- Shanxi Province Key Laboratory of Oral Diseases Prevention and New Materials, Shanxi Medical University School and Hospital of Stomatology, Taiyuan, China
With the deepening of research on condensed matter chemistry, artificially guided demineralized dentin remineralization has changed from a classical remineralization pathway of the thermodynamic deposition mode to a biomimetic mineralization mode. This new mode is more consistent with the biological mineralization process. The biomimetic mineralization model can successfully simulate natural mineralization and restore the microstructure and mechanical properties of demineralized dentin. Therefore, it has a good application value in the treatment of caries and dentin hypersensitivity and adhesive restorations. This paper analyzes the principles of guided tissue remineralization and describes new research findings related to the classical mineralization model and the novel biomaterials developed using the biomimetic mineralization mode in detail. It also describes the application of these principles to improve the dentin bonding system. It thus shares the new findings in guided tissue remineralization applied to dentin bonding systems. Finally, the existing problems in this field and future development directions are proposed.
1 Introduction
Bonded restorative techniques are becoming increasingly crucial for restoring the morphology and function of dentition with the development of minimally invasive and aesthetic concepts in the dental discipline. Clinically, dental defect restoration is mainly accomplished through bonding techniques (Chen et al., 2021).
Enamel bonding with a low-viscosity bonding resin forms a reticular structure on the enamel surface after acid etching, which is the main factor for achieving micromechanical retention. At the same time, adhesive monomers form complexes with Ca2+ in the enamel to further improve the bond strength (Bernales Sender et al., 2020). However, the dentin produces a network of demineralized collagen fibrils after clinical acid etching, altering its structure because of its inherently non-homogeneous structure (Breschi et al., 2018). In addition, collagen fibrils exposed during the aging of the dentin bonding interface for various reasons are weak structures. These weak structures compromise the stability of the bonding interface (Xu et al., 2021). This makes dentin bonding a challenge even today.
The main components of biological hard tissues, such as teeth, constitute organic-inorganic composite multilayered structural materials made of mineral crystals in an ordered configuration, considered a special class of condensed biological matter. With the understanding of condensed matter, many researchers have designed and developed many organic-inorganic composite biomimetic materials similar to natural substances. Like natural substances, the developed biomimetic materials have multi-level ordered layered structures, controlled morphology, and excellent properties. Thus, they have good prospects for application as medical restorative materials (Sang et al., 2020). Some scholars have recently attempted to use the dentin remineralization technique to form mineral deposits in the gaps within and between the demineralized dentin collagen fibrils so that the exposed collagen is re-encapsulated by mineral deposits. This re-encapsulation protects the dentin collagen fibrils and improves the stability of the adhesive interface (Liang et al., 2018a). Some experiments have demonstrated that inducing remineralization of exposed collagen fibrils in a hybrid layer improves the durability of resin–dentin bonding (Toledano et al., 2021). The proposed method of dentin remineralization and related studies have provided a crucial experimental basis for enhancing the durability of resin–dentin bonding. This paper reviews novel findings in recent years concerning the effect of guided tissue remineralization and the promotion of dentin bonding.
2 Mineralization of dentin
Dentin remineralization is clinically important for treating dentin caries and dentin hypersensitivity and increasing the durability of the dentin–resin material bond. Three basic conditions must be met for dentin remineralization. First, an intact collagen fibril structure must be present as a scaffold for mineral crystal growth (Zhou et al., 2020). Second, residual mineral crystals in the growth center or, at least in the case of complete demineralization, a newly formed nucleation center must be present (Xiang et al., 2021). Third, a mineral source containing calcium and phosphorus must be provided (Dai et al., 2021). The complete spatial relationship between minerals, scaffolds, and the soluble matrix is essential for achieving the ultrastructure of the biomineral matrix required for restoring the demineralized dentin, which is the main challenge for successful restoration (He et al., 2019).
Most early studies on dentin remineralization were based on the classical crystallization pathway. This pathway involves the large-scale deposition of calcium phosphate (CaP) crystals in collagen in a liquid environment containing mineral ions (Wu et al., 2020). However, their results were poor because they did not mimic the hydroxyapatite (HAP) arrangement in natural dental collagen fibrils and were not suitable for clinical applications. Dentin biomimetic remineralization has become a popular research topic. This is because of the advances in the study of collagen and non-collagenous proteins (NCPs) and a shift toward exploring processes involving intrafibrillar remineralization that are more similar to natural tooth formation (Amornkitbamrung et al., 2022).
2.1 “Top-down” remineralization approach of dentin
Conventional remineralization methods rely on calcium supplementation and deposition of large crystals by phosphate ions on the collagen surface (Figure 1), based on the classical theory of ion-mediated crystallization (Tao et al., 2019).
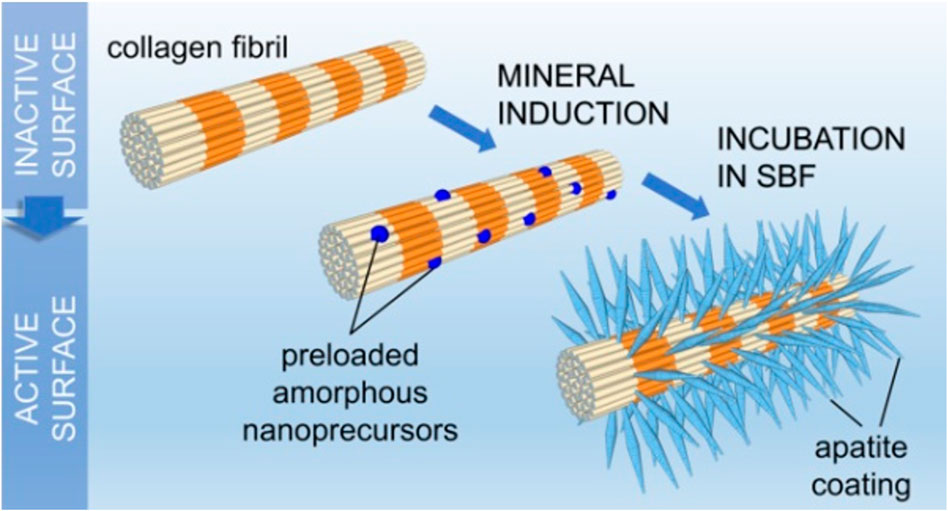
FIGURE 1. Process of extrafibrous mineralization in the simulated body fluid (Bim-Junior et al., 2021).
Cao and Li (2016) successfully formed a dense remineralized layer consisting of well-defined hexagonal nanorod crystals with a diameter of approximately 100 nm on the demineralized dentin surface. The remineralized layer was formed using agar gels rich in Ca2+, F−, and PO43−. Other researchers have used resins pre-loaded with fluoride (Saxena et al., 2018; Reise et al., 2021) or bonding agents loaded with a bioactive glass (BAG) (Daneshpoor and Pishevar, 2020; Saffarpour et al., 2017) to promote the remineralization of bare collagen. The use of pre-loaded resins or bonding agents also enhanced the resistance of the bonding interface to secondary caries. Dickens et al. (2003) used a new resin-based Ca-PO4 cement composed of tetracalcium and dicalcium phosphate and carboxylated monomers and other monomers as a restoration support base or lining material to remineralize diseased dental hard tissues. Minimally invasive high-intensity focused ultrasound has recently been used to enhance dentin remineralization. In this technique, high-amplitude ultrasound energy is focused on the overlying tissue by using a transducer. This resulted in high tension waves that produce changes through bubble clouds to enhance the transfer and interaction of calcium and phosphorus ion donors within the demineralized dentin matrix to remineralize dentin collagen fibrils (Daood and Fawzy, 2020).
However, this conventional remineralization approach focuses only on the supplementation of mineralized substrates to facilitate crystal deposition. It does not recognize the vital role of NCPs in regulating the deposition rate and orientation of nascent mineral crystals. Liu et al(2011) demonstrated that “top-down” mineralization occurs not by spontaneous nucleation of minerals on the organic matrix but by epitaxial growth on the existing seed lattice. The orientation of these mineral lattices is determined by the lattice of the seed microcrystals (Iijima et al., 2019). Similarly, Lima’s group (de Lima et al., 2020) immersed normal dentin discs and dentin discs demineralized with 37% phosphoric acid in a remineralizing solution (pH 7.6) enriched with calcium and phosphorus ions for 10 days at 37°C and observed through scanning electron microscopy (SEM). The SEM images showed a newly formed remineralized layer on the entire surface of the intact dentin, covering the originally exposed dentinal tubules. By contrast, the demineralized dentin discs exhibited almost no new mineral deposition on the dentin surface and the lumen of the tubules. They explained this finding based on the classical theory of remineralization that a pre-existing seed lattice can be used as a nucleation site to form new crystals (Aprillia et al., 2021). The surface cannot trigger remineralization of collagenous fibrils even in a supersaturated solution if no pre-existing crystal seeds are available.
Mineralization occurs mainly on scaffold substrates containing more seeded microcrystals. This means the mineral distribution of the demineralized surface layer influences the characteristics of subsequent mineralization, including the location and density of mineral deposition (El Gezawi et al., 2019). Furthermore, if the seed lattice is lacking or negligible, remineralization does not occur (Dai et al., 2011). The dentin mineralized in this manner is entirely different from the natural dentin in mechanical properties and the microstructure. Therefore, methods to achieve natural-like mineralization on fully demineralized dentin must be identified.
2.2 “Bottom-up” biomimetic remineralization approach of dentin
Biomineral condensed materials do not directly complete the final structure; their growth process involves, for example, a transformation between liquid to solid or amorphous to condensed crystalline states (Mao et al., 2020). With the continuous research on the dentin remineralization theory, the dentin biomimetic remineralization technique is gradually becoming a widely followed research direction. This is because of its advantages, such as controlled deposition of amorphous precursors on demineralized dentin collagen fibrils to form ordered apatite crystal structures and the absence of the need to rely on the remaining seed crystals on the dentin collagen fibrils as a starting point (Figure 2).
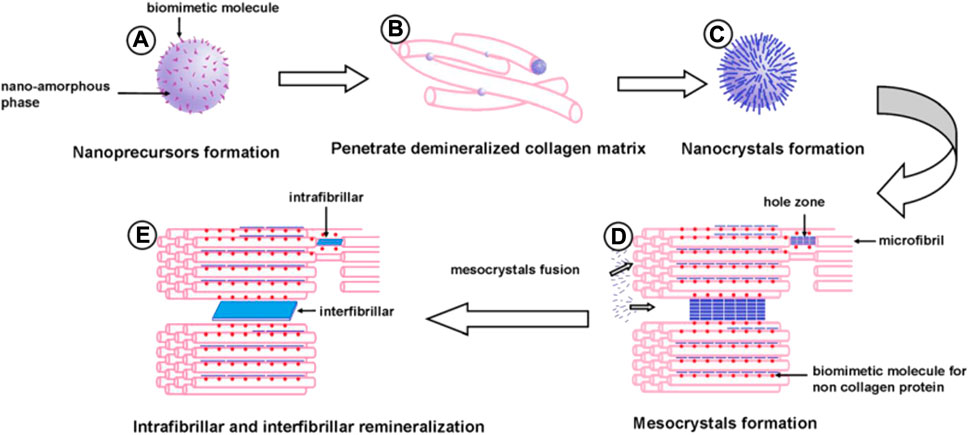
FIGURE 2. Schematic illustration of dentine bioremineralization: (A) metastable amorphous calcium phosphate nanoprecursors with biomimetic moleculars (BM1) formation; (B) nanoprecursors penetrate into a demineralized collagen matrix; (C) nanospheres transform into apatite nanocrystals; (D) biomimetic molecules (BM2) simulating non-collagenous protein bind with specific sites of the collagen molecule and a large number of nanocrystals with BM1 array in an orderly fashion to form mesocrystals; (E) the mesocrystals fuse to form single crystals, and intrafibrillar and interfibrillar remineralization of dentine are obtained (Zhong et al., 2015).
The core of dentin biomimetic remineralization theory is the formation of metastable amorphous calcium phosphate (ACP) nanoprecursors by atoms or molecules under the stabilizing effect of NCPs in hard tissues (Figure 2A) (Nurrohman et al., 2017). Subsequently, ACP readily penetrates the demineralized collagen matrix by molecular sieving (Figure 2B) (Qu et al., 2020). ACP then gradually transforms into crystals due to its low interfacial energy (Figure 2C). NCPs with CaP binding and collagen-binding functions bind to specific sites of collagen molecules, and many nanocrystals can penetrate the demineralized dentin (Sadoon et al., 2020). These infiltrated nanocrystals are guided by anions in biomimetic molecules anchored to collagen binding sites. Apatite nanocrystals form intrafibrillar and interfibrillar remineralized crystals along the surface of dentin collagen microfibrils and dentin collagen fibrils (Figure 2D) (Niu et al., 2014). The formation of new nanocrystals induced by biomimetic molecules may guide other apatite nanocrystals through self-assembly to produce larger and more stable polymeric mesocrystals. These mesocrystals eventually transform into larger plates of apatite crystals within and along the surface of dentin collagen fibrils (Figure 2E). The mineralization process is “bottom-up,” culminating in intra- and interfibrillar mineralization of dentin.
In 2009, Tay and Pashley (2009) pioneered the use of ACP and non-collagen analogs (polyacrylic acid (PAA) and polyvinyl phosphonic acid) to mineralize the demineralized areas inside and outside the dentin collagen fibrils formed due to acid etching. This led to the restoration of the original structural features of the dentin collagen fibrils. According to the literature, intrafibrillar mineral crystals are the main source of mechanical strength of dentin collagen fibrils and a vital sign of successful biomimetic mineralization (Gargouri et al., 2020). Subsequently, Malacarne et al. (2006) found a significant increase in bond durability of remineralized dentin samples after bonding with resin. They thus provided an experimental basis for subsequent remineralization to enhance dentin bond durability. The current research direction of guided tissue remineralization mainly has three aspects: biomimetic molecules, collagen, and amorphous nanoprecursors.
2.2.1 Role of non-collagenous proteins and their analogs in remineralization
NCPs and their analogs have been widely studied, considering their key role in controlling mineralization during crystal nucleation and growth (Bachli et al., 2019). NCPs reduce the nucleation activation energy and the driving force required for apatite formation (Di Foggia et al., 2019). The contemporary concept of biomineralization describes that acidic NCPs (e.g., dentin matrix protein I) stabilize calcium and phosphate ions in solutions to form pre-nuclear clusters. The pre-nucleated clusters aggregate to form ACP nanoparticles (NACPs) and localize in the intrafibrillar regions of collagenous fibrils. Crystalline alignment occurs in these intrafibrillar regions, culminating in the formation of monoapatite crystals in the interstitial regions within the collagen molecules (Bacino et al., 2019). Crystal growth in these intrafibrillar interstitial regions is beneficial because the mechanical properties of the dentin extracellular matrix are enhanced with minerals occupying these regions (Toledano et al., 2022). This also protects collagen molecules from enzymatic and acidic “attacks” (Padovano et al., 2015). Although the role of NCPs in regulating dentin type I collagen fibrils’ biomimetic mineralization has long been discovered, the complex process of obtaining natural NCPs and the high cost of their use have limited further research and their development. Inspired by the polyelectrolyte properties of NCPs (including mechanisms such as capillary forces (Olszta et al., 2007), size exclusion, charge interactions (Nudelman et al., 2010; Liu et al., 2021), and osmotic and charge balance (Niu et al., 2017)), researchers have attempted to use NCP analogs to stabilize NACPs for intrafibrillar remineralization (Shen et al., 2019) (Figure 3; Table 1).
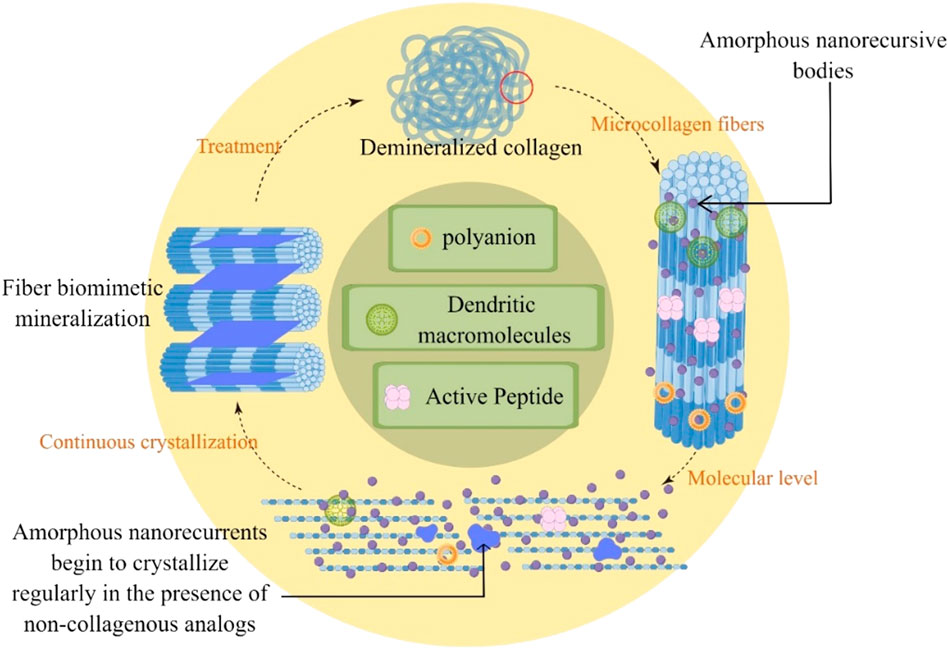
FIGURE 3. Remineralization of demineralized dentin with non-collagenous protein analogs through guided continuous crystallization of amorphous nanoprecursive bodies between collagen.
2.2.1.1 Polyanions
Polyacrylic acid, polyglutamic acid, polyaspartic acid (pAsp), and carboxylated polyethylene glycols are often used to simulate the negative charge properties of the deprotonated active form of NCP. Bryan D. Quan and Sone (2018) showed that both pAsp and polyglutamic acid acted as HAP nucleating agents in the same remineralization solution, inhibiting the formation of HAP from ACP in the solution. Zhao et al(2021) added aspartic acid (Asp) to a mineralization solution containing PAA and used transmission electron microscopy (TEM) and SEM to evaluate the remineralized layer of the demineralized dentin. Asp promoted the crystallization kinetics of PAA-stabilized ACP to HAP and accelerated the remineralization time to 2 days, equivalent to 7 days of mineralization without Asp. Wang’s group (Wang et al., 2021) prepared mineralized membranes composed of hydroxypropyl methylcellulose (HPMC) and pAsp-stabilized NACPs. HPMC, which contains multiple hydroxyl groups, is a film-forming material that forms a film when dried and gradually becomes a gel in a humid environment. HPMC was used as a carrier for pAsp-NACPs to provide biomimetic mineralization. The results showed that the mineralized film exhibited remineralization after 24 h, and the entire demineralized dentin thickness increased by 3–4 μm after 72–96 h.
Polydopamine (PDA), inspired by mussel proteins, can be used as a biomimetic analog to introduce functional groups on the surface of type I collagen as nucleation sites for CaP crystals (Amornkitbamrung et al., 2022). The main effect of PDA on the mineralized layer is due to its surface charged groups, such as catechol or amino groups. These groups can strongly interact with the surrounding calcium and phosphorus ions (Ryu et al., 2010). PDA added to primers can induce CaP mineral deposition on the surface of samples by forming irreversible deposits through the oxidative polymerization of dopamine under slightly alkaline conditions (Murari et al., 2020). Qu et al. (2020) significantly improved the mineralization degree and mechanical properties of remineralized dentin by using PDA. Moreover, they found that PDA could accelerate mineralization by reducing the interface between collagen and liquid ACP. This thus provided a new understanding for further shortening the time required for remineralization treatment. Zhou et al. (2012) also accomplished the sealing of dentin tubules through PDA-induced tooth remineralization, signifying that PDA may be used as a potential treatment for dentin hypersensitivity.
2.2.1.2 Active peptides
Chien et al. (2017) demonstrated that pretreatment with amphiphilic peptides enhanced collagen sorting and mineralization and induced functional remineralization of dentin damage in vitro. In the vicinity of dentinal tubules, newly formed apatite nanocrystals were co-linearly aligned with the c-axis parallel to the tubule perimeter. Restoration of the tissue ultrastructure resulted in high mechanical strength. The observation that aromatic groups interact with collagen while hydrophilic side chains bind to mineralized components highlights the potential of synthetic sequence-defined biomimetic polymers to mimic tissue remineralization as NCPs. Liang et al. (2015b) utilized an aspartate-serine-serine (DSS) peptide containing eight repeats (8DSS) to demonstrate that the 8DSS peptide has good binding strength to demineralized dentin. Moreover, the revealed that 8DSS induces nanocrystal precipitation on the dentin surface and in dentin tubules. The mechanical properties of 8DSS-coated samples were also significantly improved. All the aforementioned experiments showed that active peptides could mineralize dentin collagen fibril surfaces and interstitial areas of microfibrils. However, according to the literature, the active peptide structure may affect the morphology of the generated and deposited HA crystals (Mannem et al., 2020). In the experiments conducted by Xiu Peng’s group, the deposits formed after remineralization of active and inactive peptides showed two different crystalline forms, namely flakes and needles (Peng et al., 2021). Therefore, in future studies, we should focus on the new HA morphology after remineralization of each active peptide to identify the most suitable active peptide for restoring the original morphology and function of dentin.
2.2.1.3 Dendrimers
Some researchers have used polyamide-amine dendrimers to model the crucial role of NCP in biomineralization. Polyamide-amine polymers (PAMAM) have become a research hotspot because of their superior biomineralization ability. Moreover, they are characterized by their internal cavities and relatively low toxicity, better biocompatibility, and wider surface area (Bae et al., 2019). In addition, they show excellent performance by loading many reactive end groups on their surface (Liang et al., 2018b). For example, hydroxyl-terminated PAMAM (PAMAM-OH) (Liang et al., 2022), phosphate-terminated PAMAM (PAMAM-PO3H2) (Zhang et al., 2015), amine-terminated PAMAM (PAMAM-NH2) (Liang et al., 2015a), and carboxyl-terminated PAMAM (PAMAM-COOH) (Xie et al., 2016) have demonstrated functionally rich remineralization ability in their respective experiments. These structures and features enable PAMAM to mimic the biomineralization of the natural organic matrix on the tooth tissue surface. This allows PAMAM to act as an organic template to control mineral nucleation and crystal growth for better dentin biomineralization (Wen et al., 2020; Liang et al., 2016).
Zhu et al. (2018) successfully induced dentin remineralization and plugged dentinal tubules with phosphorylated poly (amide-amine) dendrimers containing apigenin. Incorporation of apigenin enhanced resistance to bacteria. Liang et al. (2019a) showed that even after up to 77 days of acidic fluid stimulation, the PAMAM experimental group still underwent moderate dentin remineralization. This suggested that most PAMAM macromolecules are firmly attached to demineralized dentin depending on electrostatic interactions and the size repulsion characteristics of collagen fibrils. Liang’s group (2017b) demonstrated for the first time the remineralization function of PAMAM with NACPs on demineralized dentin in acidic solutions without calcium and phosphorus ions.
PAMAM with high plasticity is increasingly used in dental tissue biomimetic restoration. Its inherent cytotoxicity is reduced by loading negatively charged groups that attenuate the positive surface charge of dendritic macromolecules (Wang et al., 2015). However, further studies are warranted to determine whether cytotoxicity will act as a barrier to the clinical use of this method for tissue remineralization.
Several other substances have been shown to have a good role in guided tissue remineralization. Agarose is a biopolysaccharide extracted from marine red algae that can be prepared into thermally reversible gels. Agarose and its derivatives and mixtures have been widely used in tissue engineering and regenerative medicine (Beaumont et al., 2021). In agarose hydrogel systems, agarose can be used as an organic template for biomimetic mineralization to form agarose fiber-nanoscale-ACP complex precursors (Han et al., 2017). By observing the SEM image of the sample after the agarose gel was used for remineralization, a prismatic structure similar to enamel was formed on the sample surface through crystallization of amorphous particles. Thus, remineralization of demineralized dentin collagen fibrils can be realized with agarose (Zarrintaj et al., 2018).
2.2.2 Importance of collagen in biomineralization
Dentin collagen fibrils provide a scaffold for mineral deposition. Therefore, a deeper understanding of the hierarchical self-assembly of mineralized collagen is indispensable for inducing dentin remineralization. Collagen constitutes 90% of the organic matrix, with the predominant protein being type I collagen (Zhao et al., 2020).
In early studies, collagen was considered to be unable to induce apatite formation and NCPs or their analogs had to be added. However, many studies have demonstrated that the collagen structure is necessary for directing apatite alignment and growth (Liang et al., 2019b). Qin et al(2021) concluded that type I collagen, which provides the three-dimensional structural framework for dentin remineralization, cannot by itself induce nucleation of carbonate apatite in the ACP phase. However, an in vitro study (Wang et al., 2012) showed that type I collagen could nucleate apatite in the absence of any other vertebrate calcified tissue extracellular matrix molecules to initiate and orient the growth of carbonate apatite minerals. They similarly concluded that the collagen matrix influenced the structural features of apatite at the atomic scale and controlled the size and three-dimensional distribution of apatite at a larger scale. The results showed that charged groups in collagen provide nucleation sites for inducing apatite nucleation. The experimental results of Su et al. (2021) suggested that type I collagen has a complex and ordered structure and plays a positive role in guiding the penetration of minerals into nucleation. Nevertheless, whether collagen alone can form intrafibrillar mineralization remains controversial. Wu et al. (2015) used DC electric fields to promote the mineralization of collagenous fibrils in the absence of NCPs or their analogs. This provides a novel idea on whether collagen can induce mineralization alone.
2.2.3 Role of amorphous nanoprecursors
Experiments have demonstrated that nanostructured CaPs in various forms of biomimetic CaPs are more effective in remineralization treatments than their macroscopic-sized counterpart. This is because of their biomimetic nature, higher surface area, reactivity, and better ability to adhere and penetrate the dentin. Compared with other crystalline CaP phases such as octacalcium phosphate [Ca8H2(PO4)6·5H2O] and tricalcium phosphate [Ca3(PO4)2], NACPs are widely used in the oral field because of their ability to release large amounts of Ca2+ and PO43- ions (Figure 4) (Iafisco et al., 2018).
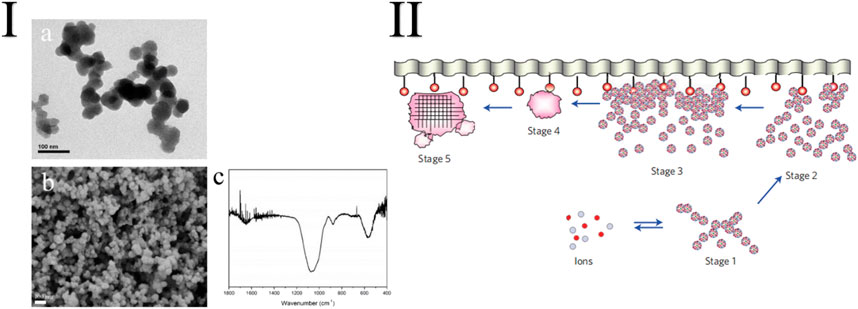
FIGURE 4. I: TEM (A) and SEM (B) images and FTIR characterization (C) of the synthetic NACPs (Zhang et al., 2019). II: Schematic representation of the different stages of surface-directed mineralization of CaP from SBF at 37°C. Stage 1: loose aggregation of prenucleation clusters in the equilibrium with ions in the solution. Stage 2: aggregation of prenucleation clusters in the presence of the monolayer with loose aggregates still present in the solution. Stage 3: aggregation leads to densification near the monolayer. Stage 4: nucleation of amorphous spherical particles only at the monolayer surface. Stage 5: development of crystallinity following oriented nucleation directed by the monolayer (Dey et al., 2010).
The amorphous phase strategy is also used by numerous organisms for mineralization, and its advantages can be explained in three ways (Tang et al., 2021). First, the highly hydrated amorphous phase has a liquid-like fluidity and plasticity, considered the basis for constructing complex minerals, especially in extremely small compartments (Athanasiadou Carneiro, 2021). Second, ACP is a concentrated phase of calcium and phosphate with higher mineralization efficiency and is easily transported compared with ionic solutions (Lotsari et al., 2018). Third, ACP is more soluble than HAP and therefore has more flexible and controllable properties in terms of recombination and fusion (Mu et al., 2021).
Apatite is the main inorganic component of dentin; therefore, the ordered redeposition of apatite is essential for the biomimetic remineralization of dentin (Cao et al., 2015). ACP particles are nanosized with a range of 20–300 nm. When observed through TEM, the morphology of dried CaP solids is usually curvilinear rather than the polyhedral angular shape of crystalline CaP (Wang et al., 2017).
ACP is a precursor of HAP (Wu et al., 2017) and slowly releases calcium ions into aqueous media to form HAP on hard tissues (Toledano et al., 2021). However, the instability of ACP limits its application for remineralization. By exploring natural dentin biomineralization, researchers have demonstrated that NCPs, such as dentin matrix protein 1 (DMP-1) and dentin salivary phosphoprotein (DSPP), can bind Ca2+ to stabilize ACP at the nanoscale and slow down the formation of large crystals (de Melo Pereira and Habibovic, 2018; Niu et al., 2021a; Saxena et al., 2019). ACP is a sub-stable phase that lacks long-range atomic organization and readily transforms into a thermodynamically stable crystalline phase such as octacalcium phosphate or apatite under supersaturated conditions (Braga, 2019; Xie et al., 2022). Under in vivo conditions or in the presence of certain ions, ACP may persist for some time due to kinetic stabilization. However, ACP is sub-stable in its wet state, and the exact mechanism of ACP stabilization remains unclear.
During remineralization, nanoprecursors are first introduced as a transitional stage in the formation of mineralized collagen fibrils (Lotsari et al., 2018), finally forming a well-crystallized apatite phase. ACP nanoprecursors are fluid, permeate demineralized collagen, and stabilize their transformation by adding NCP analogs to CaP medium (Yang et al., 2022). Therefore, the ACP particles could not be automatically converted into apatite crystals before entering the collagen fibrils. These nanoscale ACP particles are then electrostatically attracted to the collagen interstitial region that serves as the entry site. Finally, the alignment of charged amino acids within the interstitial and overlapping regions produces nucleation sites that guide the rearrangement and crystallization of ACP clusters along the collagen microfibrils and the collagen fibril surface.
Remineralized dentin collagen has good mechanical properties and is resistant to collagen degradation, bridging nano-microleakage gaps and significantly affecting the long-term bonding performance of the dentin‒adhesive resin interface. This suggests that biomimetic remineralization can be used as a long-term strategy for improving the durability of the dentin‒adhesive resin interface (Chen et al., 2020).
3 Application of guided tissue remineralization in dentin bonding
3.1 Factors influencing dentin bonding failure
Dentin bonding systems have evolved from the all-acid etching bonding theory (Fusayama et al., 1979) to the self-etching bonding theory (Kimochi et al., 1999; Chen et al., 2021). The bond strength has improved completely compared with the previous ones. Although immediate dentin bonding strength has improved, the durability of dentin bonding remains limited (Yamauchi et al., 2020). The annual failure rate of direct restorations is as high as 7.9% (Laske et al., 2016). Moreover, resin dentin bonding relies on the penetration of adhesive monomers into demineralized collagen fibrils, which are entangled and cured to form a hybrid layer for micromechanical retention (Zhou et al., 2019). However, due to the limited penetration of the resin monomer, many dentin collagen fibrils in the hybrid layer are not encapsulated by the resin monomer after demineralization (Sauro et al., 2015). Incorporating hydrophilic groups into the resin allows the bonding resin to continue to absorb water molecules for a long period, exerting a hydrolytic effect on the resin (Ito et al., 2005). Because of repeated mechanical stimulation induced by mastication and endogenous hydrolytic enzymes (Ye et al., 2017; Perdigao et al., 2013), the dentin collagen fibrils not encapsulated by the resin monomer hydrolyze (Hashimoto et al., 2003). After hydrolysis, these dentin collagen fibrils gradually undergo creep and cyclic fatigue fracture. This fracture destroys the structure of the original dentin bonding interface and significantly increases the uncertainty of dentin bond strength and durability (Zhang et al., 2020; Porto et al., 2018). Many cariogenic bacteria, represented by Streptococcus mutans, exist in the complex microenvironment of the oral cavity. When active, they produce large amounts of acid. This acid can demineralize the hard tissue of teeth and activate endogenous protease to decompose collagen fibril (Niu et al., 2021b). Thus, dentin bonding in the carious state is more complex, and the presence of bacteria further reduces the stability of the hybrid layer (Ekambaram et al., 2015).
Protection of the hybrid layer from attack by internal and external risk factors is essential for strengthening the dentin‒resin bond. Minerals play an extremely crucial role in protecting the stability of the hybrid layer. The guided tissue remineralization technique remineralizes demineralized dentin collagen fibrils. It can replace water in the hybrid layer, and the newly synthesized minerals can encapsulate the exposed collagen so that endogenous enzymes are not activated. This circumvents the challenges affecting dentin bonding in terms of residual water and the insufficient performance of resin in the hybrid layer (Lin et al., 2016). This technique partially or completely restores the mechanical strength and natural structure of naturally mineralized dentin. Researchers have added more ingredients, such as silver ions, chlorhexidine, and NaF (Barbosa-Martins et al., 2018), to the remineralization system to increase the stability of the bonding interface. Additional ingredients also inhibited the activity of various cariogenic bacteria in the oral cavity (Tao et al., 2020) or the activity of hydrolytic enzymes, such as matrix metalloproteases, in the bonding interface (Liang et al., 2017a). This significantly solves the problems of current dentin bonding systems and has a good application prospect (Figure 5; Table 2).
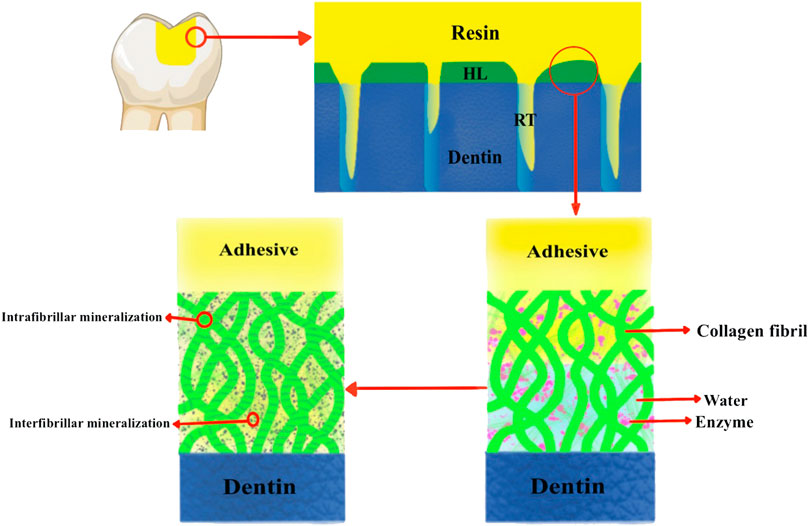
FIGURE 5. Schematic diagram of the principle of guiding tissue remineralization to promote dentin bonding. HL is the hybrid layer; RT is the resin tag.
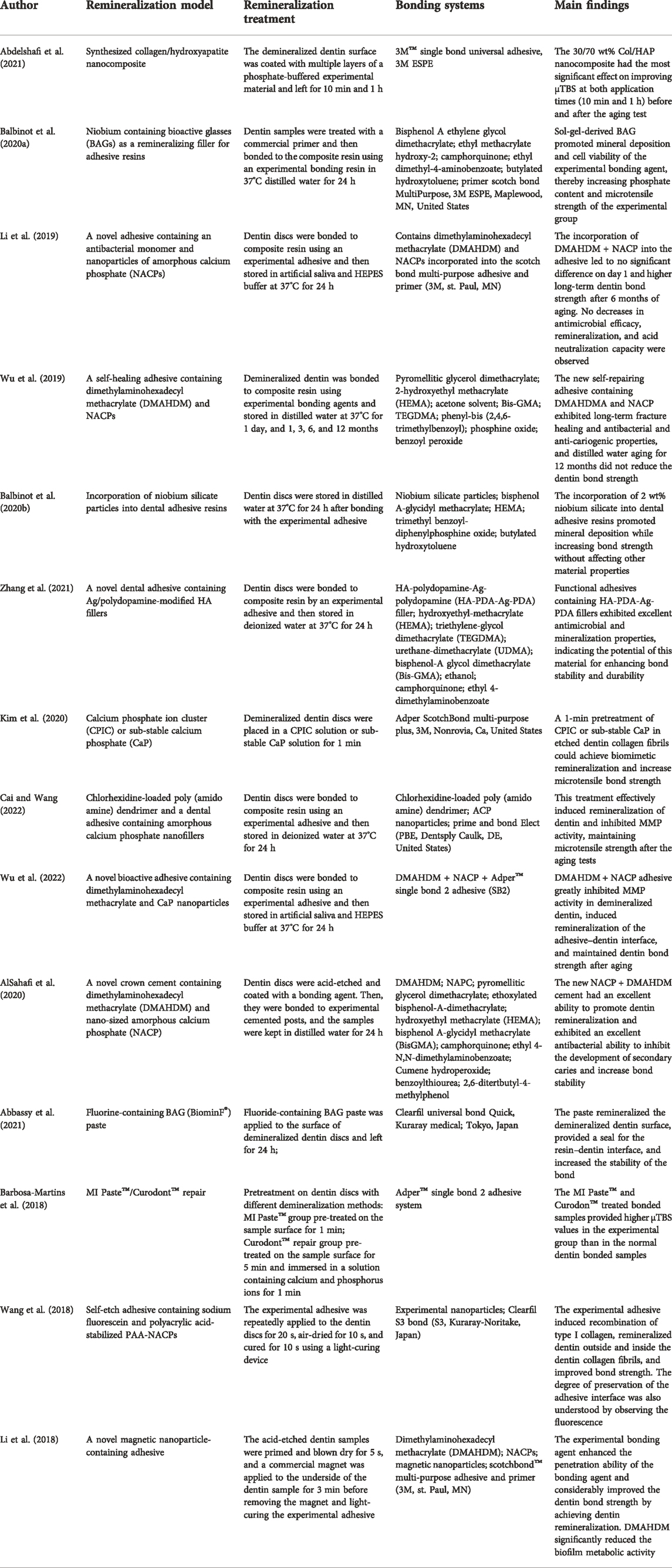
TABLE 2. Research results on the promotion of the bonding effect after dentin remineralization treatment.
3.2 Application of remineralization in an acid etch-and-rinse bonding system
Acid etching is the first step in resin dentin bonding. Using 32%–37% phosphoric acid in the etch-and-rinse bonding system, this step removes the smear layer and completely demineralizes the area within 5‒8 μm of the dentin. Therefore, in etch-and-rinse systems, the remineralization treatment should ensure that the remineralized material fills the area with the resin monomer. In addition, it should ensure that the remineralized material completely covers the deeper demineralized dentin not infiltrated by the resin monomer to prevent problems such as nanoleakage and collagen hydrolysis. Researchers have confirmed through continuous experiments that dentin samples, after biomimetic remineralization, still exhibit nascent nanocrystal deposits on the completely demineralized collagen fibrils under a transmission electron microscope. These deposits are observed even after applying etch-and-rinse bonding systems (Sauro et al., 2015). In addition, microtensile bond strength tests of dentin-bonded samples after long-term aging have demonstrated that the stability and durability of the resin–dentin bond could be effectively increased through biomimetic remineralization (Niu et al., 2014).
In the last decade, satisfactory results have been achieved in remineralizing dentin surfaces using BAG to form a CaP-rich layer (Bakry et al., 2011). The ability of fluorinated BAGs (FBGs) to form apatite in simulated body fluids has been previously demonstrated experimentally (Lusvardi et al., 2009). The addition of fluoride inhibits the increase in pH in the aqueous solution and forms fluorapatite (FAP). FAP is more chemically stable than HAP or carbonated HAP compared with fluoride-free glass (Brauer et al., 2010). Characterization of various studies showed that FBG pastes improved the acid resistance of the edges of demineralized dentin cavity surfaces without affecting the shear bond strength of dentin-bonded specimens (Abbassy et al., 2021). In addition, the FBG paste remineralized the demineralized dentin surface in a short time. This may be due to the nature of the paste that releases unstable calcium and phosphate ions without forming stable HAP crystals (Brauer et al., 2010).
3.3 Application of remineralization in a self-etch bonding system
Unlike etch-and-rinse bonding agents, self-etch bonding agents contain polymerizable methacrylate-based acidic resin monomers that simultaneously acid etch and penetrate the dentin matrix. Their efficacy varies widely, as does the depth of dentin demineralization. Because of the limited penetration capacity of the resin monomer, electron microscopy of the bonded samples treated with a self-etch bonding system revealed the presence of water channels extending from the hybrid layer to the adhesive layer after bonding. This indicated the presence of some demineralized collagen not encapsulated by the resin, which is one of the main factors causing bond failure (Braga and Fronza, 2020). Bonded samples treated with different self-etch systems showed different results after prolonged immersion in simulated body fluids rich in Ca2+ and PO43- without the involvement of NCP. The dentin samples treated with a highly acidic self-etch bonding system with the greatest mineralization depth did not even undergo new crystal deposition. This is because remineralization in the absence of NCP, the classical crystallization model, must be based on seed microcrystals remaining in the matrix as the core for new mineral crystallization. However, seed microcrystals are no longer present in the area of complete demineralization caused by acid etching in this group (Babaie et al., 2021). After the biomimetic remineralization treatment, under the microscope, all appear as continuous, tightly arranged mineral crystals following the collagen fibril arrangement. Because the space within the collagenous fibrils is occupied, intrafibrillar mineralization can be assumed to have occurred (Figure 6) (Kim et al., 2010).
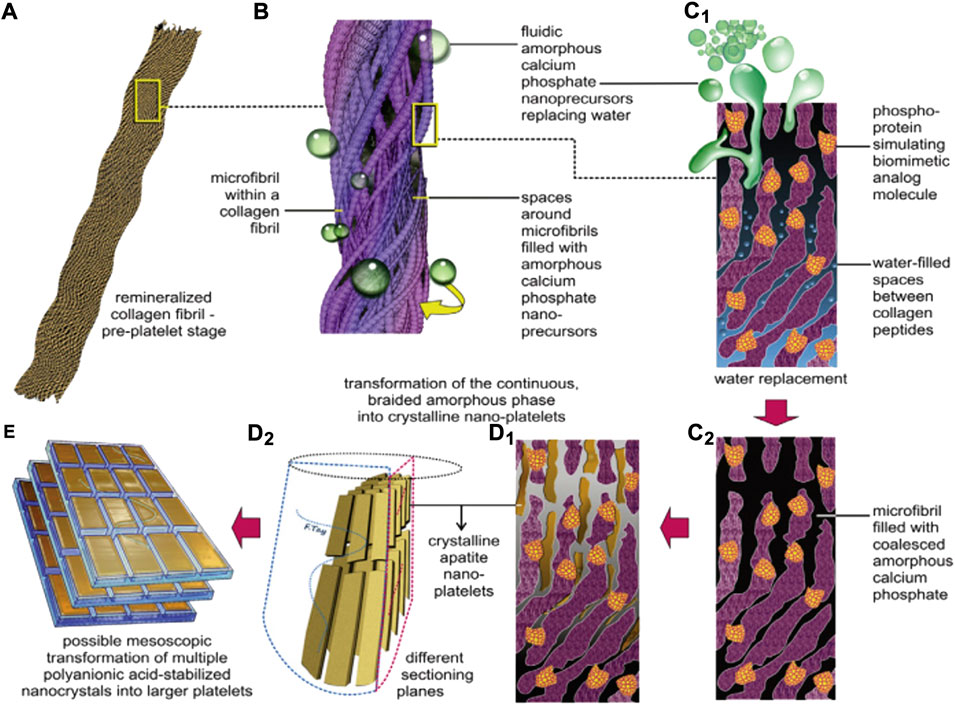
FIGURE 6. A schematic summarizing the features of biomimetic intrafibrillar remineralization observed in the completely demineralized resin-infiltrated collagen matrices created in human dentin. (A) Demineralized collagen fibril. (B) Rope-like microfibril infiltrated with fluidic ACP droplets generated by the biomimetic remineralization system. (C1) A view of a microfibril at the molecular level. ACP can be seen infiltrating the spaces around the collagen molecules through capillary action. (C2) Coalescence of the ACP in the microfibril. (D1,D2) Transformation of continuous braided amorphous precursors into nanocrystals, and their assembly into metastable mesocrystals. (E) Hypothetical fusion of mesocrystals into larger, single-crystalline platelets (Kim et al., 2010).
The function of acidic monomers in self-etch bonding systems is not limited to acid etching and penetration of the dentin matrix; however, with the increasing understanding of their properties, acidic monomers can also play a greater role. Acid functional monomers, such as methacryloyloxydecyl phosphate (MDP), can be chemically bonded to HAP to form a periodic nanolayer structure (Yoshihara et al., 2011). After bonding to the composite resin by the self-etch bonding system, the microtensile strength of dentin discs pretreated with the prefabricated ACP-MDP suspension increased by 59.1% compared with that of untreated bonded samples (Zhang et al., 2019).
In the clinical treatment process, the high technique sensitivity of dentin bonding or the dislodging of restoration caused by the long-term aging of the bonding agent is also among the main causes of secondary caries. The operator cannot directly observe the presence of the bonding agent; therefore, the condition cannot be detected and treated in time in the self-etch bonding system. Thus, incorporating fluorescein into the self-etching adhesive is a novel idea (Wang et al., 2018). Sodium fluorescein and PAA-NACPs were incorporated into the adhesive in the self-etch system to produce a new fluorescent adhesive and maintain the fluorescence intensity for a long period. The fluorescence facilitates the operator’s observation of the bonding interface while remineralizing it. This is a new approach for improving the bond durability of dentin and preventing secondary caries.
4 Conclusion and prospects
In summary, guided tissue remineralization enhances the deposition of mineral crystals within and between the collagen fibrils. This solves the problems of decreased collagen strength due to demineralization, nano-penetration due to insufficient resin infiltration, enzymatic digestion due to endogenous enzyme activation, and hydrolysis of resin within the hybrid layer of the dentin bonding system. Furthermore, the enhanced hybrid layer mineralization can effectively solve the aforementioned problems, improving the bond strength performance of the dentin bonding system. Products based on remineralization theory have been applied commercially. For example, MI Paste (GC Comp, Japan), whose main active ingredient is casein phosphopeptide-ACP (CPP-ACP), can remineralize dentin and enhance bonding strength (Dos Santos et al., 2016). However, because of the relatively complex oral environment in which dentin is located, such products have few clinical applications and lack long-term follow-up investigation. Further clinical data collection is required to explore whether these products play an active role in the durability of dentin bonding. However, guided tissue remineralization is still a promising solution for clinical applications.
Condensed matter chemistry studies have improved the understanding of the processes of organic substrates regulating nucleation, transformation, and growth in inorganic mineralized substances in biomineralization. As a special class of condensed matter, the theoretical mechanism of mineralization in dentin has progressed from the classical mineralization theory to the biomimetic remineralization theory. The biomimetic remineralization theory emphasizes the orderly deposition and interaction of collagen fibril scaffolds, NCPs and their analogs, and calcium and phosphorus substrates. Biomimetic remineralization mimics the natural biomineralization process by modifying and directing the self-assembly of calcium and phosphorus substrates in the collagen fibril framework through NCPs. Accordingly, biomimetic remineralization achieves an orderly cascade of crystal growth until mineralization is complete. Although these studies have yielded good results, many defects and shortcomings remain: 1) Although new mineralized deposits were formed in the remineralized dentin, the deposition thickness was still between tens and hundreds of microns. In addition, most studies have not considered the involvement of bacteria, and most cariogenic bacteria in the oral cavity produce high amounts of acid, which may affect the results of biomimetic remineralization (Tao et al., 2021). 2) For most dental restorations, biomineralization of the restorative material itself is not desirable. The restorative material should have a minimal or even zero biomineralization tendency. However, the enamel or dentin beneath the restoration may benefit from interfacial biomineralization. This tendency may lead to calculus formation (Vallittu et al., 2018). 3) Since current in vitro studies of dentin remineralization rely on the immersion of isolated teeth in a remineralizing solution (Tan et al., 2020), remineralization takes a long time (Cai et al., 2017) and cannot be effectively applied in clinical practice. 4) The cytotoxicity of remineralized materials should also be considered in the clinical application of tissue remineralization materials. Pure Portland-based cements commonly used in remineralization experiments may be potentially cytotoxic due to their high alkalinity (pH = 12) and the presence of arsenic ions, which is unacceptable for clinical applications. Remineralizing materials should have no cytotoxicity or should have no adverse effects on humans.
Future research on biomimetic mineralization should focus on deepening the understanding of mineral nucleation, aggregation, crystallization, and transformation processes in biomineralization. The mineralization precursor ACP can be converted to mineral crystals by adding the precursors to solid composite resins or dissolving them in remineralizing solutions. However, the optimal ACP phase for guiding tissue remineralization needs to be further investigated. This would allow us to find the best way for ACP participation in the mineralization process and achieve the most efficient mineralization deposition of calcium and phosphorus substrates. As a key component of guided tissue remineralization, NCPs and their analogs should be designed and formed into more diverse organic-inorganic composite biomaterials that promote bionanomineralization with the development of condensed matter chemistry. Bionanomineralization would facilitate finding the most suitable concentration for participation, the best biocompatibility, and the best mineralization effect. Furthermore, guided tissue remineralization should be explored from the perspective of clinical convenience and rapid response. This can be done by moving beyond the in vitro guidance phase in the laboratory and focusing on the complex oral microenvironment and the long guidance time in practical applications. We believe that with the continuous enrichment and understanding of the biomimetic mineralization principle, the fruitful results of guided tissue remineralization can become a new type of treatment in dentistry. This new treatment would provide more efficient solutions for caries, dentin hypersensitivity, and adhesive restoration.
Author contributions
WY and LM wrote the manuscript, RC and YX helped in literature search, and BL and BZ helped to revise the manuscript.
Funding
This study was supported by Natural Science Foundation of Shanxi Province, China (No. 201701D121144) and Shanxi Medical University School and Hospital of Stomatology Program (No.KY201908).
Conflict of interest
The authors declare that the research was conducted in the absence of any commercial or financial relationships that could be construed as a potential conflict of interest.
Publisher’s note
All claims expressed in this article are solely those of the authors and do not necessarily represent those of their affiliated organizations, or those of the publisher, the editors and the reviewers. Any product that may be evaluated in this article, or claim that may be made by its manufacturer, is not guaranteed or endorsed by the publisher.
References
Abbassy, M. A., Bakry, A. S., Hill, R., and Habib Hassan, A. (2021). Fluoride bioactive glass paste improves bond durability and remineralizes tooth structure prior to adhesive restoration. Dent. Mater 37 (1), 71–80. doi:10.1016/j.dental.2020.10.008
Abdelshafi, M. A., Fathy, S. M., Elkhooly, T. A., Reicha, F. M., and Osman, M. F. (2021). Bond strength of demineralized dentin after synthesized collagen/hydroxyapatite nanocomposite application. J. Mech. Behav. Biomed. Mater 121, 104590. doi:10.1016/j.jmbbm.2021.104590
Alsahafi, R., Balhaddad, A. A., Mitwalli, H., Ibrahim, M. S., Melo, M. A. S., Oates, T. W., et al. (2020). Novel crown cement containing antibacterial monomer and calcium phosphate nanoparticles. Nanomater. (Basel) 10 (10), 2001. doi:10.3390/nano10102001
Amornkitbamrung, U., In, Y., Wang, Z., Song, J., Oh, S. H., Hong, M. H., et al. (2022). c-Axis-Oriented platelets of crystalline hydroxyapatite in biomimetic intrafibrillar mineralization of polydopamine-functionalized collagen type I. ACS Omega 7 (6), 4821–4831. doi:10.1021/acsomega.1c05198
Aprillia, I., Suprastiwi, E., Megantoro, A., Trisna, L. P., Utami, B., and Yana, S. (2021). The effect of rice husk nanosilica hydroxyl compound on dentin biomineralization. J. Adv. Pharm. Technol. Res. 12 (3), 227–231. doi:10.4103/japtr.JAPTR_227_21
Athanasiadou, D., and Carneiro, K. M. M. (2021). DNA nanostructures as templates for biomineralization. Nat. Rev. Chem. 5 (2), 93–108. doi:10.1038/s41570-020-00242-5
Babaie, E., Bacino, M., White, J., Nurrohman, H., Marshall, G. W., Saeki, K., et al. (2021). Polymer-Induced Liquid Precursor (PILP) remineralization of artificial and natural dentin carious lesions evaluated by nanoindentation and microcomputed tomography. J. Dent. (Shiraz). 109, 103659. doi:10.1016/j.jdent.2021.103659
Bachli, K., Schmidlin, P. R., Wegehaupt, F., Paque, F., Ramenzoni, L., and Botter, S. (2019). Remineralization of artificial dentin caries using dentin and enamel matrix proteins. Mater. (Basel) 12 (13), 2116. doi:10.3390/ma12132116
Bacino, M., Girn, V., Nurrohman, H., Saeki, K., Marshall, S. J., Gower, L., et al. (2019). Integrating the PILP-mineralization process into a restorative dental treatment. Dent. Mat. 35 (1), 53–63. doi:10.1016/j.dental.2018.11.030
Bae, J., Son, W. S., Yoo, K. H., Yoon, S. Y., Bae, M. K., Lee, D. J., et al. (2019). Effects of poly(amidoamine) dendrimer-coated mesoporous bioactive glass nanoparticles on dentin remineralization. Nanomater. (Basel) 9 (4), 591. doi:10.3390/nano9040591
Bakry, A. S., Tamura, Y., Otsuki, M., Kasugai, S., Ohya, K., and Tagami, J. (2011). Cytotoxicity of 45S5 bioglass paste used for dentine hypersensitivity treatment. J. Dent. (Shiraz). 39 (9), 599–603. doi:10.1016/j.jdent.2011.06.003
Balbinot, G. S., Collares, F. M., Herpich, T. L., Visioli, F., Samuel, S. M. W., and Leitune, V. C. B. (2020a). Niobium containing bioactive glasses as remineralizing filler for adhesive resins. Dent. Mat. 36 (2), 221–228. doi:10.1016/j.dental.2019.11.014
Balbinot, G. S., Leitune, V. C. B., Ogliari, F. A., and Collares, F. M. (2020b). Niobium silicate particles promote in vitro mineral deposition on dental adhesive resins. J. Dent. (Shiraz). 101, 103449. doi:10.1016/j.jdent.2020.103449
Barbosa-Martins, L. F., Sousa, J. P., Alves, L. A., Davies, R. P. W., and Puppin-Rontanti, R. M. (2018). Biomimetic mineralizing agents recover the micro tensile bond strength of demineralized dentin. Mater. (Basel) 11 (9), 1733. doi:10.3390/ma11091733
Beaumont, M., Tran, R., Vera, G., Niedrist, D., Rousset, A., Pierre, R., et al. (2021). Hydrogel-forming algae polysaccharides: From seaweed to biomedical applications. Biomacromolecules 22 (3), 1027–1052. doi:10.1021/acs.biomac.0c01406
Bernales Sender, F. R., Castaneda Via, J. A., and Tay, L. Y. (2020). Influence of different phosphoric acids before application of universal adhesive on the dental enamel. J. Esthet. Restor. Dent. 32 (8), 797–805. doi:10.1111/jerd.12636
Bim-Junior, O., Curylofo-Zotti, F., Reis, M., Alania, Y., Lisboa-Filho, P. N., and Bedran-Russo, A. K. (2021). Surface-directed mineralization of fibrous collagen scaffolds in simulated body fluid for tissue engineering applications. ACS Appl. Bio Mat. 4 (3), 2514–2522. doi:10.1021/acsabm.0c01507
Braga, R. R. (2019). Calcium phosphates as ion-releasing fillers in restorative resin-based materials. Dent. Mat. 35 (1), 3–14. doi:10.1016/j.dental.2018.08.288
Braga, R. R., and Fronza, B. M. (2020). The use of bioactive particles and biomimetic analogues for increasing the longevity of resin-dentin interfaces: A literature review. Dent. Mat. J. 39 (1), 62–68. doi:10.4012/dmj.2019-293
Brauer, D. S., Karpukhina, N., O'Donnell, M. D., Law, R. V., and Hill, R. G. (2010). Fluoride-containing bioactive glasses: Effect of glass design and structure on degradation, pH and apatite formation in simulated body fluid. Acta Biomater. 6 (8), 3275–3282. doi:10.1016/j.actbio.2010.01.043
Breschi, L., Maravic, T., Cunha, S. R., Comba, A., Cadenaro, M., Tjaderhane, L., et al. (2018). Dentin bonding systems: From dentin collagen structure to bond preservation and clinical applications. Dent. Mat. 34 (1), 78–96. doi:10.1016/j.dental.2017.11.005
Cai, X., Han, B., Liu, Y., Tian, F., Liang, F., and Wang, X. (2017). Chlorhexidine-loaded amorphous calcium phosphate nanoparticles for inhibiting degradation and inducing mineralization of type I collagen. ACS Appl. Mat. Interfaces 9 (15), 12949–12958. doi:10.1021/acsami.6b14956
Cai, X., and Wang, X. (2022). Chlorhexidine-loaded poly (amido amine) dendrimer and a dental adhesive containing amorphous calcium phosphate nanofillers for enhancing bonding durability. Dent. Mat. 38 (5), 824–834. doi:10.1016/j.dental.2022.04.009
Cao, C. Y., and Li, Q. L. (2016). Scanning electron microscopic analysis of using agarose hydrogel microenvironment to create enamel prism-like tissue on dentine surface. J. Dent. (Shiraz). 55, 54–60. doi:10.1016/j.jdent.2016.09.015
Cao, C. Y., Mei, M. L., Li, Q. L., Lo, E. C., and Chu, C. H. (2015). Methods for biomimetic remineralization of human dentine: A systematic review. Int. J. Mol. Sci. 16 (3), 4615–4627. doi:10.3390/ijms16034615
Chen, L., Chen, W., Yu, Y., Yang, J., Jiang, Q., Wu, W., et al. (2021). Effect of chlorhexidine-loaded poly(amido amine) dendrimer on matrix metalloproteinase activities and remineralization in etched human dentin in vitro. J. Mech. Behav. Biomed. Mat. 121, 104625. doi:10.1016/j.jmbbm.2021.104625
Chen, R., Jin, R., Li, X., Fang, X., Yuan, D., Chen, Z., et al. (2020). Biomimetic remineralization of artificial caries dentin lesion using Ca/P-PILP. Dent. Mat. 36 (11), 1397–1406. doi:10.1016/j.dental.2020.08.017
Chien, Y. C., Tao, J., Saeki, K., Chin, A. F., Lau, J. L., Chen, C. L., et al. (2017). Using biomimetic polymers in place of noncollagenous proteins to achieve functional remineralization of dentin tissues. ACS Biomater. Sci. Eng. 3 (12), 3469–3479. doi:10.1021/acsbiomaterials.7b00378
Dai, L., Liu, Y., Salameh, Z., Khan, S., Mao, J., Pashley, D. H., et al. (2011). Can caries-affected dentin be completely remineralized by guided tissue remineralization? Dent. Hypotheses 2 (2), 59–68. doi:10.5436/j.dehy.2010.1.00011
Dai, L. L., Mei, M. L., Chu, C. H., and Lo, E. C. M. (2021). Remineralizing effect of a new strontium-doped bioactive glass and fluoride on demineralized enamel and dentine. J. Dent. (Shiraz). 108, 103633. doi:10.1016/j.jdent.2021.103633
Daneshpoor, N., and Pishevar, L. (2020). Comparative evaluation of bioactive cements on biomimetic remineralization of dentin. J. Clin. Exp. Dent. 12 (3), e291–e299. doi:10.4317/jced.56500
Daood, U., and Fawzy, A. S. (2020). Minimally invasive high-intensity focused ultrasound (HIFU) improves dentine remineralization with hydroxyapatite nanorods. Dent. Mat. 36 (3), 456–467. doi:10.1016/j.dental.2020.01.005
De Lima, J. F. M., Aguiar Jordao Mainardi, M. D. C., Puppin-Rontani, R. M., Pereira Rodrigues-Filho, U., Suzy Liporoni, P. C., Calegaro, M. L., et al. (2020). Bioinspired catechol chemistry for dentin remineralization: A new approach for the treatment of dentin hypersensitivity. Dent. Mat. 36 (4), 501–511. doi:10.1016/j.dental.2020.01.012
De Melo Pereira, D., and Habibovic, P. (2018). Biomineralization-inspired material design for bone regeneration. Adv. Healthc. Mat. 7 (22), e1800700. doi:10.1002/adhm.201800700
Dey, A., Bomans, P. H., Muller, F. A., Will, J., Frederik, P. M., De With, G., et al. (2010). The role of prenucleation clusters in surface-induced calcium phosphate crystallization. Nat. Mat. 9 (12), 1010–1014. doi:10.1038/nmat2900
Di Foggia, M., Prati, C., Gandolfi, M. G., and Taddei, P. (2019). An in vitro study on dentin demineralization and remineralization: Collagen rearrangements and influence on the enucleated phase. J. Inorg. Biochem. 193, 84–93. doi:10.1016/j.jinorgbio.2019.01.004
Dickens, S. H., Flaim, G. M., and Takagi, S. (2003). Mechanical properties and biochemical activity of remineralizing resin-based Ca-PO4 cements. Dent. Mat. 19 (6), 558–566. doi:10.1016/s0109-5641(02)00105-7
Dos Santos, R. A., De Lima, E. A., Montes, M., and Braz, R. (2016). Pre-treating dentin with chlorhexadine and CPP-ACP: Self-etching and universal adhesive systems. Acta Biomater. Odontol. Scand. 2 (1), 79–85. doi:10.1080/23337931.2016.1203265
Ekambaram, M., Yiu, C. K. Y., and Matinlinna, J. P. (2015). Bonding of resin adhesives to caries-affected dentin – a systematic review. Int. J. Adhesion Adhesives 61, 23–34. doi:10.1016/j.ijadhadh.2015.04.011
El Gezawi, M., Wolfle, U. C., Haridy, R., Fliefel, R., and Kaisarly, D. (2019). Remineralization, regeneration, and repair of natural tooth structure: Influences on the future of restorative dentistry practice. ACS Biomater. Sci. Eng. 5 (10), 4899–4919. doi:10.1021/acsbiomaterials.9b00591
Fusayama, T., Nakamura, M., Kurosaki, N., and Iwaku, M. (1979). Non-pressure adhesion of a new adhesive restorative resin. J. Dent. Res. 58 (4), 1364–1370. doi:10.1177/00220345790580041101
Gargouri, W., Kammoun, R., Elleuche, M., Tlili, M., Kechaou, N., and Ghoul-Mazgar, S. (2020). Effect of xylitol chewing gum enriched with propolis on dentin remineralization in vitro. Arch. Oral Biol. 112, 104684. doi:10.1016/j.archoralbio.2020.104684
Han, M., Li, Q. L., Cao, Y., Fang, H., Xia, R., and Zhang, Z. H. (2017). In vivo remineralization of dentin using an agarose hydrogel biomimetic mineralization system. Sci. Rep. 7, 41955. doi:10.1038/srep41955
Hashimoto, M., Ohno, H., Sano, H., Kaga, M., and Oguchi, H. (2003). In vitro degradation of resin-dentin bonds analyzed by microtensile bond test, scanning and transmission electron microscopy. Biomaterials 24 (21), 3795–3803. doi:10.1016/s0142-9612(03)00262-x
He, L., Hao, Y., Zhen, L., Liu, H., Shao, M., Xu, X., et al. (2019). Biomineralization of dentin. J. Struct. Biol. X. 207 (2), 115–122. doi:10.1016/j.jsb.2019.05.010
Iafisco, M., Degli Esposti, L., Ramirez-Rodriguez, G. B., Carella, F., Gomez-Morales, J., Ionescu, A. C., et al. (2018). Fluoride-doped amorphous calcium phosphate nanoparticles as a promising biomimetic material for dental remineralization. Sci. Rep. 8 (1), 17016. doi:10.1038/s41598-018-35258-x
Iijima, M., Ishikawa, R., Kawaguchi, K., Ito, S., Saito, T., and Mizoguchi, I. (2019). Effects of pastes containing ion-releasing particles on dentin remineralization. Dent. Mat. J. 38 (2), 271–277. doi:10.4012/dmj.2018-015
Ito, S., Hashimoto, M., Wadgaonkar, B., Svizero, N., Carvalho, R. M., Yiu, C., et al. (2005). Effects of resin hydrophilicity on water sorption and changes in modulus of elasticity. Biomaterials 26 (33), 6449–6459. doi:10.1016/j.biomaterials.2005.04.052
Kim, H., Choi, A., Gong, M. K., Park, H. R., and Kim, Y. I. (2020). Effect of remineralized collagen on dentin bond strength through calcium phosphate ion clusters or metastable calcium phosphate solution. Nanomater. (Basel) 10 (11), 2203. doi:10.3390/nano10112203
Kim, J., Arola, D. D., Gu, L., Kim, Y. K., Mai, S., Liu, Y., et al. (2010). Functional biomimetic analogs help remineralize apatite-depleted demineralized resin-infiltrated dentin via a bottom-up approach. Acta Biomater. 6 (7), 2740–2750. doi:10.1016/j.actbio.2009.12.052
Kimochi, T., Yoshiyama, M., Urayama, A., and Matsuo, T. (1999). Adhesion of a new commercial self-etching/self-priming bonding resin to human caries-infected dentin. Dent. Mat. J. 18 (4), 437–443. doi:10.4012/dmj.18.437
Laske, M., Opdam, N. J., Bronkhorst, E. M., Braspenning, J. C., and Huysmans, M. C. (2016). Longevity of direct restorations in Dutch dental practices. Descriptive study out of a practice based research network. J. Dent. (Shiraz). 46, 12–17. doi:10.1016/j.jdent.2016.01.002
Li, Y., Hu, X., Ruan, J., Arola, D. D., Ji, C., Weir, M. D., et al. (2019). Bonding durability, antibacterial activity and biofilm pH of novel adhesive containing antibacterial monomer and nanoparticles of amorphous calcium phosphate. J. Dent. (Shiraz). 81, 91–101. doi:10.1016/j.jdent.2018.12.013
Li, Y., Hu, X., Xia, Y., Ji, Y., Ruan, J., Weir, M. D., et al. (2018). Novel magnetic nanoparticle-containing adhesive with greater dentin bond strength and antibacterial and remineralizing capabilities. Dent. Mat. 34 (9), 1310–1322. doi:10.1016/j.dental.2018.06.001
Liang, K., Gao, Y., Li, J., Liao, Y., Xiao, S., Zhou, X., et al. (2015a). Biomimetic mineralization of collagen fibrils induced by amine-terminated PAMAM dendrimers-PAMAM dendrimers for remineralization. J. Biomaterials Sci. Polym. Ed. 26 (14), 963–974. doi:10.1080/09205063.2015.1068606
Liang, K., Gao, Y., Tao, S., Weir, M. D., Zhou, C., Li, J., et al. (2022). Dentin remineralization in acidic solution without initial calcium phosphate ions via poly(amido amine) and calcium phosphate nanocomposites after fluid challenges. Clin. Oral Investig. 26 (2), 1517–1530. doi:10.1007/s00784-021-04124-y
Liang, K., Gao, Y., Xiao, S., Tay, F. R., Weir, M. D., Zhou, X., et al. (2019a). Poly(amido amine) and rechargeable adhesive containing calcium phosphate nanoparticles for long-term dentin remineralization. J. Dent. (Shiraz). 85, 47–56. doi:10.1016/j.jdent.2019.04.011
Liang, K., Wang, S., Tao, S., Xiao, S., Zhou, H., Wang, P., et al. (2019b). Dental remineralization via poly(amido amine) and restorative materials containing calcium phosphate nanoparticles. Int. J. Oral Sci. 11 (2), 15. doi:10.1038/s41368-019-0048-z
Liang, K., Weir, M. D., Reynolds, M. A., Zhou, X., Li, J., and Xu, H. H. K. (2017a). Poly (amido amine) and nano-calcium phosphate bonding agent to remineralize tooth dentin in cyclic artificial saliva/lactic acid. Mater. Sci. Eng. C 72, 7–17. doi:10.1016/j.msec.2016.11.020
Liang, K., Weir, M. D., Xie, X., Wang, L., Reynolds, M. A., Li, J., et al. (2016). Dentin remineralization in acid challenge environment via PAMAM and calcium phosphate composite. Dent. Mat. 32 (11), 1429–1440. doi:10.1016/j.dental.2016.09.013
Liang, K., Xiao, S., Shi, W., Li, J., Yang, X., Gao, Y., et al. (2015b). 8DSS-promoted remineralization of demineralized dentin in vitro. J. Mat. Chem. B 3 (33), 6763–6772. doi:10.1039/c5tb00764j
Liang, K., Xiao, S., Weir, M. D., Bao, C., Liu, H., Cheng, L., et al. (2018a). Poly (amido amine) dendrimer and dental adhesive with calcium phosphate nanoparticles remineralized dentin in lactic acid. J. Biomed. Mat. Res. 106 (6), 2414–2424. doi:10.1002/jbm.b.34050
Liang, K., Xiao, S., Wu, J., Li, J., Weir, M. D., Cheng, L., et al. (2018b). Long-term dentin remineralization by poly(amido amine) and rechargeable calcium phosphate nanocomposite after fluid challenges. Dent. Mat. 34 (4), 607–618. doi:10.1016/j.dental.2018.01.001
Liang, K., Zhou, H., Weir, M. D., Bao, C., Reynolds, M. A., Zhou, X., et al. (2017b). Poly(amido amine) and calcium phosphate nanocomposite remineralization of dentin in acidic solution without calcium phosphate ions. Dent. Mat. 33 (7), 818–829. doi:10.1016/j.dental.2017.04.016
Lin, H. P., Lin, J., Li, J., Xu, J. H., and Mehl, C. (2016). In vitro remineralization of hybrid layers using biomimetic analogs. J. Zhejiang Univ. Sci. B 17 (11), 864–873. doi:10.1631/jzus.B1600151
Liu, C., Hao, Z., Yang, T., Wang, F., Sun, F., and Teng, W. (2021). Anti-acid biomimetic dentine remineralization using inorganic silica stabilized nanoparticles distributed electronspun nanofibrous mats. Int. J. Nanomedicine 16, 8251–8264. doi:10.2147/IJN.S331321
Liu, Y., Mai, S., Li, N., Yiu, C. K., Mao, J., Pashley, D. H., et al. (2011). Differences between top-down and bottom-up approaches in mineralizing thick, partially demineralized collagen scaffolds. Acta Biomater. 7 (4), 1742–1751. doi:10.1016/j.actbio.2010.11.028
Lotsari, A., Rajasekharan, A. K., Halvarsson, M., and Andersson, M. (2018). Transformation of amorphous calcium phosphate to bone-like apatite. Nat. Commun. 9 (1), 4170. doi:10.1038/s41467-018-06570-x
Lusvardi, G., Malavasi, G., Menabue, L., Aina, V., and Morterra, C. (2009). Fluoride-containing bioactive glasses: Surface reactivity in simulated body fluids solutions. Acta Biomater. 5 (9), 3548–3562. doi:10.1016/j.actbio.2009.06.009
Malacarne, J., Carvalho, R. M., De Goes, M. F., Svizero, N., Pashley, D. H., Tay, F. R., et al. (2006). Water sorption/solubility of dental adhesive resins. Dent. Mat. 22 (10), 973–980. doi:10.1016/j.dental.2005.11.020
Mannem, R., Yousuf, M., and Sreerama, L. (2020). Nanostructures formed by custom-made peptides based on amyloid peptide sequences and their inhibition by 2-hydroxynaphthoquinone. Front. Chem. 8, 684. doi:10.3389/fchem.2020.00684
Mao, L. B., Gao, H. L., Meng, Y. F., Yang, Y. L., Meng, S., and Yu, S. (2020). Biomineralization: A condensed matter chemistry. Prog. Chem. 32 (8), 1086–1099. doi:10.7536/PC200430
Mu, Z., Kong, K., Jiang, K., Dong, H., Xu, X., Liu, Z., et al. (2021). Pressure-driven fusion of amorphous particles into integrated monoliths. Science 372 (6549), 1466–1470. doi:10.1126/science.abg1915
Murari, G., Bock, N., Zhou, H., Yang, L., Liew, T., Fox, K., et al. (2020). Effects of polydopamine coatings on nucleation modes of surface mineralization from simulated body fluid. Sci. Rep. 10 (1), 14982. doi:10.1038/s41598-020-71900-3
Niu, J., Li, D., Zhou, Z., Zhang, J., Liu, D., Zhao, W., et al. (2021a). The incorporation of phosphorylated chitosan/amorphous calcium phosphate nanocomplex into an experimental composite resin. Dent. Mat. J. 40 (2), 422–430. doi:10.4012/dmj.2019-427
Niu, J. Y., Yin, I. X., Wu, W. K. K., Li, Q. L., Mei, M. L., and Chu, C. H. (2021b). Remineralising dentine caries using an artificial antimicrobial peptide: An in vitro study. J. Dent. (Shiraz). 111, 103736. doi:10.1016/j.jdent.2021.103736
Niu, L. N., Jee, S. E., Jiao, K., Tonggu, L., Li, M., Wang, L., et al. (2017). Collagen intrafibrillar mineralization as a result of the balance between osmotic equilibrium and electroneutrality. Nat. Mat. 16 (3), 370–378. doi:10.1038/nmat4789
Niu, L. N., Zhang, W., Pashley, D. H., Breschi, L., Mao, J., Chen, J. H., et al. (2014). Biomimetic remineralization of dentin. Dent. Mat. 30 (1), 77–96. doi:10.1016/j.dental.2013.07.013
Nudelman, F., Pieterse, K., George, A., Bomans, P. H., Friedrich, H., Brylka, L. J., et al. (2010). The role of collagen in bone apatite formation in the presence of hydroxyapatite nucleation inhibitors. Nat. Mat. 9 (12), 1004–1009. doi:10.1038/nmat2875
Nurrohman, H., Carneiro, K. M. M., Hellgeth, J., Saeki, K., Marshall, S. J., Marshall, G. W., et al. (2017). The role of protease inhibitors on the remineralization of demineralized dentin using the PILP method. PLoS One 12 (11), e0188277. doi:10.1371/journal.pone.0188277
Olszta, M. J., Cheng, X., Jee, S. S., Kumar, R., Kim, Y.-Y., Kaufman, M. J., et al. (2007). Bone structure and formation: A new perspective. Mater. Sci. Eng. R Rep. 58 (3), 77–116. doi:10.1016/j.mser.2007.05.001
Padovano, J. D., Ravindran, S., Snee, P. T., Ramachandran, A., Bedran-Russo, A. K., and George, A. (2015). DMP1-derived peptides promote remineralization of human dentin. J. Dent. Res. 94 (4), 608–614. doi:10.1177/0022034515572441
Peng, X., Han, S., Wang, K., Ding, L., Liu, Z., and Zhang, L. (2021). The amelogenin-derived peptide TVH-19 promotes dentinal tubule occlusion and mineralization. Polym. (Basel) 13 (15), 2473. doi:10.3390/polym13152473
Perdigao, J., Reis, A., and Loguercio, A. D. (2013). Dentin adhesion and MMPs: A comprehensive review. J. Esthet. Restor. Dent. 25 (4), 219–241. doi:10.1111/jerd.12016
Porto, I., Nascimento, T. G., Oliveira, J. M. S., Freitas, P. H., Haimeur, A., and FrançA, R. (2018). Use of polyphenols as a strategy to prevent bond degradation in the dentin-resin interface. Eur. J. Oral Sci. 126 (2), 146–158. doi:10.1111/eos.12403
Qin, H., Long, J., Zhou, J., Wu, L., and Xie, F. (2021). Use of phosphorylated PAMAM and carboxyled PAMAM to induce dentin biomimetic remineralization and dentinal tubule occlusion. Dent. Mat. J. 40 (3), 800–807. doi:10.4012/dmj.2020-222
Qu, Y., Gu, T., Du, Q., Shao, C., Wang, J., Jin, B., et al. (2020). Polydopamine promotes dentin remineralization via interfacial control. ACS Biomater. Sci. Eng. 6 (6), 3327–3334. doi:10.1021/acsbiomaterials.0c00035
Quan, B. D., and Sone, E. D. (2018). The effect of polyaspartate chain length on mediating biomimetic remineralization of collagenous tissues. J. R. Soc. Interface 15 (147), 20180269. doi:10.1098/rsif.2018.0269
Reise, M., Kranz, S., Heyder, M., Jandt, K. D., and Sigusch, B. W. (2021). Effectiveness of casein phosphopeptide-amorphous calcium phosphate (CPP-ACP) compared to fluoride products in an in-vitro demineralization model. Mater. (Basel) 14 (20), 5974. doi:10.3390/ma14205974
Ryu, J., Ku, S. H., Lee, H., and Park, C. B. (2010). Mussel-inspired polydopamine coating as a universal route to hydroxyapatite crystallization. Adv. Funct. Mat. 20 (13), 2132–2139. doi:10.1002/adfm.200902347
Sadoon, N. Y., Fathy, S. M., and Osman, M. F. (2020). Effect of using biomimetic analogs on dentin remineralization with bioactive cements. Braz. Dent. J. 31 (1), 44–51. doi:10.1590/0103-6440202003083
Saffarpour, M., Mohammadi, M., Tahriri, M., and Zakerzadeh, A. (2017). Efficacy of modified bioactive glass for dentin remineralization and obstruction of dentinal tubules. J. Dent. 14 (4), 212–222.
Sang, Y. H., Pan, H. H., and Tang, R. K. (2020). Condensed-matter chemistry in biomineralization. Prog. Chem. 32 (8), 1100–1114. doi:10.7536/PC200431
Sauro, S., Osorio, R., Watson, T. F., and Toledano, M. (2015). Influence of phosphoproteins' biomimetic analogs on remineralization of mineral-depleted resin-dentin interfaces created with ion-releasing resin-based systems. Dent. Mat. 31 (7), 759–777. doi:10.1016/j.dental.2015.03.013
Saxena, N., Cremer, M. A., Dolling, E. S., Nurrohman, H., Habelitz, S., Marshall, G. W., et al. (2018). Influence of fluoride on the mineralization of collagen via the polymer-induced liquid-precursor (PILP) process. Dent. Mat. 34 (9), 1378–1390. doi:10.1016/j.dental.2018.06.020
Saxena, N., Habelitz, S., Marshall, G. W., and Gower, L. B. (2019). Remineralization of demineralized dentin using a dual analog system. Orthod. Craniofac. Res. 22 (1), 76–81. doi:10.1111/ocr.12271
Shen, M., Lin, M., Zhu, M., Zhang, W., Lu, D., Liu, H., et al. (2019). MV-mimicking micelles loaded with PEG-serine-ACP nanoparticles to achieve biomimetic intra/extra fibrillar mineralization of collagen in vitro. Biochimica Biophysica Acta - General Subj. 1863 (1), 167–181. doi:10.1016/j.bbagen.2018.10.005
Su, Q. Q., Zhang, C., Mai, S., Lin, H. C., and Zhi, Q. H. (2021). Effect of poly (γ-glutamic acid)/tricalcium phosphate (γ-PGA/TCP) composite for dentin remineralization in vitro. Dent. Mat. J. 40 (1), 26–34. doi:10.4012/dmj.2019-324
Tan, S., Chen, S., Wang, Y., Wu, F., Shi, Y., Wang, J., et al. (2020). Enhanced effect of nano-monetite hydrosol on dentin remineralization and tubule occlusion. Dent. Mat. 36 (6), 816–825. doi:10.1016/j.dental.2020.03.028
Tang, S., Dong, Z., Ke, X., Luo, J., and Li, J. (2021). Advances in biomineralization-inspired materials for hard tissue repair. Int. J. Oral Sci. 13 (1), 42. doi:10.1038/s41368-021-00147-z
Tao, S., He, L., Xu, H. H. K., Weir, M. D., Fan, M., Yu, Z., et al. (2019). Dentin remineralization via adhesive containing amorphous calcium phosphate nanoparticles in a biofilm-challenged environment. J. Dent. (Shiraz). 89, 103193. doi:10.1016/j.jdent.2019.103193
Tao, S., Su, Z., Xiang, Z., Xu, H. H. K., Weir, M. D., Fan, M., et al. (2020). Nano-calcium phosphate and dimethylaminohexadecyl methacrylate adhesive for dentin remineralization in a biofilm-challenged environment. Dent. Mat. 36 (10), e316–e328. doi:10.1016/j.dental.2020.08.001
Tao, S., Yang, X., Liao, L., Yang, J., Liang, K., Zeng, S., et al. (2021). A novel anticaries agent, honokiol-loaded poly(amido amine) dendrimer, for simultaneous long-term antibacterial treatment and remineralization of demineralized enamel. Dent. Mat. 37 (9), 1337–1349. doi:10.1016/j.dental.2021.06.003
Tay, F. R., and Pashley, D. H. (2009). Biomimetic remineralization of resin-bonded acid-etched dentin. J. Dent. Res. 88 (8), 719–724. doi:10.1177/0022034509341826
Toledano, M., Toledano-Osorio, M., Hannig, M., Carrasco-Carmona, A., Osorio, M. T., Garcia-Godoy, F., et al. (2022). Zn-Containing adhesives facilitate collagen protection and remineralization at the resin-dentin interface: A narrative review. Polym. (Basel) 14 (3), 642. doi:10.3390/polym14030642
Toledano, M., Vallecillo-Rivas, M., Aguilera, F. S., Osorio, M. T., Osorio, E., and Osorio, R. (2021). Polymeric zinc-doped nanoparticles for high performance in restorative dentistry. J. Dent. (Shiraz). 107, 103616. doi:10.1016/j.jdent.2021.103616
Vallittu, P. K., Boccaccini, A. R., Hupa, L., and Watts, D. C. (2018). Bioactive dental materials-Do they exist and what does bioactivity mean? Dent. Mat. 34 (5), 693–694. doi:10.1016/j.dental.2018.03.001
Wang, H., Xiao, Z., Yang, J., Lu, D., Kishen, A., Li, Y., et al. (2017). Oriented and ordered biomimetic remineralization of the surface of demineralized dental enamel using HAP@ACP nanoparticles guided by Glycine. Sci. Rep. 7, 40701. doi:10.1038/srep40701
Wang, T., Yang, S., Wang, L., and Feng, H. (2015). Use of multifunctional phosphorylated PAMAM dendrimers for dentin biomimetic remineralization and dentinal tubule occlusion. RSC Adv. 5 (15), 11136–11144. doi:10.1039/c4ra14744h
Wang, Y., AzaïS, T., Robin, M., ValléE, A., Catania, C., Legriel, P., et al. (2012). The predominant role of collagen in the nucleation, growth, structure and orientation of bone apatite. Nat. Mat. 11 (8), 724–733. doi:10.1038/nmat3362
Wang, Z., Ouyang, Y., Wu, Z., Zhang, L., Shao, C., Fan, J., et al. (2018). A novel fluorescent adhesive-assisted biomimetic mineralization. Nanoscale 10 (40), 18980–18987. doi:10.1039/c8nr02078g
Wang, Z., Zhou, Z., Fan, J., Zhang, L., Zhang, Z., Wu, Z., et al. (2021). Hydroxypropylmethylcellulose as a film and hydrogel carrier for ACP nanoprecursors to deliver biomimetic mineralization. J. Nanobiotechnology 19 (1), 385. doi:10.1186/s12951-021-01133-7
Wen, Y., Wang, J., Luo, J., and Yang, J. (2020). Remineralization of dentine tubules induced by phosphate-terminated PAMAM dendrimers. Heliyon 6 (12), e05886. doi:10.1016/j.heliyon.2020.e05886
Wu, J., Zhou, C., Ruan, J., Weir, M. D., Tay, F., Sun, J., et al. (2019). Self-healing adhesive with antibacterial activity in water-aging for 12 months. Dent. Mat. 35 (8), 1104–1116. doi:10.1016/j.dental.2019.05.004
Wu, L., Cao, X., Meng, Y., Huang, T., Zhu, C., Pei, D., et al. (2022). Novel bioactive adhesive containing dimethylaminohexadecyl methacrylate and calcium phosphate nanoparticles to inhibit metalloproteinases and nanoleakage with three months of aging in artificial saliva. Dent. Mat. 38 (7), 1206–1217. doi:10.1016/j.dental.2022.06.017
Wu, Q., Mei, M. L., Wu, X., Shi, S., Xu, Y., Chu, C. H., et al. (2020). Remineralising effect of 45S5 bioactive glass on artificial caries in dentine. BMC Oral Health 20 (1), 49. doi:10.1186/s12903-020-1038-4
Wu, X. T., Mei, M. L., Li, Q. L., Cao, C. Y., Chen, J. L., Xia, R., et al. (2015). A direct electric field-aided biomimetic mineralization system for inducing the remineralization of dentin collagen matrix. Mater. (Basel) 8 (11), 7889–7899. doi:10.3390/ma8115433
Wu, Z., Wang, X., Wang, Z., Shao, C., Jin, X., Zhang, L., et al. (2017). Self-etch adhesive as a carrier for ACP nanoprecursors to deliver biomimetic remineralization. ACS Appl. Mat. Interfaces 9 (21), 17710–17717. doi:10.1021/acsami.7b01719
Xiang, K., Chen, L., Chen, W., and Yang, D. (2021). Remineralization of dentin induced by a compound of polyamide-amine and chlorhexidine in a resin dentin bonding microenvironment. Ann. Transl. Med. 9 (6), 472. doi:10.21037/atm-21-472
Xie, F., Long, J., Yang, J., Qin, H., Lin, X., and Chen, W. (2022). Effect of a new modified polyamidoamine dendrimer biomimetic system on the mineralization of type I collagen fibrils: An in vitro study. J. Biomaterials Sci. Polym. Ed. 33 (2), 212–228. doi:10.1080/09205063.2021.1982642
Xie, F., Wei, X., Li, Q., and Zhou, T. (2016). In vivo analyses of the effects of polyamidoamine dendrimer on dentin biomineralization and dentinal tubules occlusion. Dent. Mat. J. 35 (1), 104–111. doi:10.4012/dmj.2015-209
Xu, J., Chen, Y., Li, X., Lei, Y., Shu, C., Luo, Q., et al. (2021). Reconstruction of a demineralized dentin matrix via rapid deposition of CaF2 nanoparticles in situ promotes dentin bonding. ACS Appl. Mat. Interfaces 13 (43), 51775–51789. doi:10.1021/acsami.1c15787
Yamauchi, S., Wang, X., Egusa, H., and Sun, J. (2020). High-performance dental adhesives containing an ether-based monomer. J. Dent. Res. 99 (2), 189–195. doi:10.1177/0022034519895269
Yang, J., Huang, J., Qin, H., Long, J., Lin, X., and Xie, F. (2022). Remineralization of human dentin type I collagen fibrils induced by carboxylated polyamidoamine dendrimer/amorphous calcium phosphate nanocomposite: An in vitro study. J. Biomaterials Sci. Polym. Ed. 33 (5), 668–686. doi:10.1080/09205063.2021.2008789
Ye, Q., Spencer, P., Yuca, E., and Tamerler, C. (2017). Engineered peptide repairs defective adhesive-dentin interface. Macromol. Mat. Eng. 302 (5), 1600487. doi:10.1002/mame.201600487
Yoshihara, K., Yoshida, Y., Hayakawa, S., Nagaoka, N., Irie, M., Ogawa, T., et al. (2011). Nanolayering of phosphoric acid ester monomer on enamel and dentin. Acta Biomater. 7 (8), 3187–3195. doi:10.1016/j.actbio.2011.04.026
Zarrintaj, P., Manouchehri, S., Ahmadi, Z., Saeb, M. R., Urbanska, A. M., Kaplan, D. L., et al. (2018). Agarose-based biomaterials for tissue engineering. Carbohydr. Polym. 187, 66–84. doi:10.1016/j.carbpol.2018.01.060
Zhang, H., Yang, J., Liang, K., Li, J., He, L., Yang, X., et al. (2015). Effective dentin restorative material based on phosphate-terminated dendrimer as artificial protein. Colloids Surfaces B Biointerfaces 128, 304–314. doi:10.1016/j.colsurfb.2015.01.058
Zhang, J., He, X., Yu, S., Zhu, J., Wang, H., Tian, Z., et al. (2021). A novel dental adhesive containing Ag/polydopamine-modified HA fillers with both antibacterial and mineralization properties. J. Dent. (Shiraz). 111, 103710. doi:10.1016/j.jdent.2021.103710
Zhang, L., Wang, W., Wang, C., Li, M., Wang, Z., Su, Z., et al. (2019). Interaction of ACP and MDP and its effect on dentin bonding performance. J. Mech. Behav. Biomed. Mat. 91, 301–308. doi:10.1016/j.jmbbm.2018.12.017
Zhang, Z., Yu, J., Yao, C., Yang, H., and Huang, C. (2020). New perspective to improve dentin-adhesive interface stability by using dimethyl sulfoxide wet-bonding and epigallocatechin-3-gallate. Dent. Mat. 36 (11), 1452–1463. doi:10.1016/j.dental.2020.08.009
Zhao, I. S., Yin, I. X., Mei, M. L., Lo, E. C. M., Tang, J., Li, Q., et al. (2020). Remineralising dentine caries using sodium fluoride with silver nanoparticles: An in vitro study. Int. J. Nanomedicine 15, 2829–2839. doi:10.2147/IJN.S247550
Zhao, L., Sun, J., Zhang, C., Chen, C., Chen, Y., Zheng, B., et al. (2021). Effect of aspartic acid on the crystallization kinetics of ACP and dentin remineralization. J. Mech. Behav. Biomed. Mat. 115, 104226. doi:10.1016/j.jmbbm.2020.104226
Zhong, B., Peng, C., Wang, G., Tian, L., Cai, Q., and Cui, F. (2015). Contemporary research findings on dentine remineralization. J. Tissue Eng. Regen. Med. 9 (9), 1004–1016. doi:10.1002/term.1814
Zhou, W., Liu, S., Zhou, X., Hannig, M., Rupf, S., Feng, J., et al. (2019). Modifying adhesive materials to improve the longevity of resinous restorations. Int. J. Mol. Sci. 20 (3), 723. doi:10.3390/ijms20030723
Zhou, Y. Z., Cao, Y., Liu, W., Chu, C. H., and Li, Q. L. (2012). Polydopamine-induced tooth remineralization. ACS Appl. Mat. Interfaces 4 (12), 6901–6910. doi:10.1021/am302041b
Zhou, Z., Ge, X., Bian, M., Xu, T., Li, N., Lu, J., et al. (2020). Remineralization of dentin slices using casein phosphopeptide-amorphous calcium phosphate combined with sodium tripolyphosphate. Biomed. Eng. OnLine 19 (1), 18. doi:10.1186/s12938-020-0756-9
Keywords: guided tissue remineralization, biomimetic mineralization, dentin bonding, non-collagenous proteins, amorphous calcium phosphate
Citation: Yao W, Ma L, Chen R, Xie Y, Li B and Zhao B (2022) Guided tissue remineralization and its effect on promoting dentin bonding. Front. Mater. 9:1026522. doi: 10.3389/fmats.2022.1026522
Received: 24 August 2022; Accepted: 11 October 2022;
Published: 26 October 2022.
Edited by:
Junling Wu, Shandong University, ChinaReviewed by:
Amaury Pozos, Autonomous University of San Luis Potosí, MexicoLisha Gu, Sun Yat-Sen University, China
Copyright © 2022 Yao, Ma, Chen, Xie, Li and Zhao. This is an open-access article distributed under the terms of the Creative Commons Attribution License (CC BY). The use, distribution or reproduction in other forums is permitted, provided the original author(s) and the copyright owner(s) are credited and that the original publication in this journal is cited, in accordance with accepted academic practice. No use, distribution or reproduction is permitted which does not comply with these terms.
*Correspondence: Wei Yao, eWFvd2F5QDE2My5jb20=; Bin Zhao, U3htdTA2ODhAMTI2LmNvbQ==
†These authors have contributed equally to this work and share first authorship