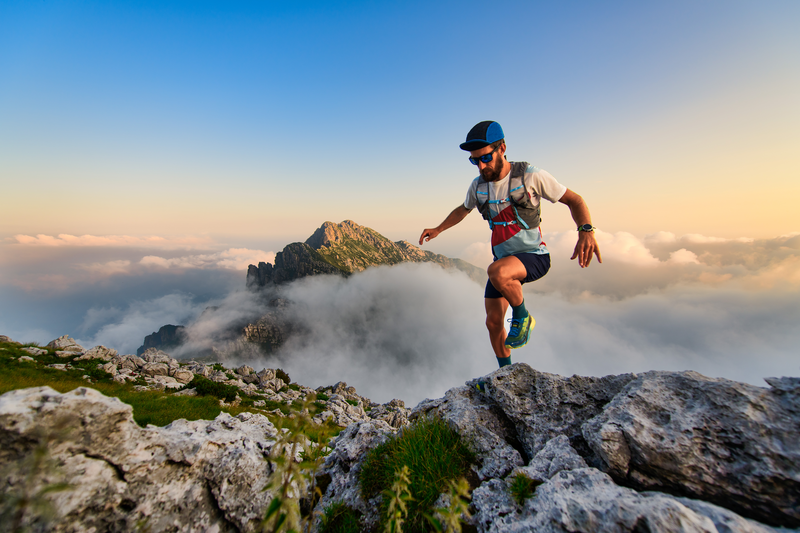
94% of researchers rate our articles as excellent or good
Learn more about the work of our research integrity team to safeguard the quality of each article we publish.
Find out more
ORIGINAL RESEARCH article
Front. Mater. , 21 December 2022
Sec. Structural Materials
Volume 9 - 2022 | https://doi.org/10.3389/fmats.2022.1064885
Development of concrete using alternative materials has become very important in the quest to achieve sustainable development in the built environment. However, it is critical to continually modify concrete mixtures to correct deficiencies of fresh and long-term properties. In this study, natural rubber latex and bamboo fiber were added as constituent materials in concrete, and the effects of the constituents on concrete were explored. Bamboo fiber (BF) and natural rubber latex (NRL) were added in proportions of 0%, 1%, and 1.5%. The study determined the workability (slump) of fresh concrete mixes, strength, and water absorption properties of the hardened samples after curing them in water for 7, 14, and 28 days. The morphology of the concrete samples was explored using SEM-EDX equipment. The results showed that samples having 1% bamboo fiber content and 1% rubber latex had the highest compressive strength among all the presented samples. Furthermore, samples containing equal but lower percentages of both bamboo and NRL had the highest compressive strength comparable to that of the control. This research showed the feasibility of combining bamboo fiber and rubber latex for an alternative eco-friendly construction approach to enhance the performance of conventional concrete in terms of tensile strength and flexural strength properties.
Concrete is a high-quality material used in the construction of various structures and can provide a degree of resistance to imposed loads within its design capacity in buildings (Jung, 2019). Concrete is good in compression, but a significant deficiency of concrete is its poor tensile strength, which has brought about the development of reinforced concrete. Steel bars support the concrete when subjected to forces that would place the structural member in tension (Awoyera et al., 2021). Another limitation of concrete material is the development of crack openings when exposed to excessive loads. However, conventional reinforcing steel is expensive and even scarce in some developing countries; hence, alternative materials such as fibers are utilized as a reinforcement to improve the properties of concrete.
Fibers used in concrete are grouped into synthetic fiber and natural fiber. Synthetic fibers include carbon, nylon, and plastic, while natural fibers can be obtained from animals and plants. Fibers obtained from animals include wool, fleece, and silk, while examples of fibers derived from plants are palm fiber, coconut fiber, ramin, and bamboo fiber (Hirde and Dudhal, 2016). Natural fiber is low-cost, readily available, and possesses appreciable mechanical properties. An example is bamboo fiber, which can help prevent contamination by collecting nitrogen from waste water, and it can reduce the carbon dioxide in its environment (Karthik et al., 2018).
The shape and dimension of the fiber used are significant. A short, hair-shaped fiber will affect the concrete after the first few hours by reducing cracks as the concrete stiffens/sets but will not cause any incremental rise in the tensile strength of the concrete (Haryanto et al., 2021).
Generally, fiber-reinforced concrete is known as a composite material that consists of concrete mixed with discrete, homogeneously dispersed, disjointed fibers (Aditya et al., 2017). The modification of concrete constituents using fibers and admixtures has become essential for enhancing the performance of concrete. According to Hirde et al. (Hirde and Dudhal, 2016), fiber-modified concrete demonstrates upgraded mechanical and durability properties, such as improved flexure strength, workability, tensile strength, and bonding strength. Conventional concrete reinforced with fibers is typically applied in pavements, but it has subsequently been utilized for the construction of beams and foundations, either unaided (mass concrete) or with rebars as seen in studies (Harison et al., 2017; Awoyera et al., 2021). The use of fibers and polymers has been shown to have advantages over regular construction materials in terms of high tensile strength-to-weight ratio, low maintenance cost, reduced permeability which lessens the bleeding of water, and resistance to impact, to mention but a few. These characteristics make fiber- and polymer-reinforced concrete composite a viable option in innovative construction (Ramesh et al., 2021).
This study focuses on the use of bamboo fiber and natural rubber latex as admixtures in concrete. Bamboo is a readily available plant in Asian and sub-Saharan African countries, and its processes are economical and can thus reduce the high demand for steel as reinforcement (Aswathi et al., 2017; Karthik et al., 2017; Shobha et al., 2018; Kumarasamy et al., 2020). Karthik (Karthik et al., 2017), based on an analysis of bamboo FTIR and SEM images, revealed that bamboo could be very suitable as a reinforcing material and could serve as a viable alternative for steel. Their study showed that bamboo material possessed sturdily bonded particles that could adequately serve as structural members that could be placed under compression and bending. Awoyera (Awoyera et al., 2015) described bamboo fiber as a material that limits the spread of cracks in concrete, causing a desirable delay in concrete’s ultimate failure. Their study revealed increases of about 81% and 101% in flexural strength and splitting tensile strength of high-strength concrete, respectively.
Natural rubber latex is a polymer that can be harvested from the Para rubber tree and some other trees. Latex simply refers to a polymer with a water-based liquid. Rubber trees are grown in South America, the United Kingdom, Indonesia, India, and Singapore. Natural rubber latex (NRL) is incorporated into the concrete mix as a polymer admixture. Latex-modified cement concrete is feasible because the addition of the latex significantly causes an increment in the flexural and compressive strength (Dewi et al., 2017). Natural rubber latex (NRL) has been investigated as an admixture in concrete. According to Aswathi, (Aswathi et al., 2017), there is an increase in the compressive strength and tensile strength of the concrete when NRL is added in small proportions. Other authors (Muhammad and Ismail, 2012; Vinaya et al., 2014) revealed that rubber latex-modified concrete displayed upgraded water exclusion capability and durability alongside improved compressive and flexural strength.
In this experimental work, bamboo fiber and natural polymer were considered as admixtures in a conventional concrete mix. When NRL is mixed into concrete, it changes the concrete’s porous microstructural characteristics into a denser matrix. Bashir et al. (Bashir et al., 2018), based on the SEM analysis conducted, revealed that there is a reduction in inter-particle gaps as the latex content rises, which indicates that latex could be used to restrict fluid flow within NRL-modified concrete samples.
Therefore, this study aims to evaluate the workability and mechanical properties of bamboo fiber-reinforced concrete containing natural rubber latex. Open literature findings have revealed experimental works that evaluated the behavior of fiber-reinforced concrete incorporating synthetic or natural fibers and tested it under different loadings such as compression, and tensile and flexural strength (Mohajerani et al., 2019; Ban et al., 2020; Najm and Ahmad, 2021). For instance, the results of a study (Ban et al., 2020), showed an increase of about 14% in flexural strength and compressive strength of composites reinforced with glycerol-modified bamboo fibers. However, there is limited information on experimental work to evaluate the effect of combining bamboo fiber and natural polymer resin rubber latex as raw materials in concrete on the workability, mechanical, and microstructural properties of concrete. Thus, this study aims to propose an appropriate constituent of bamboo and NRL for improving the above-mentioned properties of concrete. Hence, the obtained data from this study can serve as a guide for future studies and provide extensive data for natural polymer resin rubber latex and bamboo fiber concrete development.
This study investigated the workability, mechanical, and morphology properties of bamboo fiber-reinforced concrete containing natural rubber latex. The materials utilized in the preparation of the concrete specimens include Ordinary Portland cement, granite, river sand, bamboo fiber, natural rubber latex, and mixing water.
Ordinary Portland cement was used as the main binder in the mixture. The cement conforms to the ASTM C150/C150M (ASTM C150/C150M, 2001) requirements for type 1 Portland cement. The manufacturer-supplied physical and chemical properties of the cement used are presented in Tables 1, 2, respectively.
The fine aggregate used was river sand, and the portion of it passing through a 4.75 mm sieve was used in the preparation of concrete samples. The coarse aggregate was granite (crushed stone), and the portion used was retained on the 4.75 mm standard sieve. The bamboo fiber utilized in this research was sourced from Guadua bamboo and was processed into fibers via hydrolysis alkalization with multi-phase bleaching. In this process, the bamboo trunk’s inner pith was extracted and then crushed manually using a hammer. Thereafter, the crushed bamboo cellulose was soaked for 1—3 hours in a 20% solution of sodium hydroxide (NaOH) at about 23°C. This process is called steeping, and it forms alkali cellulose. Subsequently, excess NaOH was squeezed out of the bamboo cellulose and followed by shredding. Treatment of bamboo was carried out following procedures adopted in previous studies (Karthik et al., 2018; Awoyera et al., 2021; Haryanto et al., 2021).
The shredded bamboo cellulose was allowed to dry in the open air for 24 h, thus allowing the alkali cellulose to oxidize and reduce its weight. The bamboo cellulose was also sulfurized by the addition of carbide disulfide, making it turn into a gel. A viscose solution was created via the addition of a diluted solution of NaOH, and finally, the bamboo cellulose was passed through a spinneret nozzle and later returned to the cellulose bamboo fibers.
Natural rubber latex was added to the concrete mix as an admixture in various percentages as specified in the mix design. The bamboo fiber and natural rubber latex are shown in Figure 1. The chemical properties of NRL obtained from the manufacturer data sheet are presented in Table 3.
Portable water was used for the mixing and curing of the concrete produced in this study. The preliminary tests conducted on the aggregates utilized were done according to BS EN 932–3 (BS EN 932-3, 1997) criteria.
In this investigation, the batching of constituent materials for the production of concrete samples was done by the weight method. This method is more accurate, as it takes account of voids in granular materials. To examine the varying properties of concrete, bamboo fiber and NRL were added at various percentages.
The concrete mix ratio utilized in this research effort is 1:2:4, with a water-to-cement ratio (w/c) of 0.5. A total of five different concrete samples were produced for investigation in this research: the control concrete sample and four bamboo fiber-reinforced concrete samples containing NRL in proportions 0%, 1%, and 1.5%. The percentages of the four samples containing bamboo fiber (BF) and NRL are presented in Table 4. Bamboo fiber percentages were made constant for two samples where NRL varied, and vice versa, to allow for easy assessment of data derived from tests conducted on samples. No bonding improvement material was adopted in this study; however, the mixture was thoroughly mixed to enhance the bonding of the paste and NRL.
The workability of the concrete mixes was checked using the slump tests based on standard procedures (BS EN 12350-2, 2009). Following the casting and placing of concrete in molds, the test samples were removed from the mold after 24 h and immersed in a curing tank for a maximum of 28 days. Further tests involving the hardened properties of the concrete were carried out after each curing interval was completed.
The water absorption of the concrete mixes was determined following standard procedures (BS EN and 12350-2, 2006). Generally, it is known that the lower the concrete’s water absorption, the more durable the mixture. As such, the test was performed to guide the potential application of the concrete. The water absorption of the concrete samples was calculated using the equation below.
where W1 is the weight of the wet sample, and W2 is the weight of the dry sample.
In this study, the compressive strength of concrete was determined following the procedures of BS EN, 12390-3 (BS EN and 12390-3, 2003). The test gives the measurement of the concrete’s resistance to failure when subjected to compressive forces. Concrete samples were cured in water by immersion and tested after 7, 14, and 28 days. The compressive strength of hardened 150 mm cubes was determined by dividing the load at failure by the hardened concrete sample’s surface area. A total of 45 samples were cast, and they were tested in triplicate at the end of every curing regime. The average of the three strength values was noted as the flexural strength of each mix. Figure 2 shows samples of concrete and a sample loaded in the compression testing machine during testing. The compression machine is of UNIT TEST and made in West Malaysia.
The compressive strength was then calculated as follows:
This test was performed according to the standard procedures of BS EN, 12390-6 (BS EN and 12390-6, 2006) for the determination of the concrete’s tensile strength, which is a factor in the quality of concrete. Concrete cylinders of 150 × 300 mm were used for the test. A total of 45 samples were cast, and they were tested in triplicate at the end of every curing regime. The average of the three strength values was noted as the flexural strength of each mix. The applied tensile force to the concrete that causes it to fail is referred to as the concrete’s tensile strength. Generally, it is known that tensile forces are difficult for concrete to withstand. As a result, this problem is especially important in establishing the concrete’s efficiency.
where.
T = Splitting tensile strength (N/mm2).
P = Maximum applied load (N).
D = Diameter of the specimen (mm).
L = Length of the specimen (mm).
This test was performed in accordance with criteria outlined in BS EN, 12390-5 (BS EN and 12390-5, 2003) to determine the flexural strength of concrete by examining the capacity of a concrete sample to resist bending failure. Prisms of 100 × 100 × 500 mm were used for the test. A total of 10 samples were cast, and two samples per mix were tested after 28 days of curing. The average of the two strength values was noted as the flexural strength of each mix at the end of every curing regime. Reinforcements for the prism were 12 mm main bars (4Nos) and 6 mm stirrups. The loading rate was calculated using the following formula:
where
r = loading rate.
S = rate of increase of extreme fiber
b = average specimen width
d = average specimen depth.
L = span length.
Following the mechanical tests on the samples, the morphology of selected samples was obtained using scanning electron microscopy (SEM). The equipment uses image analysis to study the surfaces of materials to measure and analyze microstructures. In this study, SEM was carried out in the secondary electron mode by the use of carbon coating in the preparation of the samples. Images were captured at a maximum magnification of ×500. The tests also aided in the easy observation of component failures, detection of hidden particles, and analysis of the interaction of substances and substrates. Crushed concrete samples were used for the microstructural examination. This microstructural examination is important because the qualities of concrete samples are determined by their microstructural forms (Mehta and Monteiro, 2014).
The results of all the tests, including fresh and hardened concrete samples, are presented and analyzed in this section. A condensed summary of the data gathered is included, as well as insight and analysis of this. Multiple tests were performed on fresh and cured concrete samples to assess the samples’ overall general performance. This section examines the workability, mechanical, and water absorption test results. The microstructural studies are also presented.
Figure 3 presents the slump test values of the fresh concrete samples. It can be observed that Mix 0 (control) accounts for 24% of the total slump value, having the greatest slump and workability among the presented samples. Mix 1 accounts for 20% of the total slump value. A trend that can be observed from the data is that samples having higher percentages of rubber latex resulted in lower slump values. These results support the findings of Aswathi (Aswathi et al., 2017), where the conclusion was made that increasing the natural rubber latex content in concrete would cause a reduction in its workability. Moreover, NRL exhibits high water-absorbing properties that could also affect the workability of the concrete. However, the workability of the concrete generally was within the range of a true slump, which is acceptable for practical application as in the case of normal concrete.
Figure 4 shows the compressive strength values of the concrete samples. For all mixtures, whether with bamboo fiber and NRL or not, the compressive strength increased with the curing regime. Mix 1 had the most compressive strength after control, right from the early strength at 7 days down to the late age strength at 28 days. At 7 days, Mix 1 had a compressive strength of 14.67 N/mm2 which was 146.7% higher than Mix 3, which had the lowest compressive strength at 7 days across all the samples. Across all five samples, compressive strength at 7 days ranged from 10.00 N/mm2 to 16.44 N/mm2, compressive strength at 14 days ranged from 12.61 N/mm2 to 25.56 N/mm2, and compressive strength at 28 days ranged from 14.67 N/mm2 to 29.33 N/mm2. Mix 3 had the least compressive strength amongst all presented samples right from after 7 days of curing to 28 days. At 28 days, Mix 3 had a compressive strength of 14.67N/mm2, which is a poor performance when compared to the 29.33 N/mm2 strength of Mix 0 at 28 days. Mix 4 with same content of BF and NRL was the second-best concrete mix. A compressive strength of 20 N/mm2 was recorded for the mix after the maximum curing regime.
For all concrete specimens, 63% of the compressive strength at 28 days was achieved at 7 days, and more so, the compressive strength was reduced with an increasing percentage of bamboo fiber and natural rubber latex. Mix 1, having 1% bamboo fiber and 1% NRL, had the highest compressive strength comparable to that of control at 28 days, which is due to the minimal, balanced, and equal proportioning of bamboo fiber and NRL in the concrete samples. The compressive strength was reduced with higher content of NRL, and this could be a result of a poor reaction of NRL with the cementitious particles. As such, the rate of hydration of the particles is slowed down, leading to lower strength development.
To observe the effect of varying bamboo fiber content in the concrete mix, natural rubber latex was kept constant at 1% and 1.5%. At 7 days, having kept the NRL content constant at 1% and increasing the bamboo fiber content thereof, it was observed that the compressive strength dropped by 42%. It was also observed that when the NRL was kept constant at 1.5% and bamboo fiber was varied between 1% and 1.5%, the samples containing 1% bamboo fiber performed better than the samples with an increased percentage of bamboo fibers. This indicates that at early strength, increasing the bamboo fiber content reduces the concrete’s compressive strength. This result positively correlates with the findings of Dewiet et al. (Dewi et al., 2017), where it was observed that higher contents of bamboo fiber reduced the load capacity of the concrete samples tested.
A similar trend can be observed at 28 days where Mix 1, having 1% bamboo fiber and 1% NRL, performed better by a high margin than Mix 3, which had an increased percentage of bamboo fiber at the same NRL content. Though in this case, when NRL was kept constant at 1.5% and bamboo fiber content was increased thereof, it was observed that samples containing higher fiber content possessed a higher load capacity. These results could be attributed to the observed trend that samples containing an equal percentage of BF and NRL performed better than samples with varying percentages of the materials.
At 7 days, having kept the bamboo fiber content constant at 1% and increasing the rubber latex content, it was noticed that the compressive strength dropped but by a rather negligible margin. Mix 1 and Mix 2 had a difference in compressive strength of 0.67 N/mm2. When bamboo fiber was made constant at 1.5% and NRL was varied between 1% and 1.5%, it was observed that the sample containing a higher latex content had a better compressive strength. At 28 days, a similar trend could be observed, except that the strength gain for Mix 1 containing 1% NRL and 1% BF was rapid compared to the others; hence there was a rise in the strength difference between Mix 1 and Mix 2. Shobha (Shobha et al., 2018) also found 1% NRL giving the highest compressive strength amongst all samples tested.
Bamboo fiber and natural rubber latex were added to the concrete mix at equal percentages and increased simultaneously. It was noticed that samples having equal percentages of bamboo fiber and rubber latex had the highest compressive strength among all the presented samples. Furthermore, samples containing equal but lower percentages of both bamboo and NRL had the highest compressive strength comparable to that of the control. This implies that to get optimum compressive strength from the modified concrete, BF and NRL are to be added at equal percentages.
Figure 5 shows the splitting tensile strength values of the concrete samples. At 7 days, Mix 2 recorded the highest splitting tensile strength amongst all the samples, including the control mix, with a value of 1.83 N/mm2. It was 53% greater than Mix 3, which recorded the lowest splitting tensile strength at 7 days. Across all five samples, splitting tensile strength at 7 days ranged from 1.04 N/mm2 to 1.83 N/mm2. Results for 14 days correlated with that of 7 days with Mix 2 containing 1% BF and 1.5% NRL, which still had the highest strength amongst the samples excluding control, having the overall highest at this curing age. Splitting tensile strength at 14 days ranged from 1.43 N/mm2 to 2.15 N/mm2.
At 28 days, Mix 1 had the overall highest splitting tensile strength among all the samples including the control. Having a value of 2.42 N/mm2, Mix 1 surpassed the splitting tensile strength of control which had a value of 2.23 N/mm2. As observed with compressive strength, Mix 3 once again had the lowest results among the presented samples. Splitting tensile strength at 28 days ranged from 1.58 N/mm2 to 2.52 N/mm2.
For all concrete specimens, an average of 67% of splitting tensile strength at 28 days was achieved at 7 days. It was observed that splitting tensile strength was reduced with an increasing percentage of bamboo fiber and natural rubber latex. It was observed that samples having differing percentages of BF and NRL achieved the lowest splitting tensile strength, especially samples that had a higher percentage of BF than NRL. Mix 1, having 1% bamboo fiber and 1% NRL, had the highest splitting tensile strength, even greater than that of the control at 28 days. This can be attributed to the minute, balanced, and equal proportioning of bamboo fiber and NRL in the concrete samples.
To observe the effect of varying bamboo fiber (BF) content on splitting tensile strength, natural rubber latex was kept constant at 1% and 1.5%. At 28 days, having kept the NRL content constant at 1% and increasing the bamboo fiber content thereof, it was noticed that the splitting tensile strength dropped by 34%. It was also observed that when the NRL was kept constant at 1.5% and bamboo fiber was varied between 1% and 1.5%, the samples containing 1% bamboo fiber performed better than the samples with an increased percentage of bamboo fibers. This indicates that increasing the bamboo fiber content reduces the concrete’s splitting tensile strength. A somewhat similar trend can be observed from the 7-day results. Singh (Singh, 2016) also revealed that increasing the percentage of BF causes a decrease in tensile strength. This may be attributed to the effect of overlapping of fibers at high volume substitution.
At 28 days, by keeping the bamboo fiber content constant at 1% and increasing the rubber latex content, it was observed that the splitting tensile strength dropped. As observed with the compressive strength, it was by a rather negligible margin. Mix 1 and Mix 2 had a difference in splitting tensile strength of 0.1 N/mm2. When bamboo fiber was kept constant at 1.5% and NRL was varied between 1% and 1.5%, it was observed that the sample containing a higher latex content had a better splitting tensile strength. When NRL is added to a concrete mix, there is a dispersion effect of the polymer to fill void spaces in the cement, which could explain the increased tensile strength of the samples. However, this could also be attributed to the fact that the sample with a higher percentage of NRL had an equal percentage of BF. This has been observed to be the optimum mode of application of these constituents.
Bamboo fiber and natural rubber latex were added to the concrete mix at equal percentages and raised at the same time to demonstrate this. The samples with the same percentages of bamboo fiber and rubber latex had the highest splitting tensile strength of all the samples tested. Furthermore, samples with equal but smaller percentages of bamboo and NRL had the highest splitting tensile strength, surpassing the control. This indicates that adding BF and NRL at equal but small percentages increases the splitting tensile strength.
Figure 6 shows the flexural strength values of the concrete samples. At 28 days, the control had the highest flexural strength among all presented samples. However, this could be attributed to the fact that the control mix was cast with steel offcuts to get an idea of how BF- and NRL-modified concrete would perform in comparison to steel reinforcements. Amongst modified samples, Mix 4 recorded the highest flexural strength at 15 N/mm2, which was 33% greater than Mix 3, having the poorest result at 10 N/mm2. Across all samples, flexural strength ranged from 10 N/mm2 to 40 N/mm2. Mix 4, which had the highest flexural strength amongst the modified samples, contained 1.5% BF and 1.5% NRL.
To observe the effect of varying BF content on the flexural strength, once again natural rubber latex was kept constant at 1% and 1.5%. At 28 days, having kept the NRL content constant at 1% and increasing the bamboo fiber content thereof, it was noticed that flexural strength dropped by 29%. Although this wasn’t the case when the NRL was kept constant at 1.5% and bamboo fiber was varied between 1% and 1.5%, the samples containing 1.5% bamboo fiber performed better than the samples with a lower percentage of bamboo fibers.
As observed from the results, having kept the BF content constant at 1% and increasing the rubber latex, a drop in flexural strength was observed. Mix 1 containing 1% NRL and Mix 2 containing 1.5% NRL had a difference of 2 N/mm2. When BF was made constant at 1.5% and NRL was varied between 1% and 1.5.%, the latter had a higher flexural strength. This indicates that increasing the amount of NRL at higher percentages of bamboo fiber results in higher flexural strength. Hirde et al. (Hirde and Dudhal, 2016) also concluded that the addition of this natural polymer would have a positive effect on the concrete’s flexural strength.
Bamboo fiber and natural rubber latex were added to the concrete mix at equal percentages and raised simultaneously to demonstrate this. As observed in the other mechanical tests, the samples with the same percentage of bamboo fiber and rubber latex had the highest performance among all the modified samples tested. A key difference between the results of this test and the others was that samples with equal and a higher percentage of bamboo and NRL had the highest flexural strength.
The water absorption rate in concrete in the curing tank was evaluated during final testing at 28 days. The water absorption was evaluated by estimating the weight variation of the sample before curing and post-curing. In essence, this procedure helps to explore the possible application of NRL concrete in a water-logged environment. Figure 7 shows the water absorption values of the concrete samples. At 9.2%, Mix 3 had the highest absorption at 28 days and was 161.40% higher than Mix 2, which had the lowest water absorption at 5.7%. Mix 3 accounted for 26.58% of the water absorption at 28 days. Across all five samples, water absorption at 28 days ranged from 5.7% to 9.2%.
Mix 2, containing 1% BF and 1.5% NRL, had the lowest levels of water absorption, hence had the best water exclusion capacity. This could be attributed to the increased NRL percentage present in the mix. NRL disperses in concrete to fill its void spaces and forms a latex film which improves the water exclusion capabilities of the concrete. Muhammad et al. (Muhammad and Ismail, 2012) also found this to be the case. Mix 3, which had the lowest water exclusion capability, contained 1.5% BF and 1% NRL; hence, an increased fiber content paired with a reduced latex content will give an increased water absorption percentage, and vice versa.
Based on the experimental analysis carried out, Mix 1 has been selected as the optimum mix. Mix 1 contains 1% BF and 1.5% NRL. Table 5 compares the test values of the optimum concrete mix and control samples.
From the slump test, the optimum concrete mix is 16.67% lower than the concrete control; hence, it had a reduced fluidity than the concrete control. Mix 1 had a greater splitting tensile strength, a 13.64% loss in compressive strength, and a 65% loss in flexural strength. Mix 0 (concrete control) had 29.33 N/mm2 compressive strength at 28 days, 40 N/mm2 flexural strength, and 2.23 N/mm2 splitting tensile strength at 28 days. Mix 1 had 25.33 N/mm2 compressive strength at 28 days, 14 N/mm2 flexural strength at 28 days, and 2.42 N/mm2 splitting tensile strength at 28 days.
Figure 8 shows the SEM and oxide composition for the mix. According to the data from Energy Dispersive X-ray analysis in the figure, Mix 0 (control mix) has an oxygen content of 74.08%, 11.38% nitrogen, 8.31% silicon, 4.92% calcium, 4.92% calcium, and 1.31% aluminum.
There are a few cavities in the sample and calcium silicate hydrate (C-S-H) gel production. The distribution of C-S-H decreases voids and boosts the specimen’s strength. The rather porous textured features displayed in this matrix indicate a level of porosity typical of regular mixtures (Castillo-Lara et al., 2020; Sanchez-Echeverri et al., 2020; Zhang et al., 2020; Caballero-Jorna et al., 2021; Aisheh et al., 2022; Najm et al., 2022). Depending on the amount of latex in the mix, modified mixes on the other hand have displayed more compact characteristics.
Figure 9 shows the SEM and oxide composition for Mix 1 at 28 days. Mix 1 (optimum mix) has an oxygen content of 71.09%, 11.88% nitrogen, 7.82% silicon, 3.83% calcium, 1.56% magnesium, 1.47% bromine, 1.46% sodium, and 0.91% iron, according to the data from Energy Dispersive X-ray analysis.
There is a dispersion of NRL in its liquid state in concrete to fill void spaces. Less obvious voids in the SEM images in Figure 9 demonstrate this. In its liquid state, NRL consists majorly of the distribution of poly 1–4 isoprene particles. However, as the dispersing medium, which is often water, is drained away, inter-particle spacing decreases until fusion is achieved, thereby resulting in a continuous film that coats cement oxides and aggregates as seen below.
Figure 10 shows the SEM and EDX for Mix 3. The Energy Dispersive X-ray analysis of Mix 3 (worst performer) has an oxygen content of 73.74%, a 14.53% silicon content, 5.06% sodium, 3.28% bromine, 1.12% iron, 1.03% magnesium, and 0.80% calcium.
As shown, there was an increased percentage of bamboo fiber that restricted the distribution of the NRL’s poly 1–4 isoprene particles in the concrete, as more voids can be observed compared to the sample with a lesser amount of fiber. This could be a major factor behind the poor strength performance exhibited by this sample.
This research concludes by suggesting the use of bamboo fiber and natural rubber latex in the production of concrete. The workability properties of the modified concrete samples were compared to that of conventional concrete. The mechanical and durability characteristics of fiber-reinforced polymer concrete samples were compared to the mechanical and durability characteristics of conventional concrete. The microstructural properties and appearance of the fiber-reinforced natural polymer concrete samples were also analyzed. Specifically, the compressive strength, splitting tensile strength, flexural strength, and water absorption were tested and compared to determine the effects of combining bamboo fibers and naturally occurring rubber latex in the production of concrete.
The following points can be concluded from this research:
• The workability properties of fresh BF-reinforced concrete containing NRL were slightly less than the workability properties of fresh control concrete.
• For optimum performance, BF and NRL should be incorporated into concrete production at equal and reduced percentages.
• Mix 1 emerged as the best concrete mix, as it attained 13.63% compressive strength in comparison with the control concrete. At 25 N/mm2, Mix 1 surpassed the BS8110 standard strength requirement for grade 1:2:4 concrete.
• Mix 4, which also contained an equal proportion of BF and NRL, emerged as the second-best concrete mix. At 20 N/mm2, Mix 4 also surpassed the BS8110 standard compressive strength requirement for grade 1:2:4 concrete.
• The SEM-EDX analysis results indicated that at higher bamboo fiber content without a corresponding increase in natural rubber latex content, there would be an increase in the void spaces present in the concrete, causing a reduction in strength.
• Higher latex content than fiber content would result in improved water exclusion capabilities.
The combination of bamboo fiber and natural rubber latex is feasible but has to be incorporated at equal and minimum percentages for balanced performance. Although higher flexural strength could be achieved at higher percentages of both materials, to get an overall balance in performance both constituents should be added at reduced percentages.
The raw data supporting the conclusion of this article will be made available by the authors, without undue reservation.
Project supervision, writing—review and editing MK, FA; Conceptualization, formal analysis writing—original draft preparation PA, investigation, writing—review and editing MM, KI; Review, formal analysis, writing—review HH, HN. All authors have read and agreed to the published version of the manuscript.
This work was funded by the Department of Medical Research, China Medical University Hospital, China Medical University, Tai-chung, Taiwan.
The authors are thankful to the Deanship of Scientific Research at Najran University for funding this work under the National Research Priorities funding program grant code (NU/NRP/SERC/11/23).
The authors declare that the research was conducted in the absence of any commercial or financial relationships that could be construed as a potential conflict of interest.
All claims expressed in this article are solely those of the authors and do not necessarily represent those of their affiliated organizations, or those of the publisher, the editors and the reviewers. Any product that may be evaluated in this article, or claim that may be made by its manufacturer, is not guaranteed or endorsed by the publisher.
The Supplementary Material for this article can be found online at: https://www.frontiersin.org/articles/10.3389/fmats.2022.1064885/full#supplementary-material
Aditya, T., Anushree, S., Diya, V., and Jerin, A. (2017). Coconut fibre reinforced concrete. Amal Jyothi College of Engineering, Kanjirappally, Kerala, India: Unpublished B.Tech Thesis.
Aisheh, Y. I. A., Atrushi, D. S., Akeed, M. H., Qaidi, S., and Tayeh, B. A. (2022). Influence of polypropylene and steel fibers on the mechanical properties of ultra-high-performance fiber-reinforced geopolymer concrete. Case Stud. Constr. Mater. 17, e01234. doi:10.1016/j.cscm.2022.e01234
Astm C150/C150M, (2001). Standard specification for Portland cement. Philadelphia, PA: Annual book of ASTM standards.ASTM.
Aswathi, K. P., Hanna, P., George, J. K., and Shameer, K. (2017). Effect of natural rubber latex as admixtures in concrete. Int. Res. J. Eng. Technol. 4, 2031–2034.
Awoyera, P. O., Ijalana, J. K., and Babalola, O. E. (2015). Influence of steel and bamboo fibres on mechanical prop-erties of high strength concrete. J. Mater. Environ. Sci. 6, 3634–3642.
Awoyera, P. O., Nworgu, T. A., Shanmugam, B., Arunachalam, K. P., Mansouri, I., Miguel, L., et al. (2021). Structural retrofitting of corroded reinforced concrete beams using bamboo fiber laminate. Materials 14, 6711. doi:10.3390/ma14216711
Ban, Y., Zhi, W., Fei, M., Liu, W., Yu, D., Fu, T., et al. (2020). Preparation and performance of cement mortar reinforced by modified bamboo fibers. Polymers 12 (11), 2650. doi:10.3390/polym12112650
Bashir, A., Gupta, C., Abubakr, M. A., and Abba, S. I. (2018). Analysis of bamboo fibre reinforced beam. J. Steel Struct. Constr. 04, 2–7. doi:10.4172/2472-0437.1000146
Bs En, 12390-3, (2003). Testing hardened concrete – part 3: Compressive strength of test specimens. London, UK: British Standard.
Bs En, 12390-5, (2003). Testing hardened concrete Part 5: Flexural strength of test specimens. London, UK: British Standard.
Bs En, 12390-6, (2006). Testing hardened concrete – part 6: Tensile Splitting strength of test specimens. London, UK: British Standard.
Caballero-Jorna, M., Roig-Flores, M., and Serna, P. (2021). A study of the flexural behavior of fiber-reinforced concretes exposed to moderate temperatures. Materials 14 (13), 3522. doi:10.3390/ma14133522
Castillo-Lara, J. F., Flores-Johnson, E. A., Valadez-Gonzalez, A., Herrera-Franco, P. J., Carrillo, J. G., Gonzalez-Chi, P. I., et al. (2020). Mechanical properties of natural fiber reinforced foamed concrete. Materials 13 (14), 3060. doi:10.3390/ma13143060
Dewi, S. M., Wijaya, M. N., and Remayanti, N. C. (2017). The use of bamboo fiber in reinforced concrete beam to reduce crack. AIP Conf. Proc. 020003 (2017), 1887. doi:10.1063/1.5003486
Harison, A., Agrawal, A., Imam, A., and Harison, A. (2017). Bamboo as an alternative to steel for green construc-tion towards low-cost housing. J. Environ. Nanotechnol. 6, 100–104. doi:10.13074/jent.2017.06.172257
Haryanto, Y., Wariyatno, N. G., Hu, H. T., Han, A. L., and Hidayat, B. A. (2021). Investigation on structural behavior of bamboo reinforced concrete slabs under concentrated load. Sains Malays. 50, 227–238. doi:10.17576/jsm-2021-5001-22
Hirde, S. K., and Dudhal, O. S. (2016). Review on polymer modified concrete and its application to concrete struc-tures. Int. J. Eng. Res., 766–769.ISSN.
Karthik, S., Rao, P. R. M., and Awoyera, P. O. (2017). Strength properties of bamboo and steel reinforced concrete containing manufactured sand and mineral admixtures. J. King Saud. Univ. - Eng. Sci. 29, 400–406. doi:10.1016/j.jksues.2016.12.003
Karthik, S., Rao, R. M., Awoyera, P., Akinwumi, I., Karthikeyan, T., Revathi, A., et al. (2018). Beneficiated pozzolans as cement replacement in bamboo-reinforced concrete: The intrinsic characteristics. Innov. Infrastruct. Solut. 50 (2018), 3. doi:10.1007/s41062-018-0157-0
Kumarasamy, K., Shyamala, G., Gebreyowhanse, H., and Kumarasamy, (2020). Strength properties of bamboo fiber reinforced concrete. {IOP} Conf. Ser. Mat. Sci. Eng. 981, 032063. doi:10.1088/1757-899x/981/3/032063
Mehta, P. K., and Monteiro, P. J. (2014). Concrete: Microstructure, properties, and materials. Columbus: McGraw-Hill Education.
Mohajerani, A., Hui, S. Q., Mirzababaei, M., Arulrajah, A., Horpibulsuk, S., Abdul Kadir, A., et al. (2019). Amazing types, properties, and applications of fibres in construction materials. Materials 12 (16), 2513. doi:10.3390/ma12162513
Muhammad, B., and Ismail, M. (2012). Performance of natural rubber latex modified concrete in acidic and sul-fated environments. Constr. Build. Mat. 31, 129–134. doi:10.1016/j.conbuildmat.2011.12.099
Najm, H. M., and Ahmad, S. (2021). The effect of metallic and non-metallic fiber on the mechanical properties of waste ceramic concrete. Innovative Infrastructure Solutions 6 (4), 204–215. doi:10.1007/s41062-021-00571-4
Najm, H. M., Nanayakkara, O., and Sabri, M. M. S. (2022). Destructive and non-destructive evaluation of fibre-reinforced concrete: A comprehensive study of mechanical properties. Materials 15 (13), 4432. doi:10.3390/ma15134432
Ramesh, M., Deepa, C., and Ravanan, A. (2021). “Bamboo fiber reinforced concrete composites,” in Bamboo fiber composites: Processing, properties and applications. Editors M. Jawaid, S. Mavinkere Rangappa, and S. Siengchin (Singapore: Springer), 127–145.SingaporeISBN 978-981-15-8489-3.
Sanchez-Echeverri, L. A., Medina-Perilla, J. A., and Ganjian, E. (2020). Nonconventional Ca (OH) 2 treatment of bamboo for the reinforcement of cement composites. Materials 13 (8), 1892. doi:10.3390/ma13081892
Shobha, S., Shashidhar, C., and Sudarsana, R. (2018). Strength studies of natural rubber latex modified high per-formance concrete. International Journal of Engineering Research & Technology (IJERT) 2.
Singh, B. P. V. K. (2016). Experimental investigation on concrete using bamboo fibre as partial replacement for course aggregate in concrete. IJSRD-International J. Sci. Res. Dev. 4, 2321–0613.
Vinaya, K. L., Sanjith, J., Ranjith, A., and Kiran, B. M. (2014). Effect of natural rubber latex on normal and high strength concrete. Int. J. Adv. Eng. Res. Sci., 8354, 344–355.
Keywords: natural rubber latex, bamboo fiber, microscale analysis, mechanical properties, concrete
Citation: Althoey F, Awoyera PO, Inyama K, Khan MA, Mursaleen M, Hadidi HM and Najm HM (2022) Strength and microscale properties of bamboo fiber-reinforced concrete modified with natural rubber latex. Front. Mater. 9:1064885. doi: 10.3389/fmats.2022.1064885
Received: 08 October 2022; Accepted: 02 December 2022;
Published: 21 December 2022.
Edited by:
Jialai Wang, University of Alabama, United StatesReviewed by:
Nur Hafizah A. Khalid, University of Technology Malaysia, MalaysiaCopyright © 2022 Althoey, Awoyera, Inyama, Khan, Mursaleen, Hadidi and Najm. This is an open-access article distributed under the terms of the Creative Commons Attribution License (CC BY). The use, distribution or reproduction in other forums is permitted, provided the original author(s) and the copyright owner(s) are credited and that the original publication in this journal is cited, in accordance with accepted academic practice. No use, distribution or reproduction is permitted which does not comply with these terms.
*Correspondence: Mohammad Mursaleen, TXVyc2FsZWVuQG1haWwuY211aC5vcmcudHc=, bXVyc2FsZWVubUBnbWFpbC5jb20=
Disclaimer: All claims expressed in this article are solely those of the authors and do not necessarily represent those of their affiliated organizations, or those of the publisher, the editors and the reviewers. Any product that may be evaluated in this article or claim that may be made by its manufacturer is not guaranteed or endorsed by the publisher.
Research integrity at Frontiers
Learn more about the work of our research integrity team to safeguard the quality of each article we publish.