- 1The State Key Laboratory Breeding Base of Basic Science of Stomatology (Hubei-MOST) and Key Laboratory for Oral Biomedicine of Ministry of Education (KLOBM), School and Hospital of Stomatology, Wuhan University, Wuhan, China
- 2Department of Cariology and Endodontics, School and Hospital of Stomatology, Wuhan University, Wuhan, China
- 3School of Power and Mechanical Engineering, The Institute of Technological Science, Wuhan University, Wuhan, China
This study investigates the repair bond strength of aged resin composites after removing different thicknesses, determine the repair performance using the same or different resin composites and describe the treated surfaces after ageing. Seventy simulated class I cavities were prepared in extracted human third molars were randomly divided into two groups and restored with a nanofilled (Filtek Z350) or a microhybrid (Clearfil APX) composite. Five specimens without ageing in each group acted as a positive control for microtensile bond strength (MTBS) test. After thermocycling, each group was randomly divided into two subgroups: Group RT1, 1 mm removed and Group RT3, 3 mm removed, followed by roughening. Ten specimens in each subgroup were repaired with the same or different composites, and MTBS tests were conducted. The surface roughness (Sa), and water contact angle of the remaining five specimens in each subgroup were measured. In every combination group, Group RT3 showed significantly higher MTBS values than Group RT1, and identical composite was not compulsory for higher repair bond strength. Removal thickness had no significant effect on the Sa in same composite group. In both the Z350 and APX groups, the water contact angle decreased with increasing removal thickness.
Introduction
Resin composites are extensively used in the treatment of dental defects because of their excellent aesthetic properties and physical properties. However, marginal microleakage, secondary caries, fracture defects, discolouration and wear influence the survival of resin composites (Drummond, 2008; Sampaio et al., 2019), and the restoration requires monitoring, refurbishing, repairing, or replacing (Hickel et al., 2010; Hickel et al., 2013). Repair is considered a minimally invasive approach requiring new restorative material to be added to replace the defective portion of an otherwise intact restoration (Hickel et al., 2013); when compared with replacement, repair can retard the “restoration death spiral” and save chair-side time and treatment cost (Blum et al., 2014; Kanzow et al., 2016).
Several retrospective studies have already demonstrated that the annual failure rate of restorations can be reduced when repair is not regarded as failure, and the longevity of repaired restorations can be prolonged, allowing their lifetimes to be similar to those of replacement restorations (Kanzow et al., 2016; Valente et al., 2016; Casagrande et al., 2017; Kanzow and Wiegand, 2020). However, Kanzow and Wiegand’s retrospective study indicated that among repaired restorations, the rate of failure of the repaired part was still higher than that of the remaining parts of the original restoration (Kanzow and Wiegand, 2020). It has also been widely investigated inin vitro studies that an increase in roughness of the aged composite surface through etching, air abrasion, polishing, and laser irradiation results in a considerable improvement in the bond strength (Barcellos et al., 2015; Valente et al., 2015; Yokokawa et al., 2015; Pilo et al., 2016; Nagano et al., 2018; Flury et al., 2019; Kanzow et al., 2019; Martos et al., 2019). Brosh et al. indicated that the repair bond between the original and newly added resin composite could be influenced by chemical and mechanical mechanisms: reaction with the surface resin matrix and exposed inorganic fillers could account for chemical bonding and macro/micromechanical attachment could account for mechanical bonding (Loomans et al., 2011; Pilo et al., 2016), when we repair the aged resin composites that had defects, such as fracture defects, discolouration, treatments are needed to create a surface for better adhesive between aged composite substrate and newly added composite. Nevertheless, the interface between the aged resin composite and the new repair part may be still the weakest link in the assembly (Valente et al., 2016), and a gold standard for the treatment of aged resin composites is absent.
left
It is worth noting that in a typical clinical situation, the clinician is unable to distinguish the composition of the aged resin composite and therefore may select a different material. Another important factor is the type of new and aged resin composites. Different resin composites have different physicochemical properties and therefore age differently (Bagheri et al., 2007; Curtis et al., 2009; Ilie and Hickel, 2009). Some authors have indicated that nanofilled composites have a higher repair bond strength than hybrid composites (Rinastiti et al., 2011; Ozcan et al., 2013). However, Cerda-Rizo et al. found that there was no statistically significant difference between the repair bond strength of nanofilled and microhybrid resin composites (Cerda-Rizo et al., 2019), and Daiki Nagano et al. indicated that nanofilled composites were inferior to microhybrid composites for repair (Nagano et al., 2018). There is still no consensus on which composite types are more suitable for repairing.
Consequently, the primary aim of this study was to investigate the repair bond strength of aged nanofilled and microhybrid resin composites after removing different thicknesses and repairing them using either the same or different composites. The secondary aim was to describe the surfaces of aged nanofilled and microhybrid resin composites after removing different thicknesses. The null hypotheses in this test were that there would be no significant difference in repair bond strength between 1) groups with different removal thicknesses, 2) different types of aged resin composites, and 3) different resin composite combinations.
Materials and Methods
Specimen Preparation
The experimental procedure used in this study is schematically illustrated in Figure 1. The compositions and manufacturers of the two resin composites and an universal adhesive used in this study are shown in Table 1. The study was approved by the Human Research Ethics Committee ([2017] Lun Shen Zi (1)), and conducted according to the Helsinki Declaration of 1975 (revised in 2002). Seventy intact caries-free human third molars with bucco-lingual dimensions of 10 (±0.5) mm, extracted for periodontal or orthodontic reasons, were collected after obtaining the patients’ informed consent. Then, after cleaning, the teeth were preserved in 0.5 wt% thymol (Sigma-Aldrich, St. Louis, MO, United States) solution at 4°C for no more than 2 months before experimentation (D Ziskind et al., 2003; Chen et al., 2020). The cusps were removed to create a flat occlusal surface via a low-speed Isomet saw (Isomet; Buehler, Evanston, IL, United States) perpendicular to the long axis of the tooth. Then, standard Class I cavities with a length, width and depth of 4, 4, and 4 mm were drilled with a flat-end diamond bur (SF13, MANI, Japan) performed with high speed and water spray. This preparation was performed by a specialist of cariology and assessed by another specialist of cariology in order to maintain consistency. After every five cavities, the bur was replaced. A william’s periodontal probe (Shanghai Jiawei dental instrument Ltd., Shanghai, China) was used to control the dimension.
Single bond universal (3M ESPE, St. Paul, MN, United States) was used in a self-etch mode in all experimental groups according to the manufacturer’s instructions. After the cavity was rubbed with brush dipped with adhesive for 20 s, the universal adhesive was air-dried for 5 s and light-cured with an LED light-curing system (Bisco Inc, Schaumburg, IL, United States)) at an output of 700 mW/cm2 for 10 s. Then, these cavities were filled with resin composites in two equal horizontal layers, and each layer was light-cured for 20 s. The simulated restorations were polished with wet silicon carbide papers after curing. The specimens, which had been randomly divided into two groups, were filled with two different resin composites: Group APX was filled with microhybrid Clearfil AP-X, and Group Z350 was filled with nanofilled Filtek Z350. All specimens were stored for 24 h in distilled water at 23 ± 1.0°C room temperature after curing.
Then, sixty specimens (n = 30/group) were aged in a temperature cycling machine (Thermo Fisher, HUAN-S Ltd., Wuhan, China), with 20,000 thermocycles at 5°C and 55°C with an immersion duration of 30 s for each bath and a transfer time of 5 s between baths. Another ten specimens (n = 5/group) were used as a positive control for the microtensile bond strength test without thermocycling.
Removal of Aged Resin Composite
After thermocycling, a flat-end diamond bur (SF13, MANI, Japan) was used to remove different thicknesses from the sixty aged resin composites as indicated by William’s periodontal probe (Shanghai Jiawei dental instrument Ltd., Shanghai, China). After every five preparations, a bur was replaced. The specimens were randomly divided into two subgroups and processed as follows: Group RT1, 1 mm of the aged composite was removed and Group RT3, 3 mm of the aged composite was removed.
Repair of Aged Resin Composite
The surfaces of twenty specimens in each group were roughened by a medium-grit bur (SF13, MANI, Japan) installed on a high-speed handpiece. Unidirectional movements were applied to the surfaces for 10 s under copious air–water spray, followed by washing and drying with oil-free compressed air. Before roughing, a digital balance was employed to train the operator to have a good command of maintaining a manual pressure of ∼0.04 N over the specimens (Valente et al., 2015). All specimens were prepared by the same operator. After every five preparations, a bur was replaced.
All the walls of the one- or 3-mm depth cavities were rubbed with adhesive for 20 s, gently air-dried for 5 s, and light-cured for 10 s. Then, a 1 mm × 4 mm × 4 mm layer of new resin composite in Groups RT1 and RT3 was added to the aged resin composite. Another 2 mm-thick resin composite was added to the new resin composite to allow for sufficient specimen length for easy microtensile bond testing. In the groups with different thicknesses removed, the resin composite used for repair was the same as that of the aged resin composite (Z350-Z350 and APX-APX) or different from that of the aged resin composite (Z350-APX and APX-Z350). Every combination group contained five specimens. The control group (n = 5/group) corresponded to the newly filled resin composite without the thermocycling described above.
Microtensile Bond Strength Analysis
After being repaired and preserved in water at 23 ± 1.0°C room temperature for 24 h, each resin composite was cut perpendicularly to the adhesive interface, and the ultimate cross-sectional area of each beam was approximately 1 mm2 (approximately 1 mm × 1 mm). For every combination group, 20 beams were selected randomly, and the microtensile bond strength (MTBS) was tested. The beams were fixed on the testing apparatus (Bisco Inc, Schaumburg, IL, United States) with cyanoacrylate, keeping the adhesive zone free, and measured at a cross-head speed of 1 mm/min. By measuring each beam’s dimension with a digital calliper and recording the maximum tensile force, the MTBS values (MPa) were calculated from dividing the maximum tensile force by the beam cross sectional area.
Failure Analysis
Fractured surfaces of the beams were observed under optical microscope with a magnification ×45 (Wild Heerbrugg Type 308700, Euromex, Heerbrugg, Switzerland). The failure modes were classified into three categories: adhesive failure occurred in the interface between substrate resin composite and adhesive layer or adhesive layer and repair resin composite without remains from other materials; cohesive failure occurred within the substrate, or within the new resin composite (including the adhesive layer and/or repair resin composite), and mixed failure at both the adhesive and cohesive areas.
Characterization of the Treated Surfaces
Another five aged resin composites in each group were randomly selected. The specimens were roughened as described above, and the surrounding tooth structure was removed. Then, the treated surfaces of the aged resin composite were examined. For surface topography testing, the specimens were analysed by a 3D optical profilometer (NewviewTM9000, Zygo, United States). Scanning was conducted for each specimen over three randomly selected 1,497.361 µm × 1,497.361 µm areas. The average of the three readings was recorded as the Sa value of each specimen.
Two microlitres of deionized water was dropped on the treated surface of the aged composite, and a profile image was photographed after 5 s to measure the contact angles via droplet shape analysis (OCA25, Dataphysics, Germany) at room temperature. Five specimens were measured for each group 2 times each to obtain an average measurement per specimen. Finally, the specimens were sputter-coated with a gold layer to aid conductivity and examined using FESEM (Sigma, Zeiss, Germany) at accelerating operating voltages of 20 kV in the secondary electron mode for taking 1,000× magnification micrographs.
Statistical Analysis
SPSS (IBM SPSS Statistics 20; IBM Corp) was used for statistical analysis. For analysis of the MTBS, three-way analysis of variance (ANOVA) was performed with the type of aged resin composite, removal thickness and type of new resin composite as the independent variables. The contact angle and surface roughness data were subjected to two-way ANOVA with the type of aged resin composite (nanofilled versus microhybrid) and the removal thickness (1 versus 3 mm) as the independent variables. The homoscedasticity of the data was validated via Tukey’s test. Multiple comparisons were conducted using post-hoc Tukey’s test. Chi-square test was used for failure mode analysis. The significance level was defined at 0.05 for all experiments.
Results
Microtensile Bond Strength
The MTBS data for all groups are displayed in Table 2. In the same combination group, a significant difference in MTBS was observed among different removal thicknesses. The control group exhibited significantly higher MTBS values than all other experimental groups. Group RT3 showed significantly higher MTBS values than Group RT1. Three-way ANOVA revealed that the aged resin composite factor (F = 9.130, p = 0.003) and removal thickness (F = 346.419, p = 0.000) factor had significant impacts on the MTBS value. Significant interactions (F = 5.993, p = 0.016) were identified between the aged resin composite and the new resin composite. Individual analysis of aged resin composite and new resin composite has been conducted and when the new resin composite was Z350, the aged resin composite played a significant role in MTBS (F = 14.958, p = 0.000). In Group RT1, Z350-Z350 showed significantly higher MTBS values than APX-Z350 (p = 0.029). This result indicated that the removal thickness, type of aged resin composite and resin composite combination contributed to the changes in MTBS. However, in Group RT3, the results of multiple comparisons indicated that the bond strength in different resin composite combinations was not significantly different.
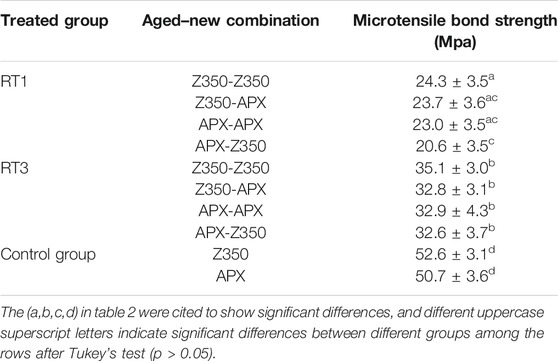
TABLE 2. Means and standard deviations (MPa) of MTBS for Group RT1 and Group RT3 repaired with the same and dissimilar composites.
Failure Mode Analysis
The failure frequency is displayed in Figure 2. The chi-square test of failure mode (p = 0.023) showed significant difference between different experimental groups. In the Group APX, the most frequent failure mode was adhesive or mixed. In the Group Z350, cohesive failures within the new resin composite and the substrate occurred more than 45%.
Characterization of the Treated Surfaces
The surface roughness results are shown in Figure 3. The average Sa (mean ± SD) of the Z350 RT1, Z350 RT3, APX RT1, and APX RT3 groups were 7.895 ± 0.454, 8.546 ± 0.913, 6.868 ± 1.166, and 8.036 ± 0.914, respectively. Two-way ANOVA revealed that neither the removal thickness (F = 0.413, p = 0.530) nor the aged resin composite (F = 3.652, p = 0.074) had a significant effect on Sa. However, compared with APX in Group RT1, Z350 in Group RT3 had higher surface roughness values. Representative FESEM images are shown in Figure 4. The FESEM figures showed that irregularities were composed of exposed fillers, fillers surrounded by resin matrix, porosities and micro-relief produced by mechanical roughening.
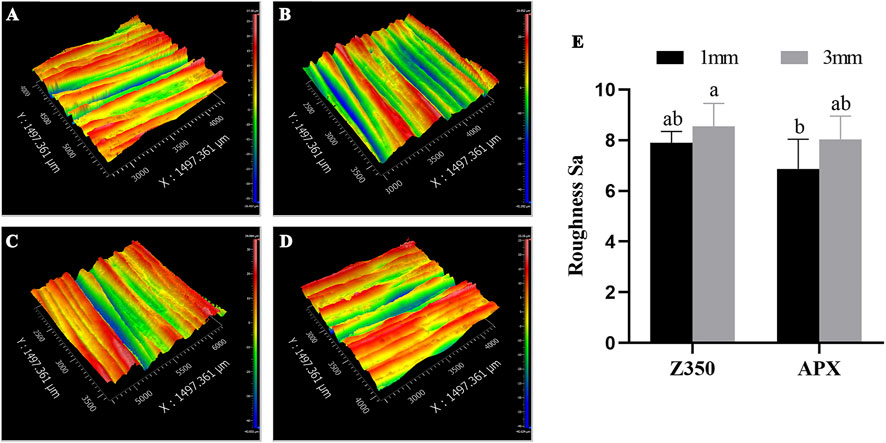
FIGURE 3. (A–D) Representative figures of surface roughness measurement from the Z350 in Group RT1 (A), Z350 in Group RT3 (B), APX in Group RT1 (C), APX in Group RT3 (D). The Sa of surface roughness of each group (E). Groups with the same superscripts are not significantly different (p > 0.05). RT1, removal thickness 1 mm; RT3, removal thickness 3 mm; Z350, Filtek Z350; APX, Clearfil AP-X.
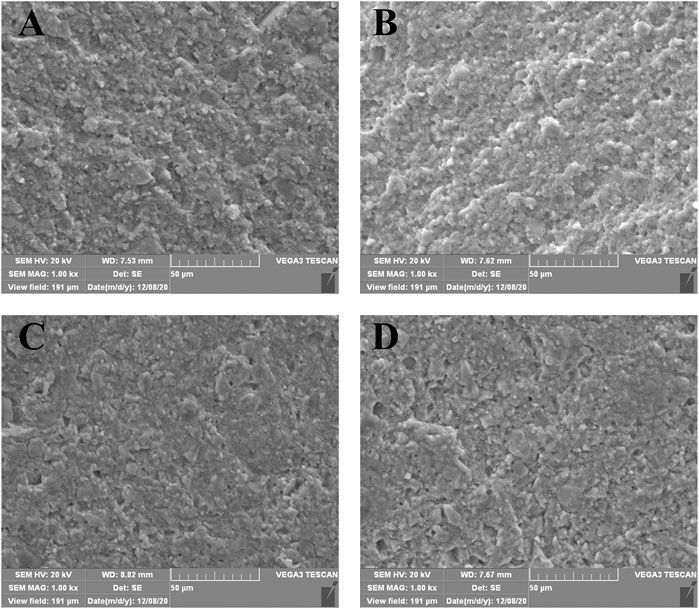
FIGURE 4. Representative FESEM figures (1,000X) after treatment. Z350 in Group RT1 (A). Z350 in Group RT3 (B). APX in Group RT1 (C). APX in Group RT3 (D). RT1, removal thickness 1 mm; RT3, removal thickness 3 mm; Z350, Filtek Z350; APX, Clearfil AP-X.
The water contact angle results are presented in Figure 5. The average water contact angles (mean ± SD) of the Z350 RT1, Z350 RT3, APX RT1, and APX RT3 groups were 62.6 ± 2.619, 54.92 ± 1.889, 69.18 ± 3.005, and 58.6 ± 4.067, respectively. Two-way ANOVA revealed that the removal thickness (F = 46.311, p = 0.000) and aged resin composite (F = 14.621, p = 0.001) had a significant effect on the water contact angle. The water contact angle decreased with increasing removal thickness in the same resin composite group and Group Z350 showed lower water contact angles than Group APX in the same removal thickness group.
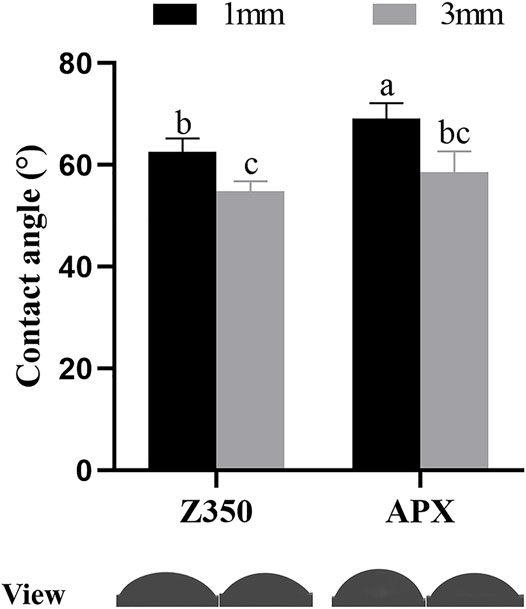
FIGURE 5. The degree of water contact angle and respective views of the different groups on the aged resin composite surfaces. Groups with same letters are not statistically different (p > 0.05)
Discussioin
At present, the resistance of repaired dental composites which is an important topic in dentistry while reparation is minimally invasive procedure rather than a complete removal of a restoration which could also produce damage risks to the remaining tooth structure and even dental pulp. Repair is gradually accepted by clinicians, but how to achieve bonding between old and new resin at the same cohesive strength as the new resin is unsolved (Kanzow et al., 2019). Clinicians doubt that removing superficial sections will achieve more stable adhesion. Evidence about whether the ageing degree at different depths influences the repair bond strength is scarce. According to the limited research, no predictions of removal thickness can be made for the highest repair bond strength, but adhering the new resin to a deep surface in the first horizontal layer of aged resin composite was considered to generate more favourable results than a superficial surface in the second horizontal layer of aged resin composite for repair. In addition, it seems impossible for clinicians to distinguish the type of aged resin composite, but in our study, the type of new resin composite added for repair played a small role in bonding strength.
In this study, to obtain surfaces with same depth that could be aged more uniformly, the specimen used was different from that used in previous studies. Resin blocks were used in previous studies, and then the specimen was removed from the mould during the ageing process (Ozcan et al., 2007; de Moraes et al., 2008; Bürgers et al., 2009; Rinastiti et al., 2011; Eliasson et al., 2014; Pilo et al., 2016; Nagano et al., 2018; Alqarni et al., 2019). This process might lead to a reduction in the difference in the effect of temperature on the external and internal properties of the resin composite. However, in our study, the teeth surrounded the resin composite in class I cavity so that the resin composites were aged from the upper surface to the lower surface. In addition, thermal cycling was used to accelerate the ageing of the restorative materials (Morresi et al., 2014; Szczesio-Wlodarczyk et al., 2020). Composite substrates with thermocycling were indicated to result in the worst repair bond compared to substrates that were immersed in boiling water or citric acid (Ozcan et al., 2007). When the specimen assembled with teeth was thermally cycled at 5°C and 55°C, the temperature in the internal part of the resin composite did not reach the water temperatures used in numerous research projects (Gale and Darvell, 1999; Eliasson and Dahl, 2020), this finding is similar to the short-term exposure to extreme temperatures in the oral environment.
The present study found that the repair bond strength in the deep area of aged resin composites was significantly higher than that in the shallow area, regardless of which resin composite combination group was used. The first null hypothesis could be rejected, while the third null hypothesis could not be rejected. When we filled class I cavities, resin composites were light cured in two equal horizontal layers. In this situation, the properties of resin composites with the depth 1 and 3 mm were considered to be same before ageing (Giorgi et al., 2015). After ageing, the properties in different depth were influenced inconsistently and 1 mm represented the upper surface while 3 mm represented the lower surface. The thermal cycling procedure depended not only on hydrolysis but also on thermal degradation (Morresi et al., 2014; Blumer et al., 2015; Szczesio-Wlodarczyk et al., 2020). In the experimental design, the physical and chemical properties of two removal thickness should be the same after light curing because of horizontal layering equally (Yoon et al., 2021). With regard to thermal degradation, the resin matrix and filler had different thermal expansion coefficients and thus, in thermal cycling, the bond between them could be damaged weakening the mechanical properties of the composite resins from strongly degraded external to inner less degraded layers. With regard to hydrolysis, more progressive degradation in the strongly degraded external than inner degraded layers results in further swelling polymeric matrix with water sorption, which allows for unreacted monomers and degradation products to diffuse out of the composite more easily (Szczesio-Wlodarczyk et al., 2020).
In this study, the repair bond strength of aged resin composites was also compared to the cohesive strength of new resin composites in the positive group. In fact, the results seem to be consistent with other research that found that the repair strength of aged resin composites, even in the group with the best bond strength, only reached approximately 60% of the cohesive strength of the new resin composite (Valente et al., 2016). In the control group, oxygen inhibited layer contributes to stable adhesion between two contiguous layers. However, during ageing, unfavourable factors such as water sorption and thermal degradation, that reduce the number of unsaturated double bond and damage the physicochemical properties of resin composite, have adverse effects on the bond strength for aged resin composites (Özcan et al., 2013; Szczesio-Wlodarczyk et al., 2020). Other surface treatments may need to be applied together to obtain better repair performance. In future studies, the resin composites before and after thermal cycling would be analysed with FTIR and some other tests (such as sorption and solubility tests and HPLC) (Özcan et al., 2013; Cebe et al., 2015) to determine the changes in chemical structure and the absolute content of the remaining C=C.
The most interesting observation was that a significant difference in the water contact angle at different depths of the resin composite was found in this study. A lower contact angle in Group RT3 implies better wettability and improved micromechanical retention between aged resin composite and adhesive layer; thus, a higher repair bond strength was acquired (Prado et al., 2018). There are a number of studies characterizing the contact angle of dental polymeric materials after different artificial ageing simulations. Other research groups found a significant decrease in the contact angle when stored in water, ethanol (96% vol), red wine (Yanikian et al., 2019), lipase and with intra-oral wear in the presence of biofilms (Kusuma Yulianto et al., 2019). In addition, the percentage of filler exposure increased after ageing (Rinastiti et al., 2011; Kusuma Yulianto et al., 2019). The notable feature of the methods used in our study was that different thicknesses of aged composites were removed, and the surface was roughened before testing. As mentioned above, consequently, fillers of the aged resin composite could be debonded with the resin matrix during these procedures, and the surface at a depth of 3 mm, which contained more stable hydrophilic fillers, showed a lower contact angle. Another possible reason was that the dispersed part of the monomer chains of the resin matrix may be oriented towards the surface after thermal cycling (Bürgers et al., 2009).
The results of this study indicated that nanofilled resin composites exhibited slightly better repair performance than microhybrid resin composites, which supports evidence from previous observations (Ozcan et al., 2013). The second null hypothesis could be rejected. This might be due to the composition of the resin matrix, in which Z350 involves bis-GMA, bis-EMA, UDMA, and TEGDMA, whereas APX involves only bis-GMA and TEGDMA. UDMA and bis-EMA could form a cross-linked network that absorbs less water than bis-GMA and TEGDMA (Sideridou and Papanastasiou, 2003). Another possible reason is that APX, which represents high-filled composite, has a lower wettability than the higher-filled composite (Baur and Ilie, 2012). It is possible that the filler type and the composition of the resin matrix both influence the consequence of repair. Furthermore, consistent with the literature, evidence from Group RT3 showed that the combination of different materials did not necessarily impair the repair strength (Rinastiti et al., 2011; Ozcan et al., 2013).
The current study found that the employment of medium-grit diamond burs generated irregular and rough surfaces at different depths with no significant difference in Sa in both aged Z350 and APX. The roughing procedure aimed to create a surface for micromechanical retention (Loomans et al., 2011). This procedure was also employed in many published articles for roughing (Rathke et al., 2008; Loomans et al., 2011; Valente et al., 2015; Wendler et al., 2016; Dieckmann et al., 2020). Although roughening the surface of the specimens before reparation procedures had cause an increase of 1 and 3 mm initial composite removal, composite was roughened under a manual pressure of ∼0.04 for only 10 s (Valente et al., 2015) and this increase could be negligible compared to removal thickness. In addition, the same roughening procedure in two removal thickness groups contributed to control variables. It was noted that irregular and rough surfaces resulted in a larger surface area for wetting with the adhesive and micromechanical attachment, thereby favouring repair. However, Lasia L. et al. (Valente et al., 2015) demonstrated that medium-grit burs produced the highest surface roughness compared with fine- and extra-fine-grit burs, while fine-grit burs produced the highest repair bond strength and similar water contact angles. Thays et al. (da Costa et al., 2012) demonstrated that the grits of the diamond burs did not influence the composite repair strength. In general, the present study was consistent with the literature in finding that the surface roughness does not necessarily influence the resin composite repair bond strength.
Last but not least, adhesive played a significant role in the repair bonding. Single bond adhesive contains specific acidic functional monomers which enhance the performance of adhesion (Van Landuyt et al., 2008). The functional monomers help conditioning the substrate surface, increase monomer penetration, and also improve chemical bonding when repairing resin composite (Van Landuyt et al., 2007; Martos et al., 2019). M. Wendler et al. considered that the presence of non-converted carbon double bonds in aged composites contributes to crucial adhesion when repairing resin composites and that the combination of 10-MDP functional monomers or saline could increase the repair bond strength (Wendler et al., 2016).
Some limitations of this study are that only two brands of resin composites were investigated, there was no standard protocol for thermocycling in vitro (Morresi et al., 2014) and physiological conditions, i.e. cyclical loads were not simulated (Frankenberger et al., 2005). Furthermore, even though some steps have been used to better stimulate the clinical situation, the extent to which the results of this study could be concluded for the clinical situation remains unknown. Thus, more brands of resin composites with different formulations should be investigated in future investigations, other physiological conditions should be simulated and clinical studies are needed.
Conclusion
Within the limitations of our study, the following conclusions can be drawn:
1 Removal of different thicknesses influences the repair bond strength of dental resin composites whether nanofiller or microhybrid resin composites are used, that is characterized by adhesion to the inner surface in a horizontal layer producing a statistically higher repair bond strength than adhesion at the corresponding external surface in upper horizontal layer.
2 Thermal cycling affects the resin composites with an impact on the repair bond strengths, with higher repair bond strength being attained for non-aged than for aged resin composite.
3 Aged nanofilled resin composites have better performance in repairing bond strength than microhybrid resin composites.
4 When the types of newly added and aged resin composites are identical, a higher repair bond strength may be obtained, but using the same resin composite to repair the aged resin is not compulsory.
Data Availability Statement
The original contributions presented in the study are included in the article/supplementary material, further inquiries can be directed to the corresponding author.
Ethics Statement
The studies involving human participants were reviewed and approved by The study was conducted according to the guidelines of the Declaration of Helsinki, and approved by the ethics committee for the School and Hospital of Stomatology, Wuhan University, China. The patients/participants provided their written informed consent to participate in this study.
Author Contributions
LZ and KY contributed to conceptualization and methodology of the study; KY, KS and LX performed formal analysis and investigation. KY; KY contributed to data curation of the study; KY wrote the original draft and LZ reviewed and edited the manuscript of the study; LZ and ZC organized supervision of the study; All authors have read and agreed to the published version of the manuscript.
Funding
This work was supported by National Natural Science Foundation of China (grant numbers 81771064, 81974148).
Conflict of Interest
The authors declare that the research was conducted in the absence of any commercial or financial relationships that could be construed as a potential conflict of interest.
Publisher’s Note
All claims expressed in this article are solely those of the authors and do not necessarily represent those of their affiliated organizations, or those of the publisher, the editors and the reviewers. Any product that may be evaluated in this article, or claim that may be made by its manufacturer, is not guaranteed or endorsed by the publisher.
References
Alqarni, D., Nakajima, M., Hosaka, K., Ide, K., Nagano, D., Wada, T., et al. (2019). The Repair Bond Strength to Resin Matrix in Cured Resin Composites after Water Aging. Dent. Mater. J. 38 (2), 233–240. doi:10.4012/dmj.2018-044
Bagheri, R., Tyas, M. J., and Burrow, M. F. (2007). Subsurface Degradation of Resin-Based Composites. Dental Mater. 23 (8), 944–951. doi:10.1016/j.dental.2006.06.035
Barcellos, D. C., Miyazaki Santos, V. M., Niu, L.-N., Pashley, D. H., Tay, F. R., and Pucci, C. R. (2015). Repair of Composites: Effect of Laser and Different Surface Treatments. Int. J. Adhes. Adhes. 59, 1–6. doi:10.1016/j.ijadhadh.2015.01.008
Baur, V., and Ilie, N. (2012). Repair of Dental Resin-Based Composites. Clin. Oral Invest. 17 (2), 601–608. doi:10.1007/s00784-012-0722-4
Blum, I., Lynch, C., and Wilson, N. (2014). Factors Influencing Repair of Dental Restorations with Resin Composite. Ccide 6, 81–87. doi:10.2147/CCIDE.S53461
Blum, I. R. (2019). Restoration Repair as a Contemporary Approach to Tooth Preservation: Criteria for Decision Making and Clinical Recommendtions. Prim. Dent J. 8, 38–43. doi:10.1308/205016819826439466
Blumer, L., Schmidli, F., Weiger, R., and Fischer, J. (2015). A Systematic Approach to Standardize Artificial Aging of Resin Composite Cements. Dental Mater. 31 (7), 855–863. doi:10.1016/j.dental.2015.04.015
Bürgers, R., Cariaga, T., Müller, R., Rosentritt, M., Reischl, U., Handel, G., et al. (2009). Effects of Aging on Surface Properties and Adhesion of Streptococcus Mutans on Various Fissure Sealants. Clin. Oral Invest. 13 (4), 419–426. doi:10.1007/s00784-009-0256-6
Casagrande, L., Laske, M., Bronkhorst, E. M., Huysmans, M. C. D. N. J. M., and Opdam, N. J. M. (2017). Repair May Increase Survival of Direct Posterior Restorations - A Practice Based Study. J. Dent. 64, 30–36. doi:10.1016/j.jdent.2017.06.002
Cebe, M. A., Cebe, F., Cengiz, M. F., Cetin, A. R., Arpag, O. F., and Ozturk, B. (2015). Elution of Monomer from Different Bulk Fill Dental Composite Resins. Dental Mater. 31 (7), e141–e149. doi:10.1016/j.dental.2015.04.008
Cerda-Rizo, E., de Paula Rodrigues, M., Vilela, A., Braga, S., Oliveira, L., Garcia-Silva, T., et al. (2019). Bonding Interaction and Shrinkage Stress of Low-Viscosity Bulk Fill Resin Composites with High-Viscosity Bulk Fill or Conventional Resin Composites. Oper. Dent. 44 (6), 625–636. doi:10.2341/18-163-L
Chen, R., Jin, R., Li, X., Fang, X., Yuan, D., Chen, Z., et al. (2020). Biomimetic Remineralization of Artificial Caries Dentin Lesion Using Ca/P-PILP. Dental Mater. 36 (11), 1397–1406. doi:10.1016/j.dental.2020.08.017
Curtis, A. R., Palin, W. M., Fleming, G. J. P., Shortall, A. C. C., and Marquis, P. M. (2009). The Mechanical Properties of Nanofilled Resin-Based Composites: The Impact of Dry and Wet Cyclic Pre-loading on Bi-axial Flexure Strength. Dental Mater. 25 (2), 188–197. doi:10.1016/j.dental.2008.06.003
da Costa, T. R. F., Serrano, A. M., Atman, A. P. F., Loguercio, A. D., and Reis, A. (2012). Durability of Composite Repair Using Different Surface Treatments. J. Dentistry 40 (6), 513–521. doi:10.1016/j.jdent.2012.03.001
de Moraes, R. R., Marimon, J. L. M., Jochims Schneider, L. F., Sinhoreti, M. A. C., Correr-Sobrinho, L., and Bueno, M. (2008). Effects of 6 Months of Aging in Water on Hardness and Surface Roughness of Two Microhybrid Dental Composites. J. Prosthodont. 17 (4), 323–326. doi:10.1111/j.1532-849X.2007.00295.x
Dieckmann, P., Baur, A., Dalvai, V., Wiedemeier, D. B., Attin, T., and Tauböck, T. T. (2020). Effect of Composite Age on the Repair Bond Strength after Different Mechanical Surface Pretreatments. J. Adhes. Dent. 22 (4), 365–372. doi:10.3290/j.jad.a44867
Drummond, J. L. (2008). Degradation, Fatigue, and Failure of Resin Dental Composite Materials. J. Dent Res. 87 (8), 710–719. doi:10.1177/154405910808700802
Eliasson, S. T., and Dahl, J. E. (2020). Effect of thermal Cycling on Temperature Changes and Bond Strength in Different Test Specimens. Biomater. Invest. Dent. 7 (1), 16–24. doi:10.1080/26415275.2019.1709470
Eliasson, S., Tibballs, J., and Dahl, J. (2014). Effect of Different Surface Treatments and Adhesives on Repair Bond Strength of Resin Composites after One and 12 Months of Storage Using an Improved Microtensile Test Method. Oper. Dent. 39 (5), E206–E216. doi:10.2341/12-429-L
Flury, S., Dulla, F. A., and Peutzfeldt, A. (2019). Repair Bond Strength of Resin Composite to Restorative Materials after Short- and Long-Term Storage. Dental Mater. 35 (9), 1205–1213. doi:10.1016/j.dental.2019.05.008
Frankenberger, R., Pashley, D. H., Reich, S. M., Lohbauer, U., Petschelt, A., and Tay, F. R. (2005). Characterisation of Resin-Dentine Interfaces by Compressive Cyclic Loading. Biomaterials 26 (14), 2043–2052. doi:10.1016/j.biomaterials.2004.07.003
Gale, M. S., and Darvell, B. W. (1999). Thermal Cycling Procedures for Laboratory Testing of Dental Restorations. J. Dent. 27, 89–99. doi:10.1016/S0300-5712(98)00037-2
Germscheid, W., de Gorre, L. G., Sullivan, B., O’Neill, C., Price, R. B., and Labrie, D. (2018). Post-curing in Dental Resin-Based Composites. Dental Mater. 34 (9), 1367–1377. doi:10.1016/j.dental.2018.06.021
Giorgi, M. C., Theobaldo, J., Nunes Leite Lima, D. A., Marchi, G. M., Ambrosano, G. M., and Baggio Aguiar, F. H. (2015). Influence of Successive Light-Activation on Degree of Conversion and Knoop Hardness of the First Layered Composite Increment. Acta Odontol. Scand. 73 (2), 126–131. doi:10.3109/00016357.2014.956334
Hickel, R., Peschke, A., Tyas, M., Mjör, I., Bayne, S., Peters, M., et al. (2010). FDI World Dental Federation: Clinical Criteria for the Evaluation of Direct and Indirect Restorations-Update and Clinical Examples. Clin. Oral Invest. 14 (4), 349–366. doi:10.1007/s00784-010-0432-8
Hickel, R., Brüshaver, K., and Ilie, N. (2013). Repair of Restorations - Criteria for Decision Making and Clinical Recommendations. Dental Mater. 29 (1), 28–50. doi:10.1016/j.dental.2012.07.006
Ilie, N., and Hickel, R. (2009). Investigations on Mechanical Behaviour of Dental Composites. Clin. Oral Invest. 13 (4), 427–438. doi:10.1007/s00784-009-0258-4
Kaiser, C., and Price, R. B. (2020). Effect of Time on the post-irradiation Curing of Six Resin-Based Composites. Dental Mater. 36 (8), 1019–1027. doi:10.1016/j.dental.2020.04.024
Kanzow, P., and Wiegand, A. (2020). Retrospective Analysis on the Repair vs. Replacement of Composite Restorations. Dental Mater. 36 (1), 108–118. doi:10.1016/j.dental.2019.11.001
Kanzow, P., Wiegand, A., and Schwendicke, F. (2016). Cost-Effectiveness of Repairing versus Replacing Composite or Amalgam Restorations. J. Dent. 54, 41–47. doi:10.1016/j.jdent.2016.08.008
Kanzow, P., Wiegand, A., Schwendicke, F., and Göstemeyer, G. (2019). Same, Same, but Different? A Systematic Review of Protocols for Restoration Repair. J. Dent. 86, 1–16. doi:10.1016/j.jdent.2019.05.021
Kruzic, J. J., Arsecularatne, J. A., Tanaka, C. B., Hoffman, M. J., and Cesar, P. F. (2018). Recent Advances in Understanding the Fatigue and Wear Behavior of Dental Composites and Ceramics. J. Mech. Behav. Biomed. Mater. 88, 504–533. doi:10.1016/j.jmbbm.2018.08.008
Kusuma Yulianto, H. D., Rinastiti, M., Cune, M. S., de Haan-Visser, W., Atema-Smit, J., Busscher, H. J., et al. (2019). Biofilm Composition and Composite Degradation during Intra-oral Wear. Dent. Mater. 35 (5), 740–750. doi:10.1016/j.dental.2019.02.024
Loomans, B. A. C., Vivan Cardoso, M., Roeters, F. J. M., Opdam, N. J. M., De Munck, J., Huysmans, M. C. D. N. J. M., et al. (2011). Is There One Optimal Repair Technique for All Composites? Dent. Mater. 27 (7), 701–709. doi:10.1016/j.dental.2011.03.013
Martos, R., Hegedüs, V., Szalóki, M., Blum, I. R., Lynch, C. D., and Hegedüs, C. (2019). A Randomised Controlled Study on the Effects of Different Surface Treatments and Adhesive Self-Etch Functional Monomers on the Immediate Repair Bond Strength and Integrity of the Repaired Resin Composite Interface. J. Dent. 85, 57–63. doi:10.1016/j.jdent.2019.04.012
Morresi, A. L., D'Amario, M., Capogreco, M., Gatto, R., Marzo, G., D'Arcangelo, C., et al. (2014). Thermal Cycling for Restorative Materials: Does a Standardized Protocol Exist in Laboratory Testing? A Literature Review. J. Mech. Behav. Biomed. Mater. 29, 295–308. doi:10.1016/j.jmbbm.2013.09.013
Nagano, D., Nakajima, M., Takahashi, M., Ikeda, M., Hosaka, K., Sato, K., et al. (2018). Effect of Water Aging of Adherend Composite on Repair Bond Strength of Nanofilled Composites. J. Adhes. Dent. 20 (5), 425–433. doi:10.3290/j.jad.a41331
Ozcan, M., Barbosa, S., Melo, R., Galhano, G., and Bottino, M. (2007). Effect of Surface Conditioning Methods on the Microtensile Bond Strength of Resin Composite to Composite after Aging Conditions. Dent. Mater. 23 (10), 1276–1282. doi:10.1016/j.dental.2006.11.007
Özcan, M., Corazza, P. H., Marocho, S. M. S., Barbosa, S. H., and Bottino, M. A. (2013). Repair Bond Strength of Microhybrid, Nanohybrid and Nanofilled Resin Composites: Effect of Substrate Resin Type, Surface Conditioning and Ageing. Clin. Oral Invest. 17 (7), 1751–1758. doi:10.1007/s00784-012-0863-5
Pilo, R., Brosh, T., Geron, V., Levartovsky, S., and Eliades, G. (2016). Effect of Silane Reaction Time on the Repair of a Nanofilled Composite Using Tribochemical Treatment. J. Adhes. Dent. 18 (2), 125–134. doi:10.3290/j.jad.a35907
Prado, M., Prochnow, C., Marchionatti, A. M. E., Baldissara, P., Valandro, L. F., and Wandscher, V. F. (2018). Ceramic Surface Treatment with a Single-Component Primer: Resin Adhesion to Glass Ceramics. J. Adhes. Dent. 20 (2), 99–105. doi:10.3290/j.jad.a40303
Rathke, A., Tymina, Y., and Haller, B. (2008). Effect of Different Surface Treatments on the Composite-Composite Repair Bond Strength. Clin. Oral Invest. 13 (3), 317–323. doi:10.1007/s00784-008-0228-2
Rinastiti, M., Özcan, M., Siswomihardjo, W., Busscher, H. J., and van der Mei, H. C. (2010). Effect of Biofilm on the Repair Bond Strengths of Composites. J. Dent Res. 89 (12), 1476–1481. doi:10.1177/0022034510381395
Rinastiti, M., Özcan, M., Siswomihardjo, W., and Busscher, H. J. (2011). Effects of Surface Conditioning on Repair Bond Strengths of Non-aged and Aged Microhybrid, Nanohybrid, and Nanofilled Composite Resins. Clin. Oral Invest. 15 (5), 625–633. doi:10.1007/s00784-010-0426-6
Sampaio, C. S., Fernández Arias, J., Atria, P. J., Cáceres, E., Pardo Díaz, C., Freitas, A. Z., et al. (2019). Volumetric Polymerization Shrinkage and its Comparison to Internal Adaptation in Bulk Fill and Conventional Composites: A μCT and OCT In Vitro Analysis. Dent. Mater. 35 (11), 1568–1575. doi:10.1016/j.dental.2019.07.025
Sideridou, I., and Papanastasiou, G. (2003). Study of Water Sorption, Solubility and Modulus of Elasticity of Light-Cured Dimethacrylate-Based Dental Resins. Biomaterials 24, 655–665. doi:10.1016/S0142-9612(02)00380-0
Szczesio-Wlodarczyk, A., Sokolowski, J., Kleczewska, J., and Bociong, K. (2020). Ageing of Dental Composites Based on Methacrylate Resins-A Critical Review of the Causes and Method of Assessment. Polymers 12 (4), 882. doi:10.3390/polym12040882
Valente, L. L., Silva, M. F., Fonseca, A. S., Münchow, E. A., Isolan, C. P., and Moraes, R. R. (2015). Effect of Diamond Bur Grit Size on Composite Repair. J. Adhes. Dent. 17 (3), 257–263. doi:10.3290/j.jad.a34398
Valente, L. L., Sarkis-Onofre, R., Gonçalves, A. P., Fernández, E., Loomans, B., and Moraes, R. R. (2016). Repair Bond Strength of Dental Composites: Systematic Review and Meta-Analysis. Int. J. Adhes. Adhes. 69, 15–26. doi:10.1016/j.ijadhadh.2016.03.020
Van Landuyt, K. L., Snauwaert, J., De Munck, J., Peumans, M., Yoshida, Y., Poitevin, A., et al. (2007). Systematic Review of the Chemical Composition of Contemporary Dental Adhesives. Biomaterials 28 (26), 3757–3785. doi:10.1016/j.biomaterials.2007.04.044
Van Landuyt, K. L., Yoshida, Y., Hirata, I., Snauwaert, J., De Munck, J., Okazaki, M., et al. (2008). Influence of the Chemical Structure of Functional Monomers on Their Adhesive Performance. J. Dent. Res. 87 (8), 757–761. doi:10.1177/154405910808700804
Vankerckhoven, H., Lambrechts, P., van Beylen, M., Davidson, C. L., and Vanherle, G. (1982). Unreacted Methacrylate Groups on the Surfaces of Composite Resins. J. Dent. Res. 61 (6), 791–796. doi:10.1177/00220345820610062801
Wendler, M., Belli, R., Panzer, R., Skibbe, D., Petschelt, A., and Lohbauer, U. (2016). Repair Bond Strength of Aged Resin Composite after Different Surface and Bonding Treatments. Materials 9 (7), 547. doi:10.3390/ma9070547
Yanikian, C. R. F., Stape, T. H. S., Tezvergil‐Mutluay, A., and Martins, L. R. M. (2019). Influence of Silica Nanocoating on Stain Susceptibility and Superficial Integrity of Dimethacrylate‐based Composites. Eur. J. Oral Sci. 127, 361–368. doi:10.1111/eos.12627
Yokokawa, M., Rikuta, A., Tsujimoto, A., Tsuchiya, K., Shibasaki, S., Matsuyoshi, S., et al. (2015). Influence of Methyl Mercaptan on the Repair Bond Strength of Composites Fabricated Using Self-Etch Adhesives. Eur. J. Oral Sci. 123 (1), 46–52. doi:10.1111/eos.12164
Yoon, S., Jung, H.-J., Knowles, J. C., and Lee, H.-H. (2021). Digital Image Correlation in Dental Materials and Related Research: A Review. Dental Mater. 37 (5), 758–771. doi:10.1016/j.dental.2021.02.024
Keywords: aged composite repair, surface treatment, bond strength, resin composite, class I cavity
Citation: Yu K, Wan M, Shi K, Xue L, Chen Z and Zhang L (2021) Removal of Different Thicknesses Influences the Repair Bond Strength of Dental Resin Composites. Front. Mater. 8:790165. doi: 10.3389/fmats.2021.790165
Received: 06 October 2021; Accepted: 11 November 2021;
Published: 03 December 2021.
Edited by:
David K Mills, Louisiana Tech University, United StatesReviewed by:
Joanna Mystkowska, Bialystok University of Technology, PolandIdalina Vieira Aoki, University of São Paulo, Brazil
Copyright © 2021 Yu, Wan, Shi, Xue, Chen and Zhang. This is an open-access article distributed under the terms of the Creative Commons Attribution License (CC BY). The use, distribution or reproduction in other forums is permitted, provided the original author(s) and the copyright owner(s) are credited and that the original publication in this journal is cited, in accordance with accepted academic practice. No use, distribution or reproduction is permitted which does not comply with these terms.
*Correspondence: Lu Zhang, luzhang2012@whu.edu.cn