- 1Department of Burn and Plastic Surgery, Shanghai Changzheng Hospital, Naval Medical University, Shanghai, China
- 2Department of Plastic Surgery, Naval Medical Center, Naval Medical University, Shanghai, China
- 3Changhai Clinical Research Unit, Shanghai Changhai Hospital, Naval Medical University, Shanghai, China
Radiation-induced damages are difficult to heal than normal wounds. This is because radiation therapy could lead to an imbalanced inflammatory response, oxidative stress response, lack of angiogenesis, and a high risk of bacterial infection, which are considered to be the greatest challenges in radiation-induced damages treatment. Hydrogels are semi-occlusive and are composed of complex hydrophilic polymers with a higher water content. Due to their excellent mechanical and biochemical property (such as adhesiveness, antibacterial, and antioxidant abilities), hydrogels represent a promising strategy that has achieved potent therapeutic efficacy in the treatment of radiation-induced skin and mucosa damages. This review systematically and comprehensively summarizes the recent progress of various types of hydrogels such as natural biopolymer, synthetic polymer, composite hydrogels and commodity dressings for the treatment of radiation-induced skin and mucosa damages.
1 Introduction
Radiation therapy or radiotherapy (RT) has become an important treatment method for malignant tumours, including adjuvant therapy after radical tumour resection or palliative RT (Benson et al., 2016). In recent years, more than 50% of patients with malignant tumours have received RT (Abshire and Lang, 2018). The ultimate goal of RT is to induce tumour death, but it inevitably affects the surrounding healthy tissue. Skin and mucosa damages, ranging from moderate to severe, have occurred in more than 95% of patients receiving RT (Joseph et al., 2021). The main characteristics of radiation-induced skin and mucosa damages are hyperplasia and high oxygen demand. Generally, skin damages caused by RT are called radiation dermatitis, and those with skin defects are called radiation-induced skin wounds; these are divided into acute and chronic stages according to the course of the disease (Bray et al., 2016). In addition to radiation-induced skin wounds, for patients with head, neck, pelvic or prostate tumours, radiation also cause mucosal damages of the oral and gastrointestinal. Since the early 20th century, these radiation-induced damages have been gradually recognized, analyzed, and treated scientifically (Haruna et al., 2017). The influence of radiation-induced damages further affected the daily or postoperative quality of life of patients with malignant tumours. Severe injuries led to the interruption of RT for patients and also affected patient prognosis (Meghrajani et al., 2013).
Dressings applied to radiation-induced damages have evolved over time with considerable progress. In the early days, dry dressings were used clinically to keep the wounds dry and thus avoid bacterial infection; at that time, the theory of dry wound healing was the mainstream theory guiding treatment (Han and Ceilley, 2017). However, this type of passive dressing, cannot continuously provide drugs for the wound, nor can it respond to the various changes in the wound conditions (Francesko et al., 2018). Continuous development of the wet wound healing theory has shifted the focus of wound dressing applications from traditional non-functional dry dressings to modern new dressings (Cao and Gao, 2021; He et al., 2021).
Emerging novel functional wound dressings, such as hydrogels, hydrocolloids, nanofibers, and foam dressings, have shown excellent effects in the treatment of radiation-induced damages (Kang and Park, 2021). As a representative novel biomaterial, hydrogels have shown great advantages in radiation-induced damages treatment and have received widespread attention. Its excellent effect has been fully verified in many studies (Xu et al., 2020). Compared to traditional dry dressings and other types of modern dressings, natural biopolymer, synthetic polymer, composite hydrogels, and some commodity dressings have become the best candidates for radiation-induced damages because of their unique properties (Klotz et al., 2016). As shown in Figure 1, on the basis of introducing the pathologic causes of radiation-induced damages, this review outlined the latest research progress of multiple hydrogels as treatment for radiation-induced skin and mucosa damages (Macmillan et al., 2007). Finally, the challenges and future development prospects of hydrogels as applied to radiation-induced damages were summarized.
2 Radiation-induced skin and mucosa damages
RT is a basic adjunctive therapy for malignant tumour, which could make the treated area of the skin and mucosa extremely physiologically vulnerable and even cause wounds that are difficult to heal for a long time (Hymes et al., 2006). Radiation-induced skin damages, also called radiation dermatitis, is skin inflammation, defect, or breakage caused by repeated RT. It can also be called radiation-induced skin wounds when there is severe skin damages. In addition to radiation-induced skin damages, for patients with head, neck, pelvic, or prostate tumours, radiation also causes tissue injury, resulting in radiation-induced mucosal damages (Sonis, 2021). Mucositis occurs mainly in the oral and gastrointestinal tract, causing pain, eating and digestive disorders, and local infections (Sroussi et al., 2017). Although the incidence and severity of radiation-induced skin and mucosa damages have decreased with the development of technology and treatment, it is still a significant adverse reaction to RT (Meghrajani et al., 2013; Beach et al., 2020). Clinically, approximately 95% of patients who received RT developed some degree of radiation-induced skin and mucosa damages, up to 85% of these patients developed moderate to severe dermatitis (Hegedus et al., 2017), and mucositis occurred in nearly 90% of patients with head and neck cancer who received RT (Maria et al., 2017). Patients with different grades and stages have great differences in disease severity, duration, and prognosis. In addition, patients with severe dermatitis and mucositis have to reduce radiation doses and delay cancer treatment in order to avoid further development of damage.
2.1 Symptoms and disease staging
Skin damages are classified as acute or chronic diseases. Acute radiation-induced skin damages usually refer to skin changes that occur within 90 days of receiving radiation exposure (Brunt et al., 2016). The National Cancer Institute has graded acute damages by symptom severity as shown in Table 1. It is divided into four stages (Hymes et al., 2006). Grade 1 skin damages include mild systemic erythema, dry desquamation, and hair loss (Martin and Nunan, 2015). This early erythema of the skin usually subsides within a few days. This skin alopecia and scaling are usually secondary to the RT effect on the sebaceous glands and hair follicles. Grade 2 skin damages present with mild to severe erythema and patchy desquamation at the skin folds. Grade 2 injuries usually occur 4–5 weeks after receiving RT, with radiation doses of 40 Gy or higher (Seegenschmiedt, 2006). Grade 3 skin damages consist of moist desquamation that occurs outside the skin folds, accompanied by pitting edema and minor traumatic hemorrhage. The main features of moist desquamation are skin necrosis, wound fibrin exudate, and pain. These injuries usually peak at 1–2 weeks after receiving the last RT, after which they heal gradually. It is very important to prevent and treat wound infection throughout the period, from the appearance of grade 3 injuries to healing (Straub et al., 2015). The formation of ulcers, bleeding, and necrosis is indicative of grade 4 damages (Censabella et al., 2014). Grade 4 skin injuries include full-thickness skin necrosis and ulceration with or without spontaneous wound bleeding. Wounds are susceptible to infection due to epidermal necrosis and defects. When grade 4 injuries appear, it is clinically necessary to pause RT until the radiation area re-epithelializes and heals. If the radiation-induced acute skin damages are treated improperly and the damage does not resolve, it can lead to gradual secondary chronic skin ulceration, fibrosis, and even tissue necrosis in the injured area (Robijns et al., 2021).
Chronic radiation-induced skin damages refer to skin injuries that persist for more than 90 days after the completion of RT (Hegedus et al., 2017). As shown in Table 1, histological changes in chronic injuries include dermal atrophy, thinning of the epidermis, and vascular damage; the main symptoms include local telangiectasia, severe pigmentation, skin necrosis, ulceration, and fibrosis (Bray et al., 2016). In addition, RT increases the risk of nonpigmented cutaneous malignancies, such as basal cell carcinoma, keratoacanthoma, and squamous cell carcinoma, which could occur in radiation-damaged areas, particularly in patients who are younger and have a longer duration of radiation exposure (Straub et al., 2015; Spałek, 2016).
Many mucosa damages after RT are usually mild and reversible and there is no definite clinical staging and grading of it. Oral mucositis typically appears 1 week after RT exposure and manifests early as acute inflammation of the oral mucosa, tongue, and pharynx. In some cases, the location of the tumour is too close to critical organs to provide enough space protecting to the surrounding organs while preserving the therapeutic dose (Karsh et al., 2018). Especially for patients with prostate and gynecological cancers, RT results in gastrointestinal mucosa side effects, such as abdominal pain, diarrhea, abdominal distention, rectal bleeding, and other symptoms, requiring dose reduction or even interruption of treatment, with a negative impact on later treatment (Hamstra et al., 2017).
2.2 Effects of RT on wound healing
Wound healing results when a series of cells and secreted cytokines work together at various locations, over a time period; and is considered one of the most complex biological processes (Han and Ceilley, 2017). However, due to continuous radiation damage, the healing process of radiation-induced skin and mucosa wounds is more complex and refractory than that of normal wounds, posing a significant challenge to clinical work. The first dose of RT immediately causes tissue damage and produces short-lived free radicals that cause irreversible breaks in biomolecules, including cell DNA, lipids of the cytomembrane, proteins, and carbohydrates, resulting in an inflammatory response (Singh et al., 2016; Ferreira et al., 2017). Persistent damage caused by repeated exposure to radiation does not allow cells and tissues to repair as planned, further leading to the loss of the protective barrier of the skin and mucosa (Schaue et al., 2015). Unlike other forms of physical or chemical skin injuries, repetitive radiation injuries disrupt the highly ordered wound healing process, leading to repeated inflammatory responses, persistent excessive reactive oxygen species (ROS) stimulation, capillary depletion, and persistent infections (Seegenschmiedt, 2006).
2.2.1 The imbalance in the inflammatory response
The pathogenesis of radiation-induced skin and mucosa damages involves direct radiation injuries and a series of inflammatory responses caused by radiation and the injuries themselves. This affects the normal physiological function of tissues and cells, and disrupts the wound healing process (Jagsi et al., 2018). Each subsequent dose of radiation produces a greater inflammatory response, leading to inflammatory cell recruitment and excessive release of inflammatory factors (de Oliveira et al., 2016; DiPietro et al., 2021). At present, it is believed that the imbalance of the inflammatory response is the main reason why radiation-induced skin damages take longer to heal than normal wounds (Hamidzadeh et al., 2017). In addition to inflammatory cells, the early inflammatory response within radiation-induced skin damages is mainly regulated and progressively amplified by a series of proinflammatory cytokines (tumour necrosis factor [TNF]-a, interleukin [IL]-1, IL-3, IL-5, IL-6), chemokines (IL-8, CC receptor, eotaxin), receptor tyrosine kinase, and adhesion molecules (vascular cell adhesion molecule [VCAM], intercellular adhesion molecule [ICAM]-1, E-selectin) (Browne and Pandit, 2015; Lierova et al., 2018).
2.2.2 The high oxidative stress response
The high risk of RT to the skin and mucosa is mainly related to the free radicals produced by radiation, which lead to a skin cascade reaction, inducing more free radical production and accumulation, and causing tissue damage (Pizzino et al., 2017). This is because radiation can interact with water (which constitutes approximately 70% of body tissues) and produce multiple ROS, which persistently damages DNA and the cell membrane and induce apoptosis (Lee et al., 2017a). In addition, excessive production of hydroxyl radicals results in inflammatory dysregulation and high oxidative stress in the microenvironment, which completely verwhelms the protective capacity of the body’s protective antioxidant system; thereby delaying wound healing (Choudhury et al., 2017). ROS, with a very high oxidation capacity, are extremely important factors in wound healing, and their role is wide-ranging, from initial stimulation of the immune response to triggering redux-dependent intracellular oxidation, as well as triggering the cell’s signaling pathways to fight bacterial infection (Pérez-Torres et al., 2021). Therefore, proper ROS levels are important for promoting wound tissue repair. However, in radiation-induced skin and mucosa wounds, tissue damage caused by free radicals leaves excessive ROS accumulation, causing high levels of oxidative stress response, leading to fracture damage to cell membrane lipids, important proteins, and nucleic acids in early wounds, and ultimately affecting wound healing (Singh et al., 2016).
2.2.3 The lack of angiogenesis
RT leads to endothelial cell and vascular damage. Endothelial cells contain extremely high levels of ceramide synthase and acidic sphingomyelinase, a large amount of which produce ceramides by radiation in the cell, resulting in subsequent apoptosis and microvascular endothelial cell loss (Lee et al., 2013). Studies have shown that even lower doses of radiation could induce endothelial ceramide production, inducing apoptosis of bovine aortic endothelial cells (Nagane et al., 2021; Tapio et al., 2021). At the same time, inadequate blood supply is a key problem resulting in the difficulty of radiation-induced skin and mucosa wounds healing, mainly manifested by the lack of micro-vascularization and delayed granulation tissue formation (Paderno et al., 2016). During normal wound healing, a disordered but abundant network of microvascular vessels forms early at the wound site and is usually denser than the normal tissue before the injury (Sheng et al., 2021). However, contrary to the normal wound healing process, studies have shown that endothelial cell loss reaches 20% within 24 h of RT, and wound perfusion decreases by 46.5% 3 days after RT (Wang et al., 2016a; Ramadan et al., 2021; Sárközy et al., 2021). Angiogenesis disorders tend to further cause the worsening of wounds, so these deserve our attention, in the treatment of radiation-induced skin and mucosa damages.
2.2.4 The invasion of pathogenic bacteria
Radiation-induced skin and mucosa wounds are more susceptible to bacterial infection than ordinary wounds during the healing process because the three pathological factors above could impair the integrity of the physical barrier while destroying the immune function. Radiation-induced skin and mucosa wounds are often considered well-suited for pathogen colonization, and also constitute the initial step for severe infection. The open sores and decreased immunity tend to induce deep tissue necrosis (Daeschlein, 2013). Although the infection cannot be regarded as being of internal cause, leading to the formation of radiation-induced skin and mucosa wounds, infection without intervention would synergistically prolong the progress of wound healing, resulting in wound deterioration and decreased quality of patient life (Li et al., 2018; Fan et al., 2021).
2.3 Prevention and treatment strategies
For patients who need multiple RT, the prevention of radiation-induced skin and mucosa wounds is the most important factor that need more attention paid in clinical practice. Skin protection includes washing with warm water and mild soap before and after RT to keep the skin clean; and using a water-based moisturizer after cleaning to avoid excessive dryness (Campbell and Illingworth, 1992; Roy et al., 2001). The use of moisturizer, gel, or wound dressing at the RT site can cause a bolus effect, so it should be used after each RT. Avoiding sun exposure and using metal-containing topical products can prevent RT related complications. The protection of the oral mucosa mainly includes keeping the mouth clean and using mouthwash regularly. The pathogenesis for the radiation-induced gastrointestinal mucosa damages is that the location of the tumour is too close to the key organs, which cannot provide enough space to protect the surrounding organs. Therefore, the use of spacer can effectively prevent intestinal mucosal injury, which was first reported in 1984 (Fischer-Valuck et al., 2017).
In patients with radiation-induced grade 1 skin damages, the symptoms can be effectively alleviated by the general prevention as shown above. After cleaning, the use of hydrophilic moisturizer can solve the symptoms including dry peeling. Topical corticosteroid is a common treatment for grade 1–2 skin damages, which can effectively solve the skin itching, irritation, burning and other uncomfortable symptoms of patients. It is recommended to use it once a day after each RT to reduce the further development of dermatitis (Omidvari et al., 2007). For wet desquamation in patients with grade 2–3 skin damages, hydrogel or hydrocolloid dressings are usually used for wound management (Bray et al., 2016). The application of dressings can absorb the excess exudate in the wound and maintain a moist environment, thus promoting healing. For patients with grade 3–4 skin damages such as defects or necrosis of epidermal, dressing application is the most basic and effective treatment method (Brunt et al., 2016). The functional dressings according to various pathogenic factors have endlessly emerged, and many research results have shown that they accelerate wound healing and improve patient comfort, this content with more details will be discussed in the next section. Significant full-thickness skin necrosis and ulceration occur in patients with grade 4 skin damages. Treatment includes multidisciplinary management and cessation of RT. Surgical debridement removing necrotic tissue, full-thickness skin or pedicle flaps grafts are the most important surgical treatment (Censabella et al., 2014). These severe acute skin toxic reactions may progress to chronic, developing into fibrosis, nonunion ulcers, and even transforming into malignant, requiring sequential surgical resection, reconstruction, and even amputation.
3 Advances in hydrogel dressings in radiation-induced skin and mucosa damages
Using traditional wound dressings, topical corticosteroids, oral antibiotics, and necrotizing skin debridement are common methods for the clinical treatment of radiation-induced skin and mucosa damages (Tao et al., 2019). Although many advanced treatment strategies for promoting radiation-induced wounds healing have been extensively explored, such as stem cell therapy, cytokine therapy, hyperbaric oxygen therapy, and low-intensity laser therapy, traditional dressing is still the most commonly used treatment strategy in clinical practice (Park et al., 2017; Montay-Gruel et al., 2019). In contrast to traditional dry wound repair strategies, some novel biomedical materials, which mimic the natural extracellular matrix (ECM), could support and promote the in vitro growth of wound cells by creating a similar in vivo environment (Wasiak et al., 2013; Wang et al., 2021). These dressings provide a moist environment for the wound surface, allowing fibroblasts and epithelial cells involved in tissue repair to migrate and proliferate rapidly, thereby promoting wound healing (Song et al., 2012).
Modern biomedical material dressings are mainly classified according to the material and are divided into foam, hydrogel dressings, and so on (Wasiak et al., 2013). Hydrogels are three-dimensional network structural gel with extremely high moisture content (Sun et al., 2018). Its hydrophilicity depends on the degree of cross-linking of polar functional groups. The three dimensional mesh structure of hydrogels gives them excellent water absorption and water retention characteristics, thereby maintaining a long-term moist environment for wounds (Sivaraj et al., 2021). At the same time, the porous mesh structure of the hydrogels is also conducive for water vapor exchange in the wound and for the delivery of nutrients, thus promoting wound healing (Dimatteo et al., 2018). Moreover, hydrogels are biocompatible and can mimic the natural ECM environment, providing a suitable environment for cell proliferation and migration (Saldin et al., 2017; Xu et al., 2020). Although most hydrogel dressings lacked sufficient antimicrobial capacity, and long-term bacterial infections might form a protective biofilm on the wound surface, further enhancing resistance and preventing the infiltration of antibiotics. Hydrogel dressings could prevent microbial aggregation and inhibit bacterial growth and infection by adjusting the amount of charge in hydrogels or combining with nano-antibacterial metal particles (Zhang et al., 2020). Hydrogels are also ideal wound dressings for radiation-induced skin and mucosa damages, and after some technical modification getting convenient to use and remove, preventing secondary damage to the wounds. Since the application of hydrogels for wound dressings was reported in 1989, researchers have been improving their performance to address a variety of clinical issues (He et al., 2021). Various types of hydrogels have been reported to meet the requirements of all aspects of the treatment. The hydrogels that have been used for radiation-induced skin and mucosa damages could be divided into natural biopolymer, synthetic polymer, composite hydrogel, and some commodity dressings. In the following, we introduced the definition, application scenarios, main application cases, and treatment mechanism of hydrogels in radiation-induced skin and mucosa wounds. Brief information about hydrogels highlighted in this article is shown in Table 2.
3.1 Natural biopolymer hydrogels
Compared with synthetic polymer hydrogels, natural biopolymer hydrogels have great application advantages, mainly in good biocompatibility and biodegradability, no immune and toxic reactions, and the final metabolites could be discharged from the body (Elkhoury et al., 2021). Polysaccharide polymer, mainly including chitosan, sodium alginate, and glycosaminoglycan, is very suitable for the preparation of hydrogels used in radiation-induced skin and mucosa damages. The polysaccharide material is important being a natural polymer that widely exists in nature, in addition to the above advantages it is also highly degradable under conditions of enzyme catalysis or by some specific organisms (Karoyo and Wilson, 2021). The degradation products are usually monosaccharides or disaccharides, which are not only biocompatible and easily absorbed, but could even provide energy for living organisms (Saldin et al., 2017). There are many functional groups in the molecular structure of natural polysaccharides, including carboxyl, hydroxyl, and amino groups, so that they can further expand their biological functions through modification under mild conditions (Voci et al., 2021). At present, natural polysaccharide hydrogel materials have become one of the most important research topics in the field of radiation-induced skin and mucosa wounds.
3.1.1 Alginate hydrogels
Alginate is a kind of negatively charged natural polysaccharide, which is mainly extracted from brown seaweed and widely used in biomedical wound repair field. It is composed of M unit containing β-1, 4-D-mannuronic acid and G unit containing α-1, 4-L-Guluronic acid with glycosidic linkage in different proportions (Aderibigbe and Buyana, 2018). Alginates containing different proportions of M and G unit have different physical properties and molecular weight (Andersen et al., 2015). For example, the immunogenicity of alginate is affected by the content of M unit, and it can induce more cytokine production compared with alginate containing higher G unit (Beaumont et al., 2021). The unique properties of alginate make it widely used in radiation-induced skin wounds treatment. It overcomes the problems existing in the traditional wound dressings currently used, such as enhancing the absorption of wound exudate, reducing bacterial infection, reducing adverse allergic reactions, and promoting wound healing (Sun and Tan, 2013). Many studies on alginate hydrogels have demonstrated its great potential in wound repair, such as the dressing designed by Straccia et al. (2015), which coated chitosan onto alginate hydrogels, achieved excellent antibacterial function. Alginate also shows excellent hemostatic properties and can be applied to bleeding wounds (Arlov and Skjåk-Bræk, 2017).
Because alginate carries negative charges, its gelation usually requires binding with divalent cations, and this ability allows it to interact with cationic polyelectrolytes and proteoglycans through electrostatic interaction (Beaumont et al., 2021). This electrostatic action has been applied to the cationic drug and molecular transfer system, but it also has certain disadvantages, for example, when used as radiation-induced skin wound dressings, it easily absorbs proteins and harmful organisms, and sticks to the damaged tissue, causing secondary damage when the dressing is replaced (Aderibigbe and Buyana, 2018). In contrast to alginate hydrogels, balanced charged hydrogel, in addition to super-hydrophilicity and other advantages, have anti-biological contamination performance due to its neutral charge advantage, becoming a novel non-stick hydrogel dressing. Some neutral charge materials have shown better anti-wound contamination ability in vitro experiments (Zhu et al., 2017). Wu et al. (2018) synthesized ultra-low-fouling zwitterionic sulfated poly (sulfobetaine methacrylate) (polySBMA) hydrogels that balanced hydrogel charge which reduced wound inflammation, promoted angiogenesis, accelerated epithelialization. This hydrogel had excellent swelling ratios, prolonged equilibrium times and good water retention effect. Animal experiments showed that after the 17th day, the wounds treated with SBMA were basically healed with less scar compared with the control group. The wound closure rate of SBMA treated mice was 98.5%, which was significantly higher than that of other groups proved the effectiveness of promoting wound healing.
In order to obtain a dressing with the advantages of both hydrogels for radiation-induced skin wounds, Zhang et al. (2021) designed a neutrally charged hydrogel based on oppositely charged polymers, in which, alginate and hyaluronic acid (HA) were negatively charged, and the water-soluble polymer polylysine (PLL) with positive charge was added to balance the charge ratios (Figure 2A). Adjusted the proportion of each component made the zeta potential of it was close to zero and all of them exhibited high water content values (>97%). Few fluorescence and bacteria was observed on the surface of hydrogel in fluorescent protein adsorption test and E. Coli and S. aureus adhesion tests, shown the effectiveness of resisting the adhesion of proteins and bacteria (Figures 2C,D). As mentioned above, abnormal oxidative stress in radiation-induced damages is one of the urgent pathological factors to be solved. Ryan et al. (2013) found that for breast cancer patients receiving chemotherapy, treatment with the oral antioxidant curcumin significantly reduced the occurrence of skin damage and the severity of symptoms, suggested that antioxidant played an active role in the treatment of radiation-induced damages. Introducing antioxidants like gallic acid into gelatin hydrogel as prepared by Thi et al. (2020) the scavenging ability of OH and DPPH radicals was increased to 91% and 58% promoted wound healing. Liu et al. (2020) prepared an injectable antioxidant baicalin hydrogel and showed the ability to accelerate wound healing. Zhang et al. (2021) also encapsulated antioxidants including curcumin and epigallocatechin gallate (EGCG) on the surface of the hydrogel and released them stably within 48 h (Figure 2B). The results showed that the equilibrium charged hydrogel had excellent hemocompatibility, and as a wound dressing, it effectively resisted bacterial and protein adsorption and avoided immune recognition. The results showed that the equilibrium charged hydrogel had excellent hemocompatibility, and as a wound dressing, it effectively resisted bacterial and protein adsorption and avoided immune recognition. At the same time, the introduction of antioxidants enabled wound dressings to quickly remove ROS, with the function of eliminating inflammation and improving vascularization, thereby significantly limited the progression of radiation-induced skin wounds and accelerating wound healing. This new hydrogel dressing has great potential in clinical application for radiation-induced skin wounds.
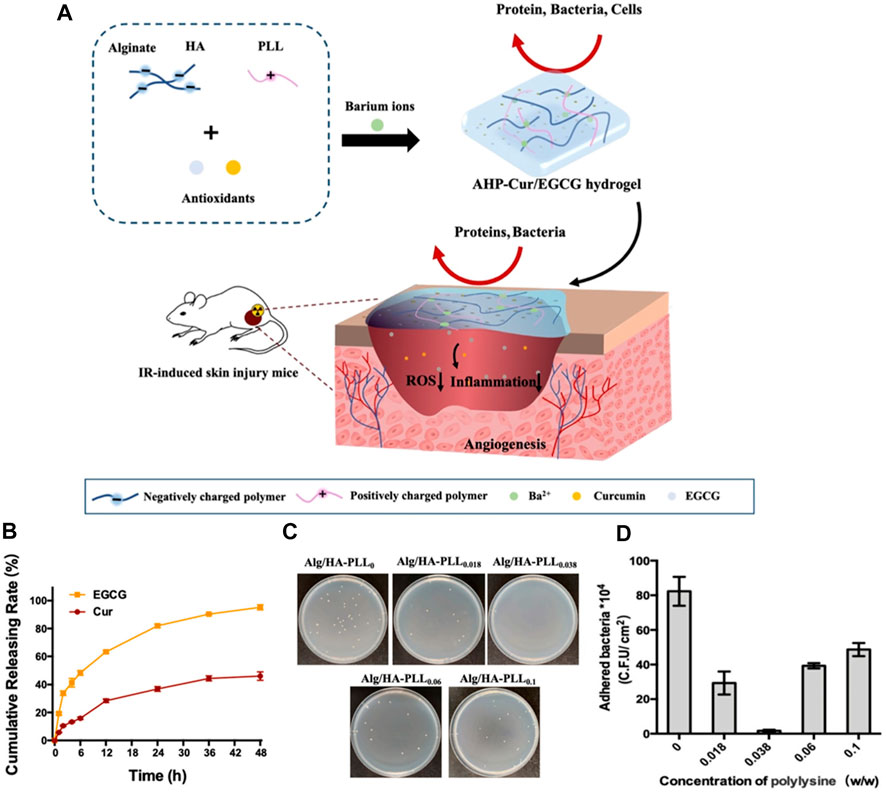
FIGURE 2. A balanced charged hydrogel with anti-biofouling and antioxidant properties for treatment of irradiation-induced skin injury. (A) The schematic of the one-step preparation of the antioxidants (Curcumin and EGCG) loaded balanced charged hydrogel and its application as the pro-healing wound dressing for the treatment of radiation-induced skin injury in mice. (B) The releasing behavior of curcumin and EGCG from the hydrogel. (C) Digital images of relative E. coli adhesion on the surfaces of different charged hydrogel samples. (D) The Colony-Forming Units of E. coli adhesion on different hydrogel samples, respectively.
Radiation-induced skin wounds are excellent locations for bacterial colonization, and severe bacterial infections would lead to inflammation out of control, inhibiting wound healing, and even dangerous consequences of death. Hao et al. (2022) found that graphene oxide polymers modified by polydopamine had great antibacterial ability, and combined it with alginate to prepare an antibacterial hydrogel applied to radiated-induced wounds. The diameter of this hydrogel is about 162 nm, which displayed a typical nanostructure. Interferon-alpha inducible protein 6 that reduced the radiosensitivity of cells was stable in hydrogel nanocarriers, and the hydrogel nanocarriers could be degraded. In radiation-induced wound model of mice, this hydrogel showed significant antibacterial and wound healing effects. The way traditional wound dressings antibacterial is different from the neutral charged or antibacterial material hydrogel, which lacked sufficient antimicrobial capacity and required a large number of antibiotics, increased the resistance of bacteria. In addition, long-term bacterial infections might form a protective biofilm on the wound surface, further enhancing resistance and preventing the infiltration of antibiotics. At present, there are a variety of new antibacterial hydrogels applied to other wounds, such as hydrogels combined with photothermal therapy, which showed excellent antibacterial and wound healing effects. However, they have not been extended to radiation-induced skin wounds. We expect that more studies such as hydrogel dressings for infected wounds would expand use indications, so that there would be more available treatment measures when facing radiation-induced skin damages.
3.1.2 Chitosan hydrogels
Chitosan is one of the most concerned and studied natural polymer materials. Chitosan is obtained by deacetylation of chitin which widely exists in nature, also known as (1 → 4) -2-Amino-2-deoxy -β-d-glucan, is formed by β-1, 4-glycosidic bonds connecting n-acetyl-D-glucosamine monomer (Cheung et al., 2015). Chitosan is a kind of natural alkaline polysaccharide with positive charge due to the presence of amino group in the molecule. The molecule has a straight chain structure and can be mixed with negatively charged macromolecules (such as chondroitin sulfate, sodium alginate, etc.) to form a hydrogel (Feng et al., 2021). Chitosan itself has good biocompatibility and no biotoxicity. In addition, studies have found that chitosan and its derivatives play a positive role in the wound healing process (Shukla et al., 2016). First, in the early stage of wound healing, it promotes the aggregation of platelets and red blood cells at the site of vascular rupture and tissue hemorrhage and inhibit fibrinolysis, thus promoting hemostasis. Secondly, for infected or contaminated wounds, it reduces inflammation, effectively removes bacteria, and reduces microbial attachment. Finally, it promotes the growth of fresh granulation tissue on wound surface and accelerate healing (Hong et al., 2017). Radiation has a strong ability to kill cells. The most important reason for the difficulty is the difficulty of cell regeneration; the bacterial infection after the formation of skin defects is also a secondary reason. The positive effects of chitosan hydrogel on both of these properties attracted extensive attention in the researches on biomaterials for radiation-induced skin wounds repair (Xu et al., 2018).
Radiation-induced skin damages generally occur in patients with malignant tumours requiring RT. Chemotherapy drugs are commonly used for malignant tumours treatment, but usually have low bioavailability when taken orally, and intravenous administration often causes irritant phlebitis and pain at the injection site. Chitosan hydrogels loaded with drugs could noninvasively deliver specific doses of active substances through the skin barrier, in a continuous or controlled manner, which promote wound healing while significantly increase patient comfort, have much potential biomedical application value (An et al., 2020). A thermosensitive hydrogel was designed by Fonseca et al. (2021) to deliver vinorelbine through the skin for the treatment of melanoma. Viyoch et al. (2005) designed a transdermal plaster, based on chitosan and starch, and cross-linked it to produce a hydrogel that produced a stable release of alpha-hydroxy acid. The study measured tartaric acid, a main component of alpha-hydroxy acid, which released from the hydrogel proportional to the square root of time. Similar hydrogel was used to encase and release curcumin, which was applied in cosmetics. Kudlacik-Kramarczyk et al. (2021) designed a chitosan hydrogel containing albumin and aloe vera juice to treat skin cancers or radiation-induced skin wounds (Figure 3). Aloe vera juice has soothing, anti-inflammatory and antibacterial effects, and is a good treatment for radiation-induced skin wounds. The results from the research of Janahmadi et al. (2019) and Koga et al. (2020) also showed that the combination of aloe vera extract and hydrogel applied to wounds promoted angiogenesis, epithelialization and collagen formation. As shown in Figure 3, the results illustrated similar conclusions that aloe juice in hydrogel promoted epithelial cell regeneration and wound healing. The addition of album to the chitosan hydrogel improved its swelling ratios, effectively absorbed wound exudate. In investigations on the crystallinity during the extension of the hydrogel, slight shifts of the signals were visible but no changes in the form of structure ordering proved tensile resistance. The survival rate of mouse fibroblast L929 was approximately 91–95% under hydrogel culture. In the treatment of malignant tumours, tumour recurrence is the most concerned problem, and RT is one of the most important therapies. After the occurrence of radiation-induced wounds, hydrogels not only served as wound dressings to promote wound healing, albumin within it could also be used as a carrier of cellular inhibitors, which tend to accumulate near tumours and inflammatory tissues after entering the body, maximizing the effect of antitumour drugs. At present, tumour vaccines and other related researches are progressing rapidly. We also expect more studies to combine bioactive molecules such as tumour vaccines or cell inhibitors with hydrogels applying to radiation-induced wounds, combining RT with chemotherapy drug therapy, and simultaneously being the treatment of radiation-induced wounds.
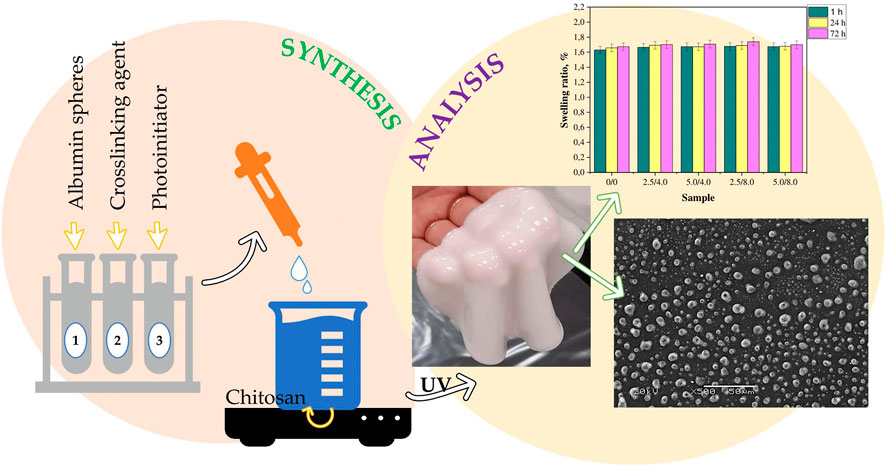
FIGURE 3. Physicochemical Characteristics of Chitosan-Based Hydrogels Containing Albumin Particles and Aloe vera Juice as Transdermal Systems Functionalized in the Viewpoint of Potential Biomedical Application. A brief diagram of the hydrogel synthesis process. Example, scanning electron microscopy images, and results of swelling studies of obtained hydrogels.
3.1.3 Hyaluronic acid hydrogels
Glycosaminoglycan, such as HA, is a general term for the glycan part of proteoglycan macromolecules, which is usually composed of a disaccharide repeating unit consisting of an amino monosaccharide and a uronic acid, and mainly exists in connective tissues of higher animals (Lohmann et al., 2017). HA is a kind of sulfate anion, hydrophilic amino sugar, containing d - glucuronic acid and n - acetyl - d - glucuronic acid disaccharides repeating units (Burdick and Prestwich, 2011). It is found throughout the body, including skin, joints, vitreous bodies, and provides lubrication and plays an important role in cell proliferation and differentiation (Graça et al., 2020). Hydrogel materials based on HA represent a class of new biomaterials with very promising applications, which can meet the needs of radiation-induced skin wounds.
HA molecules contain a large number of carboxyl and hydroxyl groups, forming hydrogen bonds in water intermolecularly, which gives it a strong water-retaining effect, and it has the ability to combine water that is four hundred times its own (Reyes-Ortega et al., 2015). Water could absorb low energy X-rays, and many studies focused on it expected to make a progress by reducing ionizing radiation damages. During RT, high-energy X-rays have a high penetration capacity and little impact on normal skin tissue when penetrating to the target site. However, low energy X-rays have weak penetration ability, during RT which is absorbed by the skin in large quantities and directly attack cellular DNA or interact with water molecules of the body to produce a large number of free radicals, resulting in tissue damages (Bray et al., 2016). HA hydrogels contain a large number of water molecules that could absorb low energy X-ray, which is an effective skin radiation protection method. Xie et al. (2022) designed a HA hydrogel (called nano-GDY@SH hydrogel in Figure 4) with both physical and chemical radiation protection (Figure 4A). The physical protection was mainly realized through the higher water content of HA hydrogel. Graphdiyne has an effective broad-spectrum free radical scavenging ability and good chemical stability (Li et al., 2021). The addition of graphdiyne makes the hydrogel possess chemical protection function. In radiation-induced skin wounds, in addition to tissues that receives radiation, producing large amounts of ROS, a sustained inflammatory response could also lead to the accumulation of ROS, exceeding the antioxidant capacity of the cells. These all hinder the transition from the inflammatory phase to the proliferative phase in the wound healing process and exposes the wound to a vicious cycle between chronic inflammation and oxidative stress (Zhao et al., 2016). Therefore, the maintenance of the intracellular redox balance and the effective improvement of the antioxidant capacity are needed to prevent abnormal immune response and even cell death. Transmission electron microscopy results showed that the diameter of nano-GDY is about 10–20 nm, which not only had good dispersion ability, but also penetrated the skin. According to the rheological experiments results, this hydrogel had good mechanical strength. Compared with water, this hydrogel had better absorption capacity of low energy X-ray radiation, and has efficient and broad-spectrum free radical scavenging ability, which effectively reduced the DNA damage of skin cells, thus improving cell survival rate. As shown in Figure 4 B, in the mouse skin wound model induced by low energy X-ray, the hydrogel further short the time of skin injury and promoted wound healing by alleviating pathological damage such as interstitial edema, keratosis insufficiency, dermal effusion, and inflammatory infiltration.
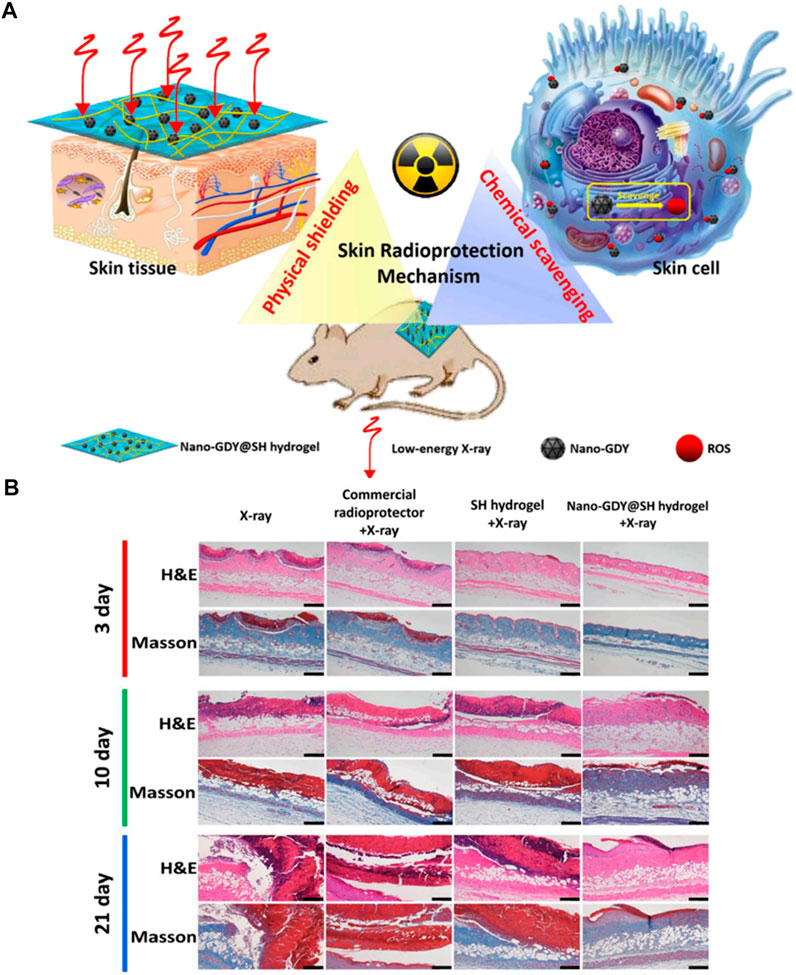
FIGURE 4. External use of the hydrogel for skin radioprotection via both physically shielding of Low-energy X-ray and chemically scavenging of Broad-spectrum free radicals. (A) The scheme of skin radioprotection by nano-GDY@SH hydrogel via both physically shielding of low-energy X-ray and chemically scavenging of broad-spectrum free radicals. (B) The histopathology analysis of skin tissue of mice after different treatments by H&E and Masson staining. Scale bar: 100 μm.
For prostate and gynecological cancer patients who need RT, the location of the tumour is too close to critical organs such as the gastrointestinal tract, to provide enough space in the body to protect the surrounding organs while preserving the therapeutic dose, which have a negative impact on the later treatment. Placing a hydrogel spacer between the tumour and the normal organ can effectively prevent the occurrence of gastrointestinal effects. Because HA has the ability to absorb large amounts of radiation, it is also used as a spacer material to prevent radiation-induced gastrointestinal effects. Prada et al. (2007) used injectable HA as a spacer to prevent rectal mucosa damage in patients undergoing RT for prostate cancer. The results showed that HA maintained its shape and position in the prostate-rectal space and remained stable for 12 months. Kishi et al. (2013) designed a high molecular weight HA hydrogel containing contrast agent to shift the esophagus away from the mediastinal target. Wilder et al. (2010) investigated Hylaform, a cross-linked HA gel injected intraperitoneally to increase the distance between the prostate and the rectum, and showed a significant reduction in acute rectal mucosa toxicity from RT for prostate cancer. Hylaform increased the distance between the prostate and rectum by 8–18 mm prior to the RT. Adverse events such as acute diarrhea occurred in 0% of the treated 10 patients compared with 29.7% of the untreated historical controls (n = 71) (p = 0.04), showing a significant reduction in acute rectal mucosa toxicity from RT for prostate cancer. However, Srinivas and Ramamurthi (2007) also observed that radiation accelerated the degradation of HA hydrogels, so clinical application of it as spacers was limited. Despite a few drawbacks, the findings suggested that HA hydrogel as spacers do reduce the occurrence of gastrointestinal effects. At present, it is necessary to develop more hydrogel spacers with more stable characteristics and stronger radiation absorption capacity, which will play a positive role in clinical practice.
3.1.4 Porcine small intestinal submucosa
Porcine small intestinal submucosa (SIS) is a collagen-based commercial biomaterial that was first used for vascular reconstruction and later widely used for clinical tissue remodeling, especially to provide structural support (Basonbul and Cohen, 2017). SIS is a kind of ECM biomaterial that mimic the ECM of natural tissues and contain complex ECM components that have positive effects on tissue remodeling and regeneration (Nie et al., 2015). Their three-dimensional network structure is very similar to that of the native tissue and constitutes a complex extracellular environment conducive to cell migration and proliferation (Yan et al., 2012). Degradation of the materials also release many bioactive ingredients, such as peptides, which act as cell regulators to induce cell recruitment and tissue remodeling. SIS comes in a variety of forms, including gels, sponges, and flakes, and has many advantages over other types of ECM scaffolds (Nie et al., 2015). SIS has no immunogenicity, and its three-dimensional porous structure allows cells to proliferate, adhere, and differentiate, and has recently been used to treat radiation-induced skin wounds.
The dynamic and complex process of radiation-induced skin wound healing can be completed only on the basis of the reconstruction of the vascular system. Radiation damages the vascular system and reduces angiogenesis, preventing the optimal completion of wound repair (Cheng et al., 2017). Without a blood supply, tissues and cells lack the nutrients and oxygen that they need to survive. In addition, microvascular dysfunction reduces the recruitment of repair cells to the site receiving radiation, thereby reducing the level of regulatory growth factors in the wound microenvironment and further affecting the synthesis of the ECM and wound repair. Wang et al. (2016b) discovered that SIS gel scaffolds were the key basis for the reconstruction of vascular networks, which sustained released vascular endothelial growth factor and basic fibroblast growth factor. The cross section of the gel was observed by SME, which showed that it was highly porous and had interconnected internal structures. The swelling ratio of it was ranging from 10% to 25%. The results of animal experiments and mouse aortic ring experiments and human umbilical vein endothelial cell tube formation experiments showed that SIS gel significantly induced neovascularization. The use of extracellular stroma scaffolds as a cell carrier for stem cell transplantation improved cell survival and maximized the therapeutic potential of stem cells. Vascular regeneration function is regulated by angiogenic factors secreted from human umbilical cord blood (hUCB)-derived- mesenchymal stem/stromal cells (MSCs), including hepatocyte growth factor (HGF) and vascular endothelial growth factor (VEGF), which play an important role in the wound healing process (Garg et al., 2014; Bandekar et al., 2021; Marofi et al., 2021). Lee et al. (Lee et al., 2017b) used SIS as a stem cell scaffold (where hUCB-MSCs were implanted) as a wound dressing to promote radiation-induced skin wound healing. Their study showed that the combination of SIS gels and hUCB-MSCs as topical dressings significantly enhanced radiation-induced skin wound shrinkage, and the histological results showed that local wound angiogenesis increased, which was also verified by tube-like structural formation assay. The ELISA results showed that the secretion of various proangiogenic factors in the SIS gel-cultured hucB-Mscs was significantly increased, and the addition of HGF in the transwell migration assay promoted the migration of HUVECs, proving that the wound healing-promoting effect was achieved by enhancing HGF secretion, promoting angiogenesis, and replenishing damaged endothelial cells. SIS gels simulates the normal skin environment and effectively promotes the healing of radiation-induced wounds while stem cells exert a powerful pro-angiogenic effect. The combination of the two is aimed at one of the most important pathological factors which is severe damage of vascular network and difficulty of vascular regeneration of radiation-induced skin wounds. The results also confirmed the power of the combination.
3.2 Synthetic polymer hydrogels
Synthetic polymers made from monomers have been wildely used as wound healing dressings at present. Because the chemical structure of synthetic polymer hydrogel is clear and physical and chemical properties are stable, it can be accurately characterized, manufactured, and precisely regulated, giving some advantages over natural biopolymer hydrogels (Cruz-Acuña and García, 2017). A wide range of synthetic dressings have been developed for general wounds treatment, including polyacrylic acid, polyethylene glycol, hydroxyethyl cellulose, and so on (Guo and Ma, 2018). Some of them have already been used in researches about radiation-induced skin and mucosa wounds.
Polyacrylic acid is a kind of non-degradable hydrophobic plastic polymer, which is broadly used in biomedical field. Polyacrylic acid is cheap to manufacture, has high mechanical strength and durability, and is easy to disinfect. Another quality of polyacrylic acid is its biological inertia, which is the basis of its wide application in biomedical fields (Guvendiren and Burdick, 2013). Bioinertia is manifested in that polyacrylic acid is non-toxic, non-degradable in organisms, does not produce degradation products that induce inflammatory reactions, does not activate various types of cell receptors, and does not cause local tissue reactions (Guo and Ma, 2018). It is important to notice that all non-degradable polymers, although bioinert, can trigger foreign body reactions, resulting in immune events and tissue fibrosis. However, when applied to the wounds, possible foreign body reactions are avoided due to the removable nature of the dressings (Guvendiren and Burdick, 2013).
Kulshrestha et al. (2020) demonstrated a polyacrylic acid hydrogel with a good healing effect on radiation-induced skin wounds, which contained 5% sildenafil citrate (SC) used to raise nitric oxide (NO) levels in wounds. NO is an active wound-healing molecule and has attracted much attention as a new agent in wound therapy (Krausz and Friedman, 2015). NO is produced by guanidine atoms at the end of arginine under the catalysis of NO synthase (NOS) and plays a very important role in all three stages of the wound healing process (Hoang et al., 2018). Its main functions are to promote cell proliferation and migration, enhance angiogenesis and vasodilation, resist pathogens, and promote tissue regeneration. Previous studies have shown that in radiation-induced skin wounds, NO levels gradually decrease over time, suggesting that NO levels are positively correlated with the degree of wound damage (Anastasio et al., 2020). The research of Vogt et al. (2013) and Cao et al. (2020) also demonstrated the increase of NO released by hydrogel dressings in wounds also effectively reduced the risk of bacterial infection. Kulshrestha et al. (2020) study demonstrated a standardized approach whereby 45 Gy of radiation was induced to the mouse’s back, to develop a radiation-induced wound model, which was a good basis for such studies. On this model, they applied 5% SC polyacrylic acid hydrogel as the treatment method, and the animal experiment results showed that the recovery rate of relative skin injury was 55 ± 0.99% and 81 ± 0.82% on day 16 and day 28, respectively, suggested hydrogel had a significant effect on promoting wound healing. At the mid-point of the experiment (day 16), the concentration of NO in the tissues of the hydrogel-treated group was significantly higher, indicated the shrinkage of wound was formed by increasing the NO concentration. As a stable drug carrier, polyacrylic acid hydrogels increased NO concentration and improved radiation-induced skin wounds healing, provided a new option for clinical application, but the mechanism was not explored in depth in this study. NO has multiple effects on tissue, and understanding how it promotes healing is the basis for designing better dressings.
Polyethylene glycol hydrogel is one of the most commonly used radioactive spacer. Water-soluble polyethylene glycol hydrogels are usually non-immunogenic and non-toxic. Injected as a liquid, they remain intact in their original position for 3 months and are hydrolyzed and excreted through the kidneys in 6 months (Karsh et al., 2018). SpaceOAR is the most widely used commercial hydrogel spacer currently approved by the US Food and Drug Administration (FDA) for use in prostate cancer RT patients. There has been a lot of research data to support the positive role of SpaceOAR in clinical application. Armstrong’s (Armstrong et al., 2021) systematic review study examined 19 related studies and included 3,622 patients. The results showed that SpaceOAR significantly reduced the rectal radiation dose and the toxicity of radiation to the gastrointestinal tract mucosa and genitourinary system for many types of RT and improved the quality of life for most patients. However, at present, there are few reports on complications or adverse reactions about SpaceOAR, and the countermeasures after the occurrence are not introduced detailedly. More clinical studies are needed to lay the foundation for wider use.
Synthetic polymers hydrogels can also be applied to patients with radiation-induced oral mucositis, and relevant products called Gelclair and MuGard have been published in clinical practice. Barber et al. (2007) studied the use of a hydroxyethyl cellulose hydrogel, Gelclair, on radiation-induced oral damages and showed a similar pain reduction effect compared to traditional methods. Allison et al. (2014) identified the effects of polyacrylic acid hydrogel (MuGard) on radiation-induced oral damages too. 120 patients were randomized to receive either MuGard or Sham Control Rinse (SC) during radiotherapy, and the treatment effect was evaluated everyday. The results showed that MuGard effectively alleviated patients’ symptoms of pain and discomfort as well as disease progression. Synthetic polymers hydrogels used in oral mucositis usually show analgesic function, because there are fewer bacteria in the mouth, and the epithelial cells grow rapidly, so that it is most important to relieve pain symptoms. There are few studies on hydrogels and related products applied to patients with radiation-induced oral mucositis, because oral mucosa has a better recovery capacity than skin, mainly focused on prevention in clinical.
3.3 Composite hydrogels
As mentioned above, natural biopolymer has good biocompatibility and biodegradability, without immune and toxic reactions, but compared with synthetic polymer, their properties are unstable, and it is difficult to carry out various functional modifications. On the other hand, although synthetic polymer can undergo various chemical modifications to possess unique properties, their biocompatibility is poor. The combination of these two kinds of polymers into biomedical materials not only improves physical properties, but also provides all the advantages of these polymers compared to polymer hydrogels alone. Zeng et al. (2011) combined synthetic polymer polyvinyl pyrrolidone (PVP) and natural polymer chitosan to prepare a new biocompatible hydrogel, which not only has good mechanical properties, but also has good degradation ability. Unlike Polyacrylic acid, PVP is a biodegradable synthetic, water soluble polymer. PVP is also used in the biomedical field because of its good biocompatibility-like wound dressings and implants (Guo and Ma, 2018). PVP mixed with natural polymers to make hydrogel not only protects the wound surface, promotes cell repair, absorbs the fluid exuded from the wound surface, but also can be absorbed by the human body (Zhang and Huang, 2020).
The combination of PVP and chitosan is widely used in the field of wound repair. PVP, chitosan, and polyvinyl alcohol (PVA) composited material designed by Rahmani et al. (2020) is a promising wound dressing, and the viable bacterial cell counting results showed that it had good inhibitory effect on E. Coli and S. aureus. This kind of composite hydrogel can also be applied to radiation-induced damages to play its unique function. Hung et al. (2021) designed a PVP-gelatin-chitosan composite containing egg-yolk oil (EYO) for the treatment of radiation-induced skin wounds. The water content of the hydrogel was higher than 90%. FTIR spectra analyses showed that the gel had good tensile properties after the proportion of each component was adjusted. The weight loss rate of the material was up to 30% within a week, which showed good degradability. EYO could promote re-epithelialization, hemostasis, and has other functions, and is widely used in the treatment of various burn wounds. The lecithin in EYO is negatively charged and often blends with chitosan. This hydrogel film had good biocompatibility, mechanical and degradation performances and provided a wet environment for wound healing. Animal experiments showed that although there was no significant difference in the time of first occurrence of radiation dermatitis compared with control group, but the application of the hydrogel obviously delayed the time of greatest wound area occurrence and reduced the wound area at the same time point. Histological results have shown that the hydrogel reduced the inflammatory response of radiation-induced skin wounds and promoted the re-epithelialization. At present, there are few composite hydrogels applied to radiation-induced wounds. It is expected that the relevant research about composite hydrogels applied to various wounds continue developing deeper and further, so a s to provide a broader choice for the treatment of radiation-induced wounds.
3.4 Commodity dressings
In the wound treatment literature, hydrocolloids or hydrogels are commonly used for acute skin wounds, burn wounds, or chronic wounds such as diabetic feet (Russi et al., 2007). However, in the past decade, hydroactive colloid gel dressings have increasingly appeared in the literature on radiation-induced skin damage care (Chu, 2022). FLAMIGEL is a hydroactive colloid gel which combines the advantages of hydrocolloid and hydrogel, and has the characteristics of absorption and moisture (Van den Plas et al., 2009). It not only absorbs excessive exudate on the wound surface, but also continuously provides moisture to the wound to maintain the optimal moist environment. This moist environment is good for wound healing, preventing wound dryness, and reducing painful scarring and infection. FLAMIGEL is suitable for the treatment of radiation-induced skin damages which main clinical manifestation is dry and wet skin desquamation. Although there was insufficient evidence to confirm the effectiveness of the gel, many clinical studies have demonstrated its positive effects on refractory wounds. Vincenzo Panasiti et al. (2006) reported using it in mycosis Fungoides skin ulcers, Van den Plas et al. (2009) reported using it in intractable wounds, and Yang et al. (2013) reported using it in burn wounds, all of which proved its convenience, pain relief, and beneficial effects in promoting healing.
In two papers published in 2014 (Censabella et al., 2014) and 2017 (Censabella et al., 2017), Sandrine Censabella et al. compared the efficacy of FLAMIGEL gel and 5% Dexpanenol in the treatment of radiation-induced skin damages in patients with breast cancer RT. Dexpannonol cream, which often used in clinic, has therapeutic effects on a variety of skin diseases, but the available evidence of its effect on radiation-induced skin damages is limited (Ebner et al., 2002). The results showed that the gel has a good preventive effect on wet desquamation caused by radiation, and the onset time of wet desquamation was significantly delayed, and the incidence was significantly reduced in RT patients.
In the second article, the authors also mentioned that for lighter patchy dry/wet desquamation, they applied Mepilex Lite, a self-adhesive silicone foam dressing, as treatment. Unlike FLAMIGEL Gel, which is used for wounds with more exudation, Mepilex Lite is used for wounds with little or no exudation. It is made of polyurethane foam, which is thin and soft (Herst et al., 2014; Walker et al., 2017). When applied, the peripheral adhesive layer should be attached to healthy skin around the wound, keeping the wound closed and providing a moist environment conducive to healing (Wooding et al., 2018). Diggelmann et al. (Diggelmann et al., 2010) investigated the efficacy of Mepilex Lite in skin erythema after breast cancer RT. The results showed that Mepilex Lite did not affect skin surface temperature and significantly reduced the severity of radiation-induced erythema. In areas with higher radiation doses like the inframammary fold, the dressing significantly reduced the appearance of skin erythema. Zhong et al. (Zhong et al., 2013) studied the application of Mepilex Lite in skin desquamation of patients with nasopharyngeal carcinoma. The results showed that Mepilex Lite significantly reduced skin healing time and improved the sleep condition of patients, which deserved further study. These studies suggested that FLAMIGEL gel and Mepilex Lite are good candidates for treatment of early stage of radiation-induced skin damages.
4 Conclusion and future directions
The morbidity rate of radiation-induced injury is high, which affects the daily life quality of patients and subsequent antitumour therapy. The emergence of multifunctional novel hydrogels has led to a new approach in the treatment of radiation-induced damages, which was originally difficult. This review introduced the formation mechanism of radiation-induced skin damages. Based on the four most important pathological mechanisms, wound healing cannot occur step by step on their own; hence, clinical intervention is needed to promote healing. Moreover, these four injury factors have also become new treatment approaches, and thereby, a variety of hydrogel dressings have been developed to promote the healing of radiation-induced skin and mucosa damages. Evidentlyan increasing number of novel hydrogels have been applied to radiation-induced damages, and many commercial hydrogels have achieved good therapeutic and preventive effects in clinical practice. But on the other hands there are still few new means of clinical treatment on radiation-induced skin and mucosa damages, which need more basic scientific research support. With the development of materials science, an increasing number of new hydrogel dressings have appeared in our field of vision, but the research scope was mostly focused on the mouse full-thickness skin defect model or diabetic wound model. Although the pathogenesis of radiation-induced wounds is special, the pathological factors are similar to other wounds. The new hydrogel could expand the application scope and play a more extensive role. Only by more basic research laying a good foundation can more commercial dressings emerge to support clinical applications. At the same time, we also expect some combination treatments, such as the combination of tumour vaccines against tumour recurrence and hydrogel dressing promoting wound healing, to open up new therapeutic directions. Therefore, we emphasized and acknowledged the potential of hydrogels in the treatment of radiation-induced damages and hoped for more researches developed to provide systematic and theoretical supports for treatment in the future.
Author contributions
YS and HC wrote the original draft; CY and LL made the graphical abstract; WZ, FX, and JG revised and approved the manuscript; WZ supervised the project. All authors have read and agreed to the published version of the manuscript.
Funding
This work was funded by Shanghai Changzheng Hospital (2020-RP08, 2019CZJS216), and National Natural Science Foundation of China (82072051).
Acknowledgments
We would like to acknowledge the support from the Shanghai Changzheng Hospital.
Conflict of interest
The authors declare that the research was conducted in the absence of any commercial or financial relationships that could be construed as a potential conflict of interest.
Publisher’s note
All claims expressed in this article are solely those of the authors and do not necessarily represent those of their affiliated organizations, or those of the publisher, the editors and the reviewers. Any product that may be evaluated in this article, or claim that may be made by its manufacturer, is not guaranteed or endorsed by the publisher.
References
Abshire, D., and Lang, M. K. (2018). The evolution of radiation therapy in treating cancer. Seminars Oncol. Nurs. 34 (2), 151–157. doi:10.1016/j.soncn.2018.03.006
Aderibigbe, B. A., and Buyana, B. (2018). Alginate in wound dressings. Pharmaceutics 10 (2), 42. doi:10.3390/pharmaceutics10020042
Allison, R. R., Ambrad, A. A., Arshoun, Y., Carmel, R. J., Ciuba, D. F., Feldman, E., et al. (2014). Multi-institutional, randomized, double-blind, placebo-controlled trial to assess the efficacy of a mucoadhesive hydrogel (MuGard) in mitigating oral mucositis symptoms in patients being treated with chemoradiation therapy for cancers of the head and neck. Cancer 120 (9), 1433–1440. doi:10.1002/cncr.28553
An, Y. H., Lee, J., Son, D. U., Kang, D. H., Park, M. J., Cho, K. W., et al. (2020). Facilitated transdermal drug delivery using nanocarriers-embedded electroconductive hydrogel coupled with reverse electrodialysis-driven iontophoresis. ACS Nano 14 (4), 4523–4535. doi:10.1021/acsnano.0c00007
Anastasio, A. T., Paniagua, A., Diamond, C., Ferlauto, H. R., and Fernandez-Moure, J. S. (2020). Nanomaterial nitric oxide delivery in traumatic orthopedic regenerative medicine. Front. Bioeng. Biotechnol. 8, 592008. doi:10.3389/fbioe.2020.592008
Andersen, T., Auk-Emblem, P., and Dornish, M. (2015). 3D cell culture in alginate hydrogels. Microarrays (Basel, Switz. 4 (2), 133–161. doi:10.3390/microarrays4020133
Arlov, Ø., and Skjåk-Bræk, G. (2017). Sulfated alginates as heparin analogues: A review of chemical and functional properties. Mol. (Basel, Switz. 22 (5), 778. doi:10.3390/molecules22050778
Armstrong, N., Bahl, A., Pinkawa, M., Ryder, S., Ahmadu, C., Ross, J., et al. (2021). SpaceOAR hydrogel spacer for reducing radiation toxicity during radiotherapy for prostate cancer. A systematic review. Urology 156, e74–e85. doi:10.1016/j.urology.2021.05.013
Bandekar, M., Maurya, D. K., Sharma, D., and Sandur, S. K. (2021). Preclinical studies and clinical prospects of wharton's jelly-derived MSC for treatment of acute radiation syndrome. Curr. Stem Cell Rep. 7 (2), 85–94. doi:10.1007/s40778-021-00188-4
Barber, C., Powell, R., Ellis, A., and Hewett, J. (2007). Comparing pain control and ability to eat and drink with standard therapy vs Gelclair: A preliminary, double centre, randomised controlled trial on patients with radiotherapy-induced oral mucositis. Support. Care Cancer 15 (4), 427–440. doi:10.1007/s00520-006-0171-1
Basonbul, R. A., and Cohen, M. S. (2017). Use of porcine small intestinal submucosa for pediatric endoscopic tympanic membrane repair. World J. Otorhinolaryngol. Head. Neck Surg. 3 (3), 142–147. doi:10.1016/j.wjorl.2017.09.001
Beach, T. A., Groves, A. M., Williams, J. P., and Finkelstein, J. N. (2020). Modeling radiation-induced lung injury: Lessons learned from whole thorax irradiation. Int. J. Radiat. Biol. 96 (1), 129–144. doi:10.1080/09553002.2018.1532619
Beaumont, M., Tran, R., Vera, G., Niedrist, D., Rousset, A., Pierre, R., et al. (2021). Hydrogel-forming algae polysaccharides: From seaweed to biomedical applications. Biomacromolecules 22 (3), 1027–1052. doi:10.1021/acs.biomac.0c01406
Benson, R., Madan, R., Kilambi, R., and Chander, S. (2016). Radiation induced liver disease: A clinical update. J. Egypt. Natl. Cancer Inst. 28 (1), 7–11. doi:10.1016/j.jnci.2015.08.001
Bray, F. N., Simmons, B. J., Wolfson, A. H., and Nouri, K. (2016). Acute and chronic cutaneous reactions to ionizing radiation therapy. Dermatol. Ther. (Heidelb). 6 (2), 185–206. doi:10.1007/s13555-016-0120-y
Browne, S., and Pandit, A. (2015). Biomaterial-mediated modification of the local inflammatory environment. Front. Bioeng. Biotechnol. 3, 67. doi:10.3389/fbioe.2015.00067
Brunt, A. M., Wheatley, D., Yarnold, J., Somaiah, N., Kelly, S., Harnett, A., et al. (2016). Acute skin toxicity associated with a 1-week schedule of whole breast radiotherapy compared with a standard 3-week regimen delivered in the UK FAST-Forward Trial. Radiother. Oncol. 120 (1), 114–118. doi:10.1016/j.radonc.2016.02.027
Burdick, J. A., and Prestwich, G. D. (2011). Hyaluronic acid hydrogels for biomedical applications. Adv. Mat. 23 (12), H41–H56. doi:10.1002/adma.201003963
Campbell, I. R., and Illingworth, M. H. (1992). Can patients wash during radiotherapy to the breast or chest wall? A randomized controlled trial. Clin. Oncol. 4 (2), 78–82. doi:10.1016/s0936-6555(05)80971-9
Cao, J., Su, M., Hasan, N., Lee, J., Kwak, D., Kim, D. Y., et al. (2020). Nitric oxide-releasing thermoresponsive pluronic F127/alginate hydrogel for enhanced antibacterial activity and accelerated healing of infected wounds. Pharmaceutics 12 (10), 926. doi:10.3390/pharmaceutics12100926
Cao, W. B., and Gao, C. Y. (2021). Research advances on multifunctional hydrogel dressings for treatment of diabetic chronic wounds. Zhonghua Shao Shang Za Zhi 37 (11), 1090–1098. doi:10.3760/cma.j.cn501120-20210715-00249
Censabella, S., Claes, S., Orlandini, M., Braekers, R., and Bulens, P. (2017). Efficacy of a hydroactive colloid gel versus historical controls for the prevention of radiotherapy-induced moist desquamation in breast cancer patients. Eur. J. Oncol. Nurs. 29, 1–7. doi:10.1016/j.ejon.2017.04.003
Censabella, S., Claes, S., Orlandini, M., Braekers, R., Thijs, H., and Bulens, P. (2014). Retrospective study of radiotherapy-induced skin reactions in breast cancer patients: Reduced incidence of moist desquamation with a hydroactive colloid gel versus dexpanthenol. Eur. J. Oncol. Nurs. 18 (5), 499–504. doi:10.1016/j.ejon.2014.04.009
Cheng, N. C., Lin, W. J., Ling, T. Y., and Young, T. H. (2017). Sustained release of adipose-derived stem cells by thermosensitive chitosan/gelatin hydrogel for therapeutic angiogenesis. Acta Biomater. 51, 258–267. doi:10.1016/j.actbio.2017.01.060
Cheung, R. C., Ng, T. B., Wong, J. H., and Chan, W. Y. (2015). Chitosan: An update on potential biomedical and pharmaceutical applications. Mar. drugs 13 (8), 5156–5186. doi:10.3390/md13085156
Choudhury, F. K., Rivero, R. M., Blumwald, E., and Mittler, R. (2017). Reactive oxygen species, abiotic stress and stress combination. Plant J. 90 (5), 856–867. doi:10.1111/tpj.13299
Chu, C. Hydrogel combine hydrocolloid dressings for treatment of radiation-induced grade 4 dermatitis in patients receiving radiotherapy to the head and neck2022.
Cruz-Acuña, R., and García, A. J. (2017). Synthetic hydrogels mimicking basement membrane matrices to promote cell-matrix interactions. Matrix Biol. 57-58, 324–333. doi:10.1016/j.matbio.2016.06.002
Daeschlein, G. (2013). Antimicrobial and antiseptic strategies in wound management. Int. Wound J. 10, 9–14. doi:10.1111/iwj.12175
de Oliveira, S., Rosowski, E. E., and Huttenlocher, A. (2016). Neutrophil migration in infection and wound repair: Going forward in reverse. Nat. Rev. Immunol. 16 (6), 378–391. doi:10.1038/nri.2016.49
Diggelmann, K. V., Zytkovicz, A. E., Tuaine, J. M., Bennett, N. C., Kelly, L. E., and Herst, P. M. (2010). Mepilex lite dressings for the management of radiation-induced erythema: A systematic inpatient controlled clinical trial. Br. J. Radiol. 83 (995), 971–978. doi:10.1259/bjr/62011713
Dimatteo, R., Darling, N. J., and Segura, T. (2018). In situ forming injectable hydrogels for drug delivery and wound repair. Adv. drug Deliv. Rev. 127, 167–184. doi:10.1016/j.addr.2018.03.007
DiPietro, L. A., Wilgus, T. A., and Koh, T. J. (2021). Macrophages in healing wounds: Paradoxes and paradigms. Int. J. Mol. Sci. 22 (2), 950. doi:10.3390/ijms22020950
Ebner, F., Heller, A., Rippke, F., and Tausch, I. (2002). Topical use of dexpanthenol in skin disorders. Am. J. Clin. Dermatol. 3 (6), 427–433. doi:10.2165/00128071-200203060-00005
Elkhoury, K., Morsink, M., Sanchez-Gonzalez, L., Kahn, C., Tamayol, A., and Arab-Tehrany, E. (2021). Biofabrication of natural hydrogels for cardiac, neural, and bone Tissue engineering Applications. Bioact. Mat. 6 (11), 3904–3923. doi:10.1016/j.bioactmat.2021.03.040
Fan, X., Yahia, L., and Sacher, E. (2021). Antimicrobial properties of the Ag, Cu nanoparticle system. Biology 10 (2), 137. doi:10.3390/biology10020137
Feng, P., Luo, Y., Ke, C., Qiu, H., Wang, W., Zhu, Y., et al. (2021). Chitosan-based functional materials for skin wound repair: Mechanisms and applications. Front. Bioeng. Biotechnol. 9, 650598. doi:10.3389/fbioe.2021.650598
Ferreira, E. B., Vasques, C. I., Gadia, R., Chan, R. J., Guerra, E. N., Mezzomo, L. A., et al. (2017). Topical interventions to prevent acute radiation dermatitis in head and neck cancer patients: A systematic review. Support. Care Cancer 25 (3), 1001–1011. doi:10.1007/s00520-016-3521-7
Fischer-Valuck, B. W., Chundury, A., Gay, H., Bosch, W., and Michalski, J. (2017). Hydrogel spacer distribution within the perirectal space in patients undergoing radiotherapy for prostate cancer: Impact of spacer symmetry on rectal dose reduction and the clinical consequences of hydrogel infiltration into the rectal wall. Pract. Radiat. Oncol. 7 (3), 195–202. doi:10.1016/j.prro.2016.10.004
Fonseca, AdM., Araújo, CdC. B., da Silva, J. H., Honório, TdS., Nasciutti, L. E., Cabral, L. M., et al. (2021). Development of transdermal based hydrogel formulations of vinorelbine with an evaluation of their in vitro profiles and activity against melanoma cells and in silico prediction of drug absorption. J. Drug Deliv. Sci. Technol. 63, 102449. doi:10.1016/j.jddst.2021.102449
Francesko, A., Petkova, P., and Tzanov, T. (2018). Hydrogel dressings for advanced wound management. Curr. Med. Chem. 25 (41), 5782–5797. doi:10.2174/0929867324666170920161246
Garg, R. K., Rennert, R. C., Duscher, D., Sorkin, M., Kosaraju, R., Auerbach, L. J., et al. (2014). Capillary force seeding of hydrogels for adipose-derived stem cell delivery in wounds. Stem Cells Transl. Med. 3 (9), 1079–1089. doi:10.5966/sctm.2014-0007
Graça, M. F. P., Miguel, S. P., Cabral, C. S. D., and Correia, I. J. (2020). Hyaluronic acid-based wound dressings: A review. Carbohydr. Polym. 241, 116364. doi:10.1016/j.carbpol.2020.116364
Guo, B., and Ma, P. X. (2018). Conducting polymers for tissue engineering. Biomacromolecules 19 (6), 1764–1782. doi:10.1021/acs.biomac.8b00276
Guvendiren, M., and Burdick, J. A. (2013). Engineering synthetic hydrogel microenvironments to instruct stem cells. Curr. Opin. Biotechnol. 24 (5), 841–846. doi:10.1016/j.copbio.2013.03.009
Hamidzadeh, K., Christensen, S. M., Dalby, E., Chandrasekaran, P., and Mosser, D. M. (2017). Macrophages and the recovery from acute and chronic inflammation. Annu. Rev. Physiol. 79, 567–592. doi:10.1146/annurev-physiol-022516-034348
Hamstra, D. A., Mariados, N., Sylvester, J., Shah, D., Karsh, L., Hudes, R., et al. (2017). Continued benefit to rectal separation for prostate radiation therapy: Final results of a phase iii trial. Int. J. Radiat. Oncology*Biology*Physics 97 (5), 976–985. doi:10.1016/j.ijrobp.2016.12.024
Han, G., and Ceilley, R. (2017). Chronic wound healing: A review of current management and treatments. Adv. Ther. 34 (3), 599–610. doi:10.1007/s12325-017-0478-y
Hao, J., Sun, M., Li, D., Zhang, T., Li, J., and Zhou, D. (2022). An IFI6-based hydrogel promotes the healing of radiation-induced skin injury through regulation of the HSF1 activity. J. Nanobiotechnology 20 (1), 288. doi:10.1186/s12951-022-01466-x
Haruna, F., Lipsett, A., and Marignol, L. (2017). Topical management of acute radiation dermatitis in breast cancer patients: A systematic review and meta-analysis. Anticancer Res. 37 (10), 5343–5353. doi:10.21873/anticanres.11960
He, S., Liang, C., Yi, C., and Wu, M. (2021). Therapeutic effect of continuous diffusion of oxygen therapy combined with traditional moist wound dressing therapy in the treatment of diabetic foot ulcers. Diabetes Res. Clin. Pract. 174, 108743. doi:10.1016/j.diabres.2021.108743
Hegedus, F., Mathew, L. M., and Schwartz, R. A. (2017). Radiation dermatitis: An overview. Int. J. Dermatol. 56 (9), 909–914. doi:10.1111/ijd.13371
Herst, P. M., Bennett, N. C., Sutherland, A. E., Peszynski, R. I., Paterson, D. B., and Jasperse, M. L. (2014). Prophylactic use of Mepitel Film prevents radiation-induced moist desquamation in an intra-patient randomised controlled clinical trial of 78 breast cancer patients. Radiother. Oncol. 110 (1), 137–143. doi:10.1016/j.radonc.2014.01.005
Hoang, Thi T. T., Lee, Y., Le Thi, P., and Park, K. D. (2018). Nitric oxide-releasing injectable hydrogels with high antibacterial activity through in situ formation of peroxynitrite. Acta Biomater. 67, 66–78. doi:10.1016/j.actbio.2017.12.005
Hong, S. C., Yoo, S. Y., Kim, H., and Lee, J. (2017). Chitosan-based multifunctional platforms for local delivery of therapeutics. Mar. drugs 15 (3), 60. doi:10.3390/md15030060
Hung, Y-C., Hsieh, S-C., Hou, S-R., Kung, J-Y., Tang, C-M., and Chang, C-J. (2021). In vivo evaluation of PVP-gelatin-chitosan composite blended with egg-yolk oil for radiodermatitis. Appl. Sci. 11 (21), 10290. doi:10.3390/app112110290
Hymes, S. R., Strom, E. A., and Fife, C. (2006). Radiation dermatitis: Clinical presentation, pathophysiology, and treatment 2006. J. Am. Acad. Dermatol. 54 (1), 28–46. doi:10.1016/j.jaad.2005.08.054
Jagsi, R., Griffith, K. A., Bellon, J. R., Woodward, W. A., Horton, J. K., Ho, A., et al. (2018). Concurrent veliparib with chest wall and nodal radiotherapy in patients with inflammatory or locoregionally recurrent breast cancer: The TBCRC 024 phase I multicenter study. J. Clin. Oncol. official J. Am. Soc. Clin. Oncol. 36 (13), 1317–1322. doi:10.1200/jco.2017.77.2665
Janahmadi, Z., Motlagh, M. R., and Zaeri, S. (2019). Enhancing rat full-thickness skin wounds with a mixed aloe/chitosan gel. Formos. J. Surg. 52 (3), 84–91. doi:10.4103/fjs.fjs_109_18
Joseph, K., Vos, L. J., Gabos, Z., Pervez, N., Chafe, S., Tankel, K., et al. (2021). Skin toxicity in early breast cancer patients treated with field-in-field breast intensity-modulated radiotherapy versus helical inverse breast intensity-modulated radiotherapy: Results of a phase III randomised controlled trial. Clin. Oncol. 33 (1), 30–39. doi:10.1016/j.clon.2020.07.005
Kang, J. I., and Park, K. M. (2021). Advances in gelatin-based hydrogels for wound management. J. Mat. Chem. B 9 (6), 1503–1520. doi:10.1039/d0tb02582h
Karoyo, A. H., and Wilson, L. D. (2021). A Review on the design and hydration properties of natural polymer-based hydrogels. Materials 14 (5), 1095. doi:10.3390/ma14051095
Karsh, L. I., Gross, E. T., Pieczonka, C. M., Aliotta, P. J., Skomra, C. J., Ponsky, L. E., et al. (2018). Absorbable hydrogel spacer use in prostate radiotherapy: A comprehensive review of phase 3 clinical trial published data. Urology 115, 39–44. doi:10.1016/j.urology.2017.11.016
Kishi, K., Iida, T., Ojima, T., Sonomura, T., Shirai, S., Nakai, M., et al. (2013). Esophageal gel-shifting technique facilitating eradicative boost or reirradiation to upper mediastinal targets of recurrent nerve lymph node without damaging esophagus. J. Radiat. Res. 54 (4), 748–754. doi:10.1093/jrr/rrs137
Klotz, B. J., Gawlitta, D., Rosenberg, A., Malda, J., and Melchels, F. P. W. (2016). Gelatin-methacryloyl hydrogels: Towards biofabrication-based tissue repair. Trends Biotechnol. 34 (5), 394–407. doi:10.1016/j.tibtech.2016.01.002
Koga, A. Y., Felix, J. C., Marques Silvestre, R. G., Lipinski, L. C., Carletto, B., Kawahara, F. A., et al. (2020). Evaluation of wound healing effect of alginate film containing Aloe vera gel and cross-linked with zinc chloride. Acta Cir. Bras. 35 (5), e202000507. doi:10.1590/s0102-865020200050000007
Krausz, A., and Friedman, A. J. (2015). Nitric oxide as a surgical adjuvant. Future Sci. OA 1 (1), Fso56. doi:10.4155/fso.15.56
Kudlacik-Kramarczyk, S., Glab, M., Drabczyk, A., Kordyka, A., Godzierz, M., Wrobel, P. S., et al. (2021). Physicochemical characteristics of chitosan-based hydrogels containing albumin particles and aloe vera juice as transdermal systems functionalized in the viewpoint of potential biomedical applications. Mater. (Basel) 14 (19), 5832. doi:10.3390/ma14195832
Kulshrestha, S., Chawla, R., Singh, S., Yadav, P., Sharma, N., Goel, R., et al. (2020). Protection of sildenafil citrate hydrogel against radiation-induced skin wounds. Burns 46 (5), 1157–1169. doi:10.1016/j.burns.2019.11.020
Lee, C., Shim, S., Jang, H., Myung, H., Lee, J., Bae, C. H., et al. (2017). Human umbilical cord blood-derived mesenchymal stromal cells and small intestinal submucosa hydrogel composite promotes combined radiation-wound healing of mice. Cytotherapy 19 (9), 1048–1059. doi:10.1016/j.jcyt.2017.06.007
Lee, M. S., Finch, W., and Mahmud, E. (2013). Cardiovascular complications of radiotherapy. Am. J. Cardiol. 112 (10), 1688–1696. doi:10.1016/j.amjcard.2013.07.031
Lee, S. Y., Jeong, E. K., Ju, M. K., Jeon, H. M., Kim, M. Y., Kim, C. H., et al. (2017). Induction of metastasis, cancer stem cell phenotype, and oncogenic metabolism in cancer cells by ionizing radiation. Mol. Cancer 16 (1), 10. doi:10.1186/s12943-016-0577-4
Li, S., Dong, S., Xu, W., Tu, S., Yan, L., Zhao, C., et al. (2018). Antibacterial hydrogels. Adv. Sci. (Weinh). 5 (5), 1700527. doi:10.1002/advs.201700527
Li, X., Guo, M., and Chen, C. (2021). Graphdiyne: From preparation to biomedical applications. Chem. Res. Chin. Univ. 37, 1176–1194. doi:10.1007/s40242-021-1343-8
Lierova, A., Jelicova, M., Nemcova, M., Proksova, M., Pejchal, J., Zarybnicka, L., et al. (2018). Cytokines and radiation-induced pulmonary injuries. J. Radiat. Res. 59 (6), 709–753. doi:10.1093/jrr/rry067
Liu, G., Bao, Z., and Wu, J. (2020). Injectable baicalin/F127 hydrogel with antioxidant activity for enhanced wound healing. Chin. Chem. Lett. 31 (7), 1817–1821. doi:10.1016/j.cclet.2020.03.005
Lohmann, N., Schirmer, L., Atallah, P., Wandel, E., Ferrer, R. A., Werner, C., et al. (2017). Glycosaminoglycan-based hydrogels capture inflammatory chemokines and rescue defective wound healing in mice. Sci. Transl. Med. 9 (386), eaai9044. doi:10.1126/scitranslmed.aai9044
Macmillan, M. S., Wells, M., MacBride, S., Raab, G. M., Munro, A., and MacDougall, H. (2007). Randomized comparison of dry dressings versus hydrogel in management of radiation-induced moist desquamation. Int. J. Radiat. Oncology*Biology*Physics 68 (3), 864–872. doi:10.1016/j.ijrobp.2006.12.049
Maria, O. M., Eliopoulos, N., and Muanza, T. (2017). Radiation-induced oral mucositis. Front. Oncol. 7, 89. doi:10.3389/fonc.2017.00089
Marofi, F., Alexandrovna, K. I., Margiana, R., Bahramali, M., Suksatan, W., Abdelbasset, W. K., et al. (2021). MSCs and their exosomes: A rapidly evolving approach in the context of cutaneous wounds therapy. Stem Cell Res. Ther. 12 (1), 597. doi:10.1186/s13287-021-02662-6
Martin, P., and Nunan, R. (2015). Cellular and molecular mechanisms of repair in acute and chronic wound healing. Br. J. Dermatol. 173 (2), 370–378. doi:10.1111/bjd.13954
Meghrajani, C. F., Co, H. C., Ang-Tiu, C. M., and Roa, F. C. (2013). Topical corticosteroid therapy for the prevention of acute radiation dermatitis: A systematic review of randomized controlled trials. Expert Rev. Clin. Pharmacol. 6 (6), 641–649. doi:10.1586/17512433.2013.841079
Montay-Gruel, P., Meziani, L., Yakkala, C., and Vozenin, M. C. (2019). Expanding the therapeutic index of radiation therapy by normal tissue protection. Br. J. Radiol. 92 (1093), 20180008. doi:10.1259/bjr.20180008
Nagane, M., Yasui, H., Kuppusamy, P., Yamashita, T., and Inanami, O. (2021). DNA damage response in vascular endothelial senescence: Implication for radiation-induced cardiovascular diseases. J. Radiat. Res. 62 (4), 564–573. doi:10.1093/jrr/rrab032
Nie, X., Xiao, D., Wang, W., Song, Z., Yang, Z., Chen, Y., et al. (2015). Comparison of porcine small intestinal submucosa versus polypropylene in open inguinal hernia repair: A systematic review and meta-analysis. PloS one 10 (8), e0135073. doi:10.1371/journal.pone.0135073
Omidvari, S., Saboori, H., Mohammadianpanah, M., Mosalaei, A., Ahmadloo, N., Mosleh-Shirazi, M. A., et al. (2007). Topical betamethasone for prevention of radiation dermatitis. Indian J. dermatol. Venereol. Leprol. 73 (3), 209. doi:10.4103/0378-6323.32755
Paderno, A., Piazza, C., Bresciani, L., Vella, R., and Nicolai, P. (2016). Microvascular head and neck reconstruction after (chemo)radiation: Facts and prejudices. Curr. Opin. Otolaryngol. Head. Neck Surg. 24 (2), 83–90. doi:10.1097/moo.0000000000000243
Panasiti, V., Devirgiliis, V., Borroni, R. G., Rossi, M., Curzio, M., Mancini, M., et al. (2006). Management of skin ulcers in a patient with mycosis fungoides. Dermatol. Online J. 12 (2), 16. doi:10.5070/d30882t9vx
Park, J. W., Hwang, S. R., and Yoon, I. S. (2017). Advanced growth factor delivery systems in wound management and skin regeneration. Mol. (Basel, Switz. 22 (8), 1259. doi:10.3390/molecules22081259
Pérez-Torres, I., Castrejón-Téllez, V., Soto, M. E., Rubio-Ruiz, M. E., Manzano-Pech, L., and Guarner-Lans, V. (2021). Oxidative stress, plant natural antioxidants, and obesity. Int. J. Mol. Sci. 22 (4), 1786. doi:10.3390/ijms22041786
Pizzino, G., Irrera, N., Cucinotta, M., Pallio, G., Mannino, F., Arcoraci, V., et al. (2017). Oxidative stress: Harms and benefits for human health. Oxidative Med. Cell. Longev. 2017, 1–13. doi:10.1155/2017/8416763
Prada, P. J., Fernandez, J., Martinez, A. A., de la Rua, A., Gonzalez, J. M., Fernandez, J. M., et al. (2007). Transperineal injection of hyaluronic acid in anterior perirectal fat to decrease rectal toxicity from radiation delivered with intensity modulated brachytherapy or EBRT for prostate cancer patients. Int. J. Radiat. Oncology*Biology*Physics 69 (1), 95–102. doi:10.1016/j.ijrobp.2007.02.034
Rahmani, H., Najafi, S. H. M., Ashori, A., Fashapoyeh, M. A., Mohseni, F. A., and Torkaman, S. (2020). Preparation of chitosan-based composites with urethane cross linkage and evaluation of their properties for using as wound healing dressing. Carbohydr. Polym. 230, 115606. doi:10.1016/j.carbpol.2019.115606
Ramadan, R., Baatout, S., Aerts, A., and Leybaert, L. (2021). The role of connexin proteins and their channels in radiation-induced atherosclerosis. Cell. Mol. Life Sci. 78 (7), 3087–3103. doi:10.1007/s00018-020-03716-3
Reyes-Ortega, F., Cifuentes, A., Rodriguez, G., Aguilar, M. R., Gonzalez-Gomez, A., Solis, R., et al. (2015). Bioactive bilayered dressing for compromised epidermal tissue regeneration with sequential activity of complementary agents. Acta Biomater. 23, 103–115. doi:10.1016/j.actbio.2015.05.012
Robijns, J., Lodewijckx, J., Claes, S., Van Bever, L., Pannekoeke, L., Censabella, S., et al. (2021). Photobiomodulation therapy for the prevention of acute radiation dermatitis in head and neck cancer patients (DERMISHEAD trial). Radiother. Oncol. 158, 268–275. doi:10.1016/j.radonc.2021.03.002
Roy, I., Fortin, A., and Larochelle, M. (2001). The impact of skin washing with water and soap during breast irradiation: A randomized study. Radiotherapy Oncol. 58 (3), 333–339. doi:10.1016/s0167-8140(00)00322-4
Russi, E. G., Merlano, M. C., Comino, A., and Numico, G. (2007). Ultrathin hydrocolloid dressing in skin damaged from alternating radiotherapy and chemotherapy plus cetuximab in advanced head and neck cancer (G.O.N.O. AlteRCC Italian trial): In regard to macmillan et al. (Int J radiat oncol biol phys 2007;68:864-872). Int. J. Radiat. Oncology*Biology*Physics 69 (2), 638–639. doi:10.1016/j.ijrobp.2007.05.063
Ryan, J. L., Heckler, C. E., Ling, M., Katz, A., Williams, J. P., Pentland, A. P., et al. (2013). Curcumin for radiation dermatitis: A randomized, double-blind, placebo-controlled clinical trial of thirty breast cancer patients. Radiat. Res. 180 (1), 34–43. doi:10.1667/rr3255.1
Saldin, L. T., Cramer, M. C., Velankar, S. S., White, L. J., and Badylak, S. F. (2017). Extracellular matrix hydrogels from decellularized tissues: Structure and function. Acta Biomater. 49, 1–15. doi:10.1016/j.actbio.2016.11.068
Sárközy, M., Varga, Z., Gáspár, R., Szűcs, G., Kovács, M. G., Kovács, Z. Z. A., et al. (2021). Pathomechanisms and therapeutic opportunities in radiation-induced heart disease: From bench to bedside. Clin. Res. Cardiol. 110 (4), 507–531. doi:10.1007/s00392-021-01809-y
Schaue, D., Micewicz, E. D., Ratikan, J. A., Xie, M. W., Cheng, G., and McBride, W. H. (2015). Radiation and inflammation. Semin. Radiat. Oncol. 25 (1), 4–10. doi:10.1016/j.semradonc.2014.07.007
Seegenschmiedt, H. (2006). Management of skin and related reactions to radiotherapy. Front. Radiat. Ther. Oncol. 39, 102–119. doi:10.1159/000090855
Sheng, L., Zhang, Z., Zhang, Y., Wang, E., Ma, B., Xu, Q., et al. (2021). A novel "hot spring"-mimetic hydrogel with excellent angiogenic properties for chronic wound healing. Biomaterials 264, 120414. doi:10.1016/j.biomaterials.2020.120414
Shukla, R., Kashaw, S. K., Jain, A. P., and Lodhi, S. (2016). Fabrication of Apigenin loaded gellan gum-chitosan hydrogels (GGCH-HGs) for effective diabetic wound healing. Int. J. Biol. Macromol. 91, 1110–1119. doi:10.1016/j.ijbiomac.2016.06.075
Singh, M., Alavi, A., Wong, R., and Akita, S. (2016). Radiodermatitis: A review of our current understanding. Am. J. Clin. Dermatol. 17 (3), 277–292. doi:10.1007/s40257-016-0186-4
Sivaraj, D., Chen, K., Chattopadhyay, A., Henn, D., Wu, W., Noishiki, C., et al. (2021). Hydrogel scaffolds to deliver cell therapies for wound healing. Front. Bioeng. Biotechnol. 9, 660145. doi:10.3389/fbioe.2021.660145
Song, A., Rane, A. A., and Christman, K. L. (2012). Antibacterial and cell-adhesive polypeptide and poly(ethylene glycol) hydrogel as a potential scaffold for wound healing. Acta Biomater. 8 (1), 41–50. doi:10.1016/j.actbio.2011.10.004
Sonis, S. T. (2021). Superoxide dismutase as an intervention for radiation therapy-associated toxicities: Review and profile of avasopasem manganese as a treatment option for radiation-induced mucositis. Drug Des. devel. Ther. 15, 1021–1029. doi:10.2147/dddt.s267400
Spałek, M. (2016). Chronic radiation-induced dermatitis: Challenges and solutions. Clin. Cosmet. Investig. Dermatol. 9, 473–482. doi:10.2147/ccid.s94320
Srinivas, A., and Ramamurthi, A. (2007). Effects of gamma-irradiation on physical and biologic properties of crosslinked hyaluronan tissue engineering scaffolds. Tissue Eng. 13 (3), 447–459. doi:10.1089/ten.2006.0196
Sroussi, H. Y., Epstein, J. B., Bensadoun, R. J., Saunders, D. P., Lalla, R. V., Migliorati, C. A., et al. (2017). Common oral complications of head and neck cancer radiation therapy: Mucositis, infections, saliva change, fibrosis, sensory dysfunctions, dental caries, periodontal disease, and osteoradionecrosis. Cancer Med. 6 (12), 2918–2931. doi:10.1002/cam4.1221
Straccia, M. C., d'Ayala, G. G., Romano, I., Oliva, A., and Laurienzo, P. (2015). Alginate hydrogels coated with chitosan for wound dressing. Mar. drugs 13 (5), 2890–2908. doi:10.3390/md13052890
Straub, J. M., New, J., Hamilton, C. D., Lominska, C., Shnayder, Y., and Thomas, S. M. (2015). Radiation-induced fibrosis: Mechanisms and implications for therapy. J. Cancer Res. Clin. Oncol. 141 (11), 1985–1994. doi:10.1007/s00432-015-1974-6
Sun, H., Hong, Y., Xi, Y., Zou, Y., Gao, J., and Du, J. (2018). Synthesis, self-assembly, and biomedical applications of antimicrobial peptide-polymer conjugates. Biomacromolecules 19 (6), 1701–1720. doi:10.1021/acs.biomac.8b00208
Sun, J., and Tan, H. (2013). Alginate-based biomaterials for regenerative medicine applications. Mater. (Basel) 6 (4), 1285–1309. doi:10.3390/ma6041285
Tao, B., Lin, C., Deng, Y., Yuan, Z., Shen, X., Chen, M., et al. (2019). Copper-nanoparticle-embedded hydrogel for killing bacteria and promoting wound healing with photothermal therapy. J. Mat. Chem. B 7 (15), 2534–2548. doi:10.1039/c8tb03272f
Tapio, S., Little, M. P., Kaiser, J. C., Impens, N., Hamada, N., Georgakilas, A. G., et al. (2021). Ionizing radiation-induced circulatory and metabolic diseases. Environ. Int. 146, 106235. doi:10.1016/j.envint.2020.106235
Thi, P. L., Lee, Y., Tran, D. L., Thi, T. T. H., Kang, J. I., Park, K. M., et al. (2020). In situ forming and reactive oxygen species-scavenging gelatin hydrogels for enhancing wound healing efficacy. Acta Biomater. 103, 142–152. doi:10.1016/j.actbio.2019.12.009
Van den Plas, D. L., Lambrecht, A., Jacobs, D., Liekens, J., and Van Hoey, M. (2009). Treatment of recalcitrant wounds of diverse etiology with a new hydroactive gel. Wounds : A compendium of clinical research and practice. Wounds. 21 (9), 243–248.
Viyoch, J., Sudedmark, T., Srema, W., and Suwongkrua, W. (2005). Development of hydrogel patch for controlled release of alpha-hydroxy acid contained in tamarind fruit pulp extract. Int. J. Cosmet. Sci. 27 (2), 89–99. doi:10.1111/j.1467-2494.2004.00250.x
Voci, S., Gagliardi, A., Molinaro, R., Fresta, M., and Cosco, D. (2021). Recent advances of taxol-loaded biocompatible nanocarriers embedded in natural polymer-based hydrogels. Gels (Basel, Switz. 7 (2), 33. doi:10.3390/gels7020033
Vogt, C., Xing, Q., He, W., Li, B., Frost, M. C., and Zhao, F. (2013). Fabrication and characterization of a nitric oxide-releasing nanofibrous gelatin matrix. Biomacromolecules 14 (8), 2521–2530. doi:10.1021/bm301984w
Walker, R. M., Gillespie, B. M., Thalib, L., Higgins, N. S., and Whitty, J. A. (2017). Foam dressings for treating pressure ulcers. Cochrane Database Syst. Rev. 10 (10), Cd011332. doi:10.1002/14651858.cd011332.pub2
Wang, H., Xu, Z., Zhao, M., Liu, G., and Wu, J. (2021). Advances of hydrogel dressings in diabetic wounds. Biomater. Sci. 9 (5), 1530–1546. doi:10.1039/d0bm01747g
Wang, W., Zhang, X., Chao, N. N., Qin, T. W., Ding, W., Zhang, Y., et al. (2016). Preparation and characterization of pro-angiogenic gel derived from small intestinal submucosa. Acta Biomater. 29, 135–148. doi:10.1016/j.actbio.2015.10.013
Wang, Y., Boerma, M., and Zhou, D. (2016). Ionizing radiation-induced endothelial cell senescence and cardiovascular diseases. Radiat. Res. 186 (2), 153–161. doi:10.1667/rr14445.1
Wasiak, J., Cleland, H., Campbell, F., and Spinks, A. (2013). Dressings for superficial and partial thickness burns. Cochrane Database Syst. Rev. 2013 (3), Cd002106. doi:10.1002/14651858.cd002106.pub4
Wilder, R. B., Barme, G. A., Gilbert, R. F., Holevas, R. E., Kobashi, L. I., Reed, R. R., et al. (2010). Cross-linked hyaluronan gel reduces the acute rectal toxicity of radiotherapy for prostate cancer. Int. J. Radiat. Oncology*Biology*Physics 77 (3), 824–830. doi:10.1016/j.ijrobp.2009.05.069
Wooding, H., Yan, J., Yuan, L., Chyou, T. Y., Gao, S., Ward, I., et al. (2018). The effect of mepitel film on acute radiation-induced skin reactions in head and neck cancer patients: A feasibility study. Br. J. Radiol. 91 (1081), 20170298. doi:10.1259/bjr.20170298
Wu, J., Xiao, Z., Chen, A., He, H., He, C., Shuai, X., et al. (2018). Sulfated zwitterionic poly(sulfobetaine methacrylate) hydrogels promote complete skin regeneration. Acta Biomater. 71, 293–305. doi:10.1016/j.actbio.2018.02.034
Xie, J., Zhao, M., Wang, C., Zhu, S., Niu, W., Yong, Y., et al. (2022). External use of nano-graphdiyne hydrogel for skin radioprotection via both physically shielding of low-energy x-ray and chemically scavenging of broad-spectrum free radicals. Chem. Eng. J., 132866. doi:10.1016/j.cej.2021.132866
Xu, Y., Li, Y., Chen, Q., Fu, L., Tao, L., and Wei, Y. (2018). Injectable and self-healing chitosan hydrogel based on imine bonds: Design and therapeutic applications. Int. J. Mol. Sci. 19 (8), 2198. doi:10.3390/ijms19082198
Xu, Z., Han, S., Gu, Z., and Wu, J. (2020). Advances and impact of antioxidant hydrogel in chronic wound healing. Adv. Healthc. Mat. 9 (5), e1901502. doi:10.1002/adhm.201901502
Yan, H., Chen, J., and Peng, X. (2012). Recombinant human granulocyte-macrophage colony-stimulating factor hydrogel promotes healing of deep partial thickness burn wounds. Burns 38 (6), 877–881. doi:10.1016/j.burns.2012.02.001
Yang, H. Z., Wang, W. K., Yuan, L. L., Wang, S. B., Luo, G. X., Wu, J., et al. (2013). Multi-center clinical trial of FLAMIGEL (hydrogel dressing) for the treatment of residual burn wound. Zhonghua Shao Shang Za Zhi 29 (2), 177–180. doi:10.3760/cma.j.issn.1009-2587.2013.02.021
Zeng, M. F., Xiao, H. Q., Zhang, X., Sun, X. D., Qi, C. Z., and Wang, B. Y. (2011). A Novel Chitosan/polyvinyl pyrrolidone (CS/PVP) three-dimensional composite and its mechanism of strength improvement. J. Macromol. Sci. Part B 50 (7), 1413–1422. doi:10.1080/00222348.2011.562072
Zhang, A., Liu, Y., Qin, D., Sun, M., Wang, T., and Chen, X. (2020). Research status of self-healing hydrogel for wound management: A review. Int. J. Biol. Macromol. 164, 2108–2123. doi:10.1016/j.ijbiomac.2020.08.109
Zhang, J., Zhu, Y., Zhang, Y., Lin, W., Ke, J., Liu, J., et al. (2021). A balanced charged hydrogel with anti-biofouling and antioxidant properties for treatment of irradiation-induced skin injury. Mater. Sci. Eng. C 131, 112538. doi:10.1016/j.msec.2021.112538
Zhang, Y., and Huang, Y. (2020). Rational design of smart hydrogels for biomedical applications. Front. Chem. 8, 615665. doi:10.3389/fchem.2020.615665
Zhao, R., Liang, H., Clarke, E., Jackson, C., and Xue, M. (2016). Inflammation in chronic wounds. Int. J. Mol. Sci. 17 (12), 2085. doi:10.3390/ijms17122085
Zhong, W. H., Tang, Q. F., Hu, L. Y., and Feng, H. X. (2013). Mepilex lite dressings for managing acute radiation dermatitis in nasopharyngeal carcinoma patients: A systematic controlled clinical trial. Med. Oncol. 30 (4), 761. doi:10.1007/s12032-013-0761-y
Keywords: radiation therapy, skin and mucosa damages, wound healing, tissue regeneration, hydrogel dressings
Citation: Su Y, Cui H, Yang C, Li L, Xu F, Gao J and Zhang W (2022) Hydrogels for the treatment of radiation-induced skin and mucosa damages: An up-to-date overview. Front. Mater. 9:1018815. doi: 10.3389/fmats.2022.1018815
Received: 14 August 2022; Accepted: 28 September 2022;
Published: 11 October 2022.
Edited by:
Ruodan Xu, China Academy of Chinese Medical Sciences, ChinaReviewed by:
Ganesh Ingavle, Symbiosis International University, IndiaJianglei Qin, Hebei University, China
Xiao Lin, Soochow University, China
Copyright © 2022 Su, Cui, Yang, Li, Xu, Gao and Zhang. This is an open-access article distributed under the terms of the Creative Commons Attribution License (CC BY). The use, distribution or reproduction in other forums is permitted, provided the original author(s) and the copyright owner(s) are credited and that the original publication in this journal is cited, in accordance with accepted academic practice. No use, distribution or reproduction is permitted which does not comply with these terms.
*Correspondence: Fei Xu, ZmVpXzMwN0AxNjMuY29t; Jie Gao, Z2FvamllaGlnaGNsZWFAMTYzLmNvbQ==; Wenjun Zhang, Z2lmdDEwMTNAMTYzLmNvbQ==
†These authors have contributed equally to this work and share first authorship