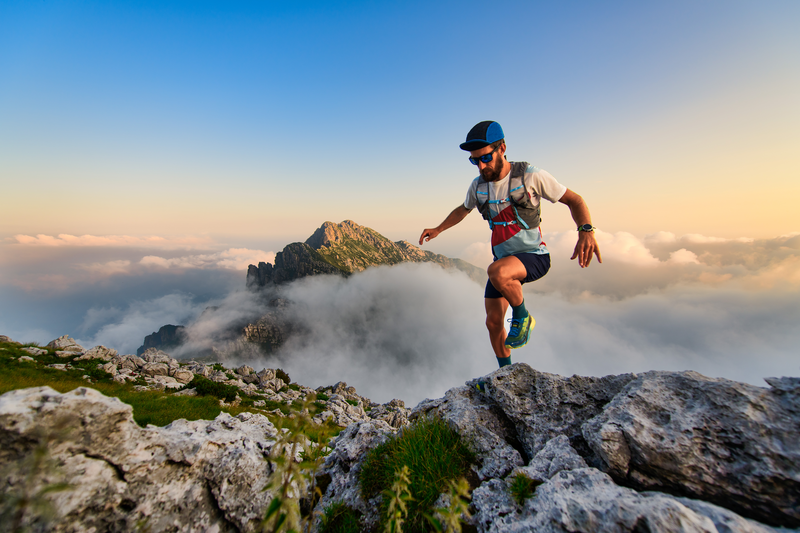
95% of researchers rate our articles as excellent or good
Learn more about the work of our research integrity team to safeguard the quality of each article we publish.
Find out more
ORIGINAL RESEARCH article
Front. Mater. , 13 September 2022
Sec. Computational Materials Science
Volume 9 - 2022 | https://doi.org/10.3389/fmats.2022.1013580
This article is part of the Research Topic Ultra-high Performance Concrete: Computation and Simulation Methods View all 5 articles
The enhancing effects of microbe cement on municipal solid waste incineration (MSWI) fly ash have been modeled by molecular dynamics and investigated experimentally. Molecular dynamics is utilized to explore the adsorption mechanism of heavy metal (Pb ions) in cementitious materials in four aspects: intensity distribution, mean square displacement (MSD), radial distribution function (RDF) and time correlation functions (TCF), revealing that the stable formation of chemical adsorption with C-S-H is the main reason. So the leaching of heavy metals can be reduced with cement hydration such as microbe cement with MSWI fly ash. Furthermore, the strengthening effects of microbe cement are inspected experimentally with a compression test, X-ray diffraction, scanning electron microscopy and atomic absorption spectrometer with a content range from 0 to 60%. The results of compressive strength, microstructure inspection and heavy metals’ leaching concentration show that the optimum content of microbe cement for solidifying and enhancing MSWI fly ash is 30% above range.
In order to solve the current problem of municipal solid waste, incineration technology is widely used to treat municipal solid waste, which tends to produce a large amount of municipal solid waste incineration (MSWI) fly ash. According to the Chinese Catalogue of Hazardous Wastes, waste incineration fly ash contains many hazardous substances that harm the environment and human beings (Jin et al., 2007). China’s standard GB16889-2008 “Standard for Pollution Control on the Landfill Site of Municipal Solid Waste” stipulates that MSWI fly ash must be appropriately cured or stabilized before landfilling. Curing technology is widely used to process MSWI fly ash (GB16889-2008, 2008; Zhu et al., 2011; Shi and Yuan, 2003; Gencel et al., 2012a; Gencel et al., 2012b; Uygunoğlu et al., 2012; Bilir et al., 2015; Tekin et al., 2017). However, cement production can be resource-intensive and release large amounts of CO2, negatively affecting the environment (Liu et al., 2007; Jin, 2016). Therefore, there is an urgent need to find a method to process MSWI fly ash.
Research scholars have studied microbial cement with low energy consumption and environmental friendliness for production to solve the problem by using a gel material to replace cement. At present, much research has been conducted at home and abroad on the properties of microbial cement and its applications. Shen (Shen, 2009) used the technology of microbial induced calcite precipitation (MICP) to repair the cracks of clay brick. The results showed that the tensile strength of the repaired clay brick could be as high as 0.5 MPa. Leelm et al. (Soon et al., 2013) used the calcium carbonate induced by Bacillus megaterium to treat the residual soil in Malaysia. They found that the shear strength and impermeability of the residual soil were improved. Our research team successfully cemented loose sand grains into sand columns with mechanical properties using the MICP technique. It proposed a cementation mechanism based on intermolecular hydrogen bonding between the organic matrix in calcite generated by microbial mineralization and the silicon in the sand (Rong et al., 2013; Rong, 2014).
The comprehensive study above found that the current research on microbial cement mainly focuses on modifying porous materials, curing loose sand particles, and repairing construction material defects. The research related to curing MSWI fly ash with smaller particle size, larger specific surface area, and higher heavy metal content is less. In this work, we attempted to explain the strengthening mechanism of microbe cement with molecular simulation on leaching heavy metals and solidify the MSWI fly ash by microbe cement and determine the optimum content of microbe cement. The adsorption process of heavy metals is modeled by the interactions between C-S-H and Pb ions. Then, the MSWI fly ash was solidified by employing microbe cement with different contents, and the solidification effects were investigated by characterizing the compressive strength, microstructure and leaching concentration of the MSWI fly ash products. The results of the study may provide a reference for the subsequent application in actual engineering.
In this paper, an adsorption model is constructed to study the adsorption mechanism of Pb ions in cement-based materials. Based on the work of Hou et al. (Yu et al., 2021), a C-S-H model with calcium to silicon ratios of 1.7 and 1.9 was established. The C-S-H matrix is cut along the interlayer direction to form exposed C-S-H surfaces. A PbCl2 solution with a concentration of 1 mol/L was placed at 6 Å on the surface of the C-S-H substrate, leaving a vacuum layer of about 4 nm on top of the solution. Taking 1.7 as an example, and the simulated box size was 21.60 × 45.04 × 100 Å3. ClayFF force field was unitized to simulate the interaction of C–S–H gel (Cygan et al., 2004). The van der Waals forces parameters for Pb ions were adopted from Sourav et al. (Mondal et al., 2012). Fix the lower edge of the C-S-H base and relax the system under the NVT ensemble. The time step and temperature were set to 1 fs and 300 K. The C-S-H and PbCl2 solutions were relaxed for 2 ns, respectively. The matrix was then equilibrated with the solution for 2 ns. Continue to run for another 2 ns to acquire and record trajectory information. The equilibrium C-S-H 1.7 adsorption model is shown in Figure 1.
The MSWI fly ash selected for this experiment was obtained from a waste incineration plant in Qinhuangdao, Hebei Province, China. Its physical phase analysis and microscopic morphology are shown in Figures 2, 3, respectively. Figure 2 shows that the main constituents of MSWI fly ash are NaCl, KCl, Ca2SiO3, CaSO4, and SiO2, and also contain some solid solution phases, such as CaAl2Si2O3 and Mg3Si4O10(OH)2. Figure 3 shows that the micro-morphology of MSWI fly ash is complex and irregular.
MSWI fly ash is classified as hazardous waste mainly because it contains heavy metals. Table 1 shows heavy metals’ contents and leaching concentrations in the MSWI fly ash. As seen in Table 1, the heavy metals in MSWI fly ash mainly include four species (Cu, Pb, Zn, and Cd), among which the leaching concentrations of Cu and Zn are much lower than our standard “Identification Standard for Solid Waste Leaching Toxicity.” In comparison, Pb and Cd leaching concentrations are higher than the standard. Therefore, subsequent experiments were conducted mainly to solidify two heavy metals, Pb and Cd.
The microorganism used in the study was a kind of urease-forming bacterium (Bacillus pasteurii, DSM 33, obtained from German Collection of Microorganisms and Cell Cultures), which can decompose urea to form CO32-. The main components of the microbial medium (Rong et al., 2020) were 5.0 g/L peptone and 3.0 g/L beef paste. After adjusting the pH of the medium to 7.0, the medium was sterilized at 121°C for 20 min and cooled on a sterile operation table. The medium was inoculated at a 2% volume fraction and incubated in a shaker at 35°C (oscillation frequency of 170 r/min) for 24 h before use. The concentration of the bacterial solution is 108 cells·mL-1.
Added 100 ml of cultured bacterial solution into the beaker, then added 50 ml of 2 mol/L urea solution and 50 ml of 2 mol/L CaCl2 solutions and left it to react for 8 hours. After removing the supernatant, the beaker was dried at 45°C for 24 h to obtain white precipitate-microbial cement. The reaction equations of bacteria induced calcium carbonate precipitation are shown in Eqs 1–3.
Firstly, microbe cement with different contents (0, 10, 20, 30, 40, 50 and 60% of the total mass) was respectively mixed with 30 g MSWI fly ash. Then, 19 ml supernatant solution was added to each mixture. Mixed them well and poured them into plastic molds (Φ3 × 12 cm), shaking them to make the interior as uniform as possible to reduce air bubbles. The mold was cured at 45°C for 3 days and then removed. Finally, the product is cut into small pieces of Φ3 × 3 cm and cured continuously at 80°C for 7 days.
The compressive strength of specimens of size Φ3 × 3 cm was tested using a compression testing machine (TYE-300, JIANYI, China). The loading rate was set at 5 mm/min. The results were averaged over three tests for each microbial cement content group. After the compression test, the specimens were analyzed for the physical phase using an x-ray diffractometer (XRD, JN-210, Rigaku, Japan). Before analysis, the samples were washed three times with anhydrous ethanol to minimize the effect of the organic matrix and other impurities.
In order to acquire the micro-morphology, a small piece of the unbroken solidification products was selected to be observed by using scanning electron microscopy (SEM, JSM-7800F, IEOL, Japan). Before observing, the samples were dried in the oven at 80°C for 24 h and sprayed gold for 120 s.
Extractant 2# was selected for the test experiment according to the Chinese standard “Solid Waste-Extraction Procedure for Leaching Toxicity-Acetic Acid Buffer Solution Method” (HJ/T 300-2007, 2008). Extractant 2# was obtained by diluting 17.25 ml of glacial acetic acid to 1 L (pH 2.64 ± 0.05). The cured products were treated with extractant 2#, and the leaching concentrations of heavy metals in different products were analyzed by atomic absorption spectrometer (900-T, EWAI, China).
As shown in Figure 4, clear adsorption peaks of Pb2+ (35Å) and Cl-(32Å) can be found near the C-S-H 1.7 surface. However, multiple adsorption peaks of Pb2+ appeared on the surface of C-S-H 1.9, which indicated that in C-S-H with a high calcium-silicon ratio, Pb2+ would invade the interior of the C-S-H matrix.
FIGURE 4. The intensity distribution of ions in different surface adsorption models: (A) C-S-H 1.7 (B) C-S-H 1.9.
The calculated results of the mean square displacement (MSD) of water molecules (Ow) and ions are shown in Figure 5. It is clear that the order of slopes in the equilibrium phase of both adsorption models are Ow>Cl->Pb2+. The diffusion behavior of water molecules is the most violent, and the diffusion behavior of Pb2+ is the slowest.
The spatial correlation between ions and water molecules was evaluated by radial distribution function (RDF), as shown in Figure 6. According to the RDF peak position of Pb-Ow in models C-S-H 1.7 and 1.9, its bond length is 2.7Å, which is almost no different from the existing literature (Bhattacharjee et al., 2009). The Cl-Ow bond length is about 3.2 Å. Not only that, Pb-Ow, has better solvation structure dynamic stability than Cl-Ow, as evidenced by the results of time correlation functions (TCF) in Figure 7. TCF can evaluate the dynamic behavior of the core-shell structure of ionic water, that is, the exchange frequency of ions with surrounding water molecules. The faster the TCF decays, the faster the exchange frequency, which means the lower the dynamic stability of the chemical bond. The TCF value of Cl-Ow decays the fastest, indicating that the surrounding water molecules are constantly iterated and updated. However, for Pb2+, the TCF decays more slowly, which means that its hydrated shell structure can remain reasonably intact.
As shown in Figure 8A, it can be seen from the RDF calculation results that there is no chemical bond between Pb2+ and oxygen in the silicon chain. Pb2+ is indirectly adsorbed to the C-S-H surface through the water core-shell structure (Figure 8B). So Pb2+ does not directly adsorb to the surface of C-S-H but forms relatively stable with C-S-H by chemical adsorption through the water core-shell structure, indicating that cement hydration products, such as microbe cement with MSWI fly ash, can restrain the leaching of heavy metals (Pb ions). On the C-S-H surface with a high calcium-silicon ratio, there are more silicon-calcium structural defects, and Pb2+ has more adsorption capacity.
FIGURE 8. (A) RDFs of ions and oxygen in silicate chains (B) the snapshot of Pb2+ adsorption outside the C-S-H interphase zone.
The solidification products of MSWI fly ash under different microbe cement contents are shown in Figure 9. As seen in Figure 9, the loose MSWI fly ash particles can all solidify together with no significant difference in appearance at different microbial cement admixtures. However, the compression testing results (Figure 10) show that when the microbe cement content increased from 0 to 30%, the compressive strength of the products increased from 0.28 to 0.59 MPa, while it decreased from 0.59 to 0.07 MPa as the microbe cement content continued to increase from 30 to 60%. The reason for the above may be because when the microbial cement content was more than 30%, it would cause new pores to be created, resulting in a loose overall structure and a decrease in compressive strength.
FIGURE 9. Solidification products of MSWI fly ash under different microbe cement contents: (A) 0%; (B) 10%; (C) 20%; (D) 30%; (E) 40%; (F) 50%; (G) 60%.
The XRD results of the different solidification products are shown in Figure 11. It can be seen that a new substance (Ca2SiO4.0.30H2O) was generated in the products. Similar to the hydration of Portland cement to produce C-S-H gel, the MSWI fly ash contains some minerals such as Ca3SiO5 and CaAl2Si2O8 (Figure 2), which could also occur in the hydration reaction to generate gel material (Ca2SiO4.0.30H2O). Both Ca2SiO4-0.30H2O and C-S-H gels have the cementing ability to solidify loose MSWI fly ash particles into a whole and have a certain mechanical strength.
In addition, our previous studies show that microbe cement contains some organic matter secreted by bacteria. The hydroxyl group (-OH) in the organic matrix interacts with the oxygen atoms in the Si-O bond and C-O bond in the sand or MSWI fly ash to form intermolecular hydrogen bonds (O-H---O) (Rong et al., 2013; Rong, 2014; Rong et al., 2019). The formation of O-H---O enhanced the interaction between loose MSWI fly ash particles and has a positive effect on the improvement of compressive strength. The schematic diagrams of the intermolecular hydrogen bonds between the -OH and the C-O bonds, the -OH and the Si-O bonds are shown in Figure 12.
FIGURE 12. Schematic diagrams of the formation of intermolecular hydrogen bonds: (A) Hydrogen bonds between Si-O and -OH; (B) Hydrogen bonds between C-O and -OH.
The microstructures of MSWI fly ash products solidified by microbe cement with different contents are shown in Figure 13.
FIGURE 13. Microstructures of MSWI products solidified by different microbe cement contents: (A) 0%; (B) 10%; (C) 20%; (D) 30%; (E) 40%; (F) 50%; (G) 60%.
It can be seen that when the microbe cement content increased from 0 to 30%, the pore size in the products became smaller and the microstructure became denser, while the changes were opposite with the microbe cement content continuing to increase from 30 to 60%. The changes of the microstructure of the solidification products indicate that different microbe cement contents have different effects on the microstructure. When the microbe cement content was less than 30%, the pores and gaps between MSWI fly ash particles were filled by the microbe cement, which made the microstructure of the solidification products denser. However, when the content was more than 30%, some new pores and gaps were formed due to the accumulation of the excessive amount of microbe cement. In addition, the excessive microbe cement mixed in the hydration product (Ca2SiO4.0.30H2O), which had a negative effect on the cementation of Ca2SiO4.0.30H2O. In this situation, the enhancing effect of O-H---O was not enough to compensate for the deficiencies caused by the excessive microbe cement. The microstructure of the solidification product became looser, and the strength of the products decreased.
The leaching concentrations of Pb and Cd of the MSWI fly ash products solidified by microbe cement with different contents are shown in Figure 14.
It can be seen that with the microbe cement content increasing from 0 to 30%, the leaching concentrations of Pb and Cd decreased. However, when the microbe cement content continued to increase from 30 to 60%, the leaching concentrations of the two heavy metals increased. When the microbe cement was 30%, the leaching concentrations of heavy metals were the lowest. After solidifying, the leaching concentrations of Pb and Cd were 4.6336 mg·L-1 and 1.7012 mg·L-1, respectively. Combined with Table 1, the solidification rates of Pb and Cd were 35 and 31%, respectively. Ultimately, the leaching concentration of Pb was lower than the Identification Standard for Solid Waste Leaching Toxicity, while that of Cd was still slightly higher than the standard. Subsequent research needs further exploration to meet the specification requirements and achieve practical application.
The decrease of leaching concentrations of heavy metals in the MSWI fly ash products is mainly attributed to the following aspects: 1) The Ca2SiO4.0.30H2O, a gel matter generated by the hydration of MSWI fly ash, has certain adsorption and encapsulation effect on heavy metals (Lv, 2012); 2) With the addition of microbe cement, a large number of intermolecular hydrogen bonds are formed in the solidification products. The existence of hydrogen bonds strengthens the interaction between particles, which has a certain solidification effect on heavy metals; 3) Due to the metabolism of microbial cells, microbial cement contains a certain amount of extracellular polymeric substance (EPS). The solidification of heavy metals by EPS is mainly through electrostatic interaction and complexation (Jin et al., 2019). 4) The molecular simulation in this paper verified that chemical adsorption of microbe cement hydration products (C-S-H) with heavy metals could reduce the leaching of Pb ions. However, according to the SEM analysis, when the microbe cement content was larger than 30%, some new pores were formed, the cementation of Ca2SiO4.0.30H2O reduced, the strength of solidification products decreased and thereby the leaching concentrations of heavy metals increased.
In this work, microbe cement was used to solidify the MSWI fly ash theoretically and experimentally. Meanwhile, the solidification effects of MSWI fly ash under different microbe cement contents were studied. Based on the study, the following conclusions are drawn.
1) The stable chemical adsorption with C-S-H through the water core-shell structure contributes mainly to the adsorption of Pb2+ rather than direct adsorption, which can be intensified with a high calcium-silicon ratio, thus restraining the leaching of heavy metals.
2) Due to the generation of hydrated gel matter Ca2SiO4.0.30H2O, the loose MSWI fly ash particles could be solidified together. Moreover, the hydrated gel matter also has a certain solidification effect on heavy metals.
3) The addition of microbe cement resulted in the formation of intermolecular hydrogen bonds, which not only improved the strength of MSWI fly ash product, but also enhanced the solidification effect of heavy metals. In addition, the microbe cement contains some EPS, which also has a certain ability to solidify the heavy metals.
4) The optimum content of microbe cement is 30%. At the content, the microstructure of the MSWI fly ash product is the densest, the compressive strength is the highest (0.59 MPa) and the leaching concentrations of heavy metals are the lowest. After solidifying, the leaching concentrations of Pb and Cd are 4.6336 mg·L-1 and 1.7012 mg·L-1, respectively (the solidification rates of Pb and Cd are 35 and 31%, respectively). Ultimately, the leaching concentration of Pb meets the standard requirement, while that of Cd is still slightly higher than the standard.
The original contributions presented in the study are included in the article/supplementary material, further inquiries can be directed to the corresponding author.
MS: Conceptualization, Methodology, Formal analysis, Writing-original draft. KH: Conceptualization, Methodology, Writing-review and editing. YZ: Conceptualization, Methodology, Writing-review and editing. GW: Data curation, Writing-review and editing. HR: Resources, Writing-review and editing.
This work was supported by the National Nature Science Foundation of China (Grant No. 51978439, 52178264), Project funded by China Postdoctoral Science Foundation (Grant No. 2019M651000), Tianjin Transportation Science and Technology Development Plan Project (Grant No. 2018-38), Shenzhen High-level Talents Research Start-up Fund, Shenzhen Institute of Information Technology Research Start-up Fund (Grant No. SZIIT2022KJ015).
Author YZ was employed by the company Beijing Municipal Luqiao Ruicheng Technology Co., Ltd.
The remaining authors declare that the research was conducted in the absence of any commercial or financial relationships that could be construed as a potential conflict of interest.
All claims expressed in this article are solely those of the authors and do not necessarily represent those of their affiliated organizations, or those of the publisher, the editors and the reviewers. Any product that may be evaluated in this article, or claim that may be made by its manufacturer, is not guaranteed or endorsed by the publisher.
Bhattacharjee, A., Hofer, T. S., Pribil, A. B., Randolf, B. R., Lim, L. H. V., Lichtenberger, A. F., et al. (2009). Revisiting the hydration of Pb (II): A QMCF MD approach. J. Phys. Chem. B 113 (39), 13007–13013. doi:10.1021/jp905848x
Bilir, T., Gencel, O., and Topcu, I. B. (2015). Properties of mortars with fly ash as fine aggregate. Constr. Build. Mater. 93, 782–789. doi:10.1016/j.conbuildmat.2015.05.095
Cygan, R. T., Liang, J. J., and Kalinichev, A. G. (2004). Molecular models of hydroxide, oxyhydroxide, and clay phases and the development of a general force field. J. Phys. Chem. B 108 (4), 1255–1266. doi:10.1021/jp0363287
GB16889-2008 (2008). Standard for pollution control on the landfill site of manicipal solid waste. China: China Environmental Science Press.
Gencel, O., Koksal, F., Ozel, C., and Brostow, W. (2012). Combined effects of fly ash and waste ferrochromium on properties of concrete. Constr. Build. Mater. 29, 633–640. doi:10.1016/j.conbuildmat.2011.11.026
Gencel, O., Ozel, C., Koksal, F., Erdogmus, E., Martínez-Barrera, G., and Brostow, W. (2012). Properties of concrete paving blocks made with waste marble. J. Clean. Prod. 21 (1), 62–70. doi:10.1016/j.jclepro.2011.08.023
HJ/T 300-2007 (2008). Solid waste-extraction procedure for leaching toxicity-acetic acid buffer solution method. China: China Environmental Science Press.
Jin, J., Wang, Q., Li, H., Tian, S. L., Hu, X. Y., Huang, Q. F., et al. (2007). Distribution and leaching characteristics of heavy metals in MSWI fly ash. Environ. Sci. Technol. 30 (4), 1–4.
Jin, M. J. (2016). Study on technology of solidification of municipal solid wastes incineration (MSWI) fly ash by cement. Chin. J. Environ. Eng. 10 (6), 3235–3241.
Jin, R. N., Wang, X. Y., Lin, X., and Huo, M. X. (2019). Research progress in the extracellular polymeric substances and their adsorption effects on heavy metals. Ind. Water Treat. 39 (1), 8–13.
Liu, Y., Kuang, Y. Q., Huang, N. S., Wu, Z. F., and Wang, C. P. (2007). Analysis of the stresses of population and economic growth on CO2 emissions from cement manufacturing. Res. Environ. Sci. 20 (1), 118–122.
Lv, Y. (2012). Research on the solidification effect and its mechanism of heavy metals in MSWI fly ash-cement clinker. Wuhan: Wuhan University of Technology.
Mondal, S., Ghosh, S., and De, S. (2012). A molecular simulation based assessment of binding of metal ions on micelles. Langmuir 28 (31), 11329–11336. doi:10.1021/la301928a
Rong, H. (2014). Preparation and binding mechanism of microbe cement. Nanjing: Southeast University.
Rong, H., Qian, C. X., and Li, Z. L. (2013). Cementation mechanism of microbe cement. J. Chin. Ceram. Soc. 41 (3), 314–319.
Rong, H., Wei, G. Q., Ma, G. W., Zhang, Y., Zheng, X., Zhang, L., et al. (2020). Influence of bacterial concentration on crack self-healing of cement-based materials. Constr. Build. Mat. 244, 118372. doi:10.1016/j.conbuildmat.2020.118372
Rong, H., Wei, G. Q., Zhang, L., Zhang, Y., Xu, R., Ning, C. Z., et al. (2019). Consolidation of municipal solid waste incineration fly ash by microbial cementing material: Effect and mechanism. Mater. Rep. 33 (11), 3757–3767.
Shen, J. Y. (2009). Experiments and applications of bio-geo materials. Beijing: Tsinghua University.
Shi, H. S., and Yuan, L. (2003). Cementitious reactivity of municipal solid waste incineration fly ash and immobilization effect by cement. J. Chin. Ceram. Soc. 31 (11), 1021–1025.
Soon, N. W., Lee, L. M., Tan, C. K., and Ling, H. S. (2013). Improvements in engineering properties of soils through microbial-induced calcite precipitation. KSCE J. Civ. Eng. 17 (4), 718–728. doi:10.1007/s12205-013-0149-8
Tekin, I., Durgun, M. Y., Gencel, O., Bilir, T., Brostow, W., and Lobland, H. (2017). Concretes with synthetic aggregates for sustainability. Constr. Build. Mater. 133, 425–432. doi:10.1016/j.conbuildmat.2016.12.110
Uygunoğlu, T., Topcu, I. B., Gencel, O., and Brostow, W. (2012). The effect of fly ash content and types of aggregates on the properties of pre-fabricated concrete interlocking blocks (PCIBs). Constr. Build. Mater. 30, 180–187. doi:10.1016/j.conbuildmat.2011.12.020
Yu, J., Hou, D. S., and Zhang, J. R. (2021). Molecular dynamics study on ultra-confined NaCl solution in the silane coupling agent modified rubber calcium silicate hydrate nano-pore. Constr. Build. Mater. 270, 121418. doi:10.1016/j.conbuildmat.2020.121418
Keywords: microbe cement, MSWI fly ash, molecular dynamics, radial distribution function, heavy metals’ leaching, compressive strength, microstructure
Citation: Sun M, Hu K, Zhang Y, Wei G and Rong H (2022) Molecular simulation and experimental investigation on enhancement of microbe cement in municipal solid waste incineration fly ash. Front. Mater. 9:1013580. doi: 10.3389/fmats.2022.1013580
Received: 07 August 2022; Accepted: 29 August 2022;
Published: 13 September 2022.
Edited by:
Xinpeng Wang, Qingdao University of Technology, ChinaReviewed by:
Depeng Chen, Anhui University of Technology, ChinaCopyright © 2022 Sun, Hu, Zhang, Wei and Rong. This is an open-access article distributed under the terms of the Creative Commons Attribution License (CC BY). The use, distribution or reproduction in other forums is permitted, provided the original author(s) and the copyright owner(s) are credited and that the original publication in this journal is cited, in accordance with accepted academic practice. No use, distribution or reproduction is permitted which does not comply with these terms.
*Correspondence: Kaiyue Hu, ODQ3MzQzNTE5QHFxLmNvbQ==
Disclaimer: All claims expressed in this article are solely those of the authors and do not necessarily represent those of their affiliated organizations, or those of the publisher, the editors and the reviewers. Any product that may be evaluated in this article or claim that may be made by its manufacturer is not guaranteed or endorsed by the publisher.
Research integrity at Frontiers
Learn more about the work of our research integrity team to safeguard the quality of each article we publish.