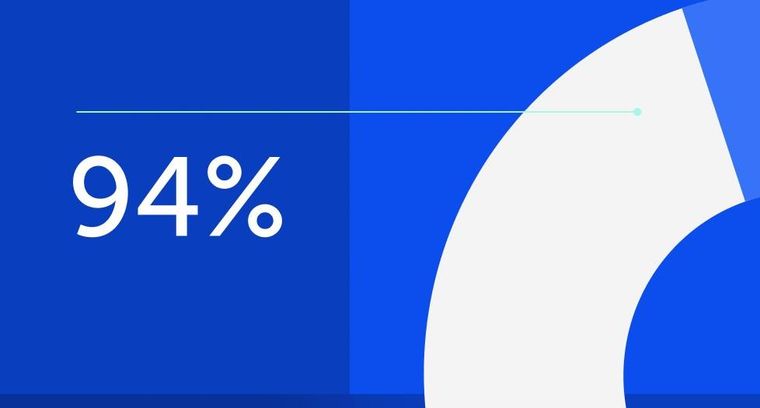
94% of researchers rate our articles as excellent or good
Learn more about the work of our research integrity team to safeguard the quality of each article we publish.
Find out more
ORIGINAL RESEARCH article
Front. Mater., 01 November 2022
Sec. Structural Materials
Volume 9 - 2022 | https://doi.org/10.3389/fmats.2022.1035551
This article is part of the Research TopicWomen in Science: Materials 2021View all 8 articles
Calcium sulfoaluminate cement is a relatively new type of cement with environmental advantages linked to its low carbon emission. However, there is a lack of knowledge on its use with supplementary cementitious materials. This article aims to study the effect of utilizing the activated flash-calcined sediment from Noyelles-sous-Lens (SC) as a supplementary cementitious material in calcium sulfoaluminate mortar. Therefore, four mixes are prepared by replacing calcium sulfoaluminate cement with 5%, 10%, and 20% of flash-calcined sediment. The reactivity of the cement pastes with and without the sediment is studied by isothermal calorimetry and by measuring the setting time. Then, the mechanical performance is tested at 1, 7, and 28 days. The results show that adding the flash-calcined sediment has an accelerator effect on cement hydration and decreases the induction period. The cumulative heat release and the compressive strength at 28 days are almost the same up to 10% of sediment substitution.
The cement industry emits more than 5% of the total man-made global CO2 emissions (Gartner and Hirao, 2015). Therefore, after the Kyoto Protocol (1997), industries were constrained to find durable solutions to reduce carbon emissions (Shi et al., 2011; Ghezloun et al., 2017; Scrivener et al., 2018). Recently, the International Conference on Climate Change (COP 25, 2019) was held in Madrid and has tried to regulate the global carbon market, climate change, global temperature increase, and water supply problems. For this purpose, several approaches have been envisaged in the construction sectors of supplementary cementitious materials (SCMs) as a substitution for ordinary Portland cement (OPC). Some solutions have been tried to use alternative fuels rather than those commonly used for material combustion in cement kilns and also the reduction of decarbonization by the replacement of some raw materials (such as calcium carbonate) by other products—already decarbonated—that confer the same chemical components. Another solution has also been considered, which consists in elaborating new types of cement, like calcium sulfoaluminate (CSA) cement, as an interesting alternative to Portland cement. CSA cement is part of the “Third Cement Series” (TCS) developed in China in the early 90s and is increasingly used in several types of applications where early high strength or shrinkage control is needed. It was also used by Kleib et al. (2018) to mitigate alkali-silica reactions in mortars. The CSA cement, also known as “green cement” is environmentally friendly due to its low-carbon footprint, and its production consumes less energy than the OPC cement (Tang et al., 2015). In fact, the clinkerization temperature of the CSA clinker is 200–300°C (Gartner and Hirao, 2015; Gartner, 2004) less than that of the Portland clinker. This calcination temperature difference represents a 25%–35% reduction in carbon dioxide emission (Hanein et al., 2018). In addition, the CSA clinker is friable, reducing the energy consumption needed for grinding compared to OPC cement, and the amount of limestone required for the CSA clinker production is lower than that for OPC clinker production, consequently reducing carbon emissions related to the calcination process (Quillin, 2001; Zhang et al., 2012; Berger et al., 2013; Su et al., 2019). The main phase in the CSA clinker is ye’elimite, also called Klein’s compound C4A3Ṧ, in addition to other secondary phases such as C2S, C4AF, and CṦ.
Several studies have been conducted to study the effects of SCM when the CSA cement is used. García-Maté et al. (2013) showed that the partial substitution of the CSA cement with fly ash (FA) has environmental and economic benefits. The authors showed that adding FA up to 15% increases mechanical performance; however, an excessive amount has a negative effect on these properties. The same result was obtained by Martin et al. (2017) who showed that the increase in the substitution by FA accelerates the hydration of the CSA cement and that adding up to 15% of FA does not have any effect on the compressive strength. The galvanic sludge has also been used by Luz et al. (2009) to substitute CSA cement by 25% in mortars. The results showed that the CSA cement hydration products encapsulate the chromium coming from the sludge (Luz et al., 2009).
Regarding the sediment, it was used as SCM with OPC cement in order to be recycled and to reduce OPC cement production (Snellings et al., 2017; Zhao et al., 2018; Van Bunderen et al., 2019). In fact, sediments are materials coming from dredged marine operations and continental watercourses and can also be defined as fine materials from the wear of continents (Wang and Gillot, 1991; Dubois et al., 2011). Several studies have already been conducted to use the sediment as SCM in OPC construction materials (Snellings et al., 2017; Amar et al., 2018; Van Bunderen et al., 2019). These studies have shown the presence of a pozzolanic effect when the sediment is added as a mineral addition in the cementitious matrix (Dang et al., 2013; Snellings et al., 2016; Amar et al., 2017; Snellings et al., 2017; Van Bunderen et al., 2019). Different methods, such as physical, chemical, biological, and thermal methods, can be used to treat sediments before using them as SCM (Amar et al., 2021).
Flash calcination is a treatment technique that has been applied in some studies for the activation of clays and to transform kaolin into metakaolin (Salvador, 1995; Salvador and Pons, 2000; Nicolas et al., 2013; Claverie et al., 2015; Inocente et al., 2021; Van Bunderen et al., 2021). Danilevich et al. (2021) have thermally activated aluminum hydroxide in gibbsite using a reactor TSEFLAR. By examining the characteristics of the final product, the study has shown that process parameters such as temperature, the residence time of the particles, and raw material consumption play a major role in activation processes. Van Bunderen et al. (2021) also studied the effect of the flash-calcined sediment on the mechanical properties and shrinkage of concretes. After testing cement replacement for 20 wt%, 30 wt%, and 40 wt%, the results demonstrated that flash-calcined sediments are suitable for the production of sustainable cement and concrete. This technique has the advantage of lowering energy consumption and gas release due to the quickness of the process, and it is applied to sediments in this study. As a matter of fact, this process includes dehydroxylation that activates the material with thermal excitation between 700 and 850°C. Activation of the clay fractions present in sediments has recently been studied (Snellings et al., 2017; Snellings et al., 2016; Scrivener et al., 2004; Teklay et al., 2016; Van Bunderen et al., 2018). These types of products are also usable as SCM with trough cement replacement (Nguyen and Castel, 2020). Amar et al. (2017) have studied the reactivity of flash-calcined sediments. By using various pozzolanic tests, it was demonstrated that the calcination process has a positive impact on the pozzolanic activity of sediments (Amar et al., 2021). Snellings et al. (2017) and Snellings et al. (2016) also used the flash calcination technique on the same sediment studied by Van Bunderen et al. (2019). This last study investigated the influence of the temperature of treatment and the final reactivity of sediments. The authors tested three calcination temperatures, 820, 865, and 905°C, and they showed the effectiveness of the process.
The use of sulfoaluminate cement is of particular interest. This cement possesses advantageous properties such as low carbon emission, fast setting, low shrinkage, and resistance to corrosion (Allevi et al., 2015; Shang et al., 2022). To help lower cement industry impact and product costs, it seems interesting to use blends of CSA and SCMs (García-Maté et al., 2013). In this approach, the benefits will come in two folds: lower CO2 emissions because of the clinker reduction, cost reduction, and alternative material reuse. Flash-calcined materials to cement may also modify blended product properties (Qian et al., 2008), such as decreased hydration heat, improved workability of mixes, and porosity (V. Ž.-C. and building Materials and undefined, 2000). A recent study conducted by Zhang et al. (Ke et al., 2021) monitored pore characteristics in CSA–SCM-blended cement in comparison to OPC. It was demonstrated that with the action of fly ash, silica fume, and slags, the pore structure of the CSA cement paste was remarkably refined. However, the replacement ratio was limited to 15% for each SCM, and waste materials such as SC were not utilized. Gastaldi et al. (2021) adopted a similar approach and studied the hydration behavior of CSA–SCM blends without calcined sediments. Furthermore, in the particular case of sediments blended with CSA, SC was shown to be of particular interest for the construction sector (Zentar et al., 2021) whereas it was not assessed for cementitious matrix utilization. First, the use of flash-calcined sediments as a substitution for the CSA cement has not been largely investigated. Also, the physicochemical properties, reactions involved, and microstructure in CSA–SC blends are not well understood. From an ecological and economic point of view, the combination of the CSA cement and sediment as SCM is advantageous. It leads to a lower cement content by replacing it with SC. Hence, on one hand, as already discussed, the production of CSA cement requires less energy than is needed in OPC manufacture. Also, on the other hand, the use of SCM reduces the quantity of the clinker to be produced.
Hence, in this article, the flash-calcined sediment from Noyelles-Sous-Lens is blended with the commercial CSA cement in order to test its effect on the hydration process and the mechanical properties of mortars.
The materials used in this study are sulfoaluminate cement (CSA)—Alpenat®—produced by VICAT® and the fluvial sediments dredged from Noyelles-sous-Lens in the north of France. The latter was dried at 100°C, then crushed, and sieved. The sediment was treated through the flash calcination method at 750°C, as described by Amar (2017).
Calcination was carried out in a flash furnace at IMT Nord-Europe in France. The flash calcination process is illustrated in Figure 1 (Amar, 2017).
Four mortar mixes were designed and produced with and without flash-calcined sediments. Formulations were made under the NF EN 196-1 standard. Mixes were prepared following a water/binder ratio of 0.5 and fine siliceous sand (NS) in a proportion of 1:3. CSA stands for the control mortar without sediment, whereas CSA5, CSA10, and CSA20 refer to mortars containing, respectively, 5%, 10%, and 20% of flash-calcined sediments. The mortar compositions are given in Table 1.
The mineralogical composition of the CSA cement and the calcined sediment was determined by X-ray diffraction (XRD) using a Bruker D2 instrument with Cu Kα radiation. The X-ray patterns were acquired in 2θ (10–80°) with a step of 0.02 and 1 s per step.
The chemical composition of the CSA cement and the flash-calcined sediment SC was determined by X-ray fluorescence (XRF) using a Bruker S4 Pioneer spectrometer.
The particle size distributions of the materials (cement, sediment, and sand) were determined using a Coulter laser granulometry type LS 13 320.
The heat flow was measured using an isothermal calorimeter at 20°C to study the reactivity of the cement paste with a water/cement ratio of 0.5. The calorimeter used is a homemade calorimeter equipped with flowmeters, allowing the calorimeter to equilibrate in less than 5 min.
The initial setting time of the cement pastes was determined according to the standard NF EN 196-3 and using the VICATRONIC I06 091 device.
The compressive strength of mortars was determined according to the standard AFNOR NF EN 196-1 on prismatic samples 40*40*160 mm. The mechanical properties were measured at 1, 7, and 28 days using the INSTRON 5500 R apparatus.
The porosity was also investigated on specimens at 28 days with the mercury porosimeter MicroActive AutoPore V9600 2.00.
The chemical and mineralogical analyses consist in determining the presence and the proportion of each chemical element and the mineralogical phases by XRF and XRD, respectively. The results show that the CSA cement contains ye’elimite, belite, anhydrite, and perovskite as major phases (Figure 2). Figure 3 shows the mineral composition of the raw sediment RS (before treatment) and SC sediment (after flash calcination). The main mineral phases identified are quartz, calcite, albite, and mica for RS and SC. The main difference is in the amorphous phases generated, as demonstrated earlier by Amar et al. (2017).
Table 2 presents the chemical composition of the CSA cement and SC. The main oxides in both materials are CaO, Al2O3, SiO2, and Fe2O3 in addition to SO3 in the CSA cement.
TABLE 2. Concentrations of the major oxide elements for the CSA cement Alpenat from VICAT and the flash-calcined sediment.
The physical properties of materials are shown in Table 3. The results show that the SC is a fine material, and this could impact the activity and water demand of the material.
The particle size distribution determined by laser granulometry shows that the CSA cement and SC sediment have approximately the same D50 (7.23 and 7.22 μm for CSA and SC, respectively). Therefore, partial substitution of cement by the flash-calcined sediment is possible. The particle size distribution is given in Figure 4.
The reactivity of cement pastes, with and without the sediment, was followed by isothermal calorimetry at 20°C. The hydration of the cement paste is an exothermic process; therefore, the heat released can be correlated with the kinetics of cement hydration.
Figure 5 presents the heat flow released and cumulative heat released for the four cement pastes: CSA, CSA5, CSA10, and CSA20. The results show that the calorimetric curves for cement pastes with and without flash-calcined sediments have the same shape. Three peaks are identified. The first peak is formed after the cement’s contact with water and the dissolution of the anhydrous grains within the first few minutes of hydration. After the first maximum, an induction period with low thermal activity is observed. Then, a second peak is formed for all of the mixes and corresponds to the reaction between ye’elimite and the sulfates coming from the anhydrite/gypsum. This reaction gives ettringite C3A.3CŠ.H32 as the main hydration product. Then, after depletion of the sulfate sources, a third peak is formed and the decomposition of ettringite will occur, leading to the production of the monosulfoaluminate hydrate. The same hydration mechanisms and isothermal curves were obtained in the literature, indicating the formation of three peaks during CSA cement paste hydration (Winnefeld and Barlag, 2009; Chen and Juenger, 2011; Wang et al., 2022; Žibret et al., 2022).
FIGURE 5. Heat flow and cumulative heat released for the four cement pastes: CSA, CSA5, CSA10, and CSA20.
When comparing the heat released from the CSA cement paste (without the SC sediment) to cement pastes with sediments (CSA5, CSA10, and CSA10), it can be seen that the peaks are shifted to the left when adding the flash-calcined sediment in the mortar as supplementary cementitious materials, indicating that the reactions are accelerated. This acceleration increases with the increase in the sediment amount in the cement paste. The initial setting time for CSA begins after 3 h of hydration, whereas for CSA5, CSA10, and CSA20, it begins after 2 h 45 min, 2 h 30 min, and 2 h 15 min of hydration, respectively.
Concerning the cumulative heat released from the cement pastes, the results show that at the beginning of the hydration process and until approximately 8 h of hydration, the cumulative heat released increases with the amount of the flash-calcined sediment in the mortar. However, after 24 h of hydration, the cumulative heat released from the CSA cement paste is the highest, and the CSA20 has the lowest cumulative heat released.
Different parameters could influence the heat release of the CSA cement according to the literature. Burris and Kurtis (2022) showed that the water/cement ratio (W/C) and the cement composition (anhydrite/ye’elimite content in the cement) have an influence on the hydration mechanisms (retarder or accelerator effect depending on the ye’elimite content in the clinker).
In our case, the acceleration effect and the reduction in the induction period when adding the flash-calcined sediment to the cement paste could be related to the additional quantity of anhydrite brought by the flash-calcined sediment. In fact, as it can be seen from the mineralogical analysis (Figure 2) of the sediment before and after treatment, flash calcination leads to the formation of anhydrite in the flash-calcined sediment compared to the raw sediment (RS) before treatment. Similar results were established in a previous study conducted by Chu et al. (2022). Therefore, more sulfates are available to react with ye’elimite when adding more SC to the cement paste, and the hydration mechanisms are accelerated. Concerning the cumulative heat release, in the first 8 h, the cement paste containing the sediment released more heat than the CSA cement paste due to the acceleration of ye’elimite hydration. However, after 24 h, the total heat released is lower for the sediment-based cement paste, especially for CSA20, due to the dilution effect. Therefore, there is less ye’elimite in the mixture to react.
The setting time results are presented in Figure 6. The initial setting time is determined when the needle penetrates the mixture to a depth of 34 ± 1 mm.
The results show that the initial setting time is reached faster when the mortar contains the flash-calcined sediment. This time decreases with the increase in sediment substitution in the cement paste. The same behavior is observed for the final setting time. The latter is shorter when adding the sediment to the cement paste.
As for the calorimetric study, many factors could influence the setting time of the cement paste, such as the temperature, W/C ratio, and cement composition (Burris and Kurtis, 2022).
Therefore, the faster setting time with sediment cement pastes could be due to the additional anhydrite brought by the flash-calcined sediment as CSA reactions rely on anhydrite availability.
The compressive strength results at 1, 7, and 28 days are presented in Figure 7 for the different mix designs.
The results show that up to 10% substitution with SC, the compressive strength of mortars is slightly higher than the reference mortar CSA without SC, especially at 1 and 7 days. At 28 days, the three mortars (CSA, CSA5, and CSA10) present almost the same compressive strength of around 34 MPa, whereas for the mix with 20% of SC substitution, the compressive strength is the lowest compared to the three other mortars and at all testing times. In addition, in 1 day, almost 80% of the compressive strength for all of the mix designs is reached.
The decrease in the compressive strength for CSA20 could be related, on one hand, to the dilution effect, which is dependent on the high cement substitution by the sediment. Therefore, the ye’elimite amount decreases considerably in the mix, and consequently, the ettringite formation decreases. These low properties are also related to the high water demand of the sediment, which increases porosity during hardening. The mechanical strength results confirm the results obtained by isothermal calorimetry where CSA20 exhibits the lowest cumulative heat released (see Section 3.2) and the lowest compressive strength. The total porosity of the mortars was measured after 28 days of hydration (Table 4). The results show that CSA20 has the highest porosity among the four designed mortars. As porosity is correlated with resistance, mortars with lower porosity present better compressive strength (CSA, CSA5, and CSA10, respectively).
TABLE 4. Mercury porosity, average pore diameter, and total pore area on the mortar tested at 28 days.
Hence, it could be considered that the presence of the calcined sediment has no significant negative effect on matrix properties for a substitution rate below 10%.
The pozzolanic activity of the formulated mortars was studied using the mechanical activity index
The mineralogical and chemical composition of the sediment (siliceous, clayey, and limestone) can be the reason why it can be used as a suitable supplementary cementitious admixture. In multiple cases, an activation process similar to that applied to clay (Gastaldini et al., 2015) must be used to enhance the sediment’s properties. Sediments have also been shown to be effective as a potential mineral addition in concretes and mortars (Chen et al., 2010; ANGER et al., 2014; Bouamrane et al., 2014). The results presented in Table 5 and Figure 8 provide evidence that processes are different and SC has a low pozzolanic activity at an early age. However, CSA5 at 28 days has a better activity (
In addition, multiple parameters such as fineness, heat treatment temperature, and calcination time may also impact directly and control the final product efficiency and pozzolanic activity (Gastaldini et al., 2015; Walker and Pavía, 2011; Zhang, 2013; Lynn et al., 2015). Therefore, a better investigation of these could be interesting and helpful in understanding the involved physicochemical processes.
Investigations have shown the benefits of the use of the flash-calcined sediment in CSA blends. The presence of SC generates an acceleration of reactions involved during hydration. This effect is attributed to the anhydrite identified in the XRD analysis. The same reason also impacts the setting. The compressive strength test demonstrated that below 10% replacement of CSA by SC, no negative effect has been identified as the mercury porosity test has also shown a similar pore structure between CSA and CSA10.
This work aims to study the effect of the substitution of the calcium sulfoaluminate cement by the flash-calcined sediment from Noyelles-sous-Lens in France. The main conclusions that can be drawn throughout this work are the following.
1) Isothermal calorimetry shows that adding the flash-calcined sediment has an acceleration effect on cement paste hydration. The presence of anhydrite in the sediment after treatment could be the reason for this acceleration. This result is consistent with the setting time test.
2) The cumulative heat release after 24 h of hydration is the same for cement pastes containing up to 10% of flash-calcined sediments in the mixture. Adding 20% sediment to the cement paste decreases the total heat released at 24 h, and this could be due to the dilution effect and the decrease in the ye’elimite content and consequently in ettringite formation.
3) The compressive strength results show, as for the calorimetry, that adding up to 10% of the sediment in the mortar does not influence the mechanical performances at 28 days.
This study shows that the flash-calcined sediment could be recycled, up to 10%, as supplementary cementitious materials in a CSA cement matrix without affecting the performance of the cementitious material. It would be interesting to continue this study in order to evaluate the effect of this substitution on the durability of the cement matrix when incorporating sediments.
The raw data supporting the conclusion of this article will be made available by the authors, without undue reservation.
JK, MA, MB, and N-EA contributed to the methodology and reviewing and editing of the study. JK and MA contributed to the investigation of the study and to the experimental program. JK and MA wrote the first draft of the manuscript. All authors contributed to the manuscript revision and read and approved the submitted version.
The authors declare that the research was conducted in the absence of any commercial or financial relationships that could be construed as a potential conflict of interest.
All claims expressed in this article are solely those of the authors and do not necessarily represent those of their affiliated organizations, or those of the publisher, the editors, and the reviewers. Any product that may be evaluated in this article, or claim that may be made by its manufacturer, is not guaranteed or endorsed by the publisher.
Allevi, S., Marchi, M., Scotti, F., Bertini, S., and Cosentino, C. (2015). Hydration of calcium sulphoaluminate clinker with additions of different calcium sulphate sources. Mat. Struct. 49149 (1), 453–466. doi:10.1617/S11527-014-0510-5
Amar, M., Benzerzour, M., Abriak, N. E., and Mamindy-Pajany, Y. (2017). Study of the pozzolanic activity of a dredged sediment from Dunkirk harbour. Powder Technol. 320, 748–764. doi:10.1016/j.powtec.2017.07.055
Amar, M., Benzerzour, M., and Abriak, N. E. N.-E. (2018). Towards the establishment of formulation laws for sediment-based mortars. J. Build. Eng. 16, 106–117. doi:10.1016/j.jobe.2017.12.011
Amar, M., Benzerzour, M., Kleib, J., and Abriak, N. E. N.-E. (2021). From dredged sediment to supplementary cementitious material: Characterization, treatment, and reuse. Int. J. Sediment Res. 36 (1), 92–109. doi:10.1016/j.ijsrc.2020.06.002
Amar, M. (2017). Étude expérimentale et numérique de la valorisaion des sédiments de dragage dans les matrices cimentaires - thèse de doctorat. Lille: IMT Lille-Douai.
Anger, B., Moulin, I., Perin, E., Thery, F., and Levacher, D. (2014). Utilisation de sédiments fins de barrage dans la fabrication de mortiers. Dunkerque: XIIIèmes JNGCGC, 953–960. doi:10.5150/jngcgc.2014.105
Berger, S., Aouad, G., Dit, C. C., Le Bescop, P., and Damidot, D. (2013). Leaching of calcium sulfoaluminate cement pastes by water at regulated pH and temperature : Experimental investigation and modeling. Cem. Concr. Res. 53, 211–220. doi:10.1016/j.cemconres.2013.06.014
Bouamrane, A., Elouazzani, D. C., Barna, L. T., and Mansouri, K., “Valorisation des boues de papeterie comme matières premières secondaires dans les mortiers de ciment Portland., ” jmaterenvironsci.Com., vol. 5, no. 2, 605–614. 2014.
Burris, L. E., and Kurtis, K. E. (2022). Water-to-cement ratio of calcium sulfoaluminate belite cements: Hydration, setting time, and strength development. Cement 8, 100032. doi:10.1016/j.cement.2022.100032
Chen, H., Ma, X., and Dai, H. (2010). Reuse of water purification sludge as raw material in cement production. Cem. Concr. Compos. 32 (6), 436–439. doi:10.1016/j.cemconcomp.2010.02.009
Chen, I. A., and Juenger, M. C. G. (2011). Synthesis and hydration of calcium sulfoaluminate-belite cements with varied phase compositions. J. Mat. Sci. 46 (8), 2568–2577. doi:10.1007/s10853-010-5109-9
Chu, D. C., Amar, M., Kleib, J., Benzerzour, M., Betrancourt, D., Abriak, N. E., et al. (2022). The pozzolanic activity of sediments treated by the flash calcination method. Waste Biomass Valorization 3, 1–20. doi:10.1007/s12649-022-01789-8
Claverie, M., Martin, F., Tardy, J., Cyr, M., De Parseval, P., Grauby, O., et al. (2015). Structural and chemical changes in kaolinite caused by flash calcination: Formation of spherical particles. Appl. Clay Sci. 114, 247–255. doi:10.1016/j.clay.2015.05.031
Dang, T. A., Kamali-Bernard, S., and Prince, W. A. (2013). Design of new blended cement based on marine dredged sediment. Constr. Build. Mat. 41, 602–611. doi:10.1016/j.conbuildmat.2012.11.088
Danilevich, V., Isupova, L., and Parmon, V. (2021). The process for preparation of active aluminum hydroxyoxide via flash calcination of gibbsite in a new energy-efficient centrifugal drum-type reactor. Clean. Eng. Technol. 3, 100118. doi:10.1016/J.CLET.2021.100118
De Larrard, F. (2000). Structures granulaires et formulation des bétons. Etudes Rech. Des. Lab. Des. ponts chaussées OA 34, 414. [Online]. Available: http://www.concretonline.com/pdf/00hormigon/art_tec/docu_ref01.pdf.
Dubois, V., Zentar, R., Abriak, N. E., and Grégoire, P. (2011). Fine sediments as a granular source for civil engineering. Eur. J. Environ. Civ. Eng. 15 (2), 137–166. doi:10.1080/19648189.2011.9693315
García-Maté, M., De La Torre, A. G., León-Reina, L., Aranda, M. A. G. G., and Santacruz, I. (2013). Hydration studies of calcium sulfoaluminate cements blended with fly ash. Cem. Concr. Res. 54, 12–20. doi:10.1016/j.cemconres.2013.07.010
Gartner, E., and Hirao, H. (2015). A review of alternative approaches to the reduction of CO2 emissions associated with the manufacture of the binder phase in concrete. Cem. Concr. Res. 78, 126–142. 10.1016/j.cemconres.2015.04.012 Accessed: Jul. 24, 2019. [Online]. Available: https://www.sciencedirect.com/science/article/pii/S0008884615001180#f0015.
Gartner, E. (2004). Industrially interesting approaches to ‘low-CO2’ cements. Cem. Concr. Res. 34 (9), 1489–1498. doi:10.1016/j.cemconres.2004.01.021
Gastaldi, D., Bertola, F., Irico, S., Paul, G., and Canonico, F. (2021). Hydration behavior of cements with reduced clinker factor in mixture with sulfoaluminate binder. Cem. Concr. Res. 139, 106261. doi:10.1016/j.cemconres.2020.106261
Gastaldini, A. L. G., Hengen, M. F., Gastaldini, M. C. C., do Amaral, F. D., Antolini, M. B., and Coletto, T. (2015). The use of water treatment plant sludge ash as a mineral addition. Constr. Build. Mat. 94, 513–520. doi:10.1016/j.conbuildmat.2015.07.038
Ghezloun, A., Saidane, A., and Merabet, H. (2017). The COP 22 new commitments in support of the paris agreement. Energy Procedia 119, 10–16. doi:10.1016/j.egypro.2017.07.040
Hanein, T., Galvez-Martos, J. L., and Bannerman, M. N. (2018). Carbon footprint of calcium sulfoaluminate clinker production. J. Clean. Prod. 172, 2278–2287. doi:10.1016/j.jclepro.2017.11.183
Inocente, J. M., Elyseu, F., Jaramillo Nieves, L. J., Jiusti, J., Cargnin, M., and Peterson, M. (2021). Production and characterization of high-reactivity metakaolins calcined in flash reactor. Appl. Clay Sci. 213, 106247. doi:10.1016/j.clay.2021.106247
Ke, G., Zhang, J., Liu, Y., and Xie, S. (2021). Pore characteristics of calcium sulfoaluminate cement paste with impact of supplementary cementitious materials and water to binder ratio. Powder Technol. 387, 146–155. doi:10.1016/J.POWTEC.2021.04.027
Kleib, J., Aouad, G., Louis, G., Zakhour, M., Boulos, M., Rousselet, A., et al. (2018). The use of calcium sulfoaluminate cement to mitigate the alkali silica reaction in mortars. Constr. Build. Mat. 184, 295–303. doi:10.1016/J.CONBUILDMAT.2018.06.215
Luz, C. A., Rocha, J. C., Cheriaf, M., and Pera, J. (2009). Valorization of galvanic sludge in sulfoaluminate cement. Constr. Build. Mat. 23 (2), 595–601. doi:10.1016/j.conbuildmat.2008.04.004
Lynn, C. J. C. J., Dhir, R. K., Ghataora, G. S., and West, R. P. (2015). Sewage sludge ash characteristics and potential for use in concrete. Constr. Build. Mat. 98, 767–779. doi:10.1016/j.conbuildmat.2015.08.122
Martin, L. H. J., Winnefeld, F., Tschopp, E., Müller, C. J., and Lothenbach, B. (2017). Influence of fly ash on the hydration of calcium sulfoaluminate cement. Cem. Concr. Res. 95, 152–163. doi:10.1016/j.cemconres.2017.02.030
Nguyen, Q. D., and Castel, A. (2020). Reinforcement corrosion in limestone flash calcined clay cement-based concrete. Cem. Concr. Res. 132, 106051. doi:10.1016/j.cemconres.2020.106051
Nicolas, R. S., Cyr, M., and Escadeillas, G. (2013). Characteristics and applications of flash metakaolins. Appl. Clay Sci. 83 (84), 253–262. doi:10.1016/j.clay.2013.08.036
Qian, G., Shi, J., Cao, Y., and Xu, Y.P. C.-J. of hazardous materials, and undefined (2008). Properties of MSW fly ash–calcium sulfoaluminate cement matrix and stabilization/solidification on heavy metals. Amsterdam, Netherlands: Elsevier. Accessed: Oct. 13, 2022. [Online]. Available: https://www.sciencedirect.com/science/article/pii/S030438940700965X.
Quillin, K. (2001). Performance of belite – sulfoaluminate cements. Cem. Concr. Res. 31, 1341–1349. doi:10.1016/s0008-8846(01)00543-9
Rozière, E., Samara, M., Loukili, A., and Damidot, D. (2015). Valorisation of sediments in self-consolidating concrete: Mix-design and microstructure. Constr. Build. Mat. 81, 1–10. doi:10.1016/j.conbuildmat.2015.01.080
Salvador, S., and Pons, O. (2000). A semi-mobile flash dryer/calciner unit to manufacture pozzolana from raw clay soils — Application to soil stabilisation. Constr. Build. Mat. 14 (2), 109–117. doi:10.1016/S0950-0618(00)00005-2
Salvador, S. (1995). Pozzolanic properties of flash-calcined kaolinite: A comparative study with soak-calcined products. Cem. Concr. Res. 25 (1), 102–112. doi:10.1016/0008-8846(94)00118-I
Scrivener, K. L., Crumbie, A. K., and Laugesen, P. (2004). The interfacial transition zone (ITZ) between cement paste and aggregate in concrete. Interface Sci. 12 (4), 411–421. doi:10.1023/B:INTS.0000042339.92990.4c
Scrivener, K. L., John, V. M., and Gartner, E. M. (2018). Eco-efficient cements: Potential economically viable solutions for a low-CO 2 cement-based materials industry. Cem. Concr. Res. 114, 2–26. doi:10.1016/j.cemconres.2018.03.015
Shang, C., Wu, C., Wang, J., Lu, L., Fu, Q., Zhang, Y., et al. (2022). Investigating mechanical properties and microstructure of reactive powder concrete blended with sulfoaluminate cement. Case Stud. Constr. Mater. 17, e01552. doi:10.1016/J.CSCM.2022.E01552
Shi, C., Jiménez, A. F., and Palomo, A. (2011). New cements for the 21st century: The pursuit of an alternative to Portland cement. Cem. Concr. Res. 41 (7), 750–763. doi:10.1016/j.cemconres.2011.03.016
Snellings, R., Cizer, O., Horckmans, L., Durdzinski, P. T., Dierckx, P., Nielsen, P., et al. (2016). Properties and pozzolanic reactivity of flash calcined dredging sediments. Appl. Clay Sci. 129, 35–39. doi:10.1016/j.clay.2016.04.019
Snellings, R., Horckmans, L., Van Bunderen, C., Vandewalle, L., and Cizer, Ö. (2017). Flash-calcined dredging sediment blended cements: Effect on cement hydration and properties. Mat. Struct. 50 (6), 241. doi:10.1617/s11527-017-1108-5
Su, T., Kong, X., Tian, H., and Wang, D. (2019). Effects of comb-like PCE and linear copolymers on workability and early hydration of a calcium sulfoaluminate belite cement. Cem. Concr. Res. 123, 105801. doi:10.1016/j.cemconres.2019.105801
Tambara, L. U. D., Cheriaf, M., Rocha, J. C., Palomo, A., and Fernández-Jiménez, A. (2020). Effect of alkalis content on calcium sulfoaluminate (CSA) cement hydration. Cem. Concr. Res. 128, 105953. doi:10.1016/j.cemconres.2019.105953
Tang, S. W., Zhu, H. G., Li, Z. J., Chen, E., and Shao, H. Y. (2015). Hydration stage identification and phase transformation of calcium sulfoaluminate cement at early age. Constr. Build. Mat. 75, 11–18. doi:10.1016/j.conbuildmat.2014.11.006
Teklay, A., Yin, C., and Rosendahl, L. (2016). Flash calcination of kaolinite rich clay and impact of process conditions on the quality of the calcines: A way to reduce CO2footprint from cement industry. Appl. Energy 162, 1218–1224. doi:10.1016/j.apenergy.2015.04.127
Van Bunderen, C., Benboudjema, F., Snellings, R., Vandewalle, L., and Cizer, O. (2021). Experimental analysis and modelling of mechanical properties and shrinkage of concrete recycling flash calcined dredging sediments. Cem. Concr. Compos. 115, 103787. doi:10.1016/j.cemconcomp.2020.103787
Van Bunderen, C., et al. (2018). “Concrete with flash-calcined dredging sediments as a novel supplementary cementitious material,” in High tech concrete: Where technology and engineering meet (Cham: Springer International Publishing), 109–116.
Van Bunderen, C., Snellings, R., Vandewalle, L., and Cizer, Ö. (2019). Early-age hydration and autogenous deformation of cement paste containing flash calcined dredging sediments. Constr. Build. Mat. 200, 104–115. doi:10.1016/j.conbuildmat.2018.12.090
V. Ž.-C. and building Materials and undefined (2000). Properties of blended sulfoaluminate belite cement. Amsterdam, Netherlands: Elsevier. Accessed: Oct. 13, 2022. [Online]. Available: https://www.sciencedirect.com/science/article/pii/S0950061800000507.
Walker, R., and Pavía, S. (2011). Physical properties and reactivity of pozzolans, and their influence on the properties of lime–pozzolan pastes. Mat. Struct. 44 (6), 1139–1150. doi:10.1617/s11527-010-9689-2
Wang, H., and Gillot, J. E. (1991). Mechanism of alkali –silica reaction and significance of calcium hydroxide. Cem. Concr. Res. 21, 647–654.
Wang, X., Guo, M. Z., Yue, G., Li, Q., and Ling, T. C. (2022). Synthesis of high belite sulfoaluminate cement with high volume of mixed solid wastes. Cem. Concr. Res. 158, 106845. doi:10.1016/j.cemconres.2022.106845
Winnefeld, F., and Barlag, S. (2009). Influence of calcium sulfate and calcium hydroxide on the hydration of calcium sulfoaluminate clinker. ZKG Int. 62 (12), 42–53.
Zentar, R., Wang, H., and Wang, D. (2021). Comparative study of stabilization/solidification of dredged sediments with ordinary Portland cement and calcium sulfo-aluminate cement in the framework of valorization in road construction material. Constr. Build. Mat. 279, 122447. doi:10.1016/J.CONBUILDMAT.2021.122447
Zhang, L. (2013). Production of bricks from waste materials – a review. Constr. Build. Mat. 47, 643–655. doi:10.1016/j.conbuildmat.2013.05.043
Zhang, X., Zhao, M., and Zhang, Y. (2012). Preparation and properties of self-pulverizing calcium sulfoaluminate cement. Constr. Build. Mat. 34, 107–113. doi:10.1016/j.conbuildmat.2012.02.006
Zhao, Z., Benzerzour, M., Abriak, N.-E. E., Damidot, D., Courard, L., and Wang, D. (2018). Use of uncontaminated marine sediments in mortar and concrete by partial substitution of cement. Cem. Concr. Compos. 93, 155–162. doi:10.1016/j.cemconcomp.2018.07.010
Keywords: calcium sulfoaluminate cement, sediment, mortar, recycling, flash calcination
Citation: Kleib J, Amar M, Benzerzour M and Abriak N-E (2022) Effect of flash-calcined sediment substitution in sulfoaluminate cement mortar. Front. Mater. 9:1035551. doi: 10.3389/fmats.2022.1035551
Received: 02 September 2022; Accepted: 13 October 2022;
Published: 01 November 2022.
Edited by:
Ya Wang, Texas A&M University, United StatesReviewed by:
Peng Zhang, National Natural Science Foundation of China, ChinaCopyright © 2022 Kleib, Amar, Benzerzour and Abriak. This is an open-access article distributed under the terms of the Creative Commons Attribution License (CC BY). The use, distribution or reproduction in other forums is permitted, provided the original author(s) and the copyright owner(s) are credited and that the original publication in this journal is cited, in accordance with accepted academic practice. No use, distribution or reproduction is permitted which does not comply with these terms.
*Correspondence: Joelle Kleib, am9lbGxlLmtsZWliQGltdC1ub3JkLWV1cm9wZS5mcg==
†These authors have contributed equally to this work
Disclaimer: All claims expressed in this article are solely those of the authors and do not necessarily represent those of their affiliated organizations, or those of the publisher, the editors and the reviewers. Any product that may be evaluated in this article or claim that may be made by its manufacturer is not guaranteed or endorsed by the publisher.
Research integrity at Frontiers
Learn more about the work of our research integrity team to safeguard the quality of each article we publish.