- 1Department of Biochemistry, Government College University Faisalabad, Faisalabad, Pakistan
- 2Department of Applied Chemistry, Government College University Faisalabad, Faisalabad, Pakistan
- 3Department of Materials, School of Engineering and Technology, National Textile University, Faisalabad, Pakistan
- 4Department of Biochemistry, CMH Institute of Medical Sciences Multan, Multan, Pakistan
- 5Department of Chemistry, Division of Science and Technology, University of Education, Lahore, Pakistan
- 6Department of Chemistry, Riphah International University, Faisalabad, Pakistan
In recent years, electrospinning has emerged as a promising technique for the preparation of nanofibers with unique properties like flexibility, high porosity and high surface area. In the context of nanodelivery systems, polymer-based nanofibers have become promising carriers of drugs and bioactive compounds ensuring their sustained release and targeted delivery. In this study, neem extract-loaded nanofibers were developed as sustained delivery systems using the electrospinning method. The chitosan, alginate and polyethylene oxide were used as the polymeric matrix for loading of aqueous extract of neem leaves. The prepared nanofibers NF1, NF2 and NF3 carrying 2%, 4% and 6% extract respectively were characterized using SEM, FTIR, XRD and TGA. Further, the as-prepared nanocomposites exhibited a high degree of swelling and dual-phase release of phytoconstituents. Moreover, the developed controlled delivery systems were tested for antifungal and antioxidant potential. Importantly, the bioactivities of the prepared nanofibers could be improved further by using organic extracts which are generally enriched with phytoconstituents. Herein, we selected biodegradable and mucoadhesive biopolymers and an aqueous extract of neem for the development of controlled-delivery nanofibers by electrospinning through a sustainable and cleaner production process. Thus, the prepared biocompatible nanofibrous systems with biphasic release profile could be employed for biomedical applications including wound dressing, soft tissue scaffolds and as transdermal carriers.
Introduction
Plants have established their worth as an endless repository of promising bioactive molecules with a broad spectrum of therapeutic potential. Bioactive phytoconstituents have facilitated the synthesis of numerous modern drugs including antitumor, anti-HIV, anti-HCV, antivirals and anti-inflammatory etc. A major portion of the global population primarily relies on plant-based traditional medicines for all sorts of ailments (Choudhari et al., 2020; Mani et al., 2020). Over the past decades, medicinal chemists have shown significant interest to isolate the bioactive molecules from the routinely used and wild varieties of plants (Yao et al., 2017). Neem (Azadirachta indica), a member of the Meliaceae family, has been declared as the tree of the 21st century by the United Nations owing to its broad spectrum of medicinal and pharmacological properties (Upadhayay and Vigyan, 2014). This wonder plant is frequently available in the Indian subcontinent and is termed as a living pharmacy or village dispensary due to its unique and diverse pharmacological activities. It carries tannins, alkaloids, coumarins, terpenoids, and flavonoids etc as bioactive phytoconstituents. Each part of the plant has proven neuroprotective, hepatoprotective, anticancer, antibiotic, anti-inflammatory and antioxidant activities etc (Prakash et al., 2022).
Owing to the best safety profiles, plant-based biologically active compounds have become attractive choices for the safer and risk-free treatment of both communicable and non-communicable diseases. However, their therapeutic effects depend upon the bioavailability in the human body which is often encumbered by issues related to their physiochemical properties and structural complexity. They show pH sensitive structural instability, poor adsorption and undesirable off-target interactions. Over the last decade, the exceptional advancements in nanotechnology have helped the developments of various nano-delivery systems like nanosuspensions, nanoemulsions, nanoparticles and nanoliposomes etc which improved the efficacy and bioavailability of drugs and bioactive compounds (Shakeri and Sahebkar, 2016; Ahmad et al., 2020; Manickam et al., 2021). Further, the improvement of bioavailability and controlled delivery of bioactive compounds depends upon the size, shape and internal structures of nanoderived assemblies. The nanoformulations enable the natural compounds to withstand effects of pH, enzymes and temperature, ensuring their structural integrity and target-specific controlled release within the biological systems (Shikov et al., 2008; Puglia et al., 2019; McClements, 2020).
In recent years, the electrospinning has emerged as a promising technique for the preparation of nanofibers with unique properties like flexibility, high porosity, high surface area and aspect ratio. The electrospun nanofibers have shown potential applications in various scientific domains including energy (Sha et al., 2022; Zhu et al., 2022), environment (Kang et al., 2022; Lv et al., 2022), drug delivery (Kang et al., 2020; Li et al., 2022), sensors (Du et al., 2022; Zhang et al., 2022), functional textiles and medicated products (Khandaker et al., 2022; Wu et al., 2022) etc. In the context of nano-delivery systems, the polymer-based nanofibers have emerged as promising carriers for natural molecules, drugs and nanomaterials with wide applications in bioremediation, electrical and biomedical applications etc (Chen et al., 2020; Marinho et al., 2021; Sameen et al., 2022). Accordingly, a number of synthetic and natural polymers have been used to prepare nanofibers as nanocarriers of drugs, nanoparticles and biomolecules etc. However, their applications as plant extract products are still very limited, which has a potential broad market (Liu et al., 2022b). The electrospinning is fast-moving the coaxial (Ning et al., 2021), tri-axial (Zhao et al., 2021), side-by-side (Wang et al., 2022), and even tri-fluid side-by-side (Liu et al., 2022b) processes for creating complex nanostructures. However, the simplest single-fluid blending processes is still the mainstream, which holds great promise for large scale production, and always acts as a pioneer to expand new applications of electrospun nanofibers. Economic production of commercial products could be made possible by devising new methodologies with minimal energy consumption. The selection of polymer and optimization of various parameters helps to prepare nanofibers with desired physio-morphological properties. The chemical nature of the polymeric material significantly influence the loading capacity, initial burst and sustained release of the bioactive molecules. They could further stimulate the migration, cell adhesion and proliferation of loaded materials. Chitosan (CS) is a polycationic biopolymer showing a broad spectrum of applications in biomedical and pharmaceutical field. It displays strong antifungal and antibacterial potential due to its polycationic nature. Further, its biodegradable, biocompatible, mucoadhesive and nontoxic nature has made it an attractive choice for the development of nanocarriers (Silva et al., 2021). However, the electrospinning of CS is extremely difficult due to its low solubility and highly viscous nature. Alginate is also a biocompatible and biodegradable biopolymer with mucoadhesive and anti-infectious potential, an ideal candidate for delivery of bioactive agents in living systems. The electrospinning capacity of alginate is also limited due to its polyelectrolytic nature (Taemeh et al., 2020). Therefore, nonspinnable polymers are blended with some co-spinning agents to improve their mechanical strength and electrospinnability. PEO is a biodegradable biopolymer approved for internal use in pharmaceutical products, cosmetics, food and products of personal care. Hence, it is suggested as a suitable co-spinning agent for nonspinnable polymers like chitosan and alginate (Grothe et al., 2016; Grimmelsmann et al., 2017; Dodero et al., 2020).
Keeping in view, the unprecedented therapeutic potential of A. indica and advancements in electrospinning technology, we envisioned to develop biopolymer-based nanofibers loaded with neem extract as eco-friendly and controlled releasing nanocarrier.
Materials and methods
Sodium alginate and chitosan 75% (deacetylated) were purchased from Sigma-Aldrich while all other chemicals were of analytical grade and received from local vender. The leaves of A. indica (AI) were arranged from botanical gardens of Agriculture University, Faisalabad.
Preparation of alginate/chitosan/PEO/AI solution
At first, 1.5% w/v sodium alginate solution was prepared using distilled water following 1% chitosan solution in 0.2% v/v acetic acid. For matrix preparation, the aforesaid solutions were mixed in 3:1 ratio. Then, 2%, 4% and 6% (w/v) A. indica leave extract solution were added in alginate/chitosan solution to prepare three precursor solutions for electrospinning. Then 1 ml of 1% PEO solution was added to each solution just before the electrospinning.
Electrospinning process
Ten mL of the each precursor solution was loaded into a syringe with the needle diameter of 0.45 mm for the preparation of nanofibers using electrospinning apparatus (Elmarco, nanospider). The precursor solution was electrospun and the nanofibers were collected at a grounded drum collector 12 cm away from the tip of the needle. The solution flow rate, the applied voltage, the humidity, and the time for electrospinning were 1 ml/h, 15 kV, 40–50%, and about 10 h, respectively. The CS/ALG/PEO/AI composite nanofibers obtained from above mentioned 3 precursor solutions were dried at room temperature overnight and named as NF1, NF2 and NF3.
Characterization of prepared nanofibers
Scanning electron microscopy
The surface morphology of the prepared NF1, NF2, and NF3 composite nanofibers was examined by a scanning electron microscope (SEM) (SEM JSM-7000 F) operating at 10 kV-voltage. The prepared fibrous samples were coated with electrically conductive carbon. The SEM images were analyzed using 10,000 times optical magnification. Finally, the fiber size distribution was studied using Origin ver. 8.
Fourier-transform infrared spectroscopy
The PerkinElmer (spectrum 100) FT-IR spectrometer was used to study the functional group interactions between polymeric constituents and neem extract. The spectra were recorded from 400 to 4,000 cm−1.
X-ray diffraction pattern
The crystalline nature of the prepared nanofibers was evaluated using the X-ray diffractometer (XRD) (Rigaku, SmartLab) operating with 40 mA current, 45 kV voltage and equipped with Cu-Kα radiation source.
Thermogravimetric analyses
Thermal degradation behaviors of the prepared nanofibers were studied under a nitrogen atmosphere using (Thermo Scientific TGA), operating at 15°C/min from 25 to 350°C.
Measurement of antifungal activity
The prepared nanofibers were tested against the following fungal strains Candida albicans, Aspergillus flavus, Microsporum canis and Fusarium solani using agar diffusion and micro-dilution methods. The fungal cultures were prepared using Sabouraud’s dextrose broth. A sterile stick was used to spread 100 µl of fungal strain on the surface of the agar plate. Then, 2 mm wells were prepared in each culture plate followed by the addition of drug (control), DMSO (negative control) and 10 µl of each nanofiber sample. Subsequently, the cultures were subjected to incubation at 37°C for 48 h. The measurement of clear zones around the nanofiber samples provided their respective antifungal activities. The experiment was performed in triplicate and the antifungal activities are given with mean ± SD (Fonseca et al., 2021).
Measurement of antioxidant activity
The antioxidant capacity of prepared nanofibers was analyzed employing the DPPH assay (Bagheri et al., 2022). Samples of different concentrations from each fiber were prepared using methanol. After that, 1 ml of 50 µg per ml DPPH was mixed with 1 ml of each sample for half an hr. The ascorbic acid was used as an equivalent standard, 50 µg per ml DPPH as control and a subsequent change in absorbance of DPPH at 517 nm was measured using UV–visible spectrophotometer (CECIL CE 7200). The antioxidant activities of prepared nanofibers were calculated as follows using a standard curve,
The % disappearance of DPPH plotted as a function of sample concentration provided IC50 values.
Releasing behavior of bioactive constituents
The prepared nanofibers NF1, NF2 and NF3 were dipped in 50 ml of acetate buffer with pH 5.5 at 37°C as a simulation of human skin (Sadri et al., 2015). The samples were stirred and incubated at room temperature. After specific time intervals, aliquots of each sample (1 ml) were taken and diluted to 5 ml with fresh buffer solution and the concentrations were measured spectrophotometrically.
Swelling and weight loss studies
For weight loss studies, prespecified weights of NF1, NF2 and NF3 were immersed in deionized water at 35 ± 2°C for specific time periods. The surface of the samples was dried with filter paper following the incubation period. The surface dried samples were kept at 50°C before calculating weight loss (Nista et al., 2015).
Weight Loss (%) = Wa-Wb/Wa × 100.
Wa = weight of the dried sample before water exposure.
Wb = weight of re-dried samples after water exposure.
The weight of the swollen samples (Sw) and the finally dried samples were used for the calculation of the swelling rate.
Swelling ratio % = Ws-Wd/Wd × 100.
Ws = swelling weight of samples after water exposure.
Wd or Wb = weight of re-dried samples after water exposure.
Results and discussion
The SEM was used to examine the morphologies of the as-prepared nanofibers (Figure 1). For alginate/chitosan/PEO/neem extract composite solutions with 2, 4 and 6% of neem extracts, nanofibers were produced from 4 to 6% solutions however, some beads were observed in case of solution with 2% neem extract. Suggesting that the high concentration of neem extract improved the nanofiber formation. The increase of phytoconstituents increased the defect-free formation of nanofibers. The viscosity and solid contents of the of the precursor solution highly influence the electrospinning process. In this case, the presence of phytoconstituents controlled the threshold viscosity of precursors facilitating the electrospinning process. The SEM analyses revealed the average diameter of each of the electrospun nanofibrous compositions was 120 nm.
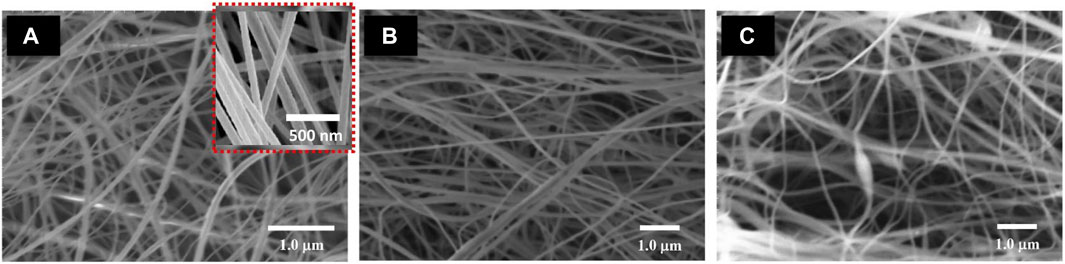
FIGURE 1. SEM images of (A) alginate/chitosan/PEO/neem extract composite (2%) (B) alginate/chitosan /PEO/neem extract composite (4%) (C) alginate/chitosan/PEO/neem extract composite (6%).
The prepared nanofibers were analyzed by FTIR (Figure 2). The available spectra of the pure alginate, chitosan, PEO and neem extract were examined. In chitosan, the peaks due to O–H and N–H stretch were observed at 3,365 and 3,302 cm−1 while amide bond at 1,654 and 1,593 cm−1. In case of alginate, O–H stretch was observed at 3,327 cm−1 while antisymmetric and symmetric carboxyl stretch at 1,603 cm−1 due to the and one at 1,413 cm−1. In our case, the spectra of the fibers showed broader peaks at 1,416 cm−1 and 1,596 cm−1 indicating the overlapping of carboxylic groups from both polymers. This corresponds to anionic complexation of amine groups of chitosan and carboxylic groups of alginate (George and Abraham, 2006; Liu et al., 2012). This interaction cause changes in the absorption bands of interacting functionalities. As a result, the amine carboxylic peaks showed significant shift of acid near 1,621 cm−1 (Cruz, 2004), (Costa Jr and Mansur, 2008). The specific peak of -NH2 group disappeared which is linked to the formation of NH3+ (Liu et al., 2012). Wang et al. also described such electrostatic interactions between amine and carboxyl groups and shifting of peaks (Wang et al., 2001). Further, the natural polyphenols in neem extract exhibit peaks due to O-H stretching at 3,414 cm−1 (Singh et al., 2010) and C-H bending vibration at 1,460 cm−1. Moreover, C-H and C-OH stretching vibrations were appeared at 2,925 and 1,238 cm−1 respectively. These peaks are attributed to the presence of flavonoids and polyphenols. In aromatic ring C=C stretching vibrations were observed at 1,644 cm−1. The carbonyl stretching vibration of carboxylic acid and C-H in CH3 peaks were observed at 1745 and 1,379 cm−1 (Elumalai and Velmurugan, 2015). The terpenoids in the neem extract exhibit C-O-C- linkage at 1,114 cm−1 (Ahmed et al., 2016). The peaks at 1,652 cm−1 and 1744 cm−1 were attributed to amide stretching and C=O stretching respectively in FTIR spectrum of synthesized composite. The binding of –COOMe group of azadirachtin of neem with the NH2 group of chitosan forms –NH-CO and reduce free -NH2 groups and shifting the peak at 3,425 cm−1 (Chakraborty et al., 2015). A single phase is developed due to the formation of the anionic complex in nanofibers.
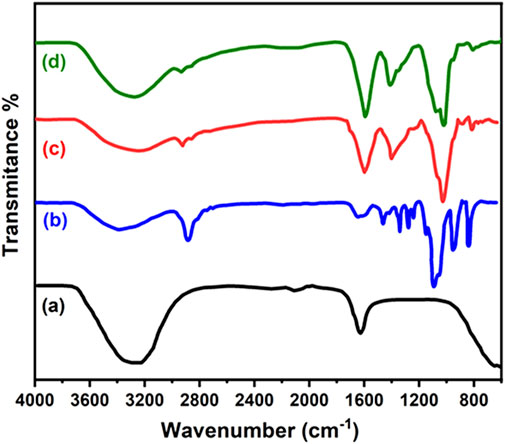
FIGURE 2. FTIR spectra of (A) pure neem leaf extract (B) alginate/chitosan/PEO/neem extract composite (2%) (C) alginate/chitosan /PEO/neem extract composite (4%) (D) alginate/chitosan/PEO/neem extract composite (6%).
The Figure 3A showed X-ray diffraction spectroscopy results revealing crystalline and amorphous nature of alginate/chitosan/PEO/neem extract composite (6%). The crystalline nature of chitosan has been validated by a peak at 2θ = 20.3⁰ which is attributed to reflection plane of (200). The predominant amorphous nature is reflected by the broad region from 20.3 to 80⁰. The hydrogen bonding caused free-energy balance which gives chitosan a semi- crystalline in nature (Costa-Júnior et al., 2009).
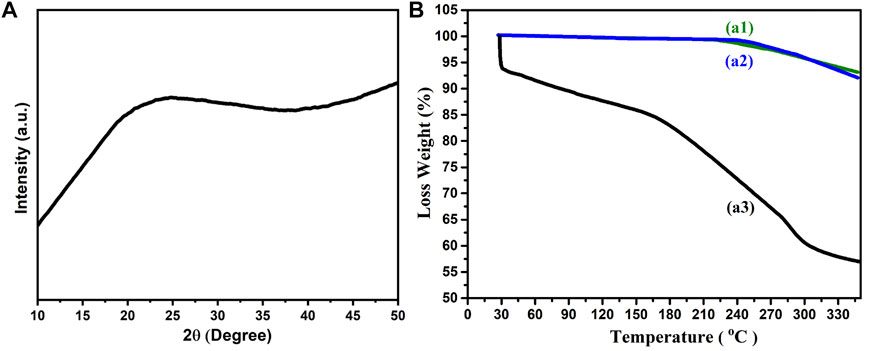
FIGURE 3. (A) XRD spectra of alginate/chitosan/PEO/neem extract composite (6%) (B) TGA spectra of (a1) alginate/chitosan/PEO/neem extract composite (2%) (b1) alginate/chitosan /PEO/neem extract composite (4%) (c1) alginate/chitosan/PEO/neem extract composite (6%).
Thermogravimetric curves of as-prepared nanofibers (chitosan, alginate, PEO and neem extract) are shown in Figure 3B. The TGA thermogram of alginate/chitosan/PEO/neem extract composite showed 2 region of weight loss; between 360 and 415°C is related to the PEO while refers to the alginate ∼160°C (Caykara et al., 2005). The peaks in range 359–391°C and 225–250°C reflects PEO and alginate degradation respectively. The degradation temperature of chitosan is influenced by its degree of acetylation and crystallinity (Wang et al., 2020). The maximum degradation of chitosan and appeared at 270°C which is less than the cited work (Nam et al., 2010). This variance is possibly due to the incorporation of neem extract and PEO, which changes the crystallinity of the as-prepared nanocomposite. The decomposition of pure chitosan membrane exhibited one exothermic peak at 307°C. The decomposition peak moves to 217°C in case of CS/PEO indicating the influence of additives on composite stability. Accordingly, we have observed different onset of weight loss for each composite due to the addition of neem extract. The alginate/chitosan/PEO/neem extract (6%) composite was thermally more stable than composites with (2 and 4%) extract. The complexation–mediated reorganization of polymeric chains influenced thermal degradation shifting it to a lower temperature region (Fuzlin et al., 2020).
The A. indica displays a broad spectrum of therapeutics due to a rich source of bioactive constitutes. The azadirachtin is the most important phytochemical while quercetin, salannin, nimbidol, nimbin and nimbolinin etc are varyingly concentrated in different parts of neem. These bioactive constituents activate antioxidant enzyme for antioxidant activities, rupture cell wall for antibacterial properties and modulate cellular pathways for chemopreventive effects. The antibacterial and antifungal activities were shown by the quercetin, β-sitoterol and other polyphenols purified from leaves samples (Rahmani et al., 2018; Saleem et al., 2018). The aqueous exacts of leaves of A. indica are already known for their strong antifungal potential (Leontopoulos et al., 2017). In our case, the prepared nanofibers were found effective against, C. albicans, A. flavus, M. canis and F. solani. The prepared nanofibers exhibited extract concentration-dependent antifungal activities (Table 1).
Reactive oxygen species (ROS) disrupt metabolic pathways and cellular signaling and delays wound healing and regeneration process. Therefore, controlled ROS generation using phytochemical-based antioxidants is a key for an accelerated wound healing. The phytochemicals especially the polyphenols quench ROS through redox reactions or they activate antioxidant enzymes (Engwa, 2018). Leave samples of A. indica were found to carry azadirachtin, nimbanene, nimbin and other polyphenolic flavonoids like β–sitosterol and quercetin (Sarah et al., 2019). The aqueous extract of A. indica leaves mainly carries azadirachtin and nimbin which are responsible for their high antioxidant potential (Airaodion et al., 2019). Another study revealed the concentration-dependent antioxidant potential of azadirachtin and nimbolide. Both of the compounds were found to upregulate enzymatic antioxidant and prevented DNA oxidation (Iman et al., 2021). Other few such studies also explored the antioxidant properties of leave, flowers, fruit and bark extracts etc. In our case, the prepared nanofibers loaded with aqueous extract exhibited concentration-dependent ROS scavenging properties (Table 2).
In our case, the prepared nanofibers loaded with leave’s aqueous extract exhibited antioxidant and antifungal potential. As the literature suggests, the aqueous extracts of A. indica leaves exhibit anti-inflammatory (Modi et al., 2021), hepatoprotective (Sani et al., 2020), antibacterial (Hikaambo et al., 2022), wound healing (Ugoeze et al., 2021), Antidiabetic (Dholi et al., 2011; Hikaambo et al., 2022), etc. Further, the organic extracts of neem leaves have been reported to show anticancer (Kashif et al., 2019), virucidal activity (Baildya et al., 2021), antimalarial activity (Udeinya et al., 2008; Akin-Osanaiya et al., 2013; Afolabi et al., 2021) and neuroprotective effects (Sandhir et al., 2021). It has always been challenging to control the initial release of hydrophilic molecules from delivery systems. The medicated nanofibers release the loaded active ingredients upon encountering water or release media. Typically, the release profile could be pulsatile showing a fast release (Bhusnure et al., 2021) or a sustained release showing a time-dependent controlled release (Abasalta et al., 2022). A fast release is desired when a quick drug action is required under conditions like heart attack, fever or pain. Whereas sustained release is preferred when administration frequency needs to be reduced in some cases. However, new electrospinning approaches like side-by-side electrospinning, electrospraying and coaxial electrospinning or their combine forms are adopted to achieve more sophisticated profiles like a biphasic or dual-phase release for special therapeutic applications (Lv et al., 2021; Liu et al., 2022a). However, these multiple-fluid electrospinning processes are not easy for large-scale productions.
The release profile greatly depends upon the hydrophobic or hydrophilic nature of the polymeric carriers. The loaded molecules are freed into the dissolution medium through a diffusion mechanism when the carrier matrix is water-insoluble (Yang et al., 2020; Jain et al., 2022). In our case, the blending of hydrophilic and hydrophobic polymers along with aqueous extract helped to control the initial release and managed a prolonged and sustained release of bioactive constituents (Figure 4). The prepared nanofibers exhibited a biphasic release pattern. A burst release was observed in the first half hr followed by a diffusion-controlled sustained release over a period of 48 h. A dual-phase or biphasic release controls the delivery of active ingredients in the most suited way in biological systems. In fact an initial pulsatile release provides quick relief followed by a sustained release for a prolonged therapeutic effect (Zhao and Cui, 2020). The maximum and the minimum release percentile at 48 h was exhibited by the sample NF3 and NF1 respectively. Presumably, the NF1 samples exhibited a slow release percentile owing to more percentage of nano-net structure and low loading of extract. An initial burst release is desirous sometimes to deliver higher amounts of active ingredients to control infections at acute injury phase. Generally, higher burst release is observed due to the direct incorporation of active ingredients into electrospinning solution which cause over accumulation of bioactive molecules on the surface of nanofibers (Amiri et al., 2020). The slow and sustained release of the extract is attributed to the structural morphology and strong interactions of carboxylic and amide groups of NFs with bioactive molecules. The high surface area and small diameter provides a short diffusion pathway for the release of active constituents. Further, higher contents of the extract caused a higher degree of swelling of nanofibrous structures resulting in faster release of active molecules. Therefore, the NF3 sample exhibited a higher initial burst followed by a sustained release due to the higher loading of molecules. The higher swelling rate helps to maintain a higher content of moisture which keeps the wound surface wet and facilitates an accelerated healing process (Prakash et al., 2021). As described earlier, increased swelling also favors the delivery of bioactive constituents for the recovery of wounds (Sasmal and Datta, 2019).
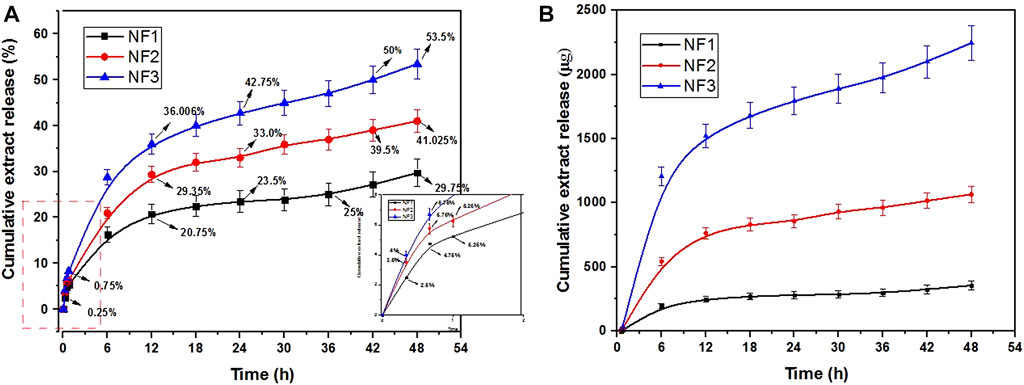
FIGURE 4. Biphasic controlled release of bioactive compounds from prepared nanofibers (A) Cumulative release (%), (B) Quantitative cumulative release.
As expected, the swelling rate of samples increased with time owing to the hydrophilic nature of the polymeric matrix (Shekarforoush et al., 2018; Rodríguez-Rodríguez et al., 2020). In weight loss studies, the samples showed a steady weight loss due to the leaching of PEO and constituents of neem extract suggesting a stable complex of polycationic and polyanionic polymers (Table 3). The samples of NF3 showed relatively a bit higher weight loss at 24 h due to the leaching of more phytoconstituents. Therefore, higher swelling rate and sustain release of active molecules suggests the prepared nanofibers as potential candidates for the development of wound dressings and other medicated materials. Further, a single-fluid electrospinning approach has been disclosed for the preparation of medicated-nanofibers with sophisticated double-phase release profile.
Conclusion
The prepared biopolymer-based nanofibers loaded with neem leave extract exhibited antioxidant and antifungal properties. They also showed high swelling rate and sustained release of phytoconstituents. Further, a single-fluid electrospinning approach has been disclosed for the preparation of medicated-nanofibers with sophisticated double-phase release profile. Thus prepared nanofibers could be employed as wound dressing, soft tissue scaffolds and as transdermal carriers.
Data availability statement
The original contributions presented in the study are included in the article/Supplementary Material, further inquiries can be directed to the corresponding author.
Author contributions
AH supervised the extraction and purification process, ZR and MAQ Supervised the electrospinning process, TA (interpreted Characterization studies, TR Data curation and analysis, RN, SI, and SB Proof reading and supervision of biological studies and TF overall supervision, conceptualization of idea, Manuscript write-up.
Conflict of interest
The authors declare that the research was conducted in the absence of any commercial or financial relationships that could be construed as a potential conflict of interest.
Publisher’s note
All claims expressed in this article are solely those of the authors and do not necessarily represent those of their affiliated organizations, or those of the publisher, the editors and the reviewers. Any product that may be evaluated in this article, or claim that may be made by its manufacturer, is not guaranteed or endorsed by the publisher.
References
Abasalta, M., Asefnejad, A., Khorasani, M. T., Saadatabadi, A. R., and Irani, M. (2022). Adsorption and sustained release of doxorubicin from N-carboxymethyl chitosan/polyvinyl alcohol/poly (ε-caprolactone) composite and core-shell nanofibers. J. Drug Deliv. Sci. Technol. 67, 102937. doi:10.1016/j.jddst.2021.102937
Afolabi, O., Simon-Oke, I., and Oladokun, O. (2021). Antiplasmodial activity of ethanolic extract of neem leaf (Azadirachta indica) in albino mice infected with plasmodium berghei. Int. Arch. Clin. Pharmacol. 7, 24. doi:10.23937/2572-3987.1510024
Ahmad, R., Srivastava, S., Ghosh, S., and Khare, S. K. (2020). Phytochemical delivery through nanocarriers: A review. Colloids Surfaces B Biointerfaces 197, 111389. doi:10.1016/j.colsurfb.2020.111389
Ahmed, S., Saifullahahmad, M., Swami, B. L., and Ikram, S. (2016). Green synthesis of silver nanoparticles using Azadirachta indica aqueous leaf extract. J. Radiat. Res. Appl. Sci. 9, 1–7. doi:10.1016/j.jrras.2015.06.006
Airaodion, A. I., Olatoyinbo, P. O., Ogbuagu, U., Ogbuagu, E. O., Akinmolayan, J. D., Adekale, O. A., et al. (2019). Comparative assessment of phytochemical content and antioxidant potential of Azadirachta indica and Parquetina nigrescens leaves. Asian Plant Res. J. 2, 1–14. doi:10.9734/aprj/2019/v2i330045
Akin-Osanaiya, B., Nok, A., Ibrahim, S., Inuwa, H., Onyike, E., Amlabu, E., et al. (2013). Antimalarial effect of neem leaf and neem stem bark extracts on Plasmodium berghei infected in the pathology and treatment of malaria. Int. J. Res. Biochem. Biophysics 3, 7–14.
Amiri, N., Ajami, S., Shahroodi, A., Jannatabadi, N., Darban, S. A., Bazzaz, B. S. F., et al. (2020). Teicoplanin-loaded chitosan-PEO nanofibers for local antibiotic delivery and wound healing. Int. J. Biol. Macromol. 162, 645–656. doi:10.1016/j.ijbiomac.2020.06.195
Bagheri, M., Validi, M., Gholipour, A., Makvandi, P., and Sharifi, E. (2022). Chitosan nanofiber biocomposites for potential wound healing applications: Antioxidant activity with synergic antibacterial effect. Bioeng. Transl. Med. 7, e10254. doi:10.1002/btm2.10254
Baildya, N., Khan, A. A., Ghosh, N. N., Dutta, T., and Chattopadhyay, A. P. (2021). Screening of potential drug from azadirachta indica (neem) extracts for SARS-CoV-2: An insight from molecular docking and MD-simulation studies. J. Mol. Struct. 1227, 129390. doi:10.1016/j.molstruc.2020.129390
Bhusnure, O. G., Gholve, S. B., Giram, P. S., Gaikwad, A. V., Udumansha, U., Mani, G., et al. (2021). Novel 5-flurouracil-Embedded non-woven PVA-PVP electrospun nanofibers with enhanced anti-cancer efficacy: Formulation, evaluation and in vitro anti-cancer activity. J. Drug Deliv. Sci. Technol. 64, 102654. doi:10.1016/j.jddst.2021.102654
Caykara, T., Demirci, S., Eroğlu, M. S., and Güven, O. (2005). Poly (ethylene oxide) and its blends with sodium alginate. Polymer 46, 10750–10757. doi:10.1016/j.polymer.2005.09.041
Chakraborty, A., Chakraborty, R., Sen, B., Kumari, P., and Sarkar, P. (2015). Antibacterial finishing of silk fabric chitosan-neem nano emulsion. Int. J. Curr. Biotechnol. 3, 10–20.
Chen, S., John, J. V., Mccarthy, A., and Xie, J. (2020). New forms of electrospun nanofiber materials for biomedical applications. J. Mat. Chem. B 8, 3733–3746. doi:10.1039/d0tb00271b
Choudhari, A. S., Mandave, P. C., Deshpande, M., Ranjekar, P., and Prakash, O. (2020). Phytochemicals in cancer treatment: From preclinical studies to clinical practice. Front. Pharmacol. 10, 1614. doi:10.3389/fphar.2019.01614
Costa, E. D. S., and Mansur, H. S. (2008). Preparação e caracterização de blendas de quitosana/poli (álcool vinílico) reticuladas quimicamente com glutaraldeído para aplicação em engenharia de tecido. Quim. Nova 31, 1460–1466. doi:10.1590/s0100-40422008000600034
Costa-Júnior, E. S., Barbosa-Stancioli, E. F., Mansur, A. A., Vasconcelos, W. L., and Mansur, H. S. (2009). Preparation and characterization of chitosan/poly (vinyl alcohol) chemically crosslinked blends for biomedical applications. Carbohydr. Polym. 76, 472–481. doi:10.1016/j.carbpol.2008.11.015
Cruz, M. C. P. (2004). Influencia do poli (etileno glicol)(PEG) no processo de microencapsulação da oxitetraciclina no sistema alginato/quitosana: Modelamento" in vitro'da liberação oral.
Dholi, S. K., Raparla, R., Mankala, S. K., and Nagappan, K. (2011). Invivo Antidiabetic evaluation of Neem leaf extract in alloxan induced rats. J. Appl. Pharm. Sci. 1, 100–105.
Dodero, A., Alloisio, M., Vicini, S., and Castellano, M. (2020). Preparation of composite alginate-based electrospun membranes loaded with ZnO nanoparticles. Carbohydr. Polym. 227, 115371. doi:10.1016/j.carbpol.2019.115371
Du, Y., Zhang, X., Liu, P., Yu, D.-G., and Ge, R. (2022). Electrospun nanofiber-based glucose sensors for glucose detection. Front. Chem. 10, 944428. doi:10.3389/fchem.2022.944428
Elumalai, K., and Velmurugan, S. (2015). Green synthesis, characterization and antimicrobial activities of zinc oxide nanoparticles from the leaf extract of Azadirachta indica (L.). Appl. Surf. Sci. 345, 329–336. doi:10.1016/j.apsusc.2015.03.176
Engwa, G. A. (2018). Free radicals and the role of plant phytochemicals as antioxidants against oxidative stress-related diseases. Phytochemicals Source Antioxidants Role Dis. Prev.
Fonseca, L. M., Souza, E. J. D., Radünz, M., Gandra, E. A., Da Rosa Zavareze, E., and Dias, A. R. G. (2021). Suitability of starch/carvacrol nanofibers as biopreservatives for minimizing the fungal spoilage of bread. Carbohydr. Polym. 252, 117166. doi:10.1016/j.carbpol.2020.117166
Fuzlin, A., Nagao, Y., Misnon, I., and Samsudin, A. (2020). Studies on structural and ionic transport in biopolymer electrolytes based on alginate-LiBr. Ionics 26, 1923–1938. doi:10.1007/s11581-019-03386-7
George, M., and Abraham, T. E. (2006). Polyionic hydrocolloids for the intestinal delivery of protein drugs: Alginate and chitosan—a review. J. Control. Release 114, 1–14. doi:10.1016/j.jconrel.2006.04.017
Grimmelsmann, N., Grothe, T., Homburg, S., and Ehrmann, A. (2017). Electrospinning and stabilization of chitosan nanofiber mats. IOP conference series: Materials science and engineering. Bristol: IOP Publishing, 102006.
Grothe, T., Brikmann, J., and Ehrmann, A. (2016). “PEO as spinnable polymer and spinning-agent for non-spinnable materials,” in Proceedings of Aachen-Dresden-Denkendorf International Textile Conference.
Hikaambo, C. N. A., Kaacha, L., Mudenda, S., Nyambe, M. N., Chabalenge, B., Phiri, M., et al. (2022). Phytochemical Analysis and Antibacterial Activity of Azadirachta indica Leaf Extracts against Escherichia coli. Pharmacol. Pharm. 13, 1–10. doi:10.4236/pp.2022.131001
Iman, M., Taheri, M., and Bahari, Z. (2021). The anti-cancer properties of neem (Azadirachta indica) through its antioxidant activity in the liver; its pharmaceutics and toxic dosage forms; a literature review. J. Complementary Integr. Med. 19, 203–211. doi:10.1515/jcim-2021-0009
Jain, D. D., Tambe, S. M., and Amin, P. D. (2022). Formulation performance window for manufacturing cellulose-based sustained-release mini-matrices of highly water-soluble drug via hot-melt extrusion technology. Cellulose 29, 3323–3350. doi:10.1007/s10570-022-04458-0
Kang, S., Hou, S., Chen, X., Yu, D., Wang, L., Li, X., et al. (2020). Energy-saving electrospinning with a concentric teflon-core rod spinneret to create medicated nanofibers. Polymers 12, 2421. doi:10.3390/polym12102421
Kang, S., Zhao, K., Yu, D.-G., Zheng, X., and Huang, C. (2022). Advances in biosensing and environmental monitoring based on electrospun nanofibers. Adv. Fiber Mat. 4, 404–435. doi:10.1007/s42765-021-00129-0
Kashif, M., Hwang, Y., Kim, W.-J., and Kim, G. (2019). In-vitro morphological assessment of apoptosis induced by nimbolide; A limonoid from Azadirachta indica (Neem Tree). Iran. J. Pharm. Res. 18, 846–859. doi:10.22037/ijpr.2019.2391
Khandaker, M., Nomhwange, H., Progri, H., Nikfarjam, S., and Vaughan, M. B. (2022). Evaluation of polycaprolactone electrospun nanofiber-composites for artificial skin based on dermal fibroblast culture. Bioengineering 9, 19. doi:10.3390/bioengineering9010019
Leontopoulos, S., Skenderidis, P., Anatolioti, V., Kokkora, M., Tsilfoglou, S., Petrotos, K., et al. (2017). Antifungal activity of Azadirachta indica aqueous and non-aqueous extracts on Colletotrichum gloeosporioides, Botryodiplodia theobromae and Fusarium solani. A first approach. Int. J. Food Biosyst. Eng. 6, 38.
Li, J., Liu, Y., and Abdelhakim, H. E. (2022). Drug delivery applications of coaxial electrospun nanofibres in cancer therapy. Molecules 27, 1803. doi:10.3390/molecules27061803
Liu, D., Yuan, X., and Bhattacharyya, D. (2012). The effects of cellulose nanowhiskers on electrospun poly (lactic acid) nanofibres. J. Mat. Sci. 47, 3159–3165. doi:10.1007/s10853-011-6150-z
Liu, H., Jiang, W., Yang, Z., Chen, X., Yu, D.-G., and Shao, J. (2022a). Hybrid films prepared from a combination of electrospinning and casting for offering a dual-phase drug release. Polymers 14, 2132. doi:10.3390/polym14112132
Liu, H., Wang, H., Lu, X., Murugadoss, V., Huang, M., Yang, H., et al. (2022b). Electrospun structural nanohybrids combining three composites for fast helicide delivery. Adv. Compos. Hybrid. Mat. 5, 1017–1029. doi:10.1007/s42114-022-00478-3
Lv, H., Guo, S., Zhang, G., He, W., Wu, Y., and Yu, D.-G. (2021). Electrospun structural hybrids of acyclovir-polyacrylonitrile at acyclovir for modifying drug release. Polymers 13, 4286. doi:10.3390/polym13244286
Lv, H., Zhang, M., Wang, P., Xu, X., Liu, Y., and Yu, D.-G. (2022). Ingenious construction of Ni(DMG)2/TiO2-decorated porous nanofibers for the highly efficient photodegradation of pollutants in water. Colloids Surfaces A Physicochem. Eng. Aspects 650, 129561. doi:10.1016/j.colsurfa.2022.129561.129561
Mani, J. S., Johnson, J. B., Steel, J. C., Broszczak, D. A., Neilsen, P. M., Walsh, K. B., et al. (2020). Natural product-derived phytochemicals as potential agents against coronaviruses: A review. Virus Res. 284, 197989. doi:10.1016/j.virusres.2020.197989
Manickam, V., Sundar, V., Panchangam, R. L., and Tamizhselvi, R. (2021). Nanotechnology in delivery and targeting of phytochemicals. Nanopharmaceuticals Princ. Appl. Vol. 2.
Marinho, B. A., de Souza, S., de Souza, A. A. U., and Hotza, D. (2021). Electrospun TiO2 nanofibers for water and wastewater treatment: A review. J. Mat. Sci. 56, 5428–5448. doi:10.1007/s10853-020-05610-6
Mcclements, D. J. (2020). Advances in nanoparticle and microparticle delivery systems for increasing the dispersibility, stability, and bioactivity of phytochemicals. Biotechnol. Adv. 38, 107287. doi:10.1016/j.biotechadv.2018.08.004
Modi, C. M., Patel, H. B., Patel, U. D., Paida, B. V., Ramchandani, D., Patel, P. M., et al. (2021). Anti-inflammatory and antioxidant potential of Azadirachta Indica flower. J. Veterinary Pharmacol. Toxicol. 20, 13.
Nam, Y. S., Park, W. H., Ihm, D., and Hudson, S. M. (2010). Effect of the degree of deacetylation on the thermal decomposition of chitin and chitosan nanofibers. Carbohydr. Polym. 80, 291–295. doi:10.1016/j.carbpol.2009.11.030
Ning, T., Zhou, Y., Xu, H., Guo, S., Wang, K., and Yu, D.-G. (2021). Orodispersible membranes from a modified coaxial electrospinning for fast dissolution of diclofenac sodium. Membranes 11, 802. doi:10.3390/membranes11110802
Nista, S. V. G., Bettini, J., and Mei, L. H. I. (2015). Coaxial nanofibers of chitosan–alginate–PEO polycomplex obtained by electrospinning. Carbohydr. Polym. 127, 222–228. doi:10.1016/j.carbpol.2015.03.063
Prakash, J., Venkataprasanna, K., Bharath, G., Banat, F., Niranjan, R., and Venkatasubbu, G. D. (2021). In-vitro evaluation of electrospun cellulose acetate nanofiber containing Graphene oxide/TiO2/Curcumin for wound healing application. Colloids Surfaces A Physicochem. Eng. Aspects 627, 127166. doi:10.1016/j.colsurfa.2021.127166
Prakash, V., Akhtar, S., Kumar, J., Mishra, S. K., and Pandey, R. R. (2022). “Neem: The multifaceted and versatile tree,” in Current trends in medicinal chemistry. Editors R. R. PANDEY, and J. KUMAR (India: Thanuj International Publishers).
Puglia, C., Pignatello, R., Fuochi, V., Furneri, P. M., Lauro, M. R., Santonocito, D., et al. (2019). Lipid nanoparticles and active natural compounds: A perfect combination for pharmaceutical applications. Curr. Med. Chem. 26, 4681–4696. doi:10.2174/0929867326666190614123835
Rahmani, A., Almatroudi, A., Alrumaihi, F., and Khan, A. (2018). Pharmacological and therapeutic potential of neem (Azadirachta indica). Pharmacogn. Rev. 12, 250. doi:10.4103/phrev.phrev_8_18
Rodríguez-Rodríguez, R., Espinosa-Andrews, H., Velasquillo-Martínez, C., and García-Carvajal, Z. Y. (2020). Composite hydrogels based on gelatin, chitosan and polyvinyl alcohol to biomedical applications: A review. Int. J. Polym. Mater. Polym. Biomaterials 69, 1–20. doi:10.1080/00914037.2019.1581780
Sadri, M., Arab-Sorkhi, S., Vatani, H., and Bagheri-Pebdeni, A. (2015). New wound dressing polymeric nanofiber containing green tea extract prepared by electrospinning method. Fibers Polym. 16, 1742–1750. doi:10.1007/s12221-015-5297-7
Saleem, S., Muhammad, G., Hussain, M. A., and Bukhari, S. N. A. (2018). A comprehensive review of phytochemical profile, bioactives for pharmaceuticals, and pharmacological attributes of Azadirachta indica. Phytotherapy Res. 32, 1241–1272. doi:10.1002/ptr.6076
Sameen, D. E., Ahmed, S., Lu, R., Li, R., Dai, J., Qin, W., et al. (2022). Electrospun nanofibers food packaging: Trends and applications in food systems. Crit. Rev. Food Sci. Nutr. 62, 6238–6251. doi:10.1080/10408398.2021.1899128
Sandhir, R., Khurana, M., and Singhal, N. K. (2021). Potential benefits of phytochemicals from Azadirachta indica against neurological disorders. Neurochem. Int. 146, 105023. doi:10.1016/j.neuint.2021.105023
Sani, I., Umar, R., Hassan, S., Faruq, U., Bello, F., Aminu, H., et al. (2020). Hepatoprotective effect of Azadirachta indica leaf fractionated extracts against snake venom toxicity on albino rats. Saudi J. Biomed. Res. 5, 112–117. doi:10.36348/sjbr.2020.v05i06.004
Sarah, R., Tabassum, B., Idrees, N., and Hussain, M. K. (2019). Bio-active compounds isolated from Neem tree and their applications. Nat. Bio-active Compd.
Sasmal, P., and Datta, P. (2019). Tranexamic acid-loaded chitosan electrospun nanofibers as drug delivery system for hemorrhage control applications. J. Drug Deliv. Sci. Technol. 52, 559–567. doi:10.1016/j.jddst.2019.05.018
Sha, Z., Boyer, C., Li, G., Yu, Y., Allioux, F.-M., Kalantar-Zadeh, K., et al. (2022). Electrospun liquid metal/PVDF-HFP nanofiber membranes with exceptional triboelectric performance. Nano Energy 92, 106713. doi:10.1016/j.nanoen.2021.106713
Shakeri, A., and Sahebkar, A. (2016). Opinion paper: Nanotechnology: A successful approach to improve oral bioavailability of phytochemicals. Recent Pat. Drug Deliv. Formul. 10, 4–6. doi:10.2174/1872211309666150611120724
Shekarforoush, E., Ajalloueian, F., Zeng, G., Mendes, A. C., and Chronakis, I. S. (2018). Electrospun xanthan gum-chitosan nanofibers as delivery carrier of hydrophobic bioactives. Mater. Lett. 228, 322–326. doi:10.1016/j.matlet.2018.06.033
Shikov, A., Pozharitskaya, O., Miroshnyk, I., Mirza, S., Karlina, M., Hirsjärvi, S., et al. (2008). Nanosystems as a tool for enhancing bioavailability of natural compounds. Pharmacia 7, 53.
Silva, A. O., Cunha, R., Hotza, D., and Machado, R. (2021). Chitosan as a matrix of nanocomposites: A review on nanostructures, processes, properties, and applications. Carbohydr. Polym. 272, 118472. doi:10.1016/j.carbpol.2021.118472
Singh, B., Sharma, D., Kumar, R., and Gupta, A. (2010). Development of a new controlled pesticide delivery system based on neem leaf powder. J. Hazard. Mater. 177, 290–299. doi:10.1016/j.jhazmat.2009.12.031
Taemeh, M. A., Shiravandi, A., Korayem, M. A., and Daemi, H. (2020). Fabrication challenges and trends in biomedical applications of alginate electrospun nanofibers. Carbohydr. Polym. 228, 115419. doi:10.1016/j.carbpol.2019.115419
Udeinya, J., Shu, E., Quakyi, I., and Ajayi, F. (2008). An antimalarial neem leaf extract has both schizonticidal and gametocytocidal activities. Am. J. Ther. 15, 108–110. doi:10.1097/mjt.0b013e31804c6d1d
Ugoeze, K. C., Aja, P. C., Nwachukwu, N., Chinko, B. C., Egwurugwu, J. N., and Oluigbo, K. E. (2021). Evaluation of the wound healing potentials of aqueous topical creams containing aqueous extract of Azadirachta indica leaves as bioactive ingredient. J. Pharm. Pharmacol. Res. 5, 176. doi:10.26502/fjppr.041
Upadhayay, U., Vigyan, P. C., Chakrabort, S., Dhama, K., and Singh, S. V. (2014). Neem (Azadirachta indica) and its potential for safeguarding health of animals and humans: A review. J. Biol. Sci. 14, 110–123. doi:10.3923/jbs.2014.110.123
Wang, L., Khor, E., and Lim, L. Y. (2001). Chitosan–alginate–CaCl2 system for membrane coat application. J. Pharm. Sci. 90, 1134–1142. doi:10.1002/jps.1067
Wang, M., Yu, D.-G., Williams, G. R., and Bligh, S. W. A. (2022). Co-loading of inorganic nanoparticles and natural oil in the electrospun janus nanofibers for a synergetic antibacterial effect. Pharmaceutics 14, 1208. doi:10.3390/pharmaceutics14061208
Wang, W., Xue, C., and Mao, X. (2020). Chitosan: Structural modification, biological activity and application. Int. J. Biol. Macromol. 164, 4532–4546. doi:10.1016/j.ijbiomac.2020.09.042
Wu, S., Dong, T., Li, Y., Sun, M., Qi, Y., Liu, J., et al. (2022). State-of-the-art review of advanced electrospun nanofiber yarn-based textiles for biomedical applications. Appl. Mater. Today 27, 101473. doi:10.1016/j.apmt.2022.101473
Yang, Y., Chang, S., Bai, Y., Du, Y., and Yu, D.-G. (2020). Electrospun triaxial nanofibers with middle blank cellulose acetate layers for accurate dual-stage drug release. Carbohydr. Polym. 243, 116477. doi:10.1016/j.carbpol.2020.116477
Yao, H., Liu, J., Xu, S., Zhu, Z., and Xu, J. (2017). The structural modification of natural products for novel drug discovery. Expert Opin. Drug Discov. 12, 121–140. doi:10.1080/17460441.2016.1272757
Zhang, Y., Han, S., Wang, M., Liu, S., Liu, G., Meng, X., et al. (2022). Electrospun Cu-doped In2O3 hollow nanofibers with enhanced H2S gas sensing performance. J. Adv. Ceram. 11, 427–442. doi:10.1007/s40145-021-0546-2
Zhao, J., and Cui, W. (2020). Functional electrospun fibers for local therapy of cancer. Adv. Fiber Mat. 2, 229–245. doi:10.1007/s42765-020-00053-9
Zhao, K., Lu, Z.-H., Zhao, P., Kang, S.-X., Yang, Y.-Y., and Yu, D.-G. (2021). Modified tri–axial electrospun functional core–shell nanofibrous membranes for natural photodegradation of antibiotics. Chem. Eng. J. 425, 131455. doi:10.1016/j.cej.2021.131455
Keywords: electrospinning, nanofibers, sustain release, nanocarriers, biopolymers, cleaner production, biphasic release, medicated nanofibers
Citation: Hameed A, Rehman TU, Rehan ZA, Noreen R, Iqbal S, Batool S, Qayyum MA, Ahmed T and Farooq T (2022) Development of polymeric nanofibers blended with extract of neem (Azadirachta indica), for potential biomedical applications. Front. Mater. 9:1042304. doi: 10.3389/fmats.2022.1042304
Received: 12 September 2022; Accepted: 02 November 2022;
Published: 25 November 2022.
Edited by:
Zeki Candan, Biomaterials and Nanotechnology Research Group | BioNanoTeam, TurkeyReviewed by:
Deng-Guang Yu, University of Shanghai for Science and Technology, ChinaHelinando Pequeno De Oliveira, Federal University of São Francisco Valley, Brazil
Copyright © 2022 Hameed, Rehman, Rehan, Noreen, Iqbal, Batool, Qayyum, Ahmed and Farooq. This is an open-access article distributed under the terms of the Creative Commons Attribution License (CC BY). The use, distribution or reproduction in other forums is permitted, provided the original author(s) and the copyright owner(s) are credited and that the original publication in this journal is cited, in accordance with accepted academic practice. No use, distribution or reproduction is permitted which does not comply with these terms.
*Correspondence: Tahir Farooq, dGFoaXJmYXJvb3Fmc2RAZ21haWwuY29t, dGZhcm9vcWZzZEBndWNmLmVkdS5waw==