were stirred at a low speed of 1,000–1,500 rpm at 170°C for 0.5 h. Then, TBM/asphalt blends were heated to 180°C and sheared at a high speed of 3,500–4,000 rpm for 1.5 h to obtain a well-dispersed phase. The testing samples were prepared in accordance with the Standard Test Methods of Bitumen and Bituminous Mixtures for Highway Engineering (Ministry of Transport of PR China, 2011).
The asphalt mixtures modified with TBM were prepared in the dry process. The aggregate for asphalt mixture was heated to 185°C, the gradation curve of aggregate used in this study is shown in Figure 2. The TBM (0.35% mass percentage of TBM to aggregate) was added into the aggregate via the mixer of the asphalt mixture and stirred at 180°C for 40°s until homogenized with the aggregates. Then, 4.5% (the optimum asphalt-aggregate ratio) asphalt was added into the blends (TBM/aggregate) and mixed for 90°s. The mineral powder was added to the mixtures and stirred constantly for 90°s at 180°C. The specimens of the dynamic stability test (three replicates) were prepared with 300°mm × 300°mm × 50°mm steel mold (Ma et al., 2016).
In this study, the basic properties including penetration (25°C), softening point, ductility (5°C), and rotational viscosity were conducted in accordance with the Standard Test Methods of Bitumen and Bituminous Mixtures for Highway Engineering (Ministry of Transport of PR China, 2011).
FTIR tests were performed to identify the possible chemical reactions between the virgin asphalt binder and TBM modifier at 665 to 3,050 cm−1 wavelengths.
The effect of PP-g-MAH content on the rheological properties of asphalt modified with TBM was evaluated by the temperature sweep test with a dynamic shear rheometer (DSR). A parallel plate with a diameter of 25.0 mm and a 1.0 mm gap was selected in the DSR tests. The temperature sweep tests were carried out from 40°C to 90°C at a constant frequency of 10 rad/s. The complex modulus (|G*|) and phase angle (δ) were obtained from the DSR tests. Then, |G*|cosδ and |G*|sinδ were calculated to evaluate the elastic properties and fatigue resistance of TBM modified asphalt, respectively.
A bending beam rheometer (BBR) was employed to evaluate the effect of PP-g-MAH dosage on the low-temperature performance of asphalt modified with TBM. The creep stiffness (S) and creep rate (m-value) were obtained at −18°C (0.5°C accuracy) from the BBR tests, The S characterized the permanent deformation resistance of the constant load, m-value characterized the stiffness change, stiffness sensitivity, and stress relaxation ability of asphalt modified with TBM. The higher the S, the more brittle modified asphalt, the worse the performance at low temperature. The higher the creep rate, the greater m-value, the better the anti-cracking performance at low temperatures (Yang, 2016).
Dynamic stability was performed through the wheel tracking test at 60°C, AC-20 gradation was selected, and the dynamic stability and rutting depth values were obtained from the wheel tracking tests (Ma et al., 2016).
Figure 3 shows that softening point of asphalt modified by 4% TBM. The TBM-modified asphalt shows a greater softening point than that of the virgin asphalt. With increasing PP-g-MAH content, the softening point gradually increases, indicating that the PP-g-MAH has a positive effect on the high-temperature performance of asphalt. This experimental observation is consistent with asphalt modified with waste plastic (Alghrafy et al., 2021). The increasing rate of softening point is lower when the PP-g-MAH dosage exceeds 7%. The possible reason is that the chemical reactions between PP-g-MAH and the hydroxyl functional groups from RPE and RPP have completed at 7% content and formed a relatively complete polymer structure. After that, the softening point of asphalt modified by TBM is not significantly affected as further TBM is added into the asphalt.
The penetration of asphalt modified by TBM is lower after the addition of PP-g-MAH, and the changes are more obvious with the increase of PP-g-MAH content. When PP-g-MAH content is more than 7%, the penetration does not change significantly (61 mm), and it remains similar level with softening point when PP-g-MAH dosage is greater than 7%. Figure 3 shows that the PP-g-MAH content has a significant influence on high-temperature performance of asphalt modified with TBM.
Figure 4 gives the effect of PP-g-MAH content on the ductility of asphalt modified by TBM. Compared with virgin asphalt [>100 cm ductility (25°C)], the ductility of TBM modified asphalt becomes lower after the addition of PP-g-MAH. The ductility decreases gradually with the increase of PP-g-MAH content which ranges from 0% to 7%. This indicates a negative effect on improving the low-temperature performance of asphalt. However, when PP-g-MAH content is above 7% (7%–9%), the ductility of modified asphalt increases slightly, and finally reaches 30 cm at 9% dosage. When the PP-g-MAH content increases to 7%, chemical reactions have been completed between hydroxyl groups (from RPP, RPE, and asphalt) and maleic anhydride groups (from PP-g-MAH), the remaining PP-g-MAH is conducive to enhancing the flexibility of the modified asphalt, the possible reason is that melting point of PP-g-MAH is relatively low only to 130°C, and this is of great significance for improving the low-temperature performance of the modified asphalt by TBM.
Figure 5A shows the FTIR results of raw RPE/RPP used in this study. The peaks of -CH3 and -CH2 appear at 1,470 cm−1, the free hydroxyl peaks appear at 2,600 cm−1, 2,670 cm−1, and 1,130 cm−1. The stretching vibrations of hydroxyl groups raise up at 3,130 cm−1 and 3,110 cm−1.
FIGURE 5. FTIR analysis of RPE/RPP (A) and TBM modified asphalt with different content of PP-g-MAH (B).
The main functional groups involved in the TBM modified asphalt at different content levels of PP-g-MAH were investigated by FTIR spectra in the wavenumber range of 680–3000cm−1, as shown in Figure 5B, at the addition of 0%, 1%, and 3% PP-g-MAH content, the FTIR curves of transmittance of TBM modified asphalt are similar and there are no obvious new characteristic peaks to occur in the infrared spectrums, and this indicates that no chemical reactions appear at lower concentration of PP-g-MAH and that interactions between the asphalt and the TBM is very limited. However, it is observed that a few typical absorption peaks were located at 1124 cm−1 (C-O-C stretch) and 1755 cm−1(C=O stretch) when the PP-g-MAH content increased to 7% from 5%, and the chemical reactions (ester compounds formed) between -OH from the asphalt and maleic anhydride groups from TBM particles are more obvious at this stage. The possible reaction mechanism is illuminated in Figure 6 [PP-g-MAH contains maleic anhydride groups, so it has active chemical properties and is easy to esterize with a hydroxyl group at high temperature (Zhang et al., 2013)]. The bonding of TBM modified asphalt with 5% PP-g-MAH dosage is consistent with that of 7% PP-g-MAH but with different intensities, and the possible reason is that PP-g-MAH is excess and all hydroxyl groups from RPE/RPP/asphalt have reacted completely at 9% PP-g-MAH dosage, so MAH groups can be seen obviously at 1896 cm−1.
The possible interaction mechanism involved in the preparation of TBM and chemical action with asphalt can be obtained from Figure 6. PP-g-MAH contains conjugated maleyl groups, and one vinyl group is connected with two carbonyl groups in the molecular structure, so PP-g-MAH has active chemical properties and is easy to esterize with a hydroxyl group at high temperature to generate new substances (Zhang et al., 2013). TBM is prepared with the twin-screw extruder and the process diagram is shown in Figure 1. First, PP-g-MAH and RPE are uniformly input from hopper A according to the ratio information in Table 1. The melt-grafting reaction is conducted at a temperature of 170°C–190°C to generate ester compounds (between anhydride and hydroxyl group generated after RPE aging, Figure 5A) by the twin-screw extrusion, as shown in the first red rectangle ( EQ \o\ac(○,Ⅰ)) of Figure 8. Simultaneously, the RPP is added into hopper B, the esterification reaction occurs under the influence of temperature and screw blending, and because the RPP and PP-g-MAH contain similar PP molecular chains, the physical-winding structure is formed by the van der Waals force, which further enhances the interfacial compatibility among three kinds of raw materials. It is beneficial to obtain TMB modifier with relatively stable performance, the related products of this reaction process are shown in the second red rectangle ( EQ \o\ac(○,Ⅱ)). Last, in the process of preparing modified asphalt network structures comprising of the molecular chain are formed after mixing and shearing at 170°C–190°C, on the one hand, a light component of matrix asphalt is adsorped by TBM, which reduces the interfacial tensions between asphalt and TBM, on the other hand, ester compounds come into being between excessive maleic anhydride groups of PP-g-MAH and matrix asphalt (-OH), and the product structure is shown in the third red rectangle ( EQ \o\ac(○,Ⅲ)).
The difference in softening point (ΔT) between the top and bottom portion of samples had been conducted to evaluate the effect of PP-g-MAH content on the high-temperature storage stability of modified asphalt with TBM. It is clearly observed from Figure 7 that the ΔT of TBM modified asphalts decreases with the increase of PP-g-MAH content. The possible reason is that chemical reactions occur between the asphalt and TBM particles, as shown in Figures 5B, 6. When the PP-g-MAH content increases from 6% to 9%, the ΔT basically remains similar, which indicates that there is no substantial phase separation or chemical reactions between the TBM and asphalt during mixing which is strong enough to resist phase separation of modified asphalt. When PP-g-MAH dosage increases to 6.7%, ΔT is 2.5°C, the storage stability of modified asphalt by TBM has completely met the standard of stable asphalt, ΔT is no greater than 2.5°C at the PP-g-MAH content of 7% and 9%, so it can be concluded that PP-g-MAH has a positive effect on storage stability, but an optimum content is important for improving its high-temperature storage stability and achieving the expected performances in construction.
FIGURE 7. Effect of PP-g-MAH content on softening points of modified. asphalt at top and bottom section.
The effect of PP-g-MAH content on the viscosity of TBM modified asphalt is illustrated in Figure 8. Based on the slope change of the viscosity curves, it is divided into three regions. In region, I, the viscosity of asphalt slightly increases with an increase in the dosage of PP-g-MAH from 0% to 3%. In region II (the dosage of PP-g-MAH from 3% to 7%), the slope of the curve is the greatest among the three regions. The slope retains constant values in region III (the dosage of PP-g-MAH from 7% to 9%). The possible reasons are as follows: In the first region, with the increasing of PP-g-MAH content, the chemical reaction between PP-g-MAH and -OH of RPE has been gradually completed in the case of a small dosage (3%). In the second region, according to the theory of similarity-compatibility, as the dosage of PP-g-MAH increases, PP-g-MAH contains PP-attracting groups (PP) which has good affinity to RPP, and hydrophobic group (oil-attracting MAH) can interact with -OH of RPP, more crosslinking (chemical interaction) and interwinding (physical interaction) appear. The ester compounds are produced from the reaction between -OH from the asphalt and maleic anhydride groups of TBM. Therefore, the increase of viscosity with an increase in PP-g-MAH content is due to the molecular interaction. More network structures of TBM are rich in asphalt which is responsible for viscosity enhancement by forming a more complex microstructure, it is most obvious in region II as compared to the other regions (Stastna et al., 2003). At a higher dosage of PP-g-MAH (i.e., 7%–9%), the viscosity remains almost unchanged because PP-g-MAH is in excess in comparison to the asphalt content. The PP-g-MAH content has a significant effect on the pumping, mixing, and workability of asphalt modified by TBM.
|G*| is the complex shear modulus of modified asphalt under cyclic shear deformation, it consists of two main parts: elastic part G' (recoverable) and viscous part G″ (irreversible). |G*| were measured directly from DSR under a range of temperatures (i.e., from 40°C to 90°C) at a constant frequency of 10 rad/s, as shown in Figure 9. Under the same conditions of temperature, |G*| increases gradually with an increasing PP-g-MAH content, under the same dosage of PP-g-MAH, |G*| decreases with the rising in testing temperature. PP-g-MAH is effective in improving rutting resistance under high-temperature conditions. In addition, the increasing ratio of |G*| is much faster from 3% to 7%, then slows down in the range of 7% and 9%. The possible reason is that the reactions between the functional groups of PP-g-MAH and -OH (from RPE/RPP/asphalt), which produces more complex polymer network structures, and the molecular chain winding may appear to increase the Van Der Waals Force, the chemical reactions between -OH from the polymers and asphalt has been basically completed at the 7% PP-g-MAH content, so |G*| of the TBM modified asphalt only increases slightly at 9% compared to 7% PP-g-MAH dosage.
The phase angle (δ) is defined as the time delay between the peak stress and strain in the sweep test. It can be used to evaluate the viscoelastic properties of the asphalt binder. The phase angle δ gradually decreases with the increase of PP-g-MAH dosage under the testing temperature condition from Figure 10, which indicates that TBM modified asphalt displays more elastic behaviors with the higher dosage of PP-g-MAH. The phase angle δ gradually increases with increasing temperature and at the same content of PP-g-MAH. The chemical reactions occur between PP-g-MAH and asphalt, light components of asphalt are absorbed by TBM chains and more complex TBM structures appear, which will be of importance to the above changes (Lu and Isacsson, 1997).
Elastic modulus (|G*|cosδ) is the elastic part of complex modulus, which refers to the energy storage modulus, it can be used to evaluate the elastic characteristic of modified asphalt. The variations of test temperature versus |G*|cosδ for different PP-g-MAH content are depicted in Figure 11. Under the same temperature conditions, |G*|cosδ value increases with the increasing of PP-g-MAH dosage, especially the change is more obvious at the lower temperature (40°C–50°C). However, at certain PP-g-MAH content, with the increase of the temperature, |G*|cosδ values decrease, as the |G*| values decrease and δ values increase (Figures 9, 10, respectively). For example, the |G*|cosδ at 70°C increases by 956.5% for different PP-g-MAH concentrations (from 0% to 9%), but it is only 585.3% at 90°C. Therefore, PP-g-MAH improves the ability of modified asphalt to return to its original state after loading.
Fatigue performance of the modified asphalt with TBM was evaluated by the fatigue parameter (|G*|sinδ), which is the energy loss of modified asphalt under cyclic load (Liu and Glover, 2015). It can be seen from Figure 12 that the fatigue performance of modified asphalt deteriorates with the addition of TBM. |G*|sinδ values increase gradually with the PP-g-MAH contents increase (from 0% to 7%). The possible reason is that new network structures may be formed from chemical reactions between PP-g-MAH and hydroxyl groups of RPE/RPP and the formation of physical crosslinking structures between molecular chains (Figure 6). It makes the modified asphalt much stiffer and reduces the fatigue performance compared to virgin asphalt. When the PP-g-MAH dosage increases from 7% to 9%, the change of |G*|sinδ value is less than that of 7%, because the reactions between PP-g-MAH and hydroxyl groups of RPE/RPP/asphalt have been completed, overall, the flexibility of PP-g-MAH material is beneficial to improve the fatigue resistance of modified asphalt (at 9% dosage, i.e., excess). According to specifications, the fatigue resistance parameter (|G*|sinδ) should have a maximum value of 5000 KPa up to 19°C, and lower values of |G*|sinδ are desirable from the standpoint of resistance to fatigue cracking, so it needs to be lucubrated in the future to meet practical engineering applications in different climate zones.
As shown in Figure 13, m-value gradually decreases in the range of 0%–5%, the reduction percent is 50.9%, but the m-value cannot meet the requirement (m-value>0.3) only at 5%–7% PP-g-MAH percentage. However, the m-value increases gradually in the other range (from 7% to 9%), the increasing ratio of m-value is about 66.7%, and the corresponding m-value is 0.45 (>0.3) at 9% PP-g-MAH dosage. With the increasing of PP-g-MAH content, the S value of modified asphalt gradually enhances except 9% dosage, and all S values are lower than 300 MPa that meets the requirements. The BBR testing results indicate that the proper PP-g-MAH concentration is critical to improving the low temperature of TBM modified asphalt. An opposite situation will occur when using the improper PP-g-MAH dosage, especially for m-value at −18°C.
Figure 14 illustrates the rutting depth and dynamic stability testing results of TBM modified asphalt with various PP-g-MAH content. The RD (rutting depth) decreases with the increase of PP-g-MAH content from 0% to 9%, regardless of the testing time (45 min or 60 min). The increase of PP-g-MAH content is conducive to improve the high-temperature performance of TBM modified asphalt mixture, which is consistent with the conclusions that waste plastics improve the dynamic stability of asphalt mixture (Polacco et al., 2005; Ahmedzade et al., 2014). It can be seen from Figure 14B that the change of PP-g-MAH content shows a more obvious effect on the dynamic stability between 0% and 3% PP-g-MAH dosage, but then the improvement is gentler with 3%–9% of PP-g-MAH. The main reason is that the chemical reaction and physical winding occur from the three different raw materials (RPE/RPP/PP-g-MAH/asphalt, Figure 6).
Although TBM-modified asphalt mixture shows a significant effect on the high-temperature performance, there are negative impacts on the fatigue resistance of asphalt modified by TBM. Therefore, a good balance should be considered on both sides, the optimum content of PP-g-MAH in the TBM should be selected to meet the practical use in road construction.
TBM was prepared with RPE, RPP, and PP-g-MAH (different content, i.e., 0%, 1%, 3%, 5%, 7%, and 9%) by twin-screw extruder, effects of PP-g-MAH dosage on the properties of asphalt and its mixture modified with TBM were analyzed, the main results were summarized as follows:
1) With an increase of PP-g-MAH content, needle penetration decreases gradually, viscosity and softening point of TBM modified asphalt increases inch by inch, ductility decreases first and then increases, and minimum value arises at 7% PP-g-MAH concentration.
2) PP-g-MAH improves the high-temperature performance (|G*|) of asphalt modified with TBM, especially in the range of [3%, 7%] than other PP-g-MAH percentage, but |G*|cosδ and δ decreases with the increase of PP-g-MAH content between 0% and 9%, however, |G*|sinδ of matrix bitumen is superior to asphalt modified by TBM within the experimental scale, so, with adding the amount 4% by weight of asphalt, in the process of TBM produced optimal PP-g-MAH content was 7% on the basis of total weight with RPE and RPP.
3) In the process of discussion and analysis of softening point, viscosity, |G*|, δ etc., the microscopic mechanism involved chemical reaction, which generates ester compounds in the high temperature between Maleic anhydride active groups of PP-g-MAH and hydroxyl groups from RPE/RPP, etc., and chain segment winding (the same chain, for instance, PP or PE) were proposed and expressed in the detailed description.
4) RD and DS show that TBM improves high-temperature properties of modified asphalt mixture, and m-value of TBM modified asphalt could fulfill the requirements (m-value>0.3) except for 5% and 7% PP-g-MAH content, and the S met the standard requirements (S < 300 MPa) completely in the under experimental conditions by BBR tests.
The original contributions presented in the study are included in the article/Supplementary Material, further inquiries can be directed to the corresponding author.
All authors listed have made a substantial, direct, and intellectual contribution to the work and approved it for publication.
This paper was supported by the Shanxi applied basic research program youth fund project (No. 201901D211552), and also was supported by Key Project of Hunan Provincial Education Department (No. 20A094).
Authors CF, SZ, XY, ZF, and GF were employed by the company Shanxi Transportation Technology Research & Development Co. Ltd.
The remaining authors declare that the research was conducted in the absence of any commercial or financial relationships that could be construed as a potential conflict of interest.
All claims expressed in this article are solely those of the authors and do not necessarily represent those of their affiliated organizations, or those of the publisher, the editors and the reviewers. Any product that may be evaluated in this article, or claim that may be made by its manufacturer, is not guaranteed or endorsed by the publisher.
Ahmedzade, P., Demirelli, K., Günay, T., Biryan, F., and Alqudah, O. (2015). Effects of Waste Polypropylene Additive on the Properties of Bituminous Binder. Proced. Manufacturing 2, 165–170. doi:10.1016/j.promfg.2015.07.029
Ahmedzade, P., Fainleib, A., Günay, T., and Grygoryeva, O. (2014). Modification of Bitumen by Electron Beam Irradiated Recycled Low Density Polyethylene. Construction Building Mater. 69, 1–9. doi:10.1016/j.conbuildmat.2014.07.027
Al-Hadidy, A. I., and Yi-qiu, T. (2009). Mechanistic Approach for Polypropylene-Modified Flexible Pavements. Mater. Des. 30 (4), 1133–1140. doi:10.1016/j.matdes.2008.06.021
Alghrafy, Y. M., Abd Alla, E.-S. M., and El-Badawy, S. M. (2021). Rheological Properties and Aging Performance of Sulfur Extended Asphalt Modified with Recycled Polyethylene Waste. Construction Building Mater. 273, 121771. doi:10.1016/j.conbuildmat.2020.121771
Ameri, M., Mohammadi, R., Mousavinezhad, M., Ameri, A., Shaker, H., and Fasihpour, A. (2020). Evaluating Properties of Asphalt Mixtures Containing Polymers of Styrene Butadiene Rubber (SBR) and Recycled Polyethylene Terephthalate (rPET) against Failures Caused by Rutting, Moisture and Fatigue. Frattura Ed. Integrità Strutturale. 14, 177–186. doi:10.3221/IGF-ESIS.53.15
Brovelli, C., Crispino, M., Pais, J., and Pereira, P. (2015). Using Polymers to Improve the Rutting Resistance of Asphalt Concrete. Construction Building Mater. 77, 117–123. doi:10.1016/j.conbuildmat.2014.12.060
Casey, D., McNally, C., Gibney, A., and Gilchrist, M. D. (2008). Development of a Recycled Polymer Modified Binder for Use in Stone Mastic Asphalt. Resour. Conservation Recycling 52 (10), 1167–1174. doi:10.1016/j.resconrec.2008.06.002
Colantonio, S., Cafiero, L., De Angelis, D., Ippolito, N. M., Tuffi, R., and Ciprioti, S. V. (2019). Thermal and Catalytic Pyrolysis of a Synthetic Mixture Representative of Packaging Plastics Residue. Front. Chem. Sci. Eng. 14, 288–303. doi:10.1007/s11705-019-1875-3
Costa, L. M. B., Silva, H. M. R. D., Peralta, J., and Oliveira, J. R. M. (2019). Using Waste Polym-Ers as a Reliable Alternative for Asphalt Binder Modification – Performance and Morphological Assessment. Construction Building Mater. 198, 237–244. doi:10.1016/j.conbuildmat.2018.11.279
Gibreil, H. A. A., and Feng, C. P. (2017). Effects of High-Density Polyethylene and Crumb Rubber Powder as Modifiers on Properties of Hot Mix Asphalt. Construction Building Mater. 142, 101–108. doi:10.1016/j.conbuildmat.2017.03.062
He, P., Chen, L., Shao, L., Zhang, H., and Lü, F. (2019). Municipal Solid Waste (MSW) Landfill: A Source of Microplastics? -Evidence of Microplastics in Landfill Leachate. Water Res. 159, 38–45. doi:10.1016/j.watres.2019.04.060
Jahromi, S. G., and Khodaii, A. (2009). Effects of Nanoclay on Rheological Properties of Bitumen Binder. Construction Building Mater. 23 (8), 2894–2904. doi:10.1016/j.conbuildmat.2009.02.027
Kalantar, Z. N., Karim, M. R., and Mahrez, A. (2012). A Review of Using Waste and Virgin Polymer in Pavement. Construction Building Mater. 33, 55–62. doi:10.1016/j.conbuildmat.2012.01.009
Keskisaari, A., and Kärki, T. (2018). The Use of Waste Materials in Wood-Plastic Composites and Their Impact on the Profitability of the Product. Resour. Conservation Recycling 134, 257–261. doi:10.1016/j.resconrec.2018.03.023
Liang, M., Sun, C., Yao, Z., Jiang, H., Zhang, J., and Ren, S. (2020). Utilization of Wax Residue as Compatibilizer for Asphalt with Ground Tire Rubber/Recycled Polyethylene Blends. Construction Building Mater. 230, 116966. doi:10.1016/j.conbuildmat.2019.116966
Liu, G., and Glover, C. J. (2015). A Study on the Oxidation Kinetics of Warm Mix Asphalt. Chem. Eng. J. 280, 115–120. doi:10.1016/j.cej.2015.05.074
Lu, X., and Isacsson, U. (1997). Rheological Characterization of Styrene-Butadiene-Styrene Copolymer Modified Bitumens. Construction Building Mater. 11 (1), 23–32. doi:10.1016/s0950-0618(96)00033-5
Ma, D., Zhao, D., Zhao, J., Du, S., Pang, J., Wang, W., et al. (2016). Functionalization of Reclaimed Polyethylene with Maleic Anhydride and its Application in Improving the High Temperature Stability of Asphalt Mixtures. Construction Building Mater. 113, 596–602. doi:10.1016/j.conbuildmat.2016.03.096
Minisstry of Transport of PR China (2011). Standard Test Methods of Bitumen and Bituminous Mixtures for Highway Engineering. Beijing: JTG E20-2011. (in Chinese).
Mohd Hasan, M. R., Colbert, B., You, Z., Jamshidi, A., Heiden, P. A., and Hamzah, M. O. (2016). A Simple Treatment of Electronic-Waste Plastics to Produce Asphalt Binder Additives with Improved Properties. Construction Building Mater. 110, 79–88. doi:10.1016/j.conbuildmat.2016.02.017
Navarro, F. J., Partal, P., Martínez-Boza, F. J., and Gallegos, C. (2010). Novel Recycled Polyethylene/Ground Tire Rubber/Bitumen Blends for Use in Roofing Applications: Thermo-Mechanical Properties. Polym. Test. 29 (5), 588–595. doi:10.1016/j.polymertesting.2010.03.010
Nien, Y.-H., Yeh, P.-H., Chen, W.-C., Liu, W.-T., and Chen, J.-H. (2008). Investigation of Flow Properties of Asphalt Binders Containing Polymer Modifiers. Polym. Compos. 29 (5), 518–524. doi:10.1002/pc.20404
Othman, A. M. (2010). Effect of Low-Density Polyethylene on Fracture Toughness of Asphalt Concrete Mixtures. J. Mater. Civ. Eng. 22 (10), 1019–1024. doi:10.1061/(asce)mt.1943-5533.0000106
Polacco, G., Berlincioni, S., Biondi, D., Stastna, J., and Zanzotto, L. (2005). Asphalt Modification with Different Polyethylene-Based Polymers. Eur. Polym. J. 41 (12), 2831–2844. doi:10.1016/j.eurpolymj.2005.05.034
Ragaert, K., Delva, L., and Van Geem, K. (2017). Mechanical and Chemical Recycling of Solid Plastic Waste. Waste Manage. 69, 24–58. doi:10.1016/j.wasman.2017.07.044
Stastna, J., Zanzotto, L., and Vacin, O. J. (2003). Viscosity Function in Polymer-Modified Asphalts. J. Colloid Interf. Sci. 259 (1), 200–207. doi:10.1016/s0021-9797(02)00197-2
Tapkın, S., Çevik, A., and Uşar, Ü. (2010). Prediction of Marshall Test Results for Polypropylene Modified Dense Bituminous Mixtures Using Neural Networks. Expert Syst. Appl. 37 (6), 4660–4670. doi:10.1016/j.eswa.2009.12.042
Yang, X. (2016). Technology and Engineering Applicationg of Asphalt Modified by Domestic Waste Old Plastics[M]. Beijing: Science Press. (in Chinese).
Yeh, P.-H., Nien, Y.-H., Chen, J.-H., Chen, W.-C., and Chen, J.-S. (2005). Thermal and Rheological Properties of Maleated Polypropylene Modified Asphalt. Polym. Eng. Sci. 45 (8), 1152–1158. doi:10.1002/pen.20386
Yin, S., Tuladhar, R., Shi, F., Shanks, R. A., Combe, M., and Collister, T. (2015). Mechanical Reprocessing of Polyolefin Waste: A Review. Polym. Eng. Sci. 55 (12), 2899–2909. doi:10.1002/pen.24182
Zhang, F., and Hu, C. (2015). The Research for Crumb Rubber/Waste Plastic Compound Modified Asphalt. J. Therm. Anal. Calorim. 124 (2), 729–741. doi:10.1007/s10973-015-5198-4
Zhang, H., Wu, X., Cao, D., Zhang, Y., and He, M. (2013). Effect of Linear Low Density-Polyethylene Grafted with Maleic Anhydride (LLDPE-G-MAH) on Properties of High Density-Polyethylene/Styrene-Butadiene-Styrene (HDPE/SBS) Modified Asphalt. Construction Building Mater. 47, 192–198. doi:10.1016/j.conbuildmat.2013.04.047
Keywords: asphalt, recycled polyethylene (RPE), recycled polypropylene (RPP), PP-g-MAH, properties
Citation: Zhang W, Hao X, Fan C, Zhang S, Ma D, Yu X, Fu Z and Feng G (2022) Effect of Polypropylene Grafted Maleic Anhydride (PP-G-MAH) on the Properties of Asphalt and its Mixture Modified With Recycled Polyethylene/Recycled Polypropylene (RPE/RPP) Blends. Front. Mater. 9:814551. doi: 10.3389/fmats.2022.814551
Received: 13 November 2021; Accepted: 19 January 2022;
Published: 27 April 2022.
Edited by:
Sanjeev Adhikari, Kennesaw State University, United StatesReviewed by:
Luke Yan, Chang’an University, ChinaCopyright © 2022 Zhang, Hao, Fan, Zhang, Ma, Yu, Fu and Feng. This is an open-access article distributed under the terms of the Creative Commons Attribution License (CC BY). The use, distribution or reproduction in other forums is permitted, provided the original author(s) and the copyright owner(s) are credited and that the original publication in this journal is cited, in accordance with accepted academic practice. No use, distribution or reproduction is permitted which does not comply with these terms.
*Correspondence: Wencai Zhang, emhhbmdrZWxhbjlAc2luYS5jb20=
Disclaimer: All claims expressed in this article are solely those of the authors and do not necessarily represent those of their affiliated organizations, or those of the publisher, the editors and the reviewers. Any product that may be evaluated in this article or claim that may be made by its manufacturer is not guaranteed or endorsed by the publisher.
Research integrity at Frontiers
Learn more about the work of our research integrity team to safeguard the quality of each article we publish.