- 1College of Engineering, Inner Mongolia University for the Nationalities, Tongliao, China
- 2College of Information Science and Technology, Dalian Maritime University, Dalian, China
For the purpose of reducing the energy consumption and construction cost of buildings, the preparation process of geopolymer based foamed concrete, which is a novel material of the wall and roof of building, had been studied in detail. Water glass and sodium hydroxide were used as the alkali activator to excite the mixture consists of slag, fly ash and Kaolin to form the geopolymer matrix, and finally the foams generated using the physical foaming method were filled into the geopolymer matrix to produce geopolymer-based foamed concrete blocks. In the preparation process, firstly one of the four parameters of foam content, water-binder ratio, water glass content, and water glass modulus had been changed separately to study the influence of a single factor on the compressive strength, dry density, thermal conductivity and specific strength of foamed concrete blocks. The experimental results show that the above four factors have different degrees of influence on the concerned performances. Next, some representative combinations of these factors were constructed by orthogonal experiment method, and the influence degree of each combination on the concerned performances was determined by means of range analysis. According to the results of analysis, the most important influencing factor in terms of thermal conductivity was the water-binder ratio, followed by foam content, water glass modulus and water glass content. When the foam content is 1.58%, the water-binder ratio is 0.45, the water glass content is 30%, and the water glass modulus is 1.2, the thermal conductivity of the prepared geopolymer foam concrete reaches 0.044 W/(m·K), which satisfies the expected requirements for heating in severe cold areas.
1 Introduction
With the development of society and the increase of population, the problem of energy shortage has become increasingly prominent, and countries all over the world have attached great importance to energy conservation and environmental protection in the construction industry (Huang, 2013). The Chinese government proposed the development of “applicable, economical and green buildings” in the “13th Five-Year Plan” (Fan et al., 2019). As an important part of the building, the design of the enclosure structure is closely related to the building energy consumption (Liu et al., 2014). In recent years, with the continuous enhancement of China’s scientific research and economic strength, relevant functional departments have put forward higher requirements on building environmental protection, and have successively introduced various policies and measures. With the strong support of these favorable policies, reforms in the construction sector have been deepened, and significant progress has been made in the research of building energy-saving technologies and building energy-saving materials (Chang et al., 2016). From the chemical composition, building insulation materials can be divided into organic materials (Silvestre et al., 2016), inorganic materials (Wang et al., 2020) and organic-inorganic composite materials (Yang et al., 2018). In China’s building materials market, organic building materials such as polystyrene foam and polyurethane foam are common (Hou et al., 2018; He, 2017). Compared with inorganic materials, organic materials have the advantages of light weight and low thermal conductivity, which made them favored by the market. Apart from the above advantages, the problems of low ignition point and easy aging of organic building materials can’t be ignored (Vuong et al., 2016). On the morning of March 9, 2021, an indoor fire in the Zhongxin Building in Shijiazhuang City, Hebei Province caused serious casualties and property losses because the fire spread to the insulation layer of the building’s external walls. The intensification of fires caused by the inflammable insulation of the exterior wall of buildings such as this occurs many times a year, seriously endangering national health and economic construction. In order to reduce the fire threat caused by organic building materials, the Ministry of Housing and Urban-Rural Development of the People’s Republic of China has issued a series of announcements to limit the excessive use of organic building materials (Xiao and Ding, 2013). Therefore, inorganic materials represented by foamed concrete have gradually become a research hotspot in the field of building materials (Yang et al., 2021).
Foamed concrete is a kind of lightweight and porous concrete that receives considerable attention from builders worldwide. The irregular voids inside are derived from the foaming agent mixture in the mortar (Amran et al., 2015). The urgent need for energy-saving and environmentally-friendly building technology has promoted the rapid promotion of foam concrete. Pure cement mixed with fine sand is commonly used for preparing foamed concrete with light weight and uniformly distributed discrete bubbles (Ramamurthy et al., 2009). In different countries such as the United Kingdom, Germany, Turkey, the Philippines and Thailand, foam concrete has become a common material required for construction (Mydin and Wang, 2011). In history, the Romans first realized that by adding blood to a mixture of small gravel, coarse sand and hot lime and stirring it with water, the resulting block containing small bubbles was more durable and practical (Hill, 2013). In 1923, Axel Eriksson was granted a patent for Portland cement-based foam concrete (Valore, 1954). After that, major improvements in production equipment and better superplasticizer and foaming agents have allowed foamed concrete to be used on a large scale, and a lot of efforts have been made to comprehensively study the characteristics and behavior of foamed concrete to simplify its usage in building structure application. Foamed concrete can be prepared by two techniques, which are called pre-foaming method and hybrid foaming method. For the former, it is necessary to prepare stable foam with the help of an air compressor, and then mix it with the basic mixture (consisting of cement, sand and water). For the latter, the foaming agent solution is mixed with the base mixture in a high-speed mixer. Since Davidovits introduced geopolymers, the concept of cement-free concrete has been formally put forward (Liu et al., 2016). The concrete can be prepared by a reaction between an alkaline solution and a raw material rich in silica and alumina, followed by curing and drying (Hardjito et al., 2004). The use of geopolymers to replace the ordinary Portland cement (OPC) to make concrete not only significantly reduces the CO2 emissions of the cement industry, but also realizes the reuse of industrial waste residues such as fly ash. In addition, geopolymer concrete also exhibits lower absorptivity than OPC concrete. As a relatively new material, the preparation process, proportion of doped components and material properties of foamed concrete are yet to be studied.
This article mainly studies the preparation technology of foamed concrete, and then expands it to introduce in detail the optimized design of the raw materials and doping ratio of foamed concrete to obtain the ideal compressive strength, dry density, thermal conductivity, and specific strength in the fresh and hardened state. The remainder of this paper is structured as follows: Section 2 provides a complete solution of preparing the geopolymer-based foamed concrete referred in this article. Next, the influence of a single factor among foam content, water-binder ratio, water glass content and water glass modulus on the compressive strength, dry density, thermal conductivity, and specific strength of foamed concrete blocks are described and discussed in Section 3. Apart from this, the results of orthogonal experiment (Xuan and Leung, 2011) and range analysis (Tang et al., 2011) of the combination of various influencing factors will also be presented. At last, the conclusions are drawn in Section 4.
2 Raw Materials and Preparation Method of Foamed Concrete
2.1 Raw Materials
The most important raw material for preparing geopolymer-based foamed concrete is geopolymer. Its basic material is usually aluminosilicate in industrial waste residue, roasted clay or natural minerals produced after smelting, or a mixture of the above substances. Here we mixed Kaolin (Inner Mongolia Hongji Co., Ltd.), slag (Shenyang Huacheng Slag Powder Manufacturing Co., Ltd.) and fly ash (Tongliao General Power Plant Co., Ltd.) in a certain proportion to form the basic material of geopolymer. The main components of slag are calcium oxide and glass body. As a by-product of coal combustion, fly ash is usually classified as siliceous (class F) or cementitious (class C). In the experiment, class C fly ash (Type I) conforming to the ASTM C 618 standard was used as the auxiliary cementing material. The chemical composition of slag and fly ash are listed in Table 1. The alkali activator was made by water glass (Shandong Yousuo Chemical Technology Co., Ltd.) and sodium hydroxide (Shenyang Chemical Reagent Factory). In order to enhance the compressive strength of the geopolymer, in addition to the above-mentioned raw materials, a small amount of H46 hollow glass beads jointly developed by Ma’anshan Institute of Mining Research and Chinese Academy of Sciences were added. The physical foaming method is used to realize the preparation of geopolymer-based foamed concrete. The current physical foaming agents mainly include rosin, synthetic, protein, and composite. Among them, the composite has the highest foaming efficiency. The high-performance composite foaming agent produced by Qingdao Xinyu Machinery Technology Co., Ltd., was used in the experiments. The foaming agent used is a nearly colorless and transparent liquid, and the dilution ratio, pH value, bleeding and foam density are 50, 7.1, <20 ml and 50 kg/m3, respectively. For extending and stabilizing the foam to maintain its long-term performance, hydroxypropyl methyl cellulose produced by a Beijing company was introduced as a foam stabilizer.
2.2 Preparation Method
The preparing process of geopolymer-based foamed concrete was shown in Figure 1. First, sodium hydroxide, water glass and water were mixed to form an alkali activator of the required modulus; subsequently, dry materials such as Kaolin, slag and fly ash are mixed in a certain proportion and poured into a pot and stir evenly (about 3 min); then, the prepared alkali activator was poured into the uniformly mixed powder and continue stirring to form a geopolymer slurry (about 1 min); finally, the prefabricated foam containing foam stabilizer was passed into a mixer filled with geopolymer slurry and quickly stirred for about 45 s to form a paste-like foamed concrete. In order to prevent the rapid loss of moisture and cause cracks, we poured the above paste into a mold and covered it with a film, and cured it in a standard curing chamber for 24 h under constant temperature and humidity (20 ± 2°C, RH 90%). After demolding, the environmental parameters were kept unchanged until the foamed concrete specimen reached the predetermined age of 28 days.
2.3 Evaluating the Properties of Foamed Concrete
The demoulded foam concrete blocks were divided to obtain 9 small blocks in 3 groups with a length, width and height of 10 cm. The measurement of the compressive strength and dry density of the foamed concrete in this test follows the relevant regulations in the industry standards of the Chinese construction industry (JG/T 266-2011). Before carrying out the experiments, first put the test block in a drying box and dry it to a constant weight. Next, measure the length, width and height twice and then take the average to calculate the volume and surface area. Finally, the average values of each group were calculated using the volumes, surface areas and masses of the 3 test blocks. The IMDRY3001 thermal conductivity tester was used to measure the thermal conductivity. In order to ensure accurate and reliable measurement results, the upper and lower surfaces of the selected test block are required to be as parallel, smooth and uniform in thickness as possible. The compression strength test uses NYL-2000D universal press, and its pressurizing speed can be set to 0.5∼1.5KN/s. During the pressurization process, the load is continuously and uniformly increased until the test block is damaged. At this time, the pressure reading of the universal press is recorded as the maximum failure load. The compressive strength f is expressed as
where F is the maximum failure load and A is the area of Surface. The calculation of dry density follows the following formula:
In Eq. 2, ρ0 is dry density, m0 is quality after drying, and V is the volume. Compared with the simple compressive strength index, the specific strength S obtained after the introduction of dry density is more objective for foam concrete performance evaluation:
3 Results and Discussion
3.1 Influencing Factors of Geopolymer-Based Foam Concrete
The performance of geopolymer-based foam concrete is affected by foam content, water-binder ratio, water glass content and water glass modulus. The foam content is defined as ratio of the mass of foaming agent and gelling material required to prepare the foam. The water-binder ratio refers to the ratio of the total water consumption to the mass of the cementations material in the material preparation process. The water glass content is the ratio of the pure water glass in the liquid water glass to the mass of the cementitious material. The water glass modulus is n in the molecular formula of water glass (n in Na2O·nSiO2).
3.1.1 Foam Content
For the research of influence of foam content on the performance of geopolymer-based foam concrete, the foam content was increased from 0.30 to 1.58% during the preparation process while keeping other conditions unchanged. The elementary mix proportions are summarized in Table 2. The compressive strength, dry density and specific strength of geopolymer-based foam concrete at the age of 28-day are shown in Figure 2. It can be found that the compressive strength and dry density of the geopolymer-based foamed concrete decreases continuously with the increase of the foam content. The above phenomenon is the result of the continuous decrease of the concrete density as the foam content increases. On the other hand, the continuous increase of foam content will cause the foam to burst and merge, which in turn makes the pores larger. Moreover, the curve of specific strength shows a trend of first increasing and then decreasing. This is because there is a significant change in the rate of decrease of the concrete dry density as the foam content increases. When the foam content increases to 0.62%, the specific strength reaches the maximum. As is shown in Figure 3, the thermal conductivity of foam concrete decreases with the increase of foam content, which indicates that the voids inside the concrete help to improve its thermal insulation performance.
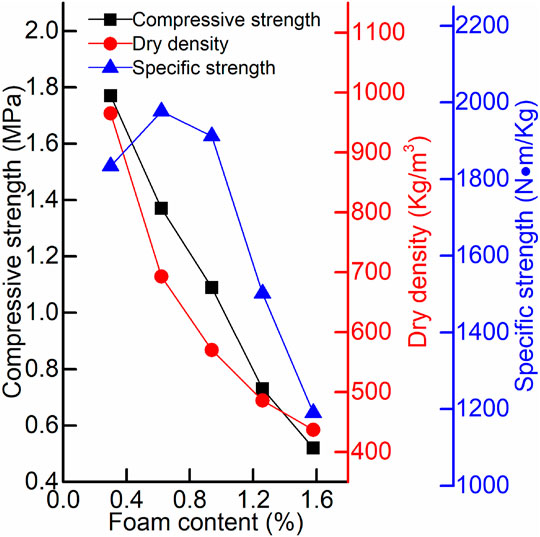
FIGURE 2. The compressive strength, dry density and specific strength of geopolymer-based foam concrete at the age of 28-day (foam content from 0.30 to 1.58%).
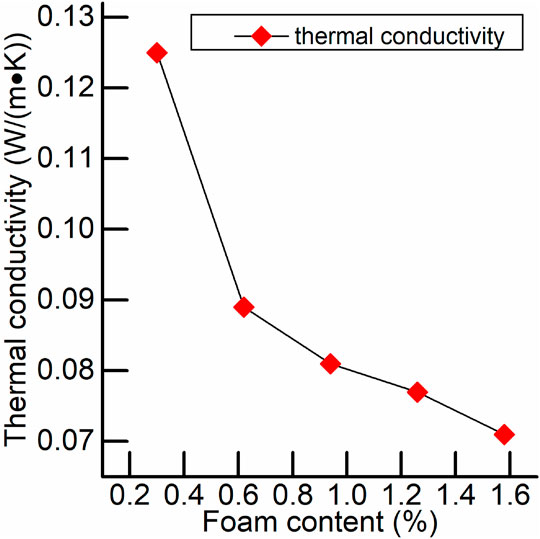
FIGURE 3. The thermal conductivity of geopolymer-based foam concrete at the age of 28-day (foam content from 0.30 to 1.58%).
3.1.2 Water-Binder Ratio
In Table 2, the foam content was fixed at 0.94%, the water-binder ratio was changed in the range of 0.35–0.55, and the preparation process was repeated. As the water-binder ratio increases, the consistency of the slurry decreases, the fluidity increases, and the stability of the concrete structure decreases. In addition, the shear resistance of the slurry to the bubbles is also continuously reduced, the bubbles continue to float up and tend to concentrate, and stress defects are prone to appear in the concentrated position. The above factors will cause the compressive strength and dry density of concrete to decrease, as shown in Figure 4. In the same way as above (Figure 2), the sudden change in the rate of decrease of the concrete dry density results in the fluctuation of the specific strength curve. When the water-binder ratio is between 0.35 and 0.45, the curve has an inflection point. The pores of foamed concrete increase with the water-binder ratio, which in turn leads to a decrease in thermal conductivity. The changing trend of the curve in Figure 5 just confirms this process.
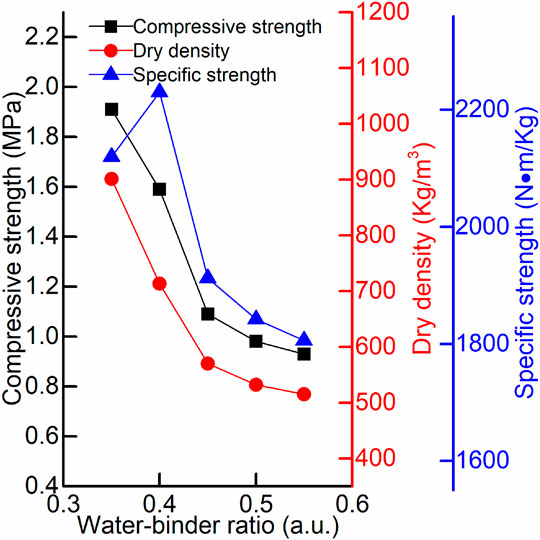
FIGURE 4. The compressive strength, dry density and specific strength of geopolymer-based foam concrete at the age of 28-day (water-binder ratio from 0.35 to 0.55).
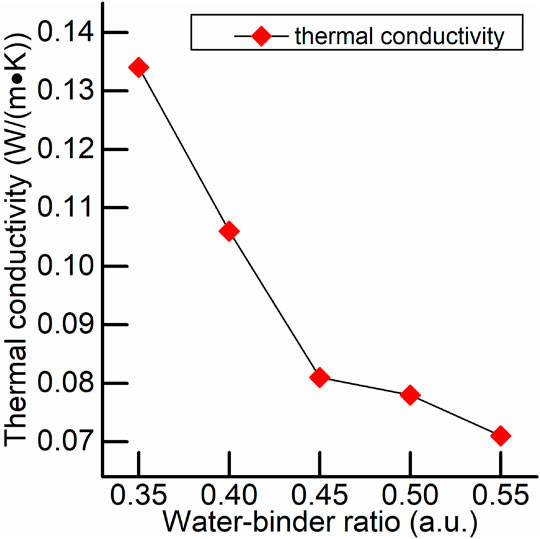
FIGURE 5. The thermal conductivity of geopolymer-based foam concrete at the age of 28-day (water-binder ratio from 0.35 to 0.55).
3.1.3 Water Glass Content
Among the materials listed in Table 2, the foam content was kept at 0.94% while the water glass content was gradually increased from 10 to 30%, and the preparation process was repeated. In Figure 6, with the increase in the amount of water glass, the compressive strength of the concrete first increases and then decreases. The main reason for this condition is the change in the degree of alkali excitation: as the content of water glass increases, the content of NaOH must be increased to keep the modulus of the water glass unchanged, resulting in stronger alkali excitation and compressive strength. However, when the content of water glass reaches a certain level, it will directly react with the geopolymer and weaken the excitation effect, thereby reducing the compressive strength. The increase of the water glass content improves the stability of the unhardened concrete paste, that is, increases the shear resistance. As can be seen in Figure 6, the larger shear resistance causes a large amount of foam to break, reducing the voids of the concrete, and thereby increasing the dry density of the concrete. While the water glass content is increasing, the specific strength of the geopolymer-based foam concrete first increases and then decreases. When the water glass content is 20%, the specific strength reaches the maximum. The increase in the amount of water glass will reduce the voids of the foamed concrete, thereby accelerating the process of heat conduction, as described in the experimental results given in Figure 7.
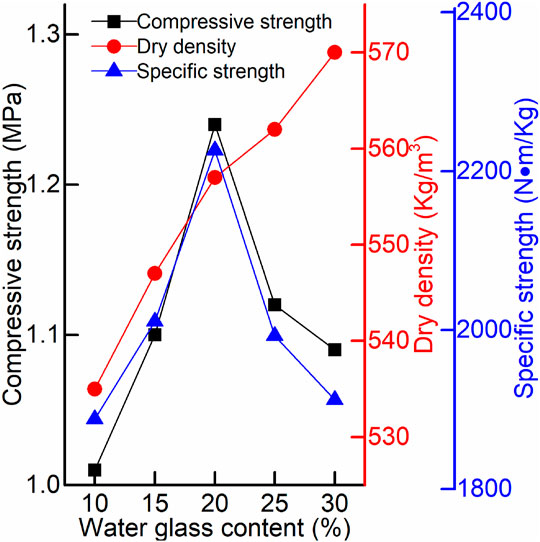
FIGURE 6. The compressive strength, dry density and specific strength of geopolymer-based foam concrete at the age of 28-day (water glass content from 10 to 30%).
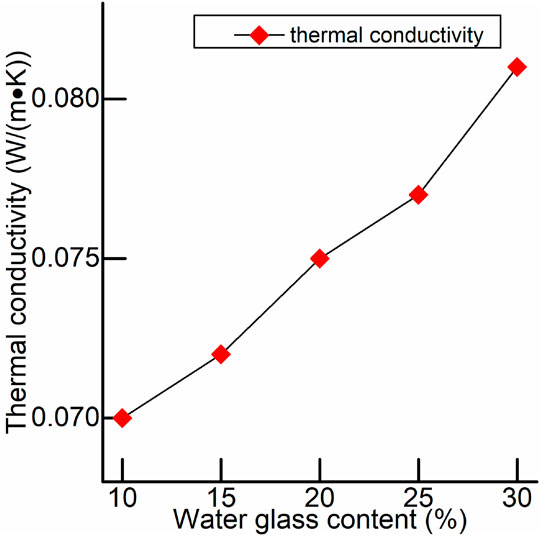
FIGURE 7. The thermal conductivity of geopolymer-based foam concrete at the age of 28-day (water glass content from 10 to 30%).
3.1.4 Water Glass Modulus
The foam content listed in Table 2 was kept at 0.94%, while the water glass modulus was gradually increased from 1.0 to 1.4. The compressive strength, dry density and specific strength of geopolymer-based foam concrete at the age of 28-day are shown in Figure 8. The increase in the modulus of the water glass causes a corresponding increase in the Si component. The appropriate increase of the Si component is conducive to the formation of C-S-H gel, thereby increasing the compressive strength of concrete and reducing its dry density. However, when the modulus of the water glass is too large, the alkaline solution will not be able to fully stimulate the activity of the slag because the pH value is not high enough. At this time, the changes in compressive strength and dry density are opposite to the above, and then the maximum value of the specific strength curve appears. In Figure 9, the fluctuation of the thermal conductivity curve indicates that a moderate increase in the modulus of the water glass produces more pores, but when it reaches a certain level, the effect of increasing the pores is no longer obvious. Instead, the increase in dry density caused by the introduction of Si components dominates.
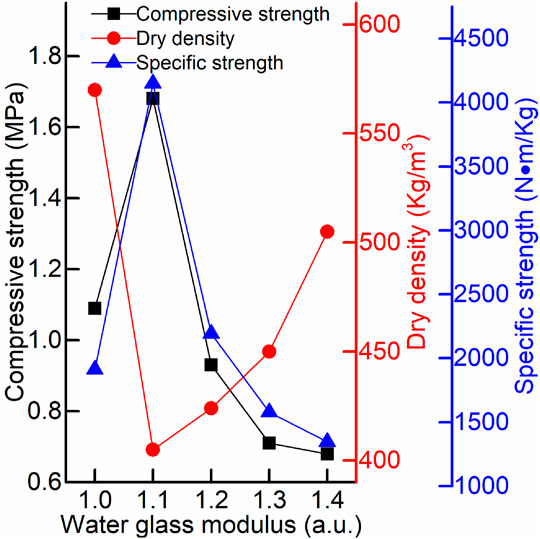
FIGURE 8. The compressive strength, dry density and specific strength of geopolymer-based foam concrete at the age of 28-day (water glass modulus from 1.0 to 1.4).
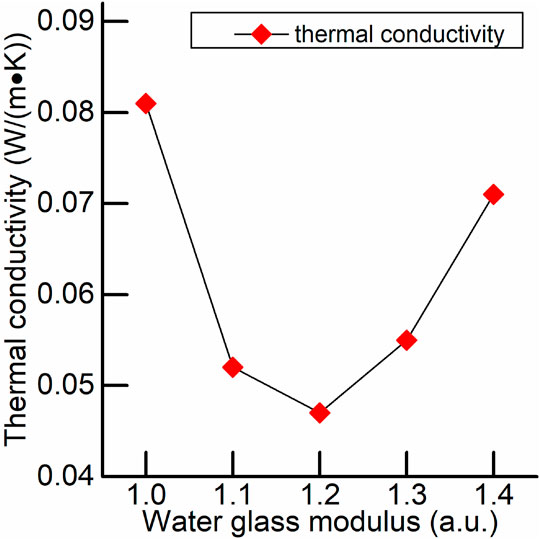
FIGURE 9. The thermal conductivity of geopolymer-based foam concrete at the age of 28-day (water glass modulus from 1.0 to 1.4).
3.2 Orthogonal Experiment and Range Analysis
3.2.1 Orthogonal Experiment
Orthogonal experiments can not only reduce the number of repeated experiments, but also help to understand the overall experimental situation through the analysis of some representative points. For this research, the foam content, water-binder ratio, water glass content and water glass modulus are considered as independent factors. Within the optimal range of these factors, three typical values are taken for each to design the L9(34) orthogonal scheme shown in Table 3, where 9 represents the orthogonal combinations of factors, 3 represents the levels of each factor, and 4 represents the number of factors. As shown in Table 3, the orthogonal experiment table must satisfy orthogonality, representativeness and comprehensive comparability. Orthogonality requires that the number of occurrences of each level in the same column is equal. Representativeness means that the experimental points in the orthogonal experiment table are evenly distributed among all possible experimental points. The comprehensive comparability ensures that the interference of other factors is eliminated to the greatest extent in the effect of each level of each factor, so that the influence of different levels of the factor on the experimental indicators can be comprehensively compared.
3.2.2 Range Analysis
In order to meet the main requirements of light weight, sturdiness and heat preservation of building enclosure materials, the orthogonal experiments were carried out with specific strength and thermal conductivity as the indicators. Range analysis is an intuitive data processing method for orthogonal experiments. Through this method, the degree of influence of each factor on a certain indicator can be evaluated, and the optimal combination of each factor for the indicator can be obtained. In Tables 4, 5 used for data analysis, Ki represents the sum of experimental results corresponding to the ith level of a certain factor, and ki in the bracket is the mean value of Ki. When ki reaches the maximum value, i is the optimal level of this factor. Once the optimal levels of each factor are determined, the optimal combination can be obtained directly. The difference between the maximum and minimum values of ki is the range R:
The larger the R value, the greater the influence of the change of this factor on the experimental results. According to this, the order of the factors affecting the certain indicator can be determined. From the results listed in Table 4, it can be seen that the water glass modulus has the greatest influence on the specific strength, followed by the foam content, the water glass content and the water-binder ratio. When the foam content, water-binder ratio, water glass content and water glass modulus are 0.30%, 0.45, 20%, and 1.2, the maximum specific strength can be obtained. Similarly, it can be seen from Table 5 that the factors affecting the thermal conductivity are water-binder ratio, foam content, water glass modulus and water glass content in order. For severe cold regions, heat preservation is the basic requirement of building enclosure structure, so the optimal combination of parameters should correspond to the minimum value but not the maximum value of thermal conductivity. This explains why the optimal combination in Table 5 is A3B2C3D2 instead of A1B1C1D1. The thermal conductivity obtained by a separate experiment is 0.044.
4 Conclusion
The preparation and characterization methods of geopolymer-based lightweight foam concrete are reported in the paper. The influences of foam content, water-binder ratio, water glass content and water glass modulus on the performance indicators of the prepared foam concrete are studied. Based on the experimental results, the following conclusions are drawn:
1) Compared with several other parameters, the water-binder ratio has the most significant effect on the thermal conductivity.
2) With the continuous increase in the foam content or water-binder ratio, the compressive strength, dry density and thermal conductivity of geopolymer-based foam concrete at the age of 28-day have been continuously reduced.
3) When the content of water glass increases, the compressive strength of geopolymer-based foam concrete at the age of 28-day first increases and then decreases, while the dry density and thermal conductivity continue to increase.
4) Through orthogonal optimization experiment and range analysis, it can be known that the optimal levels of foam content, water-binder ratio, water glass content and water glass modulus for thermal conductivity is 1.58%, 0.45, 30%, and 1.2.
Data Availability Statement
The raw data supporting the conclusion of this article will be made available by the authors, without undue reservation.
Author Contributions
The authors contributed to the present study as follows: MC and DS: conceptualization; MC and KZ: methodology; MC, SZ, and KZ: validation; MC: formal analysis, writing—original draft preparation; DS: investigation and writing—review and editing; SZ: resources, software, and visualization. All authors have read and agreed to the published version of the manuscript.
Funding
This research was funded by Natural Science Foundation of Inner Mongolia Autonomous Region (China), Grant number 2018BS06004; National Natural Science Foundation (China), Grant number F030608; Scientific Research Project of Inner Mongolia University for the Nationalities (China), Grant number NMDYB19065.
Conflict of Interest
The authors declare that the research was conducted in the absence of any commercial or financial relationships that could be construed as a potential conflict of interest.
Publisher’s Note
All claims expressed in this article are solely those of the authors and do not necessarily represent those of their affiliated organizations, or those of the publisher, the editors and the reviewers. Any product that may be evaluated in this article, or claim that may be made by its manufacturer, is not guaranteed or endorsed by the publisher.
Acknowledgments
We would also like to thank Mrs. Lynn for language editing.
References
Amran, Y. H. M., Farzadnia, N., and Abang Ali, A. A. (2015). Properties and Applications of Foamed concrete; a Review. Construction Building Mater. 101 (P1), 990–1005. doi:10.1016/j.conbuildmat.2015.10.112
Chang, R.-d., Soebarto, V., Zhao, Z.-y., and Zillante, G. (2016). Facilitating the Transition to Sustainable Construction: China's Policies. J. Clean. Prod. 131, 534–544. doi:10.1016/j.jclepro.2016.04.147
Fan, J.-L., Wang, J.-X., Hu, J.-W., Wang, Y., and Zhang, X. (2019). Optimization of China's Provincial Renewable Energy Installation Plan for the 13th Five-Year Plan Based on Renewable Portfolio Standards. Appl. Energ. 254, 113757. doi:10.1016/j.apenergy.2019.113757
Hardjito, D., Wallah, S. E., Sumajouw, D. M. J., and Rangan, B. V. (2004). Factors Influencing the Compressive Strength of Fly Ash-Based Geopolymer concrete. Civil Eng. Dimension. 6 (2), 88–93. doi:10.9744/ced.6.2
He, M. (2017). Polyurethane Foam Technology in Green Building Eco-Efficiency Design (In Chinese). Bull. Sci. Technol. 33 (1), 174–177. doi:10.13774/j.cnki.kjtb.2017.01.037
Hill, D. (2013). Ancient Roman Concrete Could Hold Secrets to Improving Modern Construction. Civ. Eng. Mag. Arch. 83 (9), 44–45. doi:10.1061/ciegag.0000655
Hou, M., Wang, Y., Zhao, H., Zhang, Q., Xie, Q., Zhang, X., et al. (2018). Halogenated Flame Retardants in Building and Decoration Materials in China: Implications for Human Exposure via Inhalation and Dust Ingestion. Chemosphere 203, 291–299. doi:10.1016/j.chemosphere.2018.03.182
Huang, Y. (2013). Development of Foreign Building Saving-Energy Technology. Adv. Mater. Res. 671–674 (2), 2126–2128. doi:10.4028/www.scientific.net/AMR.671-674.2126
Liu, M. Y. J., Alengaram, U. J., Santhanam, M., Jumaat, M. Z., and Mo, K. H. (2016). Microstructural Investigations of palm Oil Fuel Ash and Fly Ash Based Binders in Lightweight Aggregate Foamed Geopolymer concrete. Construction Building Mater. 120, 112–122. doi:10.1016/j.conbuildmat.2016.05.076
Liu, Y., Yan, H., and Lam, J. C. (2014). Thermal comfort and Building Energy Consumption Implications – A Review. Appl. Energ. 115 (4), 164–173. doi:10.1016/j.apenergy.2013.10.062
Mydin, M. A. O., and Wang, Y. C. (2011). Structural Performance of Lightweight Steel-Foamed concrete-steel Composite walling System under Compression. Thin-Walled Structures 49 (1), 66–76. doi:10.1016/j.tws.2010.08.007
Ramamurthy, K., Kunhanandan Nambiar, E. K., and Indu Siva Ranjani, G. (2009). A Classification of Studies on Properties of Foam concrete. Cement and Concrete Composites 31 (6), 388–396. doi:10.1016/j.cemconcomp.2009.04.006
Silvestre, J., Pargana, N., de Brito, J., Pinheiro, M., and Durão, V. (2016). Insulation Cork Boards-Environmental Life Cycle Assessment of an Organic Construction Material. Materials 9 (5), 394. doi:10.3390/ma9050394
Tang, S., Xu, Y., Zhu, G., and Fang, W. (2011). Orthogonal experiment Optimum Design in the Power of the Rotor on Straight-Bladed Vawt. Energ. Proced. 12, 856–861. doi:10.1016/j.egypro.2011.10.113
Valore, R. C. (1954). Cellular Concretes Part 1 Composition and Methods of Preparation. ACI J. Proc. 50 (5), 773–796. doi:10.14359/11794
Vuong, X. T., Prietzel, J., and Heitkamp, F. (2016). Measurement of Organic and Inorganic Carbon in Dolomite-Containing Samples. Soil Use Manage 32 (1), 53–59. doi:10.1111/sum.12233
Wang, Z., Gao, H., Liu, H., Liao, L., Mei, L., Lv, G., et al. (2020). Inorganic thermal Insulation Material Prepared from Pitchstone. J. Building Eng. 32 (2), 101745. doi:10.1016/j.jobe.2020.101745
Xiao, J., and Ding, T. (2013). Research on Recycled concrete and its Utilization in Building Structures in china. Front. Struct. Civ. Eng. 7 (3), 215–226. doi:10.1007/s11709-013-0212-z
Xuan, W., and Leung, D. (2011). Optimization of Biodiesel Production from Camelina Oil Using Orthogonal experiment. Appl. Energ. 88 (11), 3615–3624. doi:10.1016/j.apenergy.2011.04.041
Yang, H., Jiang, Y., Liu, H., Xie, D., Wan, C., Pan, H., et al. (2018). Mechanical, thermal and Fire Performance of an Inorganic-Organic Insulation Material Composed of Hollow Glass Microspheres and Phenolic Resin. J. Colloid Interf. Sci. 530, 163–170. doi:10.1016/j.jcis.2018.06.075
Keywords: geopolymer, foamed concrete, orthogonal experiment, thermal conductivity, range analysis
Citation: Cong M, Zhang S, Sun D and Zhou K (2021) Optimization of Preparation of Foamed Concrete Based on Orthogonal Experiment and Range Analysis. Front. Mater. 8:778173. doi: 10.3389/fmats.2021.778173
Received: 16 September 2021; Accepted: 21 October 2021;
Published: 08 November 2021.
Edited by:
Lin Xu, Jilin University, ChinaReviewed by:
Zhiling Hou, Beijing University of Chemical Technology, ChinaJiaoqing Pan, Institute of Semiconductors (CAS), China
Copyright © 2021 Cong, Zhang, Sun and Zhou. This is an open-access article distributed under the terms of the Creative Commons Attribution License (CC BY). The use, distribution or reproduction in other forums is permitted, provided the original author(s) and the copyright owner(s) are credited and that the original publication in this journal is cited, in accordance with accepted academic practice. No use, distribution or reproduction is permitted which does not comply with these terms.
*Correspondence: Menglong Cong, congml@163.com