- 1Department of Civil Engineering, Faculty of Engineering, Mugla Sitki Kocman University, Mugla, Turkey
- 2Department of Civil Engineering, Faculty of Engineering, Necmettin Erbakan University, Konya, Turkey
- 3Department of Construction, Tomarza Mustafa Akincioglu Vocational School, Kayseri University, Kayseri, Turkey
- 4Structural Engineering and Construction Department, Future University in Egypt, New Cairo, Egypt
- 5Department of Civil Engineering, University of Engineering and Technology Peshawar (Bannu Campus), Bannu, Pakistan
- 6Peter the Great St. Petersburg Polytechnic University, St. Petersburg, Russia
With the increasing number of vehicles in the world, the amount of waste tires is increasing day by day. In this case, the disposal of expired tires will cause serious environmental problems. In recent years, instead of disposing of tire wastes, most of them have been started to be recycled to produce fiber-reinforced concrete. Thus, steel fibers recovered from waste tires have been preferred as an alternative to industrial steel fibers due to their environmentally friendly and low-cost advantages. In this study, an experimental study was carried out to explore the effect of fiber content on the fresh and hardened state of the concrete. To achieve this goal, compression, splitting tensile, and flexure tests were carried out to observe the performance of the concrete with tire-recycled steel fibers with the ratios of 1%, 2% and 3%. There is an improvement in the mechanical properties of the concrete with the increase of the volume fraction of the steel fiber. However, a significant reduction in workability was observed after the addition of 2% steel fibers. Therefore, it is recommended to utilize 2% tire-recycled steel fibers in practical applications. Furthermore, experimental results of concrete with tire-recycled steel fibers were collected from the literature and empirical equations based on these results were developed in order to predict the compressive and splitting tensile strengths.
1 Introduction
Concrete consists of various ingredients, including cement, aggregates (fine and coarse), water, and some additives and it is widely used as a building material in the civil engineering applications such as dams, bridges, and roads (Shukla, 2013; Vieira et al., 2016; Seetharam et al., 2017; Alabi, 2020; Prabu et al., 2020; Zeyad, 2020; Alwesabi et al., 2021; Magbool and Zeyad, 2021; Ming et al., 2021; Abdullah et al., 2022; Adil et al., 2022; Zeyad et al., 2022). However, it has many shortcomings such as brittleness and low tensile strength. One way to eliminate these drawbacks is to use classical/conventional reinforcements, i.e., transverse, and longitudinal reinforcement. Longitudinal reinforcement resists tensile or compressive stresses. They are in the form of steel bars and placed at a specific interval in the element cross-section. Transverse reinforcement such as stirrups and ties are used to withstand shear. To mitigate shear failures, the number of transverse reinforcements is increased in the critical zones. However, this can lead to increase costs and labor demands (Yuan et al., 2020; Zamanzadeh et al., 2015; Meda et al., 2005; Failla et al., 2002). In this case, the common and efficient way is to use a certain number of fibers. Different types of fibers such as steel, glass, natural and synthetic have been widely used as reinforcement in the concrete mixture. Steel fibers are the most used fiber type to amplify the mechanical properties of concrete (Anil, 2018; Jabir et al., 2020) and they are employed in the form of short discrete lengths and different ratios of length to diameter varying between 20 and 100 in the mixture (Behbahani et al., 2011). According to some experimental studies on beams (Zamanzadeh et al., 2015; Failla et al., 2002; Jabir et al., 2020), steel fibers provide a similar -post-cracking performance when compared to beams reinforced with steel stirrups. Furthermore, steel fibers assist to enhance shear capacity, which leads to reduced crack width. In this way, the failure pattern changes from brittle shear to ductile flexural failure (Zamanzadeh et al., 2015; El-Sayed, 2019). Thus, to obtain the desired quality in concrete, it is also widely preferred to replace the stirrups in the concrete mixture completely or partially with steel fibers (Rashid and Balouch, 2017). Either industrial steel fibers or recycled steel fibers or hybrid fibers (industrial and recycled) are utilized as a reinforcement (Aghaee et al., 2015; Leone et al., 2016; Caggiano et al., 2017; Domski et al., 2017; Hu et al., 2018; Carrillo et al., 2020; Simalti and Singh, 2020). After observing the good performance of industrial steel fibers on concrete, it was concentrated on steel fibers obtained from wastes. Furthermore, research on obtaining fiber from waste materials other than natural resources or raw materials has increased since production of the industrial steel fibers has disadvantages such as greenhouse gas emissions and high cost (Groli et al., 2014; Sengul, 2016; Mastali et al., 2018; Zheng et al., 2018; Isa et al., 2020; Liew and Akbar, 2020; Gul et al., 2021; Qin and Kaewunruen, 2022).
The mostly used recycled steel fibers are obtained from expired vehicle tires (Qin and Kaewunruen, 2022). A typical tire generally consists of carbon, rubbers, steel wire, and additives (Ahmed et al., 2021). Although the service life of vehicle tires vary depending on the road type, usage, and material quality in the tires, it can reach approximately 5 years or up to 40000 km (Sengul, 2016; Barišić et al., 2021). The increase in the number of vehicles in recent years will lead to an increase in the number of wastes after the life span of tires. Then, waste and disposal stage may lead to a huge threat to the environment and high cost. Thus, instead of disposal of waste, recycling, and reuse of them in many industries are viable solutions (Karalar et al., 2022a; Alani et al., 2022; de Azevedo et al., 2022; Martínez-García et al., 2022; Qaidi et al., 2022; Zeybek et al., 2022). In addition to being environmentally friendly, steel fibers extracted from waste are more economical when compared to industrial steel fiber (Ghorpade and Rao, 2010; Mastali et al., 2018; Liew and Akbar, 2020). Many research studies were conducted to investigate the effect of tire-recycled steel fibers on the mechanical properties of concrete. Wu et al. (2016) investigated the impact of tire-recycled fibers on the behavior of concrete materials and it was shown that steel fiber lessens crack width in the concrete. Pilakoutas et al. (2004) studied the reuse of tire steel fibers as a reinforcement in concrete. They mentioned that recovered steel fibers may be replaced by conventional reinforcements, and they can help shorten construction time. Neocleous et al. (2006) evaluated the suitability of existing design guidelines for the concretes with fibers recovered from used and tried to propose a more appropriate approach for flexural design. They also developed a stress-strain curve to obtain the flexural strength of concrete with recycled fibers. Aiello et al. (2009) conducted pull-out tests on concrete with tire-recycled steel fibers obtained by the mechanical process to investigate adhesion between the concrete and the fiber matrix. According to the study, there should be an allowable volume ratio of fibers for the workability of concrete. For a traditional concrete mixer, this ratio should be less than 0.26%. However, it can be used up to 0.46% for a planetary mixer. A study conducted by Aghaee and Yazdi, (2014) stated that the usage of waste wires in concrete provides a positive effect on the mechanical properties of lightweight concrete specimens. Especially, waste wire provides high tensile and flexural strength to the concrete. Rashid and Balouch, (2017) studied the effect of steel fibers recovered from waste tires on the shear resistance of reinforced concrete beams considering four-point bending test. In addition to shear resistance, failure modes, crack propagation and width were also investigated. Workability of concrete with tire-recycled steel fiber is decreased when the amount of fibers increases. Köroğlu, (2018) searched the usage of recycled steel fibers from waste tires in functionally graded self-compacting concrete. Mechanical properties of the concrete were investigated for fresh and hardened conditions. It has been shown that the workability of fresh concrete reduces with increasing fiber content. The effect of steel fiber extracted from waste tires on the mechanical performance of concrete was examined by Zhang and Gao, (2020). Compressive strength, tensile strength, and toughness properties were compared with those of industrial fiber reinforced concrete (IFRC), taking into account different dosages. Gul et al. (2021) carried out a study to investigate the performance of concrete with recycled steel fiber extracted from waste tires. Fiber length and dosages were selected prime variables in their study. They tried to obtain the optimum dosage and length of the steel fibers used in the concrete mixture. Revuelta et al. (2021) compared mechanical behaviors of tire-cycled steel fiber reinforced concrete with those of industrial steel fibers. It has been shown that tire-recycled steel fibers and industrial steel fibers provide similar performance when the same amount of fiber is used. Senesavath et al. (2022) investigated the influence of purified and non-purified recycled tire steel fiber on fresh and hardened concrete performance. Their study showed that purified recycled tire steel fiber provides better tensile strength when compared to non-purified recycled tire steel fiber. Singh and Kaushik, (2001) and (Frančić Smrkić et al., 2017), studied concrete with steel fibers under fatigue loading. Frančić Smrkić et al. (2017) mentioned that current design models underestimate fatigue resistance of the fiber reinforced concrete and they proposed more rational model for this type of concrete.
The above-mentioned studies showed the usability of recycled steel fiber recovered from waste tires in civil engineering applications. However, there is a research need for the optimum amount of fiber in concrete. To pursue this goal, the performance of the concrete with steel fibers generated from tire wastes was investigated and compared by considering different volume fractions of steel fiber. An experimental study was conducted on some test specimens consisting of recycled steel fibers. For each test specimen, the effect of recycled steel fibers on the physical and mechanical properties of concrete was studied for fresh and hardened cases. Optimum fiber dosages were identified for concrete with tire-recycled steel fibers based on the fresh and hardened properties. More importantly is that very few empirical equations to predict the capacities of the concrete with recycled steel fibers from tires are available in the literature. To achieve this aim, the studies were collected from the literature and empirical equations were developed to predict the compression and splitting tensile capacities.
2 Experimental program
2.1 Materials and mixture proportions
CEM I 32.5 Portland Cement was utilized in this study and its chemical properties are depicted in Table 1. Maximum fine aggregate sizes of 4 mm and coarse aggregate sizes of 12 mm were utilized. The ratio of water to cement was selected as 0.60 while the ratio of cement to aggregate was selected as 0.22. The ratio of fine aggregate (0–4 mm) was around 48% while the ratio of coarse aggregate (4–12 mm) was around 52%. In mix design, 360 kg/m3 cement, 180 kg/m3 water, 790 kg/m3 fine aggregate and 850 kg/m3 coarse aggregate were added.
The steel wires obtained from tires were utilized to investigate the influence of fiber amount on the mechanical properties in terms of compressive strength, split tensile strength, and bending performance of concrete. The utilized steel wires are shown in Figure 1A. The shape of recycled steel wires obtained from tires was linear. The length of the recycled steel wires was divided into small portions before being used it. Yoo et al. (2017) revealed in their study that the medium-length of fibers exhibited higher flexural strengths compared to the longer fiber. Therefore, it was tried to obtain the same length proportion not larger than 5 cm in length to get optimum results. Figure 1B demonstrates the proportion of the lengths utilized in the steel wires. In this study, a reference mixture including no fiber and six mixtures were designed. Three different fiber volume ratios including 1%, 2%, and 3% were studied.
2.2 Mix procedure, workability, and slump test
For the mixing procedure, all aggregates, cement, and water were initially mixed in the mixer. Then, the required recycled steel wires were mixed into small pieces to prevent aggregation and to accomplish the uniform distribution of steel wires in the concrete mixture. Although the steel wires were slowly added to the mixture, aggregation was observed to initiate in the mixture with 3%. Workability was significantly decreased after a 2% fiber content ratio. Slump tests were also performed. The results of the slump test were indicated in Figure 2. It is seen that slump values with steel wires are lower than that of the reference specimen. Moreover, the slump value decreases as the fiber ratio increases.
After mixing, the mixture was poured into molds and vibrated for 30 s. The samples were kept a room temperature for 24 h after casting. Then the samples were tested after 28 days of curing.
2.3 Test procedure
Three different tests were conducted to evaluate the influence of recycled steel wire types and fiber ratio. These tests including compressive, splitting tensile, and bending strength tests are depicted in Figure 3. For each mixture, three repetitions were tested and an average of them was reported. Compression tests were conducted based on ASTM C39/C39M (C39&C39M A, 2003). ASTM C496-96 was utilized for splitting tensile test while ASTM C78/C78M-22 was used for flexural tests.
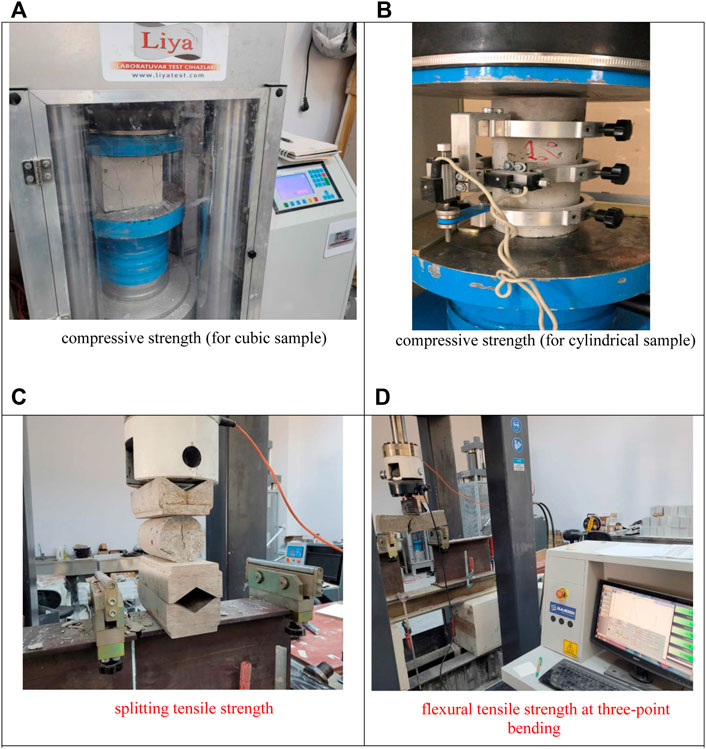
FIGURE 3. Mechanical test setups (A) compressive strength (for cubic sample) (B) compressive strength (for cylindrical sample) (C) splitting tensile strength (D) flexural tensile strength at three-point bending.
Two different compressive strength tests were carried out using cube samples with 150 × 150 × 150 mm and cylindrical samples with a diameter of 100 mm and a height of 200 mm. The speed of loading was 6 kN/s and the tests were conducted up to failure. Using cylindrical samples, compressive strength and compressive strength curves were obtained. On the other hand, cube samples were used to obtain only compressive strength. The splitting tensile tests were also utilized using cylindrical samples with a diameter of 100 mm and a height of 200 mm. The speed of loading was 5 kN/min and the tests were conducted up to a drop 50% in strength. Three-point bending test was performed to compare the flexural strength of the samples with different mixtures. The samples had dimensions of 100 × 100 × 400 mm, and a span length was 300 mm. The speed of loading was 0.5 mm/sec. The flexural behavior of the mixtures was determined by the load-displacement curves.
3 Experimental results and discussions
3.1 Compressive strength of cubic samples
Figure 4 shows the compressive test results of 150 × 150 × 150 mm cubic samples. The graph in Figure 4B shows, from left to right, the compressive strength results of samples of reference and recycled steel wires additive concrete. It is pleasing that the samples with waste material have superior compressive strength compared to the reference samples. In Figure 4B, it is evident that the rising graphite increases the compressive strength as the ratio of waste tire wire in the concrete increases.
To predict the compressive strength of the concrete with recycled steel wires, an equation was derived considering fiber volume ratio and plain concrete strength. Compressive strength with recycled steel wires can be computed using the following proposed equation:
where
The compression strength values, and their differences obtained as a result of the cubic compression test are presented in Table 2. The compression strength values results obtained by the experimental and proposed equations are very close to each other. A maximum of 2% difference was detected between the experimental and proposed equation. While the compression strengths value of the reference samples was 29.5 MPa, it increased to 34.6 MPa with a 1% tire additive. In the 2% contribution, a compressive strength raised to 38.6 MPa. Finally, compression strength with 3% additive strength was 43.2 MPa. Gao et al. (2017) found that the compressive strength increased by 1.03% in the test of 1% wire reinforced cubic concrete. The compressive strength of the tire doped specimen confirms the results of (Gao et al., 2017). Yazıcı et al. (2007) in their study added three different fiber volumes of 0.5%, 1.0%, and 1.5. They found that the strength of concrete increased between 4% and 19%. In this study, it is seen that the compressive strength of concrete increases between 17% and 42% with the tire additive. It should be noted that with the addition of waste fibers, the compressive strength of fiber-reinforced concrete increases, but after a certain value of waste fiber content, there is a decrease in workability (Yazıcı et al., 2007).
3.2 Compressive strength of cylindrical samples
Figure 5 depicts the compressive strength result of cylindrical samples. The results have not only increased the maximum strength of the concrete but also increased the toughness capacity thanks to recycled steel wire additive. As the steel wire additive ratio increased, the strength increased proportionally. Table 3 shows the compressive strength results of the cylindrical concrete samples. While the maximum strength was 29 MPa with 1% steel wire additive, the strength was 32 MPa with 2% steel wire additive. This strength increased to 37 MPa if 3% steel wire was utilized. The test results reveal that concrete with 29.5 MPa strength can be increased to 37 MPa which is a 25% increase in the capacity. Neves and Fernandes de Almeida, (2005) stated in their study that up to 1.5% of fiber additives increase the compressive strength of concrete, but decrease Young’s modulus slightly. However, in this study, it is understood from the graph that the rate of increase in waste fiber slightly increased in Young’s modulus. The obtained results support the statement that (Shah Surendra and Rangan, 1970) stated in their study that fibers significantly improved the ductility of concrete, as evidenced by increased final compressive concrete strains and toughness. Lee, (2017), in his study with 0.25%, 0.375%, and 0.50% fiber volume ratios, determined that the energy absorption capacity increased as the fibre volume ratio in the concrete increased. It is also understood from the curves in Figure 5 that the energy absorption capacity continues at higher rates depending on the increasing fibre volume ratio.
3.3 Splitting tensile strength
A splitting tensile strength test is performed to investigate the effects of steel wires on the tensile strength of concrete. Figure 6 shows the splitting tensile strength result of samples with reference and volume fracture (Vf) of 1%–3% steel wire. Table 4 demonstrates the splitting tensile strength of the samples. Splitting tensile strength increased from 2.83 to 3.24 MPa with 1% Vf steel wire. This strength increased to 3.87 when Vf of 3% steel wire was utilized.
To predict the splitting tensile strength of concrete with recycled steel wire, an equation was derived based on the fiber ratio and compressive strength of plain concrete. Splitting tensile strength with recycled steel wires can be computed using the following proposed equation:
where
3.4 Flexural performance
The flexural performance of concrete according to volume ratio is shown in Figure 7. While 1% tire provided the flexural performance of around 3.8 kN. It was increased to 5 kN when 2% steel wire was utilized. The addition of 3% fiber provided flexural strength of 6.3 kN. With the 2% tire additive, more than 100% flexural strength was obtained. Since longer waste fiber was utilized in this study, the result was better than the results of (Yoo et al., 2017).
One of the most important results obtained from this study is the toughness of concrete. Figure 8 shows the toughness values. In general, ductile materials have high toughness and brittle materials have low toughness. According to the results obtained, it is seen that the waste fiber additive provides very high toughness. Soulioti et al. (2011) in their experimental study with steel fibers with different geometries, revealed that hook-tipped fibers provide better toughness in concrete than fibers with wavy geometry. Yoo et al. (2017) have argued on the contrary that spun fibers provide the highest flexural strength, but exhibit similar strength and weaker toughness than straight fibers at a Vf equal to or greater than 1.5%. They stated that at a Vf equal to or higher than 1.0%, lower flexural strength and toughness were observed in samples with hooked fibers compared to straight ones.
3.5 Damage analysis
Beams are mechanically accepted structural elements that transfer loads of the usage area to the vertical carriers. Therefore, it is an important issue to perform damage analysis according to the additives added. It has been observed that microcracks occur in the tensile region of the beam when the fracture strength is reached, especially in fiber-reinforced samples. The addition of steel waste tires stabilized the crack development until the maximum load was reached. When the initial tensile failure was developed in the reference samples, there was a sudden decrease in load capacity, whereas a slower decrease occurred in the fiber-doped samples. As seen in Figure 9, the crack formation has moved towards the compression zone. Therefore, the stability of the beam section under the natural axis was effectively maintained by the fiber bridging region being resistant to fiber rupture. Finally, the fibers that caused the beam sample to fail were completely withdrawn. In all beam specimens, the fracture was observed at the moment constant region of the beam. The fibers drawn on the cracked surface and the tensile fractures on the concrete matrix are visible in Figure 9.
Microstructural analysis (SEM) was performed from the sample pieces taken from recycled steel wire added samples. The SEM images in Figure 10 were obtained by magnifying 500 times. The image on the right is generally projected as an enlargement of the pattern on the left. Important findings are marked on the figures. Images showing the key findings are shown in Figure 10. Figures 10A–F show detailed the interaction between waste steel fibers and concrete. Figure 10A shows hydrated cement, aggregate, and fibers. Figure 10B shows the concrete distribution of the ettringite and the spacing between them. Ettringite develops due to a high content of the mineral sulphate calcium sulfoaluminate. Ettringite is formed in mortar and concrete by the effect of sulphate. Ettringite generally increases the compressive strength of concrete thanks to its Figure 10B also shows pores associated with ettringite. Figure 10C shows the distribution of aggregates in concrete, surface quality, and voids. Cement hydration may be poor at points where voids are high. Figure 10D shows the fine aggregate and cement combination with good hydration. The interface between the steel fiber and the concrete is shown in Figure 10E. There may be a gap on one side of the fiber. Figure 10F shows the steel fiber interface up close. It is seen that it exhibits good adhesion with cementitious concrete.
4 Comparison with the existing SFRC studies
When considering existing research findings, tire-recycled fiber is feasible to enhance some properties of the concrete. By conducting experimental studies, compressive strength, flexural strength, and split tensile strength were obtained for tire-reinforced concrete with different amounts of steel fiber. In the studies, amount of fiber, type (industrial or recycled), length, and diameter of the fiber were the main variables. In this part of the study, compressive strength and split tensile strength values for plain concrete (normal concrete without fibers) and reinforced concrete with steel fibers (either industrial, recycled, or hybrid) were collected from the literature. Experimental results obtained from these existing studies conducted by many researchers (Leone et al., 2016; Aghaee et al., 2015; Gul et al., 2021; Mastali et al., 2018; Groli et al., 2014; Köroğlu, 2018; Saatçi and Batarlar, 2017; Samarakoon et al., 2019; Zhang and Gao, 2020; Centonze et al., 2012; Dehghanpour and Yılmaz, 2018; Al-Tikrite and Hadi, 2017; Vistos et al., 2018; Nataraja et al., 1999; Dorr et al., 2019; Sengul, 2018; Ł and Suchorzewski, 2018; Awal et al., 2013; Chern and Young, 1989; Najim et al., 2018; Shi et al., 2020; Rossli and Ibrahim, 2012) were presented in Figures 11, 12. Industrial fiber reinforced concrete (IFRC), recycled fiber reinforced concrete (RFRC) and hybrid fiber reinforced concrete (HFRC) cases were shown in these figures. Relative compressive strength of steel fiber reinforced concrete to the reference or plain concrete without fibers were plotted against fiber content (%) used in the mixture as shown in Figure 11. The compressive strength value of the fiber reinforced concrete is generally higher than that of plain concrete. This indicates that steel fibers play a positive role in increasing strength. Since steel fibers have high tensile toughness, they can limit degradation and crack width under different loading conditions. In other words, steel fibers provide a confinement effect on the mixture by arresting cracks when the number of fibers increases (Shah et al., 2022). This effect delays the rupture in the test specimens. However, in some experimental studies, as shown in Figure 11, it was observed that the compression strength of the reinforced concrete with steel fiber was smaller than plain concrete when fiber content increases since a high amount of fiber lead to a reduction in the workability in the concrete mixture. On the other hand, in some studies, although there was an increase in the compression strength up to a certain threshold value of the fiber dosage, further increasing the steel fiber addition led to a decrease in the compression value. The maximum increase in strength was observed study conducted by Mastali et al. (2018). The use of 1.5% industrial steel fiber resulted in a 56% increase in compression strength of the plain concrete specimens. On the other hand, the maximum decrease in compression strength occurred in the experimental study performed by Vistos et al. (2018). The utilization of 0.5% hybrid steel fiber led to a 14% reduction in the concrete strength. Fiber length also plays an important role in the compressive strength and splitting tensile strength of the fiber-reinforced concrete. Long fibers provide higher splitting tensile strength, while short fibers provide high compressive strength (Gul et al., 2021).
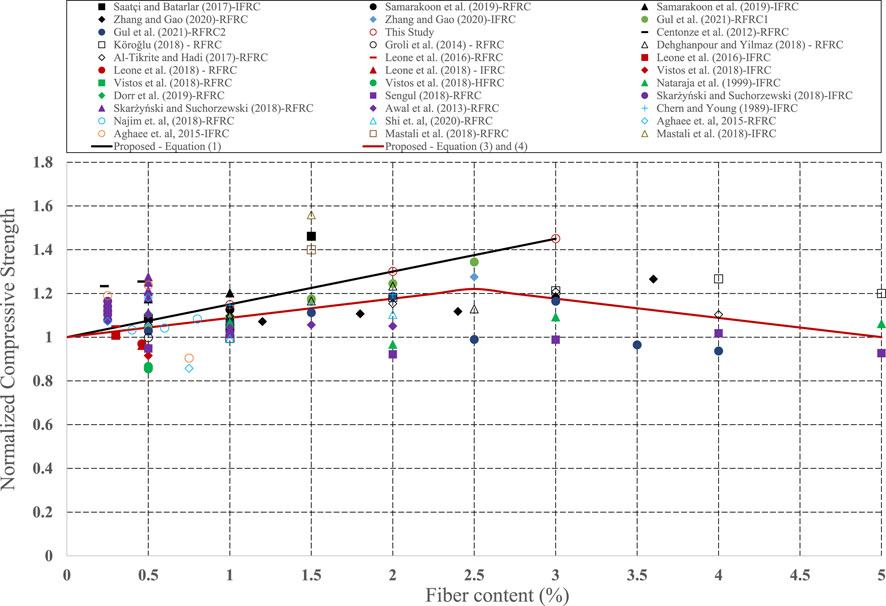
FIGURE 11. Variation of the normalized compressive strength of the steel fiber reinforced concrete with the fiber content.
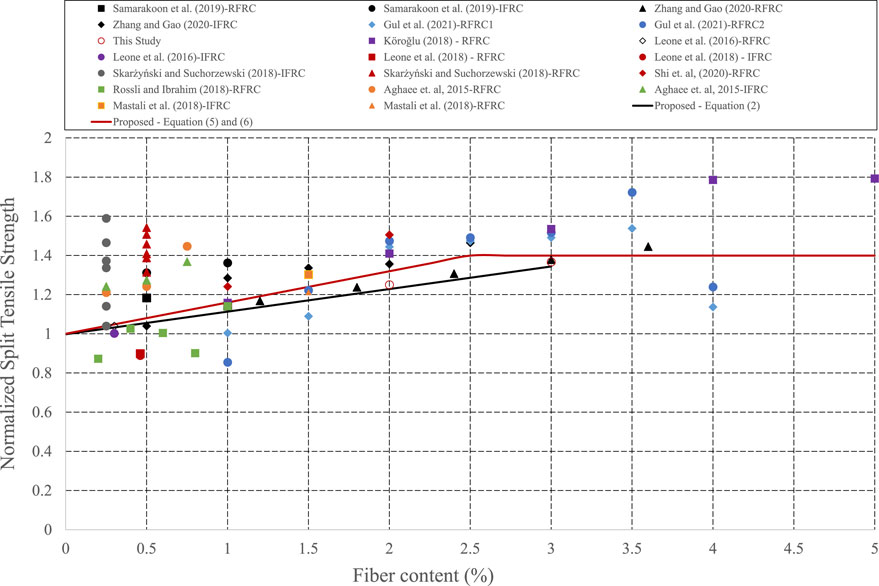
FIGURE 12. Variation of the splitting tensile strength of the steel fiber reinforced concrete with the fiber content.
In the same manner, the splitting tensile strength of fiber reinforced concrete was normalized by that of plain concrete as shown in Figure 12. There is a similar observation in the findings of the split tensile strength of the test specimens. The maximum increase in the split tensile strength was observed in the study carried out by (Köroğlu, 2018). The employing of 5.0% industrial steel fiber caused a 79% increase in the split tensile strength of functionally graded self-compacting concrete. On the other hand, the largest decrease in tensile strength was observed in the study (Gul et al., 2021). When 10.16 cm lengths of recycled steel fiber with 1% volume fracture were added, a 15% reduction occurred in the splitting tensile strength of concrete test specimens. It should be noted finding obtained from the current study showed that values for both compression strength and splitting tensile strength increase with increasing fiber content and the proposed empirical equations are shown in Figures 11, 12.
As depicted in Figures 11, 12, most normalized strength values are above 1.0; this means that steel fibers have a generally positive effect in increasing both compressive and splitting tensile strength. Considering all data collected from the literature, the average, standard deviation, maximum and minimum values of the normalized strength ratios were shown in Table 4.
As shown in the experimental part of the study, there is a linear increase in the compression strength and splitting tensile strength. Thus, by applying curve-fitting to the data obtained from the experimental study conducted in this study empirical equations were derived for practical purposes. Using these proposed equations, both compression strength and splitting tensile strength of the steel fiber reinforced concretes may be estimated considering different steel fiber amount. According to the test results, 2% is proposed as the optimum fiber amount to prevent workability issues in the concrete mixture. According to the studies conducted by Köroglu and Ashour, (2019), (Yazıcı et al., 2007; Revuelta et al., 2021; Hu et al., 2018), it was stated that volume fraction of steel fiber significantly affects the workability of concrete. Test results of Köroglu and Ashour, (2019) showed that excess fiber added in concrete reduced the workability of concrete and reduced flow diameter in flow table test. Furthermore, the optimum fiber content values for concrete mixes are recommended between 0.5% and 2.5% (Pierre et al., 1999) and (Song and Hwang, 2004). Adding a low amount of fibers prevents fiber balling and produces the concrete with homogeneous material consistency and good workability (Song and Hwang, 2004). Thus, considering our experimental test results, a 2% volume of fiber is recommended as the optimum fiber dosage for practical applications. Recommendation of 2% was also utilized in many studies (Özkılıç et al., 2021; Aksoylu et al., 2022; Karalar et al., 2022b; Çelik et al., 2022). As already mentioned, there is a decrease in the strength values after a certain fiber content. Thus, new generalized strength equations taking into account the reduction in the strengths have been developed as follows:
5 Conclusion and summary
This paper investigates the effect of steel fiber generated from expired vehicle tires on concrete performance. For this purpose, an experimental study was conducted by considering three different steel fiber contents of 1%, 2% and 3%. The engineering properties of concrete produced with waste tire in terms of the fresh and hardened cases were examined. Furthermore, empirical equations are also derived as a function of the fiber ratio to easily calculate the compressive strength and splitting tensile strength. The following can be drawn from this study:
1) The slump test results show that the slump value decreases as the fiber ratio increases. Workability of concrete with tire-recycled steel fiber is also decreased when the amount of fibers increases.
2) The compressive test results showed that compression strength of concrete in cubic samples increased with the addition of the wire steel fibers. Compared to the reference sample, the compressive strength value increased by 17% with 1% wire steel fibers additive, 30% with 2% wire steel fibers and 46% with 3% wire steel fibers. In cylindrical samples, the compressive strength of concrete also increased according to the amount of waste wire. Compared to the reference sample, the compressive strength of the concrete increased by 18% with 1% wire steel fibers additive, 45% with 2% wire steel fibers and 58% with 3% wire steel fibers.
3) The tensile test results on cylindrical samples showed there is a proportional increase with the steel fiber addition. The addition of 1%, 2%, and 3% wire steel fibers increased splitting tensile strength of concrete by 15%, 25%, and 36%, respectively, compared to plain concrete.
4) The flexural strength values show that the flexural strength increases proportionally with the addition of high wire fiber. It was observed that the addition of wire fiber gives positive results in flexural strength compared to the reference sample. The addition of 1%, 2%, and 3% wire steel fibers increased flexural strength of concrete by 77%, 272%, and 795%, respectively,
5) The toughness test results showed that there is a proportional increase between the ductility and the toughness. Respectively, 4, 16, and 26 times more toughness occurred compared to the reference sample.
6) The developed expressions for the compressive strength and splitting tensile strength can successfully estimate the capacity; therefore, these equations have the potential to be implemented into design guidelines of the concretes with fibers recovered from used tires.
7) Based on the fresh and hardened properties of concrete, 2% is recommended as the optimum of amount for tire-recycled steel fiber. It should be also noted that this ratio can be changed using very low or high water cement ratio or using superplasticizer.
Data availability statement
The raw data supporting the conclusion of this article will be made available by the authors, without undue reservation.
Author contributions
ÖZ: investigation, writing—original draft preparation, writing—review and editing YOÖ: Conceptualization, methodology, formal analysis, investigation, resources, data curation, writing—original draft preparation AD: writing—original draft preparation, writing—review and editing AÇ: formal analysis, writing—original draft preparation, writing—review and editing MA: writing—original draft preparation, writing—review and editing MMSS: resources, writing—original draft preparation, writing—review and editing, funding.
Funding
The research is partially funded by the Ministry of Science and Higher Education of the Russian Federation under the strategic academic leadership program “Priority 2030” (Agreement 075-15-2021-1333 dated 30.09.2021).
Conflict of interest
The authors declare that the research was conducted in the absence of any commercial or financial relationships that could be construed as a potential conflict of interest.
Publisher’s note
All claims expressed in this article are solely those of the authors and do not necessarily represent those of their affiliated organizations, or those of the publisher, the editors and the reviewers. Any product that may be evaluated in this article, or claim that may be made by its manufacturer, is not guaranteed or endorsed by the publisher.
References
Abdullah, G. M. S., Alshaikh, I. M. H., Zeyad A, M., Magbool, H. M., and Bakar, B. H. A. (2022). The effect of openings on the performance of self-compacting concrete with volcanic pumice powder and different steel fibers. Case Stud. Constr. Mater. 17, e01148. doi:10.1016/j.cscm.2022.e01148
Adil, W., Ur Rahman, F., S Abdullah, M. G., Tayeh, B. A., and Zeyad, A. M. (2022). Effective utilization of textile industry waste-derived and heat-treated pumice powder in cement mortar. Constr. Build. Mater. 351, 128966. doi:10.1016/j.conbuildmat.2022.128966
Aghaee, K., Yazdi, M. A., and Tsavdaridis, K. D. (2015). Investigation into the mechanical properties of structural lightweight concrete reinforced with waste steel wires. Mag. Concr. Res. 67, 197–205. doi:10.1680/macr.14.00232
Aghaee, K., and Yazdi, M. A. (2014). Waste steel wires modified structural lightweight concrete. Mat. Res. 17, 958–966. doi:10.1590/1516-1439.257413
Ahmed, H. U., Faraj, R. H., Hilal, N., Mohammed, A. A., and Sherwani, A. F. H. (2021). Use of recycled fibers in concrete composites: A systematic comprehensive review. Compos. Part B Eng. 215, 108769. doi:10.1016/j.compositesb.2021.108769
Aiello, M. A., Leuzzi, F., Centonze, G., and Maffezzoli, A. (2009). Use of steel fibres recovered from waste tyres as reinforcement in concrete: Pull-out behaviour, compressive and flexural strength. Waste Manag. 29, 1960–1970. doi:10.1016/j.wasman.2008.12.002
Aksoylu, C., Özkılıç, Y. O., Hadzima-Nyarko, M., Işık, E., and Arslan, M. H. J. S. (2022). Investigation on improvement in shear performance of reinforced-concrete beams produced with recycled steel wires from waste tires. Sustainability 14, 13360. doi:10.3390/su142013360
Al-Tikrite, A., and Hadi, M. N. S. (2017). Mechanical properties of reactive powder concrete containing industrial and waste steel fibres at different ratios under compression. Constr. Build. Mater. 154, 1024–1034. doi:10.1016/j.conbuildmat.2017.08.024
Alabi, S. A. (2020). Predictive models for evaluation of compressive and split tensile strengths of recycled aggregate concrete containing lathe waste steel fiber. Jordan J. Civ. Eng., 14.
Alani, A. A., Lesovik, R., Lesovik, V., Fediuk, R., Klyuev, S., Amran, M., et al. (2022). Demolition waste potential for completely cement-free binders. Materials 15, 6018. doi:10.3390/ma15176018
Alwesabi, E. A. H., Bakar, B. H. A., Alshaikh, I. M. H., Zeyad, A. M., Altheeb, A., and Alghamdi, H. (2021). Experimental investigation on fracture characteristics of plain and rubberized concrete containing hybrid steel-polypropylene fiber. Structures 33, 4421–4432. doi:10.1016/j.istruc.2021.07.011
Anil, N. (2018). Mechanical properties of steel fiber reinforced self-compacting concrete. Int. J. Eng. Technol. IJET 4, 33–40. doi:10.19072/ijet.340259
Awal, A. S. M. A. A. A. S. M. A., Yee, L. L., and Hossain, M. Z. (2013). Fresh and hardened properties of concrete containing steel fibre from recycled tire. Malays. J. Civ. Eng. 25.
Barišić, I., Zvonarić, M., Grubeša, I. N., and Šurdonja, S. (2021). Recycling waste rubber tyres in road construction. Archives Civ. Eng. 67, 499–512.
Behbahani, H. P., Nematollahi, B., and Farasatpour, M. (2011). Steel fiber reinforced concrete: A review.
Caggiano, A., Folino, P., Lima, C., Martinelli, E., and Pepe, M. (2017). On the mechanical response of hybrid fiber reinforced concrete with recycled and industrial steel fibers. Constr. Build. Mater. 147, 286–295. doi:10.1016/j.conbuildmat.2017.04.160
Carrillo, J., Lizarazo-Marriaga, J., and Lamus, F. (2020). Properties of steel fiber reinforced concrete using either industrial or recycled fibers from waste tires. Fibers Polym. 21, 2055–2067. doi:10.1007/s12221-020-1076-1
Çelik, A. İ., Özkılıç, Y. O., Zeybek, Ö., Özdöner, N., and Tayeh, B. A. (2022). Performance assessment of fiber-reinforced concrete produced with waste lathe fibers. Sustainability 14, 11817. doi:10.3390/su141911817
Centonze, G., Leone, M., and Aiello, M. A. (2012). Steel fibers from waste tires as reinforcement in concrete: A mechanical characterization. Constr. Build. Mater. 36, 46–57. doi:10.1016/j.conbuildmat.2012.04.088
Chern, J-C., and Young, C-H. (1989). Compressive creep and shrinkage of steel fibre reinforced concrete. Int. J. Cem. Compos. Lightweight Concr. 11, 205–214. doi:10.1016/0262-5075(89)90100-0
de Azevedo, A. R. G., Marvila, M. T., de Oliveira, M. A. B., Umbuzeiro, C. E. M., Huaman, N. R. C., and Monteiro, S. N. (2022). Perspectives for the application of bauxite wastes in the development of alternative building materials. J. Mater. Res. Technol. 20, 3114–3125. doi:10.1016/j.jmrt.2022.08.092
Dehghanpour, H., and Yılmaz, K. (2018). Mechanical and impact behavior on recycled steel fiber reinforced cementitious mortars. Sci. Her. Voronezh State Univ. Archit. Civ. Eng. 39.
Domski, J., Katzer, J., Zakrzewski, M., and Ponikiewski, T. (2017). Comparison of the mechanical characteristics of engineered and waste steel fiber used as reinforcement for concrete. J. Clean. Prod. 158, 18–28. doi:10.1016/j.jclepro.2017.04.165
Dorr, B. J., Kanali, C. L., and Onchiri, R. O. (2019). Shear performance of recycled tyres steel fibres reinforced lightweight concrete beam using palm kernel shear as partial replacement of coarse aggregate. Int. J. Eng. Res. Technol. 12, 1818–1823.
El-Sayed, T. A. (2019). Flexural behavior of RC beams containing recycled industrial wastes as steel fibers. Constr. Build. Mater. 212, 27–38. doi:10.1016/j.conbuildmat.2019.03.311
Failla, C., Toniolo, G., and Ferrara, L. (2002). Structural design of prestressed precast roof elements made with steel fibre reinforced concrete. BIBM 2002 International Conference, Istanbul.
Frančić Smrkić, M., Damjanović, D., and Baričević, A. (2017). Application of recycled steel fibres in concrete elements subjected to fatigue loading. Građevinar 69, 893–905.
Gao, D., Zhang, L., and Nokken, M. (2017). Compressive behavior of steel fiber reinforced recycled coarse aggregate concrete designed with equivalent cubic compressive strength. Constr. Build. Mater. 141, 235–244. doi:10.1016/j.conbuildmat.2017.02.136
Ghorpade, V. G., and Rao, H. S. (2010). Strength and permeability characteristics of Fibre reinforced recycled aggregate concrete with different fibres. Nat. Environ. Pollut. Technol. 9, 179–188.
Groli, G., Pérez Caldentey, A., and Soto, A. G. (2014). Cracking performance of SCC reinforced with recycled fibres–an experimental study. Struct. Concr. 15, 136–153. doi:10.1002/suco.201300008
Gul, A., Alam, B., Iqbal, M. J., Ahmed, W., Shahzada, K., Javed, M. H., et al. (2021). Impact of length and percent dosage of recycled steel fibers on the mechanical properties of concrete. Civ. Eng. J. 7, 1650–1666. doi:10.28991/cej-2021-03091750
Hu, H., Papastergiou, P., Angelakopoulos, H., Guadagnini, M., and Pilakoutas, K. (2018). Mechanical properties of SFRC using blended manufactured and recycled tyre steel fibres. Constr. Build. Mater. 163, 376–389. doi:10.1016/j.conbuildmat.2017.12.116
Isa, M. N., Pilakoutas, K., Guadagnini, M., and Angelakopoulos, H. (2020). Mechanical performance of affordable and eco-efficient ultra-high performance concrete (UHPC) containing recycled tyre steel fibres. Constr. Build. Mater. 255, 119272. doi:10.1016/j.conbuildmat.2020.119272
Jabir, H. A., Abid, S. R., Murali, G., Ali, S. H., Klyuev, S., Fediuk, R., et al. (2020). Experimental tests and reliability analysis of the cracking impact resistance of UHPFRC. Fibers 8, 74. doi:10.3390/fib8120074
Karalar, M., Bilir, T., Çavuşlu, M., Özkılıç, Y. O., and Sabri, M. M. S. Use of recycled coal bottom ash in reinforced concrete beams as replacement for aggregate. 2022, 1064604.
Karalar, M., Özkılıç, Y. O., Deifalla, A. F., Aksoylu, C., Arslan, M. H., Ahmad, M., et al. (2022). Improvement in bending performance of reinforced concrete beams produced with waste lathe scraps. Sustainability 14, 12660. doi:10.3390/su141912660
Köroglu, M. A., and Ashour, A. (2019). Mechanical properties of self-compacting concrete with recycled bead wires. rdlc 18, 501–512. doi:10.7764/rdlc.18.3.501
Köroğlu, M. A. (2018). Behavior of composite self-compacting concrete (SCC) reinforced with steel wires from waste tires. Revista de Construcción J. Constr. 17, 484–498.
Ł, Skarżyński, and Suchorzewski, J. (2018). Mechanical and fracture properties of concrete reinforced with recycled and industrial steel fibers using Digital Image Correlation technique and X-ray micro computed tomography. Constr. Build. Mater. 183, 283–299. doi:10.1016/j.conbuildmat.2018.06.182
Lee, J-H. (2017). Influence of concrete strength combined with fiber content in the residual flexural strengths of fiber reinforced concrete. Compos. Struct. 168, 216–225. doi:10.1016/j.compstruct.2017.01.052
Leone, M., Centonze, G., Colonna, D., Micelli, F., and Aiello, M. A. (2016). Experimental study on bond behavior in fiber-reinforced concrete with low content of recycled steel fiber. J. Mat. Civ. Eng. 28, 04016068. doi:10.1061/(asce)mt.1943-5533.0001534
Liew, K. M., and Akbar, A. (2020). The recent progress of recycled steel fiber reinforced concrete. Constr. Build. Mater. 232, 117232. doi:10.1016/j.conbuildmat.2019.117232
Magbool, H. M., and Zeyad, A. M. (2021). The effect of various steel fibers and volcanic pumice powder on fracture characteristics of Self-Compacting concrete. Constr. Build. Mater. 312, 125444. doi:10.1016/j.conbuildmat.2021.125444
Martínez-García, R., Jagadesh, P., Zaid, O., Șerbănoiu, A. A., Fraile-Fernández, F. J., de Prado-Gil, J., et al. (2022). The present state of the use of waste wood ash as an eco-efficient construction material: A review. Materials 15, 5349. doi:10.3390/ma15155349
Mastali, M., Dalvand, A., Sattarifard, A. R., and Illikainen, M. (2018). Development of eco-efficient and cost-effective reinforced self-consolidation concretes with hybrid industrial/recycled steel fibers. Constr. Build. Mater. 166, 214–226. doi:10.1016/j.conbuildmat.2018.01.147
Meda, A., Minelli, F., Plizzari, G. A., and Riva, P. (2005). Shear behaviour of steel fibre reinforced concrete beams. Mat. Struct. 38, 343–351. doi:10.1617/14112
Ming, Y., Chen, P., Li, L., Gan, G., and Pan, G. (2021). A comprehensive review on the utilization of recycled waste fibers in cement-based composites. Materials 14, 3643. doi:10.3390/ma14133643
Najim, K. B., Saeb, A., and Al-Azzawi, Z. (2018). Structural behaviour and fracture energy of recycled steel fibre self-compacting reinforced concrete beams. J. Build. Eng. 17, 174–182. doi:10.1016/j.jobe.2018.02.014
Nataraja, M. C., Dhang, N., and Gupta, A. P. (1999). Stress–strain curves for steel-fiber reinforced concrete under compression. Cem. Concr. Compos. 21, 383–390. doi:10.1016/s0958-9465(99)00021-9
Neocleous, K., Tlemat, H., and Pilakoutas, K. (2006). Design issues for concrete reinforced with steel fibers, including fibers recovered from used tires. J. Mat. Civ. Eng. 18, 677–685. doi:10.1061/(asce)0899-1561(2006)18:5(677)
Neves, R. D., and Fernandes de Almeida, J. C. O. (2005). Compressive behaviour of steel fibre reinforced concrete. Struct. Concr. 6, 1–8. doi:10.1680/stco.2005.6.1.1
Özkılıç, Y. O., Aksoylu, C., and Arslan, M. H. (2021). Experimental and numerical investigations of steel fiber reinforced concrete dapped-end purlins. J. Build. Eng. 36, 102119. doi:10.1016/j.jobe.2020.102119
Pierre, P., Pleau, R., and Pigeon, M. (1999). Mechanical properties of steel microfiber reinforced cement pastes and mortars. J. Mat. Civ. Eng. 11, 317–324. doi:10.1061/(asce)0899-1561(1999)11:4(317)
Pilakoutas, K., Neocleous, K., and Tlemat, H. (2004). “Reuse of tyre steel fibres as concrete reinforcement,” in Proceedings of the Institution of Civil Engineers-Engineering Sustainability. (Thomas Telford Ltd), Vol. 157 (3), 131–138.
Prabu, M., Vignesh, K., Saii Prasanna, N., Praveen, C., and Mohammed Nafeez, A. (2020). Experimental study on concrete in partial replacement of fine aggregate with lathe waste. Int. J. Sci. Eng. Res. 11, 68–72.
Qaidi, S., Najm, H. M., Abed, S. M., Özkılıç, Y. O., Al Dughaishi, H., Alosta, M., et al. (2022). Concrete containing waste glass as an environmentally friendly aggregate: A review on fresh and mechanical characteristics. Materials 15, 6222. doi:10.3390/ma15186222
Qin, X., and Kaewunruen, S. (2022). Environment-friendly recycled steel fibre reinforced concrete. Constr. Build. Mater. 327, 126967. doi:10.1016/j.conbuildmat.2022.126967
Rashid, K., and Balouch, N. (2017). Influence of steel fibers extracted from waste tires on shear behavior of reinforced concrete beams. Struct. Concr. 18, 589–596. doi:10.1002/suco.201600194
Revuelta, D., Carballosa, P., García Calvo, J. L., and Pedrosa, F. (2021). Residual strength and drying behavior of concrete reinforced with recycled steel fiber from tires. Materials 14, 6111. doi:10.3390/ma14206111
Rossli, S., and Ibrahim, I. (2012). Mechanical properties of recycled steel tire fibres in concrete. Malaysia: Fac Civil Eng, University of Technology.
Saatçi, S., and Batarlar, B. (2017). Çelik fiber katkılı etriyesiz betonarme kirişlerin davranışı. Gazi Univ. Muhendislik-Mimarlik Fak. Derg. 32, 1143–1154. doi:10.17341/gazimmfd.369512
Samarakoon, S. M. S. M. K., Ruben, P., Wie Pedersen, J., and Evangelista, L. (2019). Mechanical performance of concrete made of steel fibers from tire waste. Case Stud. Constr. Mater. 11, e00259. doi:10.1016/j.cscm.2019.e00259
Seetharam, P. G., Bhuvaneswari, C., Vidhya, S., and Vishnu Priya, M. (2017). Studies on properties of concrete replacing lathe scrap. Int. J. Eng. Res. Technol. 6, 382–386.
Senesavath, S., Salem, A., Kashkash, S., Zehra, B., and Orban, Z. (2022). The effect of recycled tyre steel fibers on the properties of concrete. Pollack 17, 43–49. doi:10.1556/606.2021.00388
Sengul, O. (2016). Mechanical behavior of concretes containing waste steel fibers recovered from scrap tires. Constr. Build. Mater. 122, 649–658. doi:10.1016/j.conbuildmat.2016.06.113
Sengul, O. (2018). Mechanical properties of slurry infiltrated fiber concrete produced with waste steel fibers. Constr. Build. Mater. 186, 1082–1091. doi:10.1016/j.conbuildmat.2018.08.042
Shah, S. H. A., Ali, B., Ahmed, G. H., Tirmazi, S. M. T., El Ouni, M. H., and Hussain, I. (2022). Effect of recycled steel fibers on the mechanical strength and impact toughness of precast paving blocks. Case Stud. Constr. Mater. 16, e01025. doi:10.1016/j.cscm.2022.e01025
Shah Surendra, P., and Rangan, B. V. (1970). Effects of reinforcements on ductility of concrete. J. Struct. Div. 96, 1167–1184. doi:10.1061/jsdeag.0002601
Shi, X., Brescia-Norambuena, L., Grasley, Z., and Hogancamp, J. (2020). Fracture properties and restrained shrinkage cracking resistance of cement mortar reinforced by recycled steel fiber from scrap tires. Transp. Res. Rec. 2674, 581–590. doi:10.1177/0361198120924407
Shukla, A. K. (2013). Application of CNC waste with recycled aggregate in concrete mix. Int. J. Eng. Res. Appl. 3, 1026–1031.
Simalti, A., and Singh, A. P. (2020). Comparative study on direct shear behavior of manufactured and recycled shredded tyre steel fiber reinforced self-consolidating concrete. J. Build. Eng. 29, 101169. doi:10.1016/j.jobe.2020.101169
Singh, S. P., and Kaushik, S. K. (2001). Flexural fatigue analysis of steel fiber-reinforced concrete. Mater. J. 98, 306–312.
Song, P. S., and Hwang, S. (2004). Mechanical properties of high-strength steel fiber-reinforced concrete. Constr. Build. Mater. 18, 669–673. doi:10.1016/j.conbuildmat.2004.04.027
Soulioti, D. V., Barkoula, N. M., Paipetis, A., and Matikas, T. E. (2011). Effects of fibre geometry and volume fraction on the flexural behaviour of steel-fibre reinforced concrete. Strain 47, e535–e541. doi:10.1111/j.1475-1305.2009.00652.x
Vieira, D. R., Calmon, J. L., and Coelho, F. Z. (2016). Life cycle assessment (lca) applied to the manufacturing of common and ecological concrete: A review. Constr. Build. Mater. 124, 656–666. doi:10.1016/j.conbuildmat.2016.07.125
Vistos, L., Galladini, D., Xargay, H., Caggiano, A., Folino, P., and Martinelli, E. (2018). “Hybrid industrial/recycled SFRC: Experimental analysis and design,” in Proceedings of Italian Concrete Days 2016. ICD 2016. Lecture Notes in Civil Engineering. Editors M. di Prisco, and M. Menegotto (Springer), Vol. 10.
Wu, Z., Shi, C., He, W., and Wu, L. (2016). Effects of steel fiber content and shape on mechanical properties of ultra high performance concrete. Constr. Build. Mater. 103, 8–14. doi:10.1016/j.conbuildmat.2015.11.028
Yazıcı, Ş., İnan, G., and Tabak, V. (2007). Effect of aspect ratio and volume fraction of steel fiber on the mechanical properties of SFRC. Constr. Build. Mater. 21, 1250–1253. doi:10.1016/j.conbuildmat.2006.05.025
Yoo, D-Y., Kim, S., Park, G-J., Park, J-J., and Kim, S-W. (2017). Effects of fiber shape, aspect ratio, and volume fraction on flexural behavior of ultra-high-performance fiber-reinforced cement composites. Compos. Struct. 174, 375–388. doi:10.1016/j.compstruct.2017.04.069
Yuan, F., Chen, M., and Pan, J. (2020). Flexural strengthening of reinforced concrete beams with high-strength steel wire and engineered cementitious composites. Constr. Build. Mater. 254, 119284. doi:10.1016/j.conbuildmat.2020.119284
Zamanzadeh, Z., Lourenço, L., and Barros, J. (2015). Recycled steel fibre reinforced concrete failing in bending and in shear. Constr. Build. Mater. 85, 195–207. doi:10.1016/j.conbuildmat.2015.03.070
Zeyad, A. M. (2020). Effect of fibers types on fresh properties and flexural toughness of self-compacting concrete. J. Mater. Res. Technol. 9, 4147–4158. doi:10.1016/j.jmrt.2020.02.042
Zeyad, A. M., Hakeem, I. Y., Amin, M., Tayeh, B. A., and Agwa, I. S. (2022). Effect of aggregate and fibre types on ultra-high-performance concrete designed for radiation shielding. J. Build. Eng. 58, 104960. doi:10.1016/j.jobe.2022.104960
Zeybek, Ö., Özkılıç, Y. O., Karalar, M., Çelik, A. İ., Qaidi, S., Ahmad, J., et al. (2022). Influence of replacing cement with waste glass on mechanical properties of concrete. Materials 15, 7513. doi:10.3390/ma15217513
Zhang, Y., and Gao, L. (2020). Influence of tire-recycled steel fibers on strength and flexural behavior of reinforced concrete. Adv. Mater. Sci. Eng. 2020, 1–7. doi:10.1155/2020/6363105
Keywords: waste tire, recycled steel fiber, fiber-reinforced concrete, compressive, splitting tensile, flexural, mechanical behavior
Citation: Zeybek Ö, Özkılıç YO, Çelik Aİ, Deifalla AF, Ahmad M and Sabri Sabri MM (2022) Performance evaluation of fiber-reinforced concrete produced with steel fibers extracted from waste tire. Front. Mater. 9:1057128. doi: 10.3389/fmats.2022.1057128
Received: 29 September 2022; Accepted: 02 November 2022;
Published: 17 November 2022.
Edited by:
Ramadhansyah Putra Jaya, Universiti Malaysia Pahang, MalaysiaReviewed by:
Piotr Smarzewski, Military University of Technology, PolandAbdullah Zeyad, Jazan University, Saudi Arabia
Copyright © 2022 Zeybek, Özkılıç, Çelik, Deifalla, Ahmad and Sabri Sabri. This is an open-access article distributed under the terms of the Creative Commons Attribution License (CC BY). The use, distribution or reproduction in other forums is permitted, provided the original author(s) and the copyright owner(s) are credited and that the original publication in this journal is cited, in accordance with accepted academic practice. No use, distribution or reproduction is permitted which does not comply with these terms.
*Correspondence: Yasin Onuralp Özkılıç, yozkilic@erbakan.edu.tr