- 1College of Electrical and New Energy, China Three Gorges University, Yichang, China
- 2State Key Laboratory of Advanced Electromagnetic Engineering and Technology, Huazhong University of Science and Technology, Wuhan, China
Polyimide is often used as the insulating material of the repulsion coil, which needs to withstand the collision of the fast repulsion mechanism in high-speed motion and temperature rise. The polyimide molecular model was established in this study. The external electric field was applied to the model through the semi-empirical method to calculate the total molecular energy, dipole moment, molecular orbital energy, and energy gap of polyimide. It was found that the total molecular energy of plasma-modified polyimide was lower, the energy gap was smaller, and the corresponding molecular properties were more stable. Then, the cell models of ordinary polyimide and plasma-modified polyimide were established by molecular dynamics simulation. The effects of plasma modification on the micro properties of polyimide were studied and compared. The free volume, mean square displacement, cohesive energy density, mechanical properties, and relative dielectric constant of polyimide models with different modified ratios were calculated. The results show that the introduction of polar groups through plasma modification can inhibit the movement of the polyimide molecular chain and improve the thermal stability of the polyimide system. The mechanical properties of polyimide are also improved due to plasma modification, and the elastic modulus is the largest when the modification rate is 20%. At the same time, the relative dielectric constant of polyimide increases with the increase of the modification rate.
Introduction
Polyimide, as a polymer composite material, has attracted much attention because of its excellent mechanical properties, stable structure under extreme environments, good insulation performance, relatively weak acid resistance, and radiation resistance (Ohya et al., 1997; Kausar, 2017; Li, 2019; Yang et al., 2020). As the core component of a mechanical DC circuit breaker, a fast repulsion mechanism is gradually required to withstand high voltage and large current (Pan et al., 2018; Li et al., 2022). Polyimide is often used as the insulating material of a repulsion coil due to its excellent mechanical properties, stable structure under extreme environments, and good insulation performance. It needs to withstand the collision of the fast repulsion mechanism in high-speed motion and temperature rise. The performance of polyimide directly determines the service life of electrical equipment. Therefore, it is urgent to improve the mechanical, thermodynamic, and insulating properties of polyimide.
In recent years, low-temperature plasma technology has been widely used in the surface modification of polymer materials to enhance the mechanical, thermodynamic, and insulating properties of polyimide (Fang, Zhi et al., 2010; Hu, Wen et al., 2015). The performance changes of polyimides at different temperatures were analyzed (Gao, Mengyan et al., 2021). Also, most of the research on polyimide focuses on improving its physical and chemical properties through nano-modification methods such as adding silica, zinc oxide, and titanium dioxide (Jia et al., 2016; Gong, Ying et al., 2018; Yang, Jiaming et al., 2018; Jia, Yanjiang et al., 2021) to the model. Most of the aforementioned studies have studied the effects of different conditions on the molecular properties of polyimides through experiments but have not analyzed and predicted the molecules at the microscopic level. In recent years, with the development of molecular simulation technology (Seyedzavvar, 2021; Takari et al., 2021; Yuan et al., 2021), the design and preparation of polyimides with better performance using simulation methods have gradually become a research hotspot (Xu, Jingcheng et al., 2018; Huang, Xuwei et al., 2020). More and more researchers have begun to pay attention to exploring the micro mechanism of the influence of plasma modification on polyimide.
Because PMDA-ODA (pyromellitic dianhydride-oxydianiline) polyimides have good heat resistance and are widely used in the production and processing process (Razdan et al., 2010), this study first uses quantum chemical calculation software to study the effect of plasma modification on the micro characteristics of polyimides under an applied electric field and then builds polyimides and plasma-modified polyimides with different proportions through molecular dynamics simulation to calculate the influence of the introduction of polar groups on the thermal stability, mechanical properties, and insulation performance.
Oly amine monomer model establishment and calculation
Polyimide molecular model establishment
The use of quantum chemical computing software in the establishment of polyamide monomers and plasma-modified polyamide monomers is shown in Figure 1 and Figure 2. Based on the key level analysis, we can know that polyamide molecular C-H, C-N key, N-H key, and C-C key are more likely to be destroyed. When the ionic body modifies, the molecular system will introduce -COOH and -OH groups. Based on this, it is selected for fracture. A C-N key on the ring, connecting the branch-OH group at C = O, adds an H at N. For a system with a large molecular weight, such as polyamide molecules, the use of semi-experience approximate calculation methods ignores certain points at the same time to replace some experience parameters instead of some points in the similar RHF equation and UHF equation (Zhang 2011), to ensure accuracy while shortening calculations time. Under the effect of adding electric fields, the Hamilton volume of the polyamide molecular system can be represented by the following formula:
Among them, H0 is the Hamilton when no external electric field is applied, and Hint is the Hamilton of the interaction between the electrical field and the molecular system. Under dipole approximation, the Hamiltonian corresponds to the interaction between the electric field intensity E and the polyimide molecular system and can be expressed as the following formula:
In the formula, μ is the molecular electric dipole moment (Wu, Yonggang et al., 2017; Yin, Wenyi et al., 2018).
Quantum chemical calculation software is used for theoretical calculation. The electric field intensity of the molecular system is gradually applied from 0 to 0.012 a. u. along the main direction of the x-axis with a gradient of 0.001 a. u. (1 a. u. = 5.142 ×1011v/m). The frequency and space optimization calculation of the polyimide molecular system is carried out by the PM6 Hamilton semi-empirical method. The stable structural characteristics of the two polyimides under the action of the external electric field are obtained.
Effect of the applied electric field on molecular energy
The external electric field at 0 ∼ 0.012 a. u. gradually increases, and the total energy change of the molecular system is shown in Figure 3. It can be seen from the figure that the total molecular energy of plasma-modified polyimide is lower than that of pure polyimide, and both are in a gradually decreasing trend. This is because the external electric field makes the internal electrons of polyimide shift toward the electric field, and the electric dipole moment μ gets bigger. According to Eq. 2, with the increase of the electric field and dipole moment, the potential energy value of Hamiltonian H increases numerically, and the total energy of the system decreases, that is, the total molecular energy decreases. With the gradual increase of electric field strength, the changing trend of the molecular dipole moment is shown in Figure 4. It can be seen from the figure that the dipole distance shows an upward trend, and there is also a dipole moment μ = q d (q is the number of charges and d is the distance between positive and negative charges). In this study, the charge of polyimide molecules will accumulate and become larger under the applied electric field. At the same time, d will also increase under the influence of the applied electric field, making the molecular dipole moment μ increase. At the same time, it can be seen from the figure that when no external electric field is applied, the dipole moment of the polyimide molecule is not 0, indicating that the polyimide molecule shows polarity and the plasma-modified polyimide has stronger polarity due to the introduction of polar groups.
Effect of the applied electric field on molecular orbital energy
With the increased applied electric field, the changing trend of the front-line orbital energy of polyimide molecules and plasma-modified polyimide molecules is shown in Figure 5 and Figure 6. With the increase in the applied electric field, the energy EL of the lowest space orbit (LUMO) gradually decreases, and the energy EH of the highest occupied orbit (HOMO) gradually increases in both molecular systems. Based on the front-line orbital theory, the larger the EL value, the smaller is the tendency of the molecular system to obtain electrons; the larger the EH value (Xu, Guoliang et al., 2012), the greater is the tendency of the molecular system to lose electrons. The changing trend of the energy gap EG is shown in Figure 7, where EG is calculated according to Eq. 3 (Li, Shixiong et al., 2020).
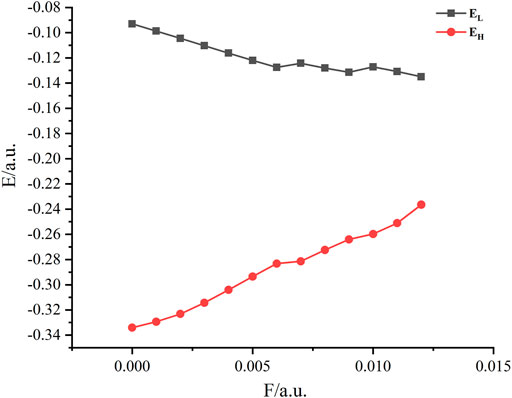
FIGURE 6. Variation of frontier orbital energy of the plasma-modified polyimide with electric field.
It can be seen from the figure that the molecular energy gap of the two polyimides decreases with the increase of the external electric field, and the energy gap of pure polyimides decreases faster. The smaller the energy gap, the more active the molecular chemical properties are, and the greater is the possibility of transition (Li 2016; Liang 2018). It can be concluded that the applied electric field will improve the electron transition ability in polyimide molecules and make the electrons excite from the originally occupied orbit to the empty orbit to form holes. When the electric field reaches a certain extent, it will affect the stability of the molecular structure and even lead to its destruction.
Establishment and calculation of the polyimide cell model
Polyimide cell model establishment
The aforementioned calculation results show that the chemical properties of plasma-modified polyimides are more stable, and their polarity is stronger than that of pure polyimides under the applied electric field environment. In the actual production process, polyimide usually exists in the form of a polymer. According to the literature, a polyimide molecular chain with a polymerization degree of 15 is established by molecular simulation software. At this time, the energy of the system is not stable, so it is necessary to use the Forcite module to minimize the energy to obtain the polyimide molecular chain, as shown in Figure 8.
Geometric optimization of the molecular chain was continued. An amorphous molecular model with a density of 1.34 g/cm3 was established under the conditions of temperature 300 K and pressure 0.1 MPa, and then, the structure of the model was optimized to obtain more reasonable polyimide periodic cells, as shown in Figure 9.
Using the same method, the plasma-modified polyimide molecule shown in Figure 2 was selected to establish periodic cells of plasma-modified polyimide with grafting ratios of 10%, 20%, and 30%, as shown in Figures 10A–C, respectively.
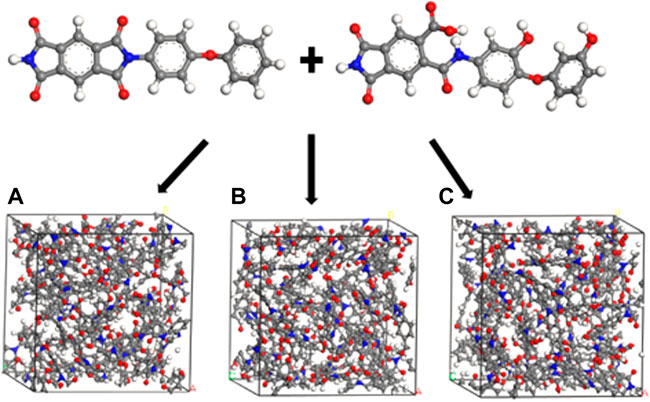
FIGURE 10. (A–C) are plasma modified polyimide models, the difference is that the proportions are different. (A) represents 10% modified proportion, (B) represents 20% modified proportion, and (C) represents 30% modified proportion, which has been mentioned in the paper, The title can serve as an overview.
Molecular dynamics simulation
At this time, the polyimide periodic model is still in an unstable state. The geometry optimization in the molecular dynamics module is used to optimize the structure and minimize the energy of the model. In order to avoid the influence of the potential depression of the local minimum value on the accuracy of the calculation process, the annealing treatment is carried out; under the pressure of 0.1 MPa, the temperature rises from 300 K to 800 K in the gradient of 20 K, the NPT ensemble is selected for one-time dynamic simulation, and then, the temperature is reduced from 800 K to 300 K under the same method for the second dynamic simulation. The structure of the model is optimized in each annealing treatment. After five cycles of annealing, the polymer molecular chain is further relaxed, and the structure of the system is more reasonable. The conformation with the lowest energy is selected for molecular dynamics simulation. First, 1ns dynamic simulation is carried out under an NPT ensemble to make the model temperature at 300 K, and then 500-ps dynamic simulation is carried out under an NVT ensemble. The configuration gives an output every 100 steps in the process. Finally, the trajectory file of the equilibrium dynamic process under the NVT ensemble is analyzed. The structure and properties of different plasma-modified polyimide systems were studied.
Free volume analysis
The internal volume of polymer materials is divided into the free body (VFV) and occupied volume (VOV). The volume of defects with atomic size and holes due to irregular stacking of atoms is the free volume, and the volume occupied by the atoms or molecules of the polymer material is the occupied volume. It is because of the existence of free volume that the molecular chain can adjust its conformation through rotation and displacement. According to the free volume theory and molecular motion theory (Fox and Flory, 1950) of Fox and Flory, holes are one of the important reasons for the movement of microparticles in materials. Therefore, the free volume theory can be used to explain the changes in thermal stability and mechanical properties of molecules in insulating materials.
The stacking capacity between polyimide molecules is characterized by calculating the fractional free volume (FFV), which is calculated by Eq. 4.
In this study, the Connolly surface of polyimide is calculated by using the Atom Volumes & Surfaces tool in molecular simulation software. The radius of the hard ball probe is the van der Waals radius of water, i.e., 0.145 nm. The Connolly surface diagrams of pure PI, 10% ion-modified PI, 20% ion-modified PI, and 30% ion-modified PI are calculated by using this method, as shown in Figures 11A–D, respectively. See Table 1 for specific data.
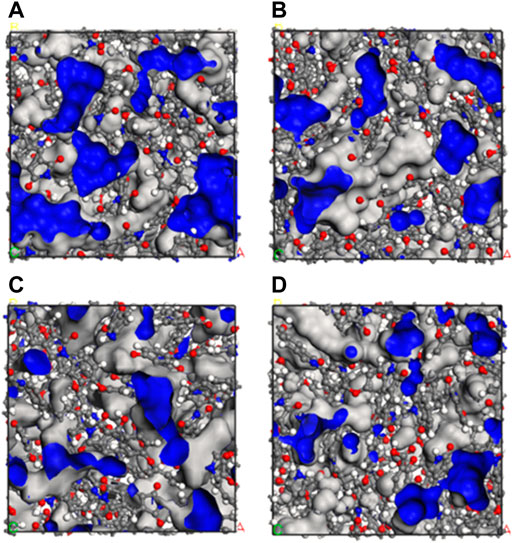
FIGURE 11. (A–D) are free volume diagrams of polyimide models. The difference is that (A) represents free volume diagrams of ordinary polyimide, (B) represents free volume diagrams of 10% plasma modified polyimide, (C) represents free volume diagrams of 20% plasma modified polyimide, and (D) represents free volume diagrams of 30% plasma modified polyimide, which have been mentioned in the paper, The title can serve as an overview.
In the figure, the blue part is the free volume of the material, and the gray part is the occupied volume of the material. It can be seen from Figure 11, and the data in the table that plasma modification will reduce the free volume of polyimide make the polyimide molecular chain more compact and orderly, and the free volume of polyimide with a 20% plasma modification rate is the smallest.
Polyimide chain movement
As a polymer, the movement of the polyimide chain will affect its mechanical properties and thermal stability. The stronger the kinematic ability of the polyimide chain, the worse will be the mechanical properties and thermal stability of the polyimide. Mean square displacement (MSD) refers to the deviation value of the position of the particle relative to the reference position after a certain evolution time, which can characterize the displacement capacity of the molecular chain segment in the model system during the process of molecular simulation. MSD is calculated by Eq. 5.
In the formula, Ri (t) and Ri (0), respectively, represent the position vectors of i molecule or atom at time t and initial time.
Figure 12 shows the mean square displacement curve of pure PI and PI with different plasma modification rates at 300 K. It can be seen from the figure that plasma modification will weaken the movement of molecular chains in the system as a whole, making the mechanical properties and thermal stability of polyimide more stable. This is consistent with the aforementioned conclusion that polyimide plasma modification will reduce the free volume fraction of the system.
Van der Waals force and cohesive energy density
The cohesive energy density (CED) can be used to judge the magnitude of intermolecular force. In general, the polarity of groups in molecules and intermolecular forces and the corresponding cohesive energy density show a positive proportional relationship. The cohesive energy density of polyimides of different systems is calculated by using the calculation task cohesive energy density task of the Forcite module under the modules directory. The results are shown in Table 2:
It can be seen from Figure 13 that because plasma modification introduces polar groups into the system, the cohesive energy density and van der Waals force of polyimide increase with the increase in the plasma modification ratio. When the modification rate is 20%, the van der Waals force of the polyimide is the highest. The greater the intermolecular force, the better will be the mechanical strength and heat resistance of the material.
Effect of plasma modification on mechanical properties of polyimides
The mechanical properties of materials can be characterized by elastic modulus. For polymer materials, good elastic modulus makes them suffer less wear under external stress such as mechanical vibration.
The static constant strain method was used to calculate the mechanical properties of the composites. Slight stress was applied to the equilibrium polyimide system to make it deform, and the stress–strain relationship was obtained. The polyimide in the simulation can be regarded as an isotropic material, so the stiffness matrix can be simplified to Eq. 6
In Eq. 6, λ and μ are the elastic constants, and the calculation method is shown in Eq. 7 and Eq. 8, respectively.
The mechanical properties in the Forcite module are used to calculate the mechanical properties of 20% plasma-modified polyimide, and the stiffness matrix is shown in Table 3.
The data on C11, C22, C33, C44, C55, and C66 in Table 3 are brought into Eq. 7 and Eq. 8 to obtain their elastic constants μ = 1.1386 and λ = 3.1149. The elastic constant is brought into Eq. 9, and the elastic modulus of 20% plasma-modified polyimide is 4.67 GPa.
The elastic modulus of pure polyimide, 10% plasma-modified polyimide, and 30% plasma-modified polyimide is calculated by the same method, as shown in the figure. It can be seen from Figure 14 that the plasma modification performance significantly improves the elastic modulus of polyimide. With the increase in the modification rate, the elastic modulus of polyimide shows an overall upward trend, and the elastic modulus of polyimide with a 20% modification rate is the largest.
It can be seen from Figure 14 that plasma modification will improve the mechanical properties of polyimide. In order to more intuitively analyze its impact on the performance of the model, external stress from 0 to 4 GPa is applied to the model along the x-axis direction. In the process, 0.05 GPa is used as a gradient, and the corresponding material strain under stress is recorded, as shown in Figure 15.
It can be seen from the figure that the strain produced by the pure polyimide and the plasma-modified polyimide models with different proportions under the action of external stress shows an increasing trend, and the strain variation range is relatively stable when the stress is small. With the increase in external stress, the material gradually deforms greatly, and the pure polyimide model should change greatly. It is demonstrated that plasma modification can enhance the elastic modulus of polyimide.
Relative permittivity
Under the action of the external electric field, the medium will induce a charge inside the medium, which will weaken the external electric field. At this time, the ratio of the external electric field to the induced electric field in the medium is the relative permittivity ε. It is commonly used to indicate the polarization level of a dielectric, usually ε; the larger the dielectric, the stronger the polarity is. Dielectric polarization is the phenomenon in which the dielectric produces the elastic displacement of the bound charge and the steering displacement of the dipole under the action of electricity. It is mainly divided into electron displacement polarization, ion displacement polarization, steering polarization, and space charge polarization. In this study, the dielectric constant is calculated by calculating the fluctuation of the dipole moment through writing the Perl script language program, that is, considering the charge imbalance of polar molecules; each model is run for 50ps under the NVT ensemble, and then, the dipole moment of the simulation system is obtained as the output. The relative dielectric constant is calculated through the following formula:
Here, M is the output dipole moment of each step, V is the volume of the box, T is the temperature, K is the Boltzmann constant, and ε0 is the vacuum dielectric constant. Taking the PI model as an example, the calculated dielectric constant data are shown in Table 4:
According to the data, the dipole moment can be calculated, and the relative dielectric constant of PI is 3.31, which is consistent with the experimental results in reference (Zhou, 2020), indicating the rationality of the calculation results. The dielectric constants of plasma-modified polyimide with different modification ratios are also calculated by the Perl script, as shown in Figure 16.
Due to the introduction of oxygen-containing and nitrogen-containing polar groups, the dielectric constant of plasma-modified polyimide increases with the increase of the modification rate, which is also consistent with the stronger polarity of polyimide after modification.
Conclusion
1) Quantum chemical calculation software was used to calculate the energy and energy gap between polyimide and plasma-modified polyimide under an applied electric field. After ionic modification, the molecular energy of polyimide is lower, the energy gap is smaller, and the molecular structure and properties are more stable under the applied electric field.
2) Since the polar groups are connected to the polyimide molecular chain by plasma modification, part of the free volume in the original system is occupied. After modification, the free volume of the polyimide decreases, the interaction force between the polyimide molecular chains increases, and the movement capacity of the molecular chain decreases. Moreover, when the modification rate is 20%, the free volume fraction is the smallest.
3) Compared with pure polyimide, the mean square displacement of the molecular chain of plasma-modified polyimide is also limited, and when the modification ratio is 20%, the mean square displacement is the smallest. Plasma modification can effectively inhibit the movement of the molecular chain of polyimide, thus improving the thermal stability of the polyimide system.
4) Plasma modification can effectively improve the mechanical properties of polyimide, and when the modification ratio is 20%, the maximum elastic modulus of polyimide is 4.67 GPa. According to the stress–strain curve, it can be seen that under the same applied stress, the pure polyimide has a large corresponding stress change, and the structure is more vulnerable to be affected.
Data availability statement
The raw data supporting the conclusions of this article will be made available by the authors, without undue reservation.
Author contributions
All authors listed have made a substantial, direct, and intellectual contribution to the work and approved it for publication.
Conflict of interest
The authors declare that the research was conducted in the absence of any commercial or financial relationships that could be construed as a potential conflict of interest.
Publisher’s note
All claims expressed in this article are solely those of the authors and do not necessarily represent those of their affiliated organizations, or those of the publisher, the editors, and the reviewers. Any product that may be evaluated in this article, or claim that may be made by its manufacturer, is not guaranteed or endorsed by the publisher.
References
Fang, Zhi., Yang, Hao., Si, Qiong., Zheng, Xiangyang., and Xie, Xiangqian. (2010). Improvement of surface energy of solar panel back film by dielectric barrier discharge treatment. High. Volt. Technol. 02, 417–422. doi:10.13336/j.1003-6520.hve.2010.02.024
Fox, T, G., and Flory, P, J. (1950). Second order transition temperatures and related properties of polystyrene. I. Influence of molecular weight. J. Appl. Phys. 21 (6), 581–591. doi:10.1063/1.1699711
Gao, Mengyan., Wang, Changou., Jia, Yan., Zhai, Lei., Mo, song., he, Minhui., et al. (2021). Research and progress on anisotropic thermal conductivity of polyimide film. Mater. J. Polym. 10, 1283–1297. doi:10.11777/j.issn1000-3304.2021.21094
Gong, Y., Qing, Li., Tian, J., and Zushun, Xu. (2018). On preparation and properties of hyperbranched Co-polyimide/ZnO composite film. Adhesion. doi:10.3969/j.issn.1001-5922.2018.10.003
Hu, Wen., Li, Li., Liu, Yunlong., Liu, Lun., Ge, Yafeng., and Lin, Fuchang. (2015). Experimental study on current characteristics of atmospheric pressure air dispersion discharge under nanosecond pulse excitation. Chin. J. Electr. Eng. 16, 4263–4270. doi:10.13334/j.0258-8013.pcsee.2015.16.031
Huang, Xuwei., Li, Qingmin., and Zou, Liang. (2020). TiO in polyimide nanocomposite insulation system study on the microscopic mechanism of particle agglomeration. Insul. Mater. 02, 14–21. doi:10.16790/j.cnki.1009-9239.im.2020.02.003
Jia, M., Li, Y., He, C., and Huang, X. (2016). Soluble perfluorocyclobutyl aryl ether-based polyimide for high-performance dielectric material. ACS Appl. Mat. Interfaces 8 (39), 26352–26358. doi:10.1021/acsami.6b09383
Jia, Yanjiang., An, Yuancheng., Zhang, Yan., Zhi, Xinxin., Zhang, Xiumin., Liu, Jingang., et al. (2021). Preparation and properties of polyimide films modified by nano colloidal silica. Insul. Mater. 11, 100–107. doi:10.16790/j.cnki.1009-9239.im.2021.11.014
Kausar, Ayesha. (2017). Progression from polyimide to polyimide composite in proton-exchange membrane fuel cell: A review. Polym. Plast. Technol. Eng. 56, 1375–1390. doi:10.1080/03602559.2016.1275688
Li, Tao. (2016). Molecular design of organic thermoactive delayed fluorescent materials. Heilongjiang, China: Harbin Institute of Technology. [master's thesis][Heilongjiang (Harbin)].
Li, Tong. (2019). Molecular dynamics simulation of the effect of plasma modification on polyimide [master's thesis]. [Sichuan (chendu)]. Chengdu, Sichuan, China: Southwest Jiaotong University.
Li, Bin., Li, Pengyu., Wen, Weijie., and Liu, Haijin. (2022). Performance analysis and resonant commutation method of mechanical DC circuit breaker. J. Electrotech. 9, 2139–2149. doi:10.19595/j.cnki.1000-6753.tces.211871
Li, S., Chen, D., Zhang, Z., long, Z., and Qin, H. (2020). Circular C18 ground state properties and excitation characteristics under external electric field. Acta Phys. Sin. 10, 103101–103176. doi:10.7498/aps.69.20200268
Liang, Yidan. (2018). Study on evaluation method of electrical performance of SF6 substitute gas based on density functional theory [master's thesis]. Hunan, China: Hunan University. [Hunan (Changsha)].
Ohya, H., Kudryavtsev, V, V., and Semenova, S, I. (1997). Polyimide membranes: Applications, fabrications, and properties. Desalination 109 (2), 225. doi:10.1016/S0011-9164(97)00067-2
Pan, Yuan., Yuan, Zhao., Chen, Lixue., Xu, Min., and Liu, Liming. (2018). Research on coupled mechanical high voltage DC circuit breaker Chinese. J. Electr. Eng. 24, 7113–7120+7437. doi:10.13334/j.0258-8013.pcsee.181724
Razdan, M., Entenberg, A., Debies, T., Parekh, B., Rai, P., and Takacs, G, A. (2010). Surface modification of poly(biphenyl dianhydride-para-phenylene diamine) (BPDA-PDA) and poly(pyromellitic dianhydride-oxydianiline) (PMDA-ODA) polyimides with UV photo-oxidation. J. Adhesion Sci. Technol. 24 (6), 1153–1166. doi:10.1163/016942409X12598231568465
Seyedzavvar, M., Boga, C., Akar, S., and Pashmforoush, F. (2021). Molecular dynamic approach to predict thermo-mechanical properties of poly (butylene terephthalate)/CaCO3 nanocomposites. Mater. Today Commun. 28, 102602. doi:10.1016/j.mtcomm.2021.102602
Takari, A., Ghasemi, A. R., Hamadanian, M., Sarafrazi, M., and Najafidoust, A. (2021). Molecular dynamics simulation and thermo-mechanical characterization for optimization of three-phase epoxy/TiO2/SiO2 nano-composites. Polym. Test. 93, 106890. doi:10.1016/j.polymertesting.2020.106890
Wu, Yonggang., Luo, Ya., and LingHu, Rongfeng. (2017). Study on molecular characteristics and potential energy function of CO under external electric. Field. J. Sichuan Univ. Nat. Sci. Ed. 54 (5), 6. doi:10.3969/j.issn.0490-6756.2017.05.019
Xu, Guoliang., Xie, Huixiang., Yuan, Wei., Zhang, Xianzhou., and Liu, Yufang. (2012). Luminescence characteristics of SiN molecules under external electric field. J. Phys. 04, 101–106. doi:10.7498/aps.61.043104
Xu, Jingcheng., Dai, Sichang., Li, Haoliang, and Yang, Junhe. (2018). Molecular dynamics simulation of the thermal conductivity of graphitized graphene/polyimide films. New carbon Mater. 03, 213–220. doi:10.1016/S1872-5805(18)60336-1
Yang, Jiaming., Zhao, Hong., Zheng, Changji., Guo, Xiaofei., Wang, Xuan., Han, Baozhong., et al. (2018). Moisture adsorption mechanism of nano MgO/LDPE and SiO2/LDPE composite media and its effect on DC conductivity. J. Compos. Mater. 07, 1832–1840. doi:10.13801/j.cnki.fhclxb.20171114.008
Yang, Xinyu., Xie, Jun., Fu, Kexin., Ge, Jingyuan., Zhou, Huahao., Yang, Xueming., et al. (2020). Influence mechanism of an hydride/amine curing agent and doped nano SiO2 on thermodynamic properties of epoxy resin. Insul. Mater. 12, 20–26. doi:10.16790/j.cnki.1009-9239.im.2020.12.004
Yin, Wenyi., Liu, Yuzhu., Zhang, Xiangyun., Li, Bingsheng., and Qin, Chaochao (2018). Study on physical properties and spectra of SiC in radiation field. J. atomic Mol. Phys. 01, 1–6.
Yuan, Z., Zhang, X., Wang, Y., Nie, W., Yin, J., Ma, S., et al. (2021). Thermophysical properties of the binary mixtures of iso-octane with methyl hexanoate, n-decane with methyl decanoate and methyl octanoate: Experimental investigation and molecular dynamic simulation. Fluid Phase Equilibria 544-545, 113099. doi:10.1016/j.fluid.2021.113099
Zhang, Weiguo (2011). Theoretical study on the structure and adsorption performance of TS-1 molecular sieve [master's thesis] [shandong (qing dao)]. Qingdao, Shandong, China: Qingdao University.
Keywords: polyimide, molecular dynamics simulation, thermal stability, mechanical properties, relative permittivity
Citation: Ding C, Chen C, Yuan Z and Chen L (2022) Molecular simulation of the effect of plasma modification on the microscopic properties of polyimide. Front. Mater. 9:1018882. doi: 10.3389/fmats.2022.1018882
Received: 14 August 2022; Accepted: 02 September 2022;
Published: 29 September 2022.
Edited by:
Xuetong Zhao, Chongqing University, ChinaCopyright © 2022 Ding, Chen, Yuan and Chen. This is an open-access article distributed under the terms of the Creative Commons Attribution License (CC BY). The use, distribution or reproduction in other forums is permitted, provided the original author(s) and the copyright owner(s) are credited and that the original publication in this journal is cited, in accordance with accepted academic practice. No use, distribution or reproduction is permitted which does not comply with these terms.
*Correspondence: Zhao Yuan, hustyuanzhao@qq.com