- 1School of Electronic and Information Engineering, Changshu Institute of Technology, Changshu, China
- 2Suzhou Key Laboratory of Advanced Lighting and Display Technologies, Changshu Institute of Technology, Changshu, China
- 3School of Physical Science and Technology, Soochow University, Suzhou, China
Gallium sulfide (GaS) is a layered metal monochalcogenide semiconductor that has recently garnered considerable attention in various fields. In this study, we investigated the nonlinear absorption characteristics of multilayer β-GaS thin films on sapphire substrate by using femtosecond open-aperture Z-scan method. The β-GaS films exhibit saturable absorption behavior at 532 nm while nonlinear absorption appears under 650 nm excitation. The nonlinear absorption coefficient of β-GaS was determined to be −1.8 × 10–8 m/W and 4.9 × 10–8 m/W at 532 and 650 nm, respectively. The carrier dynamics of β-GaS films was studied via femtosecond transient absorption (TA) measurements. The TA results demonstrated that β-GaS films have broad photo-induced absorption in the visible regime and sub-nanosecond lifetime. Our results indicate that gallium sulfide has large nonlinear optical response and long carrier lifetime, which could be applied in future photonic devices.
Introduction
Two-dimensional (2D) semiconductors, such as transition metal dichalcogenides (TMDC) and black phosphorene (BP), have attracted significant research attention in the last decade due to their intriguing physical properties and prospects for technological applications in optoelectronics, sensors, nanoelectronics, etc (Mak and Shan, 2016; Akinwande et al., 2019; Liu et al., 2020). The electrical, optical and thermal properties of various 2D materials such as MoS2, WSe2, h-BN, Bi2Te3 and SnSe have been widely studied (Briggs et al., 2019; Tan et al., 2020). Pervious results demonstrate that the bandgap of 2D semiconductors is thickness- and size-dependent. And the weak van der Waals forces between adjacent layers in 2D semiconductors enable the formation of layered van der Waals heterojunctions, which is essential for transistor and detector applications (Liu et al., 2016). However, the stability upon exposure to air impedes the applications of 2D semiconductors in practical devices. Therefore, investigation of novel 2D materials with superior properties and stability is critical for future development of 2D semiconductor optoelectronic and photonic devices.
Group-III metal monochalcogenide MX (M = Ga and Ge, X is a chalcogen such as S and Se) is also a layered semiconductor with atomic-thick layers of metal and chalcogen atoms, which has received research interest in recent years (Cai et al., 2019; Yang and Hao, 2019). Compared to TMDC and BP, the photo-physical properties of metal monochalcogenides are still elusive. Gallium sulfide (GaS) is a member of the group-III monochalcogenide semiconductors. Pervious results show that bulk GaS has an indirect bandgap of 2.5 eV and direct bandgap of 3.0 eV (Ho and Lin, 2006; Jastrzebski et al., 2019). Recently, a number of research groups have investigated the electronic and optical response of GaS in both bulk and 2D forms. Their results show that GaS has fascinating properties that could be used in transistors, LEDs, photodetectors, catalysts, etc (Late et al., 2012; Hu et al., 2013; Harvey et al., 2015; Jung et al., 2015; Lu et al., 2020; Tverjanovich et al., 2020; Zappia et al., 2021). Despite these efforts, however, few reports are available in literature about the nonlinear optical (NLO) properties of GaS (Allakhverdiev et al., 1997; Kato and Umemura, 2011; Isik et al., 2013; Karatay, 2019; Deckoff-Jones et al., 2021; Xu et al., 2021). And pervious results on NLO response of GaS were carried out with picosecond (ps), nanosecond (ns) and continuous wave (CW) laser (Isik et al., 2013; Karatay, 2019; Xu et al., 2021). The ultrafast NLO properties of GaS films are scarcely explored. On the other hand, a deeper understanding of the carrier dynamics in semiconductor material is extremely useful for the development of optoelectronic and photonic devices (Zhu and Cheng, 2020; Alfurayj et al., 2021). However, the ultrafast carrier dynamics of GaS is rarely addressed.
Herein, we report the nonlinear absorption response and carrier dynamics of a multilayer β-GaS single-crystalline thin film under femtosecond laser excitation. We demonstrate, through femtosecond open-aperture Z-scan measurement, that the nonlinear absorption coefficient of GaS films is −1.8 × 10–8 m/W and 4.9 × 10–8 m/W under 532 and 650 nm excitation, respectively. And the femtosecond TA data reveal that the carrier relaxation processes in GaS films have two exponential components. The large NLO coefficient and long carrier lifetime of the β-GaS thin films indicate that GaS is a promising candidate for future NLO devices.
Materials and Methods
Sample
The multilayer β-GaS thin films with a thickness of 800 nm were deposited on a 500 μm thick sapphire substrate by atomic layer deposition (ALD) technique (Nanjing MKNANO Technology Co., Ltd). The size of β-GaS film and the sapphire substrate was 5 × 5 and 10 × 10 mm, respectively. The deposited β-GaS films have a hexagonal crystal structure. And the XRD and Raman characterization of β-GaS films can be found elsewhere (Mukenano, 2021).
DFT Calculation
In our theoretical calculation, we used the experimental hexagonal lattice constant of a = 3.626 Ȧ and c = 17.425 Ȧ. The electronic band structure was based on the DFT with the generalized gradient approximation (GGA) (Perdew et al., 1996), The accurate projector-augmented wave (PAW) method (Blöchl, 1994), as implemented in the Vienna abinitio simulation package (VASP) (Kresse and Furthmüller, 1996), was used. The valence configurations of Ga and S atoms adopted in the present calculations were 3d104s24p1 and 3s23p4, respectively. A large plane wave cutoff of 550 eV and the small total energy convergence criterion of 10–5 eV were used throughout. Fine Monkhorst-Pack k-meshes of 24 × 24 × 8 were used for the Brillouin zone integrations.
Nonlinear Optical Measurements
The ultrafast nonlinear absorption properties of the multilayer β-GaS thin films were measured by open-aperture Z-scan technique. The Z-scan system in this study is similar to the pervious report (Shen et al., 2020). In brief, the laser was emitted from an optical parametric amplifier (Light Conversion ORPHEUS) pumped with a Yb:KGW femtosecond fiber laser (Light Conversion PHAROS-SP). The pulse duration and laser repetition rate of the OPA were 190 fs and 20 Hz, respectively. The laser wavelength used in Z-scan measurement were tuned to 532 and 650 nm. The sample placed on the mobile stage was moved along the z-axis with respect to the focal point of a 200 mm focal lens. The laser beams were measured by two energy detectors (Laser probe, Rjp-765) connected to an energy meter (Laser probe, Rj-7620). The Z-scan system was calibrated using a 2 mm thick ZnSe semiconductor.
Femtosecond TA Measurement
The ultrafast carrier dynamics in the multilayer β-GaS thin films were measured by femtosecond transient absorption (TA) spectroscopy. The TA spectroscopy was performed in the two-beam transmission geometry. The laser source was the same in the Z-scan measurements. We used pump wavelengths of 355 nm with photon energy of 3.49 eV, which is larger than the bandgap of β-GaS (∼2.5 eV). The pump fluence was kept below 50 μJ/cm2 to avoid high-order carrier relaxation processes and thermal damage of the GaS thin films. The probe beam was a white light supercontinuum generated using a sapphire crystal. The pump and probe beam illuminated the films on the front side. The time resolution of the TA measurement system was ∼280 fs. Details of our TA measurement can be found in the previous report (Li et al., 2021).
Results and Discussion
Atomic Structure
Figure 1A shows the atomic structure of bulk β-GaS. It is shown that the β-GaS has a hexagonal lattice, and the stacking type of β-GaS comprises with monolayer consisting of Ga and S in the stacking sequence of S-Ga-Ga-S along the c axis. Therefore, the layered atomic structure of β-GaS is clearly shown. Because of the comparatively large thickness of our β-GaS film (∼800 nm), the quantum confinement effect is negligible in the DFT calculation. Therefore, the DFT result of bulk GaS is also applicable to the β-GaS film in this study. The calculated scalar-relativistic band structure of β-GaS is shown in Figure 1B. The DFT results demonstrate that the β-GaS is an indirect bandgap semiconductor, which agrees well with pervious experimental report (Ho and Lin, 2006).
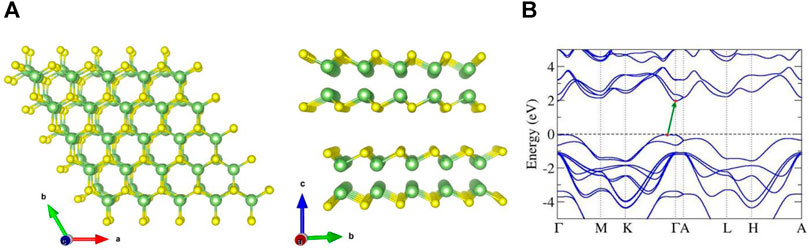
FIGURE 1. (A) The atomic structural models for GaS thin films. Green and yellow dots correspond to Ga and S atom, respectively. (For interpretation of the references to color in this figure legend, the reader is referred to the Web version of this article). (B) Band structure of bulk β-GaS calculated by DFT simulation. The green arrow indicates the indirect band gap.
Open Aperture Z-Scan
The open aperture Z-scan curves of the multilayer β-GaS thin films measured under 532 and 650 nm excitation were shown in Figures 2A,B, respectively. The pulse energy used in Z-scan measurements was 3 and 12 nJ for 532 and 650 nm, and corresponding laser intensity at beam focus was 1.45 GW/cm2 and 3.43 GW/cm2 for 532 and 650 nm, respectively. The sapphire substrate was also measured under the same experimental condition and found to has negligible nonlinear response. The Z-scan curves in Figure 2 show that multilayer β-GaS thin films have saturable absorption (SA) behavior at 532 nm, while reverse saturable absorption (RSA) appears under 650 nm excitation. Due to the short pulse duration (190 fs) and low repetition rate of our laser system, the thermal nonlinearity can be negligible in our measurement. The photon energy of light at 532 and 650 nm is 2.33 and 1.91 eV, respectively. Therefore, the β-GaS film (Eg ∼ 2.5 eV) was unable to be excited with one photon with energy of 1.91 eV (650 nm). Hence, the RSA behavior at 650 nm can be attributed to the two-photon absorption mechanism. On the other hand, laser excitation at 532 nm was near-resonant with the bandgap of β-GaS. As a result, the SA behavior at 532 nm could be attributed to the state bleaching of band edge state or shallow defect state in β-GaS. (Christodoulides et al., 2010). The Z-scan curves were fitted using the standard Z-scan theory (Sheik-Bahae et al., 1990), and the effective nonlinear absorption coefficients were determined to be −1.8 × 10–8 m/W and 4.9 × 10–8 m/W for 532 and 650 nm, respectively. Pervious results demonstrate that the film thickness has a significant influence on the NLO response of 2D material (Zhang et al., 2018; Verrone et al., 2020). However, the experimental data of β-GaS thin film with different thickness was not available due to the limitation of film deposition system and the low laser damage threshold of few-layer thin film.
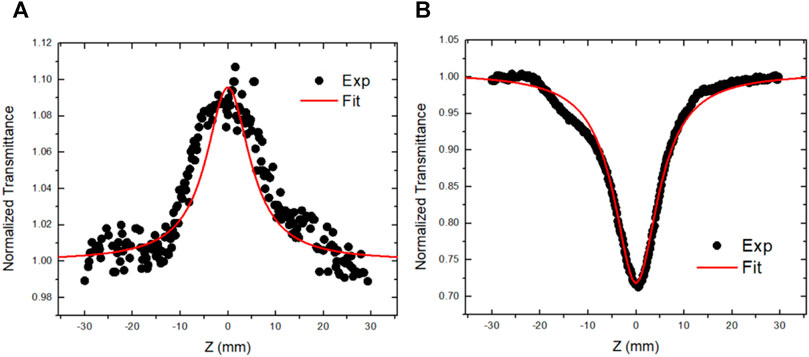
FIGURE 2. Femtosecond open aperture Z-scan curves of the multilayer β-GaS thin films under (A) 532 nm and (B) 650 nm excitations, respectively. The dots are the experimental data and the solid lines are the theoretical fitting.
The NLO parameters of multilayer β-GaS thin films and various metal monochalcogenide and 2D layered materials reported in recent literatures (Kürüm et al., 2010; Isik et al., 2013; Dong et al., 2016; Ren et al., 2016; Karatay, 2019; Ertap, 2018; Jia et al., 2020) are summarized in Table 1. Note that it should be cautious to compare the definite value of NLO coefficients measured at different wavelength and different pulse duration. However, the data in Table 1 clearly demonstrates that the multilayer β-GaS thin films have excellent nonlinear absorption response. A large β on the order of 10–8 m/W is observed, which is comparable to the reported values of PdSe2 and WS2 and higher than that of electrochemical graphene oxide (GO) (Dong et al., 2016; Ren et al., 2016; Jia et al., 2020), highlighting the strong NLO effect in the β-GaS film. The large NLO coefficient of β-GaS film could be ascribed to (a) homogeneously deposited film with improved crystalline compared to the amorphous or polycrystalline forms (Kumar et al., 2016), and (b) the ratio of photon energy to bandgap at 650 nm (532 nm) is 0.76 (0.93), which is close to the maximum value of the dispersion curve of β based on two parabolic-band model in semiconductors (Christodoulides et al., 2010). According to the Z-scan data, the imaginary part of the third-order nonlinear susceptibility can be deduced through the equation
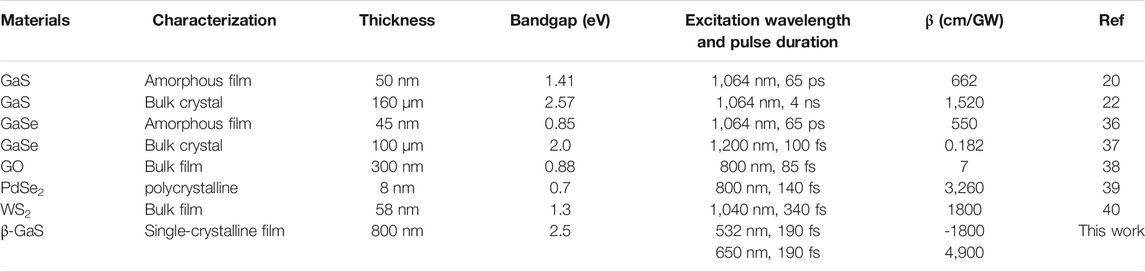
TABLE 1. Summary of the nonlinear absorption coefficients obtained in this study and reported in recent literatures.
Femtosecond TA Results
To understand the origin of strong light absorption, ultrafast carrier dynamics in β-GaS thin films were studied with femtosecond TA spectroscopy. Figure 3 a and b shows the two-dimensional (2D) contour plot of TA and one-dimensional (1D) slice of TA spectra for the β-GaS thin films under 355 nm excitation, respectively. All TA spectra show periodical interference fringes, which are the characteristics of a highly homogeneous film thickness. Similar results were reported from the measurements of the TA spectra in GaN thin films (Fang et al., 2016). The TA spectra show strong photo-induced absorption (PIA) band around 650 nm, while the amplitude of TA is rather weak below 520. These results agree well with the Z-scan measurements, while could be attributed to the state bleaching around bandgap of β-GaS (∼2.5 eV). Moreover, a careful inspection of TA spectra in Figure 3B reveals that the TA signals remains almost the same at 1 and 10 ps, then the TA signals decay within hundreds of picoseconds. These results indicate that the β-GaS thin films have long carrier lifetime.
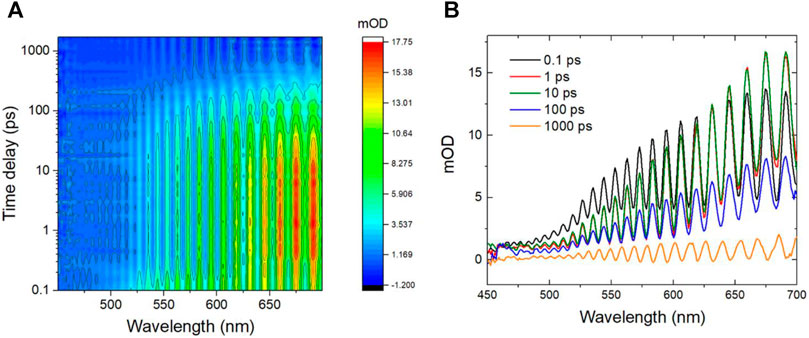
FIGURE 3. (A) 2-D contour plot of transient absorption as a function of probe wavelength and delay time for the multilayer β-GaS thin films under 355 nm pump. (B) 1-D slice from the contour plot of transient absorption of GaS films at various delay times.
To elucidate the carrier relaxation dynamics in multilayer β-GaS thin films, we have chosen the probe wavelength at 645.6 nm (near the peak of PIA band). The normalized TA decay curve at 645.6 nm of β-GaS thin films under 355 nm excitation is shown in Figure 4. It is found that the TA signal rises instantaneously and reaches the maximum at ∼0.3 ps, which is limited by our instrument response. The TA signal remains almost constant from 0 to 10 ps, followed by an exponential decay. To analyze the TA results, we used the multi-exponential decay model (Li et al., 2017):
where τi and Ai are the time constants and amplitudes of the decay components, respectively and A0 is the offset (set as zero in the fitting). The fitting curves are shown as the solid lines in Figure 4. The exponential decay analysis indicates that the decay processes in β-GaS thin films consist of two kinetic components with time constants of 85 ± 19 ps and 450 ± 50 ps, respectively. The fast and slow decay components could be attributed to the defect trapping and inter-band carrier recombination in β-GaS thin films, respectively. These results are analogous to that reported for other semiconductor materials (Fang et al., 2016; Li et al., 2017; Kong et al., 2018; Yan et al., 2020; Li et al., 2021). Our TA measurements sheds light on the carrier relaxation mechanism in GaS materials, which could be helpful to develop a clear understanding on the carrier dynamics in Group-III metal monochalcogenide semiconductors.
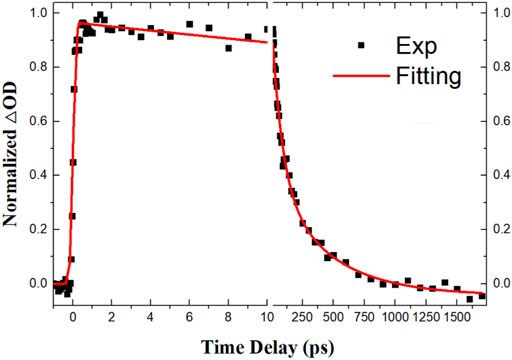
FIGURE 4. TA decay dynamics extracted at 645.6 nm for multilayer β-GaS thin films. The red lines are theoretical fitting results.
Conclusion
In summary, the nonlinear absorption response of multilayer β-GaS thin films prepared via ALD was charactered by using open-aperture Z-scan method at femtosecond time regime. The β-GaS thin films show strong saturable absorption and reverse saturable absorption under 532 and 650 nm excitation, which is attributed to the state bleaching and two-photon absorption mechanism. Femtosecond transient absorption spectroscopy reveals that the carrier relaxation dynamics in the β-GaS thin films consistent of a fast (tens of picoseconds) and slow (hundreds of picoseconds) processes, which is related to the defect trapping and recombination, respectively. The excellent nonlinear optical coefficient and long carrier lifetime indicate that the metal monochalcogenide GaS is highly promising for applications in optical modulation, optical limiting and solar energy conversion devices.
Data Availability Statement
The original contributions presented in the study are included in the article/supplementary material, further inquiries can be directed to the corresponding authors.
Author Contributions
HL, ZL, and YL contributed conception and design of the study. ZL and YC contributed the testing of samples. HL, KY, and YK performed the DFT simulation. HL wrote the first draft of the manuscript. ZL and YL wrote sections of the manuscript. All authors contributed to manuscript revision, read, and approved the submitted version.
Funding
The authors gratefully acknowledge the financial support from the National Natural Science Foundation of China (NSFC) (grant no. 62174016) and Suzhou Science and Technology Project (GrantNo.SZS2020313).
Conflict of Interest
The authors declare that the research was conducted in the absence of any commercial or financial relationships that could be construed as a potential conflict of interest.
Publisher’s Note
All claims expressed in this article are solely those of the authors and do not necessarily represent those of their affiliated organizations, or those of the publisher, the editors and the reviewers. Any product that may be evaluated in this article, or claim that may be made by its manufacturer, is not guaranteed or endorsed by the publisher.
References
Akinwande, D., Huyghebaert, C., Wang, C.-H., Serna, M. I., Goossens, S., Li, L.-J., et al. (2019). Graphene and Two-Dimensional Materials for Silicon Technology. Nature 573, 507–518. doi:10.1038/s41586-019-1573-9
Alfurayj, I. A., Li, Z., and Burda, C. (2021). Interfaces and Interfacial Carrier Dynamics in Perovskites. J. Phys. Chem. C 125,(28) 15113–15124. doi:10.1021/acs.jpcc.1c01849
Allakhverdiev, K., Ismailov, F., Kador, L., and Braun, M. (1997). Second-harmonic Generation in GaS Crystals. Solid State. Commun. 104, 1–3. doi:10.1016/s0038-1098(97)00269-x
Blöchl, P. E. (1994). Projector Augmented-Wave Method. Phys. Rev. B 50 (24), 17953–17979. doi:10.1103/physrevb.50.17953
Briggs, N., Subramanian, S., Lin, Z., Li, X., Zhang, X., Zhang, K., et al. (2019). A Roadmap for Electronic Grade 2D Materials. 2d Mater. 6 (2), 022001. doi:10.1088/2053-1583/aaf836
Cai, H., Gu, Y., Lin, Y.-C., Yu, Y., Geohegan, D. B., and Xiao, K. (2019). Synthesis and Emerging Properties of 2D Layered III-VI Metal Chalcogenides. Appl. Phys. Rev. 6, 041312. doi:10.1063/1.5123487
Christodoulides, D. N., Khoo, I. C., Salamo, G. J., Stegeman, G. I., and Van Stryland, E. W. (2010). Nonlinear Refraction and Absorption: Mechanisms and Magnitudes. Adv. Opt. Photon. 2, 60–200. doi:10.1364/aop.2.000060
Deckoff-Jones, S., Pelgrin, V., Zhang, J., Lafforgue, C., Deniel, L., Guerber, S., et al. (2021). Enhancing SiN Waveguide Optical Nonlinearity via Hybrid GaS Integration. J. Opt. 23, 025802. doi:10.1088/2040-8986/abe7d7
Dong, N., Li, Y., Zhang, S., McEvoy, N., Zhang, X., Cui, Y., et al. (2016). Dispersion of Nonlinear Refractive index in Layered WS_2 and WSe_2 Semiconductor Films Induced by Two-Photon Absorption. Opt. Lett. 41, 3936–3939. doi:10.1364/ol.41.003936
Ertap, H. (2018). Nonlinear Absorption, SHG Behavior and Carrier Dynamics of Nd and Pr Doped GaSe Single Crystals. Opt. Mater. 83, 99–103. doi:10.1016/j.optmat.2018.05.073
Fang, Y., Yang, J., Yang, Y., Wu, X., Xiao, Z., Zhou, F., et al. (2016). Ultrafast Carrier Dynamics in Ap-Type GaN Wafer under Different Carrier Distributions. J. Phys. D: Appl. Phys. 49, 045105. doi:10.1088/0022-3727/49/4/045105
Harvey, A., Backes, C., Gholamvand, Z., Hanlon, D., McAteer, D., Nerl, H. C., et al. (2015). Preparation of Gallium Sulfide Nanosheets by Liquid Exfoliation and Their Application as Hydrogen Evolution Catalysts. Chem. Mater. 27, 3483–3493. doi:10.1021/acs.chemmater.5b00910
Ho, C. H., and Lin, S. L. (2006). Optical Properties of the Interband Transitions of Layered Gallium Sulfide. J. Appl. Phys. 100, 083508. doi:10.1063/1.2358192
Hu, P., Wang, L., Yoon, M., Zhang, J., Feng, W., Wang, X., et al. (2013). Highly Responsive Ultrathin GaS Nanosheet Photodetectors on Rigid and Flexible Substrates. Nano Lett. 13, 1649–1654. doi:10.1021/nl400107k
Isik, P., Karatay, A., Yaglioglu, H., Elmali, A., Kurum, U., Ates, A., et al. (2013). The Effect of Film Thickness, Se/S Ratio and Annealing Temperature on the Nonlinear Absorption Behaviors in Amorphous GaSexS1−x Thin Films. Opt. Commun. 288, 107–113. doi:10.1016/j.optcom.2012.09.064
Jastrzebski, C., Olkowska, K., Jastrzebski, D. J., Wierzbicki, M., Gebicki, W., and Podsiadlo, S. (2019). Raman Scattering Studies on Very Thin Layers of Gallium Sulfide (GaS) as a Function of Sample Thickness and Temperature. J. Phys. Condens. Matter 31 (7), 075303. doi:10.1088/1361-648x/aaf53b
Jia, L., Wu, J., Yang, T., Jia, B., and Moss, D. J. (2020). Large Third-Order Optical Kerr Nonlinearity in Nanometer-Thick PdSe2 2D Dichalcogenide Films: Implications for Nonlinear Photonic Devices. ACS Appl. Nano Mater. 3, 6876–6883. doi:10.1021/acsanm.0c01239
Jung, C. S., Shojaei, F., Park, K., Oh, J. Y., Im, H. S., Jang, D. M., et al. (2015). Red-to-Ultraviolet Emission Tuning of Two-Dimensional Gallium Sulfide/Selenide. ACS Nano 9, 9585–9593. doi:10.1021/acsnano.5b04876
Karatay, A. (2019). Controlling of Two Photon Absorption Properties by Altering Composition Ratio of GaSxSe1−x Crystals. Opt. Laser Technology 111, 6–10. doi:10.1016/j.optlastec.2018.09.024
Kato, K., and Umemura, N. (2011). Sellmeier Equations for GaS and GaSe and Their Applications to the Nonlinear Optics in GaS_xSe_1−x. Opt. Lett. 36, 746–747. doi:10.1364/ol.36.000746
Kong, D., Jia, Y., Ren, Y., Xie, Z., Wu, K., and Lian, T. (2018). Shell-Thickness-Dependent Biexciton Lifetime in Type I and Quasi-Type II CdSe@CdS Core/Shell Quantum Dots. J. Phys. Chem. C 122, 14091–14098. doi:10.1021/acs.jpcc.8b01234
Kresse, G., and Furthmüller, J. (1996). Efficient Iterative Schemes Forab Initiototal-Energy Calculations Using a Plane-Wave Basis Set. Phys. Rev. B 54 (16), 11169–11186. doi:10.1103/physrevb.54.11169
Kumar, S., Anil Kumar, K. V., Dharmaprakash, S. M., and Das, R. (2016). Phase-Dependent Ultrafast Third-Order Optical Nonlinearities in Metallophthalocyanine Thin Films. J. Appl. Phys. 120, 123104. doi:10.1063/1.4962977
Kürüm, U., Yüksek, M., Yaglioglu, H. G., Elmali, A., Ateş, A., Karabulut, M., et al. (2010). The Effect of Thickness And/or Doping on the Nonlinear and Saturable Absorption Behaviors in Amorphous GaSe Thin Films. J. Appl. Phys. 108, 063102. doi:10.1063/1.3486047
Late, D. J., Liu, B., Luo, J., Yan, A., Matte, H. S. S. R., Grayson, M., et al. (2012). GaS and GaSe Ultrathin Layer Transistors. Adv. Mater. 24 (26), 3549–3554. doi:10.1002/adma.201201361
Li, Z.-G., Cao, H., Song, A., Liang, L., Wu, X., Yang, J., et al. (2017). Ultrafast Carrier Dynamics in Type-II ZnO-SnO Heterostructure Thin Films. Appl. Phys. Lett. 110, 172102. doi:10.1063/1.4982225
Li, Z., Wu, Z., Wang, X., Cao, H., Liang, L., Yang, J., et al. (2021). Ultrafast Carrier Dynamics of Amorphous Zinc Tin Oxide Graded Thin Films. J. Phys. Chem. C 125, 9350–9355. doi:10.1021/acs.jpcc.0c10511
Liu, C., Chen, H., Wang, S., Liu, Q., Jiang, Y.-G., Zhang, D. W., et al. (2020). Two-Dimensional Materials for Next-Generation Computing Technologies. Nat. Nanotechnol. 15, 545–557. doi:10.1038/s41565-020-0724-3
Liu, Y., Weiss, N. O., Duan, X., Cheng, H.-C., Huang, Y., and Duan, X. (2016). Van der Waals Heterostructures and devices. Nat. Rev. Mater. 1, 16042. doi:10.1038/natrevmats.2016.42
Lu, Y., Chen, J., Chen, T., Shu, Y., Chang, R. J., Sheng, Y., et al. (2020). Controlling Defects in Continuous 2D GaS Films for High‐Performance Wavelength‐Tunable UV‐Discriminating Photodetectors. Adv. Mater. 32 (7), 1906958. doi:10.1002/adma.201906958
Mak, K. F., and Shan, J. (2016). Photonics and Optoelectronics of 2D Semiconductor Transition Metal Dichalcogenides. Nat. Photon 10, 216–226. doi:10.1038/nphoton.2015.282
Mukenano (2021). Available at: http://www.mukenano.com/index.php?m=&c=Show&a=index&cid=105&id=585 (Accessed September 05, 2021).
Perdew, J. P., Burke, K., and Ernzerhof, M. (1996). Generalized Gradient Approximation Made Simple. Phys. Rev. Lett. 77, 3865–3868. doi:10.1103/physrevlett.77.3865
Ren, J., Zheng, X., Tian, Z., Li, D., Wang, P., and Jia, B. (2016). Giant Third-Order Nonlinearity from Low-Loss Electrochemical Graphene Oxide Film with a High Power Stability. Appl. Phys. Lett. 109, 221105. doi:10.1063/1.4969068
Sheik-Bahae, M., Said, A. A., Wei, T.-H., Hagan, D. J., and Van Stryland, E. W. (1990). Sensitive Measurement of Optical Nonlinearities Using a Single Beam. IEEE J. Quan. Electron. 26, 760–769. doi:10.1109/3.53394
Shen, L., Li, Z., Wu, X., Zhou, W., Yang, J., and Song, Y. (2020). Ultrafast Broadband Nonlinear Optical Properties and Excited-State Dynamics of Two Bis-Chalcone Derivatives. RSC Adv. 10, 15199–15205. doi:10.1039/d0ra01592j
Tan, T., Jiang, X., Wang, C., Yao, B., and Zhang, H. (2020). 2D Material Optoelectronics for Information Functional Device Applications: Status and Challenges. Adv. Sci. 7 (11), 2000058. doi:10.1002/advs.202000058
Tverjanovich, A., Khomenko, M., Bereznev, S., Fontanari, D., Sokolov, A., Usuki, T., et al. (2020). Glassy GaS: Transparent and Unusually Rigid Thin Films for Visible to Mid-IR Memory Applications. Phys. Chem. Chem. Phys. 22, 25560–25573. doi:10.1039/d0cp04697c
Verrone, R.-N., Moisset, C., Lemarchand, F., Campos, A., Cabié, M., Perrin-Pellegrino, C., et al. (2020). Thickness-Dependent Optical Nonlinearities of Nanometer-Thick Sb2Te3 Thin Films: Implications for Mode-Locking and Super-Resolved Direct Laser Writing. ACS Appl. Nano Mater. 3, 7963–7972. doi:10.1021/acsanm.0c01445
Xu, K., Zhang, B. Y., Hu, Y., Khan, M. W., Ou, R., Ma, Q., et al. (2021). A High-Performance Visible-Light-Driven All-Optical Switch Enabled by Ultra-thin Gallium Sulfide. J. Mater. Chem. C 9, 3115–3121. doi:10.1039/d0tc05676f
Yan, T., Han, Y., Fu, Q., Xu, T., Yin, S., Wu, W., et al. (2020). Ultrafast Carrier Relaxation in SnSe (X=1, 2) Thin Films Observed Using Femtosecond Time-Resolved Transient Absorption Spectroscopy. Opt. Mater. 108, 110440. doi:10.1016/j.optmat.2020.110440
Yang, Z., and Hao, J. (2019). Recent Progress in 2D Layered III-VI Semiconductors and Their Heterostructures for Optoelectronic Device Applications. Adv. Mater. Technol. 4 (8), 1900108. doi:10.1002/admt.201900108
Zappia, M. I., Bianca, G., Bellani, S., Curreli, N., Sofer, Z., Serri, M., et al. (2021). Two-Dimensional Gallium Sulfide Nanoflakes for UV-Selective Photoelectrochemical-Type Photodetectors. J. Phys. Chem. C 125, 11857–11866. doi:10.1021/acs.jpcc.1c03597
Zhang, J., Jiang, T., Zhou, T., Ouyang, H., Zhang, C., Xin, Z., et al. (2018). Saturated Absorption of Different Layered Bi2Se3 Films in the Resonance Zone. Photon. Res. 6, C8–C14. doi:10.1364/prj.6.0000c8
Keywords: gallium sulfide, metal monochalcogenide, Z-scan technique, transient absorption spectroscopy, layered semiconductor
Citation: Lu H, Chen Y, Yang K, Kuang Y, Li Z and Liu Y (2021) Ultrafast Nonlinear Optical Response and Carrier Dynamics in Layered Gallium Sulfide (GaS) Single-Crystalline Thin Films. Front. Mater. 8:775048. doi: 10.3389/fmats.2021.775048
Received: 14 September 2021; Accepted: 04 October 2021;
Published: 22 October 2021.
Edited by:
Ching-Hwa Ho, National Taiwan University of Science and Technology, TaiwanReviewed by:
Dongsheng Tang, Hunan Normal University, ChinaGuoen Weng, East China Normal University, China
Copyright © 2021 Lu, Chen, Yang, Kuang, Li and Liu. This is an open-access article distributed under the terms of the Creative Commons Attribution License (CC BY). The use, distribution or reproduction in other forums is permitted, provided the original author(s) and the copyright owner(s) are credited and that the original publication in this journal is cited, in accordance with accepted academic practice. No use, distribution or reproduction is permitted which does not comply with these terms.
*Correspondence: Yushen Liu, eXNsaXVAY3NsZy5lZHUuY24=; Zhongguo Li, emdsaUBjc2xnLmVkdS5jbg==