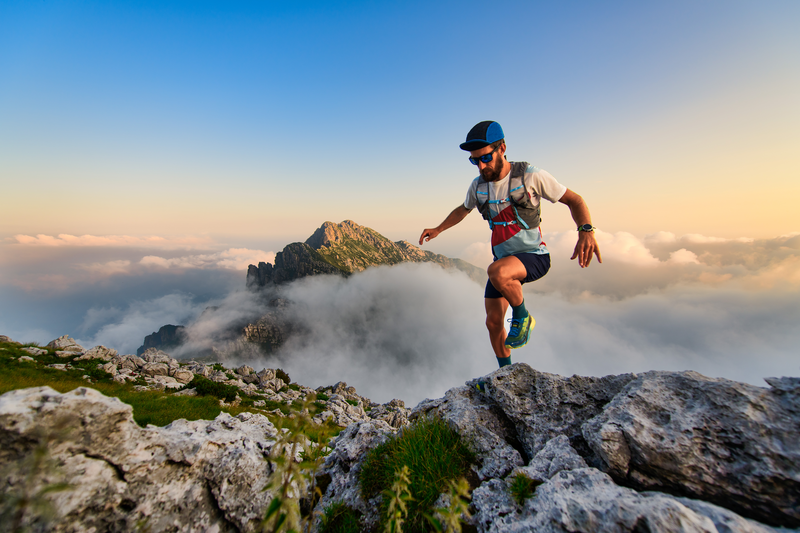
95% of researchers rate our articles as excellent or good
Learn more about the work of our research integrity team to safeguard the quality of each article we publish.
Find out more
MINI REVIEW article
Front. Mater. , 16 November 2021
Sec. Polymeric and Composite Materials
Volume 8 - 2021 | https://doi.org/10.3389/fmats.2021.773205
The ongoing COVID-19 pandemic caused by SARS-CoV-2 has significantly affected the world, creating a global health emergency. For controlling the virus spread, effective and reliable diagnostic and therapeutic measures are highly expected. Using proper biomedical materials to produce detection kits/devices and personal protective equipment (PPE), such as swabs and masks, has become the focus since they play critical roles in virus diagnostics and prevention. Electrospun polymer composites have garnered substantial interest due to their potential to provide antiviral healthcare solutions. In this review, we summarized the recent efforts in developing advanced antiviral electrospun polymer composites for virus detection and prevention. We highlighted some novel strategies for developing effective antiviral personal protective equipment (PPE), including self-sterilization, reusability, and potential antiviral drug encapsulation. Besides, we discussed the current challenges and future perspectives for improving the materials’ performance to achieve better virus detection, antiviral, prevention, and therapeutics.
The worldwide COVID-19 pandemic caused by the novel coronavirus SARS-CoV-2 has significantly impacted the world’s economy and people’s life (Chakraborty et al., 2020; The Lancet, 2020; Tu et al., 2020; Gao et al., 2021; Yüce et al., 2021). The number of confirmed cases across the world so far has reached more than 200 million, and the number of deaths has gone beyond 4.5 million1. Based on the current pandemic status analysis, it is not very likely that the pandemic will end in a short period due to the rapid mutation of the coronavirus. Therefore, developing strategies for accurate and rapid diagnostics, efficient protection, and effective therapeutics is in high demand to control the virus spread (Alsharif and Qurashi, 2021; Majumder and Minko, 2021; Rai et al., 2021). To achieve the goal, multidisciplinary research is needed. Scientists from various fields such as chemistry, materials science, and biotechnology need to work together to tackle multiple challenges (Colombani et al., 2021; Guo et al., 2021; Jarai et al., 2021). For example, biologists and biochemists are working hard to reveal the structures of viral proteins and identify the critical pathways for the infection. Materials scientists and biochemists focus on developing effective point-of-care (POC) diagnostic kits or devices for rapid and accurate diagnosis. Biologists, chemists, and clinicians are collaborating to innovate effective treatment and vaccination strategies (Dinnes et al., 2020; Smith et al., 2020; Parupudi et al., 2021). From a materials science perspective, developing efficient antiviral materials is critical in protecting against the virus, particularly during the pandemic (Tang P. et al., 2020; Zhou et al., 2020; Luzuriaga et al., 2021; Seyfoori et al., 2021; Tang et al., 2021). Up to date, some antiviral materials in the forms of nanoparticles, hydrogels, and fibers have been developed to tackle COVID-19, where most of them are based on electrospun polymer composites (Mahmood et al., 2020; Park, 2020; Rowan and Laffey, 2021).
Electrospun polymer composites (Bhardwaj and Kundu, 2010; Chowdhury et al., 2021; Pandit et al., 2021) have been widely used for making personal protective equipment (PPE) against virus infections (Haggag et al., 2019; Karagoz et al., 2021). They are composed of nano/microscale electrospun fibers with tailored compositions for specific application needs. These composites generally have a high surface-to-volume ratio, tunable porosity and mechanical properties, and designed functionalities such as hydrophobicity and bio-responsiveness.
Here in this mini-review, we briefly summarized the recent progress on antiviral electrospun polymer composites for controlling the COVID-19 pandemic, focusing on virus sampling and detection, antiviral protection, drug encapsulation and delivery, and materials sustainability. We also discussed the current challenges and future opportunities for improving the performance of materials for better viral protection, detection, and treatment.
Efficient sampling and rapid detection help control the spread of viruses. For viral detection, immunoassays and polymerase chain reaction (PCR)-based tests are widely used. These two detection techniques require different sampling strategies. Immunoassays are usually applied for the viral detection of infected patients. They require finger-prick or blood-draw for the detection of generated antibodies. In comparison, the PCR-based tests are typically less invasive, and it is used to detect viral DNA or RNA. The PCR-based tests are applied widely to detect the single-stranded RNA virus SARS-CoV-2 during the COVID-19 pandemic. Sampling techniques such as swab sampling and bronchoalveolar lavage are adopted to collect specimens from the environments and individuals2 (Liao et al., 2020; Nourmoradi et al., 2021; Rahmani et al., 2020). Electrospun polymer composites are considered good materials for developing highly efficient sampling strategies, particularly swab sampling.
Swab sampling is a standard method used to collect biological specimens, and it is the most popular diagnostic technique during the COVID-19 pandemic (Whulanza et al., 2020; Wyllie et al., 2020; Rokney et al., 2020; McCarthy et al., 2021). To ensure early and accurate diagnostic outcomes, good swab fabrication techniques are required. For example, achieving sufficient biomarkers collection from limited biological samples and later efficient recovery from the swab is one key aspect (Vermeiren et al., 2020; Pan et al., 2020; West et al., 2020). McCarthy et al. reported a new class of nanofiber swabs tipped with hierarchical 3D nanofiber objects produced by expanding electrospun membranes with a solids-of-revolution-inspired gas foaming technique (Figure 1) (Whulanza et al., 2020; McCarthy et al., 2021). In the study, they generated radially aligned gelatin-coated PCL nanofiber objects with cylindrical shapes and bonded them to plastic swab sticks. The developed nanofiber swabs significantly improved the absorption and release of proteins, cells, viruses, etc., from solutions and surfaces. The nanofiber swabs in SARS-CoV-2 detection showed reduced false negative rates at two viral concentrations. They pushed the detection limit to a ten times lower viral concentration than classical flocked and cotton swabs (Whulanza et al., 2020; McCarthy et al., 2021). Moreover, both electrospinning and lyophilization are easy to scale up. Therefore, nanofiber swabs have great potential for broader diagnostic applications.
FIGURE 1. Electrospun nanofiber swabs for detection of SARS-CoV-2 and other biological specimens. (A) The fabrication process of nanofiber swab tips; (B) The microstructures of the nanofiber swabs and their usage method [Adapted with permission from (McCarthy et al., 2021). Copyright (2021) American Chemical Society].
One key and effective strategy of preventing virus infection is physical protection. Face masks with specific cut-off sizes are mainly used as barriers to reduce human-to-human transmission. In general, there are two types of masks: face (surgical) masks and respirator masks, which are used in low- and high-risk medical settings, respectively, for providing satisfying protection (Tebyetekerwa et al., 2020). The outbreak of the novel coronavirus SARS-CoV-2 has witnessed a worldwide rapid surge in face mask needs and facilitated the rapid technological development of antiviral face masks. Besides serving as a physical barrier to reduce virus transmission, it could be much more attractive if a face mask has additional functionalities such as encapsulation of anti-virus agents, self-sterilization, and reusability.
Recently, an innovative and stimuli-responsive face mask was developed based on electrospun polycaprolactone (PCL) composite fibers integrated with antiviral polyphosphate (Müller et al., 2021). Compared to traditional face masks solely based on a passive filtration principle, the developed masks could attract aerosol droplets and kill the captured viruses. Specifically, the polyphosphate components present in the mask can transform into a gel-like coacervate upon contact with divalent cations and virus-containing protein-like mucin in aerosol droplets. Additionally, using calcium polyphosphate nanoparticles as drug carriers in the mask to incorporate tretinoin could further block the function of the envelope (E) protein of SARS-COV-2. In another study, Nikhil et al. fabricated phytochemicals-encapsulated 3-ply cotton-PLA-cotton layered biodegradable face masks for protecting individuals from bacterial and viral infections (Patil et al., 2021). In this work, a needleless electrospinning technique was used to construct a close-packed mesh structure of a nanofibrous mat, leading to effective adsorption of particulate matter, aerosol particles, and bacterial targets deep inside the filtration layer. Besides, the mat achieved enhanced air permeability (differential pressure of 35.78 Pa/cm2) and better bacterial filtration efficiency (97.9%) (Patil et al., 2021).
Conventional polypropylene (PP)-based medical masks suffer severe issues such as increased electrostatic adsorption of viral particles associated with the water vapor exhaled by wearers. Recently, a new medical mask composed of electrospun polyvinyl alcohol (PVA) as an inner layer was fabricated to replace the PP melt-blown layer in conventional medical masks. Since the PVA is rich in hydroxyl groups, the electrospun polyvinyl alcohol (PVA) formed hydrogen bonds with water molecules exhaled by the human body. The trapped water molecules thus increased the triboelectricity and enhanced charge retention performance in a high humidity environment (Wang et al., 2021).
Besides synthetic materials, biomass was also used to develop a biobased antiviral face mask to control the spread of SARS-COV-2. A recent study explored the design and fabrication of an antiviral face mask using licorice root extracts, which has antimicrobial properties due to glycyrrhetinic acid (GA) and glycyrrhizin (GL) (Chowdhury et al., 2021). With electrospinning, a nanofibrous membrane using licorice root extract was successfully fabricated. Nanofibers were about 15–30 μm in diameter with random porosity and orientation and could potentially capture and kill the virus.
Some inorganic nanomaterials have also been used to integrate antiviral properties into the electrospun polymer composites (Castro-Mayorga et al., 2017; Karagoz et al., 2021). For example, silver nanoparticles (AgNPs) have antibacterial and antiviral properties and have been widely applied in a broad range of biomedical applications (Galdiero et al., 2011; Haggag et al., 2019; Jeremiah et al., 2020; Chen and Liang, 2020). A coating technique using electrospun poly 3-hydroxybutyrate-co-3-hydroxyvalerate (PHBV)/AgNPs fiber mats have been developed to achieve antiviral surfaces. The coating showed good antiviral properties and efficacy against norovirus surrogates (Castro-Mayorga et al., 2017). In another study, researchers fabricated multifunctional electrospun poly (methyl methacrylate) (PMMA) nanofibers decorated with ZnO nanorods and Ag nanoparticles (PMMA/ZnO–Ag NFs) as protective mats (Figure 2A) (Karagoz et al., 2021). The mats showed good antibacterial and antiviral properties for killing Gram-negative and Gram-positive bacteria and inhibiting corona and influenza viruses, respectively. Moreover, the incorporated photocatalyst enhanced the degradation of organic pollutants, enabling self-cleaning protective mats. Such multifunctional materials showed great potential for use in protective clothing applications.
FIGURE 2. Examples of the electrospinning process for the fabrication of the functional mask layers. (A) The schematic of the synthesis of ZnO nanorods, preparation of the electrospinning solution of PMMA and ZnO nanorods with in situ generated AgNPs, and fabrication of the PMMA/ZnO-Ag nanofibers for further PPE usage [Adapted with permission from (Karagoz et al., 2021). Copyright (2021) American Chemical Society]. (B) The illustration of the incorporated N-doped TiO2 nanoparticles into the PVA, PEO, CNF mixture for electrospinning to engineer photocatalytic antiviral face masks [Adapted with permission from (Li Q. et al., 2021). Copyright (2021) American Chemical Society].
Electrospun drug-loaded fibers have shown great potential in drug delivery systems. They have several merits, such as the high surface area, tunable diameters and structures, good porosity, functionalization, and potential to encapsulate various active substances, making them suitable for drug loading and sustained release (Balusamy et al., 2020). Acyclovir (ACY) is one of the most effective and selective antiviral drugs used to treat herpes viruses but is only slightly soluble in water. Yu et al. have undertaken several investigations into ACY-encapsulated fibers, using both slow and fast dissolving polymers. They reported electrospun polyacrylonitrile (PAN) nanofibers loaded with ACY could be used as a nanofiber-based drug delivery system showed a sustained drug release profile over 12 h (Yu et al., 2010). They further developed a new type of solid dispersion in the form of core-sheath nanofibers using coaxial electrospinning to deliver ACY (Yu et al., 2011). Additionally, Shekh et al. chemically modified electrospun PAN nanofibers with oxidized chitosan (OC) to promote the effective transdermal delivery for ACY (Shekh et al., 2020). For treating human immunodeficiency virus (HIV) infection, combinative drug therapy can enhance efficacy while reducing toxicity and addressing the emergence of drug resistance (Greco and Vicent, 2009). Blakney et al. developed a co-delivery system for the prevention of HIV in 2014. In the study, they prepared polyvinyl alcohol (PVA) based electrospun fabrics with different microscale geometries for the co-delivery of a hydrophilic (TFV) and hydrophobic (LNG) drug with high drug loading (Blakney et al., 2014). Griffithsin (GRFT), a biological antiviral lectin, can inhibit HIV-1 infection by adhering and inactivating HIV-1 immediately upon contact. Griffithsin-modified electrospun fibers were made using poly (lactic-co-glycolic acid) (PLGA) to prevent HIV infection (Grooms et al., 2016). Recently, researchers developed a new type of electrospun fibers that incorporated the GRFT to provide dual-purpose protection against HIV-1 and HSV-2 infections. These fibers were composed of polyethylene oxide (PEO), polyvinyl alcohol (PVA), and polyvinylpyrrolidone (PVP) (Tyo et al., 2020). Furthermore, Baskakova et al. developed electrospun fibers containing acyclovir, ciprofloxacin, and cyanocobalamin to treat cytomegalovirus infections for patients with AIDS (Baskakova et al., 2016). These electrospun fiber-based drug delivery systems hold great promise in current investigations and future clinical practices. With the development of the antiviral drugs, such as for COVID-19 (Alshammari et al., 2021; Ohashi et al., 2021), this technique could be applied to the on-demand therapeutic applications, showing great prospectives in the clinic.
Personal protective equipment (PPE), such as masks and protective suits, is expected to prevent and control SARS-CoV-2 attacks both effectively and efficiently (Leung et al., 2020a; Leung et al., 2020b; Karim et al., 2020; Weiss et al., 2020; Tang Z. et al., 2020; Zangmeister et al., 2020). However, conventional PPE can only set a physical barrier for viral entry without virus-killing ability. As a result, the virus will accumulate on the surface of PPE, and the arbitrary disposal of medical PPE may cause cross-contamination (Li Q. et al., 2021). Besides, the massive use and disposal of polypropylene for mask manufacturing have caused burdens to the environment due to its weak degradability (over 10 years by soil) (Canopoli et al., 2020). Therefore, developing PPE with a combination of antiviral properties and reusability is highly desired for tackling the COVID-19 pandemic and the future underlying infection outbreak in a more sustainable way. Li et al. developed techniques to fabricate fabrics with real-time self-antiviral capabilities using a highly efficient aggregation-induced emission photosensitizer (namely, ASCP-TPA) (Li B. et al., 2021). These ASCP-TPA-attached fabrics (ATaFs) showed rapid and real-time antiviral capabilities to kill mouse coronavirus murine hepatitis virus A59 (MHV-A59) under ultralow-power lamp light irradiation (Horváth et al., 2020; Tang Z. et al., 2020). Furthermore, the ATaFs showed good washability, photostability, and reusability (>100 times with persistent use for more than 2 weeks), offering a sustainable solution to the global PPE supply shortage (Li B. et al., 2021). In addition, Li et al. reported reusable, biodegradable, and antibacterial masks made with electrospun polymer fibers composed of PVA, PEO, and CNF. The electrospun polymer composites were modified with a nitrogen-doped TiO2 and TiO2 mixture for achieving photocatalytic effect under simulated/natural sunlight for bacterial disinfection (Figure 2B) (Li Q. et al., 2021). The fabricated masks showed good mechanical performance with ten times elastic modulus and two times tensile strength higher than a commercial single-use mask.
A virus is a biological particle at the nanoscale level, making detection and prevention challenging. To ensure efficient virus capture for the detection or effective protection, it typically requires that the materials used in such practices have structural features at nanoscale similar to the size of the virus. Electrospinning offers a convenient way to fabricate nanofibers with well-controlled structures and properties. Electrospun polymer nanofibers have been used to make virus-blocking platforms, such as PPE, showing great promise in preventing the spread of virus. However, some challenges remain, and more efforts are required. For example, rapid virus detection of high accuracy and a low price is needed. To achieve this goal, tailoring surface chemistry of materials for better virus capturing and developing cost-effective manufacturing methods to reduce the detection cost should be considered. Furthermore, the delivery of antiviral drugs can be integrated in the system with rational material designs to improve the therapeutic ability of electrospun polymer composites. Besides synthetic polymers, natural polymers such as silk and chitosan can also be used for making antiviral electrospun polymer composites since these natural polymers are biocompatible and the processing is generally environmentally friendly. Lastly, in terms of sustainability, developing reusable or recyclable electrospun polymer composites for antiviral protection is of great interest with respect to the economic costs and future environmental impacts.
JL, WW, RJ wrote the manuscript and CG conceived the idea of the study, supervised, and wrote the manuscript.
This work was supported by Foundation of Westlake University and National Natural Science Foundation of China (Award No. 52103129).
The authors declare that the research was conducted in the absence of any commercial or financial relationships that could be construed as a potential conflict of interest.
All claims expressed in this article are solely those of the authors and do not necessarily represent those of their affiliated organizations, or those of the publisher, the editors and the reviewers. Any product that may be evaluated in this article, or claim that may be made by its manufacturer, is not guaranteed or endorsed by the publisher.
1https://www.who.int/publications/m/item/weekly-operational-update-on-covid-19---6-september-2021.
2https://www.cdc.gov/coronavirus/2019-ncov/lab/guidelines-clinical-specimens.html.
Alshammari, M. B., Ramadan, M., Aly, A. A., El-Sheref, E. M., Bakht, M. A., Ibrahim, M. A. A., et al. (2021). Synthesis of Potentially New Schiff Bases of N-Substituted-2-Quinolonylacetohydrazides as Anti-COVID-19 Agents. J. Mol. Struct. 1230, 129649. doi:10.1016/j.molstruc.2020.129649
Alsharif, W., and Qurashi, A. (2021). Effectiveness of COVID-19 Diagnosis and Management Tools: A Review. Radiography 27, 682–687. doi:10.1016/j.radi.2020.09.010
Balusamy, B., Celebioglu, A., Senthamizhan, A., and Uyar, T. (2020). Progress in the Design and Development of "Fast-Dissolving" Electrospun Nanofibers Based Drug Delivery Systems - A Systematic Review. J. Controlled Release 326, 482–509. doi:10.1016/j.jconrel.2020.07.038
Baskakova, A., Awwad, S., Jiménez, J. Q., Gill, H., Novikov, O., Khaw, P. T., et al. (2016). Electrospun Formulations of Acyclovir, Ciprofloxacin and Cyanocobalamin for Ocular Drug Delivery. Int. J. Pharmaceutics 502, 208–218. doi:10.1016/j.ijpharm.2016.02.015
Bhardwaj, N., and Kundu, S. C. (2010). Electrospinning: a Fascinating Fiber Fabrication Technique. Biotechnol. Adv. 28, 325–347. doi:10.1016/j.biotechadv.2010.01.004
Blakney, A., Jiang, Y., Woodrow, K., and Krogstad, E. (2014). Delivery of Multipurpose Prevention Drug Combinations from Electrospun Nanofibers Using Composite Microarchitectures. Ijn 9, 2967–2978. doi:10.2147/ijn.s61664
Canopoli, L., Coulon, F., and Wagland, S. T. (2020). Degradation of Excavated Polyethylene and Polypropylene Waste from Landfill. Sci. Total Environ. 698, 134125. doi:10.1016/j.scitotenv.2019.134125
Castro-Mayorga, J. L., Randazzo, W., Fabra, M. J., Lagaron, J. M., Aznar, R., and Sánchez, G. (2017). Antiviral Properties of Silver Nanoparticles against Norovirus Surrogates and Their Efficacy in Coated Polyhydroxyalkanoates Systems. LWT - Food Sci. Technology 79, 503–510. doi:10.1016/j.lwt.2017.01.065
Chakraborty, C., Sharma, A. R., Sharma, G., Bhattacharya, M., and Lee, S. S. (2020). SARS-CoV-2 Causing Pneumonia-Associated Respiratory Disorder (COVID-19): Diagnostic and Proposed Therapeutic Options. Eur. Rev. Med. Pharmacol. Sci. 24, 4016–4026. doi:10.26355/eurrev_202004_20871
Chen, L., and Liang, J. (2020). An Overview of Functional Nanoparticles as Novel Emerging Antiviral Therapeutic Agents. Mater. Sci. Eng. C 112, 110924. doi:10.1016/j.msec.2020.110924
Chowdhury, M. A., Shuvho, M. B. A., Shahid, M. A., Haque, A. K. M. M., Kashem, M. A., Lam, S. S., et al. (2021). Prospect of Biobased Antiviral Face Mask to Limit the Coronavirus Outbreak. Environ. Res. 192. 110294. doi:10.1016/j.envres.2020.110294
Colombani, T., Rogers, Z. J., Eggermont, L. J., and Bencherif, S. A. (2021). Harnessing Biomaterials for Therapeutic Strategies against COVID-19. Emergent Mater. 4, 1–10. doi:10.1007/s42247-021-00171-z
Dinnes, J., Deeks, J. J., Adriano, A., Berhane, S., Davenport, C., Dittrich, S., et al. (2020). Rapid, point-of-care Antigen and Molecular-Based Tests for Diagnosis of SARS-CoV-2 Infection. Cochrane Database Syst. Rev. 8. Cd013705. doi:10.1002/14651858.CD013705
Galdiero, S., Falanga, A., Vitiello, M., Cantisani, M., Marra, V., and Galdiero, M. (2011). Silver Nanoparticles as Potential Antiviral Agents. Molecules 16, 8894–8918. doi:10.3390/molecules16108894
Gao, Z., Xu, Y., Sun, C., Wang, X., Guo, Y., Qiu, S., et al. (2021). A Systematic Review of Asymptomatic Infections with COVID-19. J. Microbiol. Immunol. Infect. 54, 12–16. doi:10.1016/j.jmii.2020.05.001
Greco, F., and Vicent, M. J. (2009). Combination Therapy: Opportunities and Challenges for Polymer-Drug Conjugates as Anticancer Nanomedicines. Adv. Drug Deliv. Rev. 61, 1203–1213. doi:10.1016/j.addr.2009.05.006
Grooms, T. N., Vuong, H. R., Tyo, K. M., Malik, D. A., Sims, L. B., Whittington, C. P., et al. (2016). Griffithsin-Modified Electrospun Fibers as a Delivery Scaffold to Prevent HIV Infection. Antimicrob. Agents Chemother. 60, 6518–6531. doi:10.1128/aac.00956-16
Guo, L., Bi, W., Wang, X., Xu, W., Yan, R., Zhang, Y., et al. (2021). Engineered Trimeric ACE2 Binds Viral Spike Protein and Locks it in “Three-Up” Conformation to Potently Inhibit SARS-CoV-2 Infection. Cell Res. 31, 98–100. doi:10.1038/s41422-020-00438-w
Haggag, E., Elshamy, A., Rabeh, M., Gabr, N., Salem, M., Youssif, K., et al. (2019). Antiviral Potential of green Synthesized Silver Nanoparticles of Lampranthus Coccineus and Malephora Lutea. Ijn Vol. 14, 6217–6229. doi:10.2147/ijn.s214171
Horváth, E., Rossi, L., Mercier, C., Lehmann, C., Sienkiewicz, A., and Forró, L. (2020). Photocatalytic Nanowires‐Based Air Filter: Towards Reusable Protective Masks. Adv. Funct. Mater. 30. 2004615. doi:10.1002/adfm.202004615
Jarai, B. M., Stillman, Z., Bomb, K., Kloxin, A. M., and Fromen, C. A. (2021). Biomaterials-Based Opportunities to Engineer the Pulmonary Host Immune Response in COVID-19. ACS Biomater. Sci. Eng. 7, 1742–1764. doi:10.1021/acsbiomaterials.0c01287
Jeremiah, S. S., Miyakawa, K., Morita, T., Yamaoka, Y., and Ryo, A. (2020). Potent Antiviral Effect of Silver Nanoparticles on SARS-CoV-2. Biochem. Biophysical Res. Commun. 533, 195–200. doi:10.1016/j.bbrc.2020.09.018
Karagoz, S., Kiremitler, N. B., Sarp, G., Pekdemir, S., Salem, S., Goksu, A. G., et al. (2021). Antibacterial, Antiviral, and Self-Cleaning Mats with Sensing Capabilities Based on Electrospun Nanofibers Decorated with ZnO Nanorods and Ag Nanoparticles for Protective Clothing Applications. ACS Appl. Mater. Inter. 13, 5678–5690. doi:10.1021/acsami.0c15606
Karim, N., Afroj, S., Lloyd, K., Oaten, L. C., Andreeva, D. V., Carr, C., et al. (2020). Sustainable Personal Protective Clothing for Healthcare Applications: A Review. ACS Nano 14, 12313–12340. doi:10.1021/acsnano.0c05537
Leung, C. C., Lam, T. H., and Cheng, K. K. (2020). Mass Masking in the COVID-19 Epidemic: People Need Guidance. The Lancet 395, 945. doi:10.1016/s0140-6736(20)30520-1
Leung, N. H. L., Chu, D. K. W., Shiu, E. Y. C., Chan, K.-H., McDevitt, J. J., Hau, B. J. P., et al. (2020). Respiratory Virus Shedding in Exhaled Breath and Efficacy of Face Masks. Nat. Med. 26, 676–680. doi:10.1038/s41591-020-0843-2
Li, B., Wang, D., Lee, M. M. S., Wang, W., Tan, Q., Zhao, Z., et al. (2021). Fabrics Attached with Highly Efficient Aggregation-Induced Emission Photosensitizer: Toward Self-Antiviral Personal Protective Equipment. ACS Nano. 15, doi:10.1021/acsnano.1c06071
Li, Q., Yin, Y., Cao, D., Wang, Y., Luan, P., Sun, X., et al. (2021). Photocatalytic Rejuvenation Enabled Self-Sanitizing, Reusable, and Biodegradable Masks against COVID-19. ACS Nano. 15, doi:10.1021/acsnano.1c03249
Liao, W. T., Hsu, M. Y., Shen, C. F., Hung, K. F., and Cheng, C. M. (2020). Home Sample Self-Collection for COVID-19 Patients. Adv. Biosyst. 4. e2000150. doi:10.1002/adbi.202000150
Luzuriaga, M. A., Shahrivarkevishahi, A., Herbert, F. C., Wijesundara, Y. H., and Gassensmith, J. J. (2021). Biomaterials and Nanomaterials for Sustained Release Vaccine Delivery. Wiley Interdiscip. Rev. Nanomed. Nanobiotechnol. 13 (6), e1735. doi:10.1002/wnan.1735
Mahmood, S. U., Crimbly, F., Khan, S., Choudry, E., and Mehwish, S. (2020). Strategies for Rational Use of Personal Protective Equipment (PPE) Among Healthcare Providers during the COVID-19 Crisis. Cureus 12. e8248. doi:10.7759/cureus.8248
Majumder, J., and Minko, T. (2021). Recent Developments on Therapeutic and Diagnostic Approaches for COVID-19. Aaps j 23, 14. doi:10.1208/s12248-020-00532-2
McCarthy, A., Saldana, L., Ackerman, D. N., Su, Y., John, J. V., Chen, S., et al. (2021). Ultra-absorptive Nanofiber Swabs for Improved Collection and Test Sensitivity of SARS-CoV-2 and Other Biological Specimens. Nano Lett. 21, 1508–1516. doi:10.1021/acs.nanolett.0c04956
Müller, W. E. G., Neufurth, M., Lieberwirth, I., Muñoz-Espí, R., Wang, S., Schröder, H. C., et al. (2021). Triple-target Stimuli-Responsive Anti-COVID-19 Face Mask with Physiological Virus-Inactivating Agents. Biomater. Sci. 14;9(18):6052–6063. doi:10.1039/d1bm00502b
Nourmoradi, H., Mohammadi-Moghadam, F., Hemati, S., and Kakaei, H. (2021). Virus-sampling Technologies in Different Environments. Environ. Health Manage. Novel Coronavirus Dis. (Covid-19) 2021, 41–63. doi:10.1016/b978-0-323-85780-2.00010-x
Ohashi, H., Watashi, K., Saso, W., Shionoya, K., Iwanami, S., Hirokawa, T., et al. (2021). Potential Anti-COVID-19 Agents, Cepharanthine and Nelfinavir, and Their Usage for Combination Treatment. iScience 24, 102367. doi:10.1016/j.isci.2021.102367
Pan, Y., Long, L., Zhang, D., Yuan, T., Cui, S., Yang, P., et al. (2020). Potential False-Negative Nucleic Acid Testing Results for Severe Acute Respiratory Syndrome Coronavirus 2 from Thermal Inactivation of Samples with Low Viral Loads. Clin. Chem. 66, 794–801. doi:10.1093/clinchem/hvaa091
Pandit, P., Maity, S., Singha, K., Annu, M. Uzun., Shekh, M., Ahmed, S., et al. (2021). Potential Biodegradable Face Mask to Counter Environmental Impact of Covid-19, Clean. Eng. Technology 4, 100218. doi:10.1016/j.clet.2021.100218
Park, S. H. (2020). Personal Protective Equipment for Healthcare Workers during the COVID-19 Pandemic. Infect. Chemother. 52, 165–182. doi:10.3947/ic.2020.52.2.165
Parupudi, T., Panchagnula, N., Muthukumar, S., and Prasad, S. (2021). Evidence-based point-of-care Technology Development during the COVID-19 Pandemic. BioTechniques 70, 58–67. doi:10.2144/btn-2020-0096
Patil, N. A., Gore, P. M., Jaya Prakash, N., Govindaraj, P., Yadav, R., Verma, V., et al. (2021). Needleless Electrospun Phytochemicals Encapsulated Nanofibre Based 3-ply Biodegradable Mask for Combating COVID-19 Pandemic. Chem. Eng. J. 416, 129152. doi:10.1016/j.cej.2021.129152
Rahmani, A. R., Leili, M., Azarian, G., and Poormohammadi, A. (2020). Sampling and Detection of corona Viruses in Air: A Mini Review. Sci. Total Environ. 740, 140207. doi:10.1016/j.scitotenv.2020.140207
Rai, P., Kumar, B. K., Deekshit, V. K., Karunasagar, I., and Karunasagar, I. (2021). Detection Technologies and Recent Developments in the Diagnosis of COVID-19 Infection. Appl. Microbiol. Biotechnol. 105, 441–455. doi:10.1007/s00253-020-11061-5
Rokney, A., Valinsky, L., Vranckx, K., Feldman, N., Agmon, V., Moran-Gilad, J., et al. (2020). WGS-based Prediction and Analysis of Antimicrobial Resistance in Campylobacter Jejuni Isolates from Israel. Front. Cel. Infect. Microbiol. 10, 365. doi:10.3389/fcimb.2020.00365
Rowan, N. J., and Laffey, J. G. (2021). Unlocking the Surge in Demand for Personal and Protective Equipment (PPE) and Improvised Face Coverings Arising from Coronavirus Disease (COVID-19) Pandemic - Implications for Efficacy, Re-use and Sustainable Waste Management. Sci. Total Environ. 752, 142259. doi:10.1016/j.scitotenv.2020.142259
Seyfoori, A., Amereh, M., Dabiri, S. M. H., Askari, E., Walsh, T., and Akbari, M. (2021). The Role of Biomaterials and Three Dimensional (3D) In Vitro Tissue Models in Fighting against COVID-19. Biomater. Sci. 9, 1217–1226. doi:10.1039/d0bm01616k
Shekh, M. I., Amirian, J., Stadler, F. J., Du, B., and Zhu, Y. (2020). Oxidized Chitosan Modified Electrospun Scaffolds for Controllable Release of Acyclovir. Int. J. Biol. Macromolecules 151, 787–796. doi:10.1016/j.ijbiomac.2020.02.230
Smith, M. J., Hayward, S. A., Innes, S. M., and Miller, A. S. C. (2020). Point‐of‐care Lung Ultrasound in Patients with COVID ‐19 - a Narrative Review. Anaesthesia 75, 1096–1104. doi:10.1111/anae.15082
Tang, P., Zhang, Z., El-Moghazy, A. Y., Wisuthiphaet, N., Nitin, N., and Sun, G. (2020). Daylight-Induced Antibacterial and Antiviral Cotton Cloth for Offensive Personal Protection. ACS Appl. Mater. Inter. 12, 49442–49451. doi:10.1021/acsami.0c15540
Tang, Z., Kong, N., Zhang, X., Liu, Y., Hu, P., Mou, S., et al. (2020). A Materials-Science Perspective on Tackling COVID-19. Nat. Rev. Mater. 5, 1–14. doi:10.1038/s41578-020-00247-y
Tang, Z., Zhang, X., Shu, Y., Guo, M., Zhang, H., and Tao, W. (2021). Insights from Nanotechnology in COVID-19 Treatment. Nano today 36. 101019. doi:10.1016/j.nantod.2020.101019
Tebyetekerwa, M., Xu, Z., Yang, S., and Ramakrishna, S. (2020). Electrospun Nanofibers-Based Face Masks. Adv. Fiber Mater. 2, 161–166. doi:10.1007/s42765-020-00049-5
The Lancet, L. (2020). COVID-19 Vaccines: No Time for Complacency. The Lancet 396, 1607. doi:10.1016/s0140-6736(20)32472-7
Tu, Y. F., Chien, C. S., Yarmishyn, A. A., Lin, Y. Y., Luo, Y. H., Lin, Y. T., et al. (2020). A Review of SARS-CoV-2 and the Ongoing Clinical Trials. Int. J. Mol. Sci. 21. doi:10.3390/ijms21072657
Tyo, K. M., Lasnik, A. B., Zhang, L., Jenson, A. B., Fuqua, J. L., Palmer, K. E., et al. (2020). Rapid-Release Griffithsin Fibers for Dual Prevention of HSV-2 and HIV-1 Infections. Antimicrob. Agents Chemother. 64, e02139-19. doi:10.1128/AAC.02139-19
Vermeiren, C., Marchand-Senécal, X., Sheldrake, E., Bulir, D., Smieja, M., Chong, S., et al. (2020). Comparison of Copan ESwab and FLOQSwab for COVID-19 Diagnosis: Working Around a Supply Shortage. J. Clin. Microbiol. 58, e00669-20. doi:10.1128/JCM.00669-20
Wang, N., Feng, Y., Zheng, Y., Zhang, L., Feng, M., Li, X., et al. (2021). New Hydrogen Bonding Enhanced Polyvinyl Alcohol Based Self‐Charged Medical Mask with Superior Charge Retention and Moisture Resistance Performances. Adv. Funct. Mater. 31, 2009172. doi:10.1002/adfm.202009172
Weiss, C., Carriere, M., Fusco, L., Capua, I., Regla-Nava, J. A., Pasquali, M., et al. (2020). Toward Nanotechnology-Enabled Approaches against the COVID-19 Pandemic. ACS Nano 14, 6383–6406. doi:10.1021/acsnano.0c03697
West, C. P., Montori, V. M., and Sampathkumar, P. (2020). COVID-19 Testing. Mayo Clinic Proc. 95, 1127–1129. doi:10.1016/j.mayocp.2020.04.004
Whulanza, Y., Supriadi, S., Chalid, M., Kreshanti, P., Agus, A. A., Napitupulu, P., et al. (2020). Setting Acceptance Criteria for a National Flocked Swab for Biological Specimens during the COVID-19 Pandemic. IJTech 11, 888–899. doi:10.14716/ijtech.v11i5.4335
Wyllie, A. L., Fournier, J., Casanovas-Massana, A., Campbell, M., Tokuyama, M., Vijayakumar, P., et al. (2020). Saliva or Nasopharyngeal Swab Specimens for Detection of SARS-CoV-2. N. Engl. J. Med. 383, 1283–1286. doi:10.1056/nejmc2016359
Yu, D.-G., Zhu, L. M., Branford-White, C. J., Yang, J. H., Wang, X., Li, Y., et al. (2011). Solid Dispersions in the Form of Electrospun Core-Sheath Nanofibers. Ijn 6, 3271–3280. doi:10.2147/ijn.s27468
Yu, D. G., Branford‐White, C., Li, L., Wu, X. M., and Zhu, L. M. (2010). The Compatibility of Acyclovir with Polyacrylonitrile in the Electrospun Drug‐loaded Nanofibers. J. Appl. Polym. Sci. 117, 1509–1515. doi:10.1002/app.32019
Yüce, M., Filiztekin, E., and Özkaya, K. G. (2021). COVID-19 Diagnosis -A Review of Current Methods. Biosens. Bioelectron. 172, 112752. doi:10.1016/j.bios.2020.112752
Zangmeister, C. D., Radney, J. G., Vicenzi, E. P., and Weaver, J. L. (2020). Filtration Efficiencies of Nanoscale Aerosol by Cloth Mask Materials Used to Slow the Spread of SARS-CoV-2. ACS Nano 14, 9188–9200. doi:10.1021/acsnano.0c05025
Keywords: electrospun polymer composites, biomaterials, biomedical application, antiviral properties, COVID-19
Citation: Li J, Wang W, Jiang R and Guo C (2021) Antiviral Electrospun Polymer Composites: Recent Advances and Opportunities for Tackling COVID-19. Front. Mater. 8:773205. doi: 10.3389/fmats.2021.773205
Received: 09 September 2021; Accepted: 12 October 2021;
Published: 16 November 2021.
Edited by:
Evie L. Papadopoulou, Italian Institute of Technology (IIT), ItalyReviewed by:
Peng-Cheng Ma, University of Chinese Academy of Sciences, ChinaCopyright © 2021 Li, Wang, Jiang and Guo. This is an open-access article distributed under the terms of the Creative Commons Attribution License (CC BY). The use, distribution or reproduction in other forums is permitted, provided the original author(s) and the copyright owner(s) are credited and that the original publication in this journal is cited, in accordance with accepted academic practice. No use, distribution or reproduction is permitted which does not comply with these terms.
*Correspondence: Chengchen Guo, Z3VvY2hlbmdjaGVuQHdlc3RsYWtlLmVkdS5jbg==
Disclaimer: All claims expressed in this article are solely those of the authors and do not necessarily represent those of their affiliated organizations, or those of the publisher, the editors and the reviewers. Any product that may be evaluated in this article or claim that may be made by its manufacturer is not guaranteed or endorsed by the publisher.
Research integrity at Frontiers
Learn more about the work of our research integrity team to safeguard the quality of each article we publish.