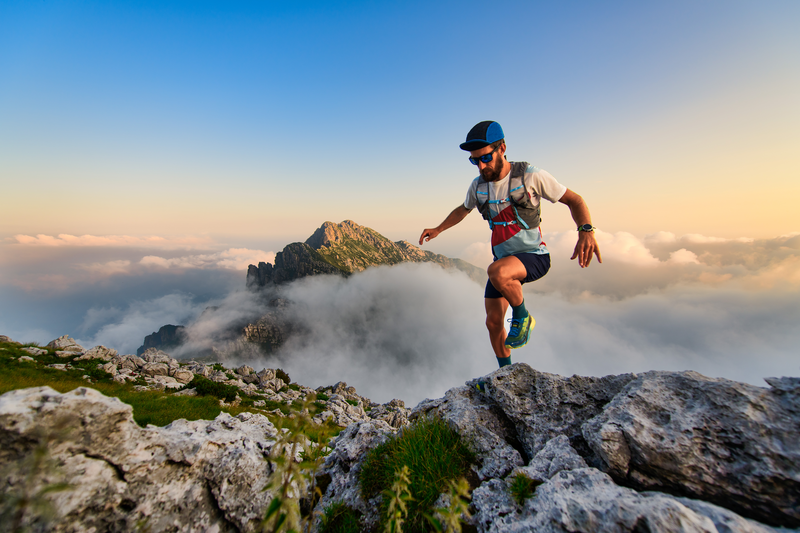
94% of researchers rate our articles as excellent or good
Learn more about the work of our research integrity team to safeguard the quality of each article we publish.
Find out more
MINI REVIEW article
Front. Mater. , 03 November 2021
Sec. Polymeric and Composite Materials
Volume 8 - 2021 | https://doi.org/10.3389/fmats.2021.769436
This article is part of the Research Topic Polymer Blends for Drug Release Systems View all 10 articles
Chronic and debilitating diseases can be marginally cured by anti-inflammatory, antiseptic, and antibiotic drugs, there is still need for more efficacious delivery approaches. Biodegradable and biocompatible polymeric hydrogels are essential requirements for drug release systems due to sustained or targeted drug delivery. Irradiation crosslinking of polymers is considered a safe route for the fabrication of hydrogels because crosslinking takes place without addition of unnecessary toxic reagents such as initiators or crosslinkers. This technology is a useful way to induce sterilization and crosslinking in a single step. Several natural and synthetic polymers in different combinations are crosslinked through high energy ionizing radiation such as electron beam and gamma ray irradiation. Polymeric hydrogels prepared using these techniques exhibit good gel fraction, swelling ratio, and mechanical properties. In addition, hydrogels possess drug loading and release characteristics, antimicrobial characteristics, and in-vivo/in-vitro cytocompatibility. The advantage of biodegradable and biocompatible drug release systems is the controlled release of drugs without deleterious effects on targeted sites. This mini review about irradiation crosslinked hydrogels will provide sufficient guidelines for new researchers to proceed further in this field.
The term “hydrogel” was first introduced and illustrated in 1894 with inorganic salt-based colloidal gels (Buwalda et al., 2014). Irradiation technology based hydrogels were introduced in 1958, when poly (vinyl alcohol) (PVA) was crosslinked using gamma ray irradiation (Buwalda et al., 2014). Lim and Wichterle pioneered the development of poly (HEMA) hydrogels, which exhibited swollen characteristics for contact lens applications (Wichterle and Lím, 1960). Hydrogels are hydrophilic crosslinked networks with three dimensional structures that can absorb large amounts of physiological fluids or water without being dissolution due to chemical or physical interactions; they can mimic soft tissue (Hoare and Kohane, 2008). Polymeric materials from natural, semi-synthetic, and synthetic origins can be utilized for hydrogel fabrication. The preparation of hydrogels includes crosslinking of linear chains or simultaneous crosslinking and polymerization of monomers with poly functional monomers (Nugent and Higginbotham, 2007).
In polymeric hydrogels, crosslink density and hydrophilicity of the polymer are governing factors for the swelling capability and extent of swelling. Generally, mass fragments of water in the swollen state of the hydrogel are greater than mass fragments of polymer. Hydrogels can be further classified into two classes–permanent gels (chemical gels), which involve covalent linkages of chains, and pseudo gels (physical gels), which involve chain entanglements, electrostatic forces, hydrophobic interactions, or hydrogen bonds (physical gels are usually non-permanent and heating can convert them back to polymer solutions) (Benamer et al., 2006).
Currently, several crosslinking methods (chemical, physical, and irradiation) are being used (Syed K.H. Gulrez et al., 2010). Among the chemical methods, the most commonly used chemical additives are formaldehyde and glutaraldehyde. However, several studies have reported that chemical additives provoke cytotoxicity, which makes these method undesirable for pharmaceutical and biomedical applications (Yang et al., 2010). The irradiation crosslinking method is promising and clean process due to several advantages such as hydrogel fabrication and sterilization in a single step, simple process control, and absence of toxic reagents (crosslinkers and initiators), which could be difficult to remove (Benamer et al., 2006; Krklješ et al., 2007).
Stimuli responsive superabsorbents or hydrogels are triggered with even small external stimuli and undergo abrupt changes in network structure, growth, permeability, and mechanical strength due to the presence of so-called smart or environmentally sensitive hydrogels. Chemical stimuli comprising of chemical agents, ionic factors, and pH, alter the interaction at the molecular level between polymer chains, and interactions between solvents and polymer chains. On the other hand, physical stimuli include pressure, light, temperature, magnetic fields, electric fields, intensity of various energy sources, and mechanical stress, which alter interactions at the molecular level at critical onset points. Dual responsive hydrogels are another class that involves a combination of two stimuli responses in one hydrogel. Biochemical stimuli responsive hydrogels involve responses to enzyme, antigens, ligands, and several other biochemical entities. Hence, responsive hydrogels have huge potential for use as biomaterials for biotechnology, biomedical, and pharmaceutical applications (Zhang et al., 2015).
Recently, drug development has led to several controlled/targeted drug delivery approaches that consist of suitable polymer carriers containing encapsulated drugs and that deliver drugs in sustained fashion/novel route or both. Drugs can be made accessible at numerous locations within the body by choosing suitable biocompatible carriers. Targeted drug delivery to various tissues or organs can be simultaneously complemented with auxiliary benefits of obtaining both temporally and spatially controlled release, in accordance with the fundamental purpose of drug delivery. Controlled release of a drug and targeted drug delivery to diseased organs or tissues have several advantages compared to the oral (traditional) route, such as that higher drug concentration makes therapy more effective; reduction in undesired side effects and duration of therapy; and drug release sustains improved pharmacokinetics over an extended time period. In the end, convenient and simplified dosage are attributed to the wellbeing of the patient because they reduce the necessity of potentially uncomfortable repeated dosing and shorten the chances of inappropriate dispensing (Ražem and Katušin-Ražem, 2008).
In this mini review, our focus is on discussing the fabrication of natural and synthetic polymers base hydrogels for drug release systems. We explicitly describe the utilization of gamma ray and electron beam irradiation techniques for hydrogel development, the mechanism of crosslinking, the impact of crosslinking, and applications of these methods in drug release systems.
Natural polymer-based hydrogels can be prepared through chemical or physical crosslinking mechanisms (Ullah et al., 2015; Lou et al., 2020). The chemical crosslinking procedure induces higher mechanical strength and durability due to interconnectivity fostered through covalent bonds. The irradiation fabrication of a hydrogel is preferable as it produces sterile and pure hydrogels quickly and in the absence of toxic chemical agents (Moghaddam et al., 2019). Nevertheless, natural polymer hydrogel fabrication in aqueous solution through high ionizing energy irradiation method produces low molecular weight products, instead of good hydrogel formation, due to chain cleavage reactions (Hafezi Moghaddam et al., 2019). To overcome this issue, a blend of natural polymer and vinyl reagent or synthetic polymer has been used instead of mere natural polymers (Prusty and Swain, 2018; Moghaddam et al., 2019).
In comparison to neat polymers, polymer blends are regarded as a dominant material in the fabrication of better performance low-cost products. Composites and blends are expanding the utilization of polymers from renewable resources to recently-developed valuable products (Yu et al., 2006). The main purpose of blend formation of two or more polymers is to optimize the blend performance and to not dramatically alter the individual properties (Matveev et al., 2000). Nowadays, it is common practice to use the polymer modification process to blend two or more polymers to acquire desired properties. Polymer blends produce rare and superior properties that homo-polymers do not exhibit. Generally, chemical, physical, and irradiation techniques are used for the synthesis of polymer blends. In particular, the irradiation method is very appropriate tool that involves modification or improvement of polymeric materials through degradation, grafting, or cross-linking. Numerous research studies have been carried out in this regard (Güven et al., 1999; Bhattacharya, 2000). Natural and synthetic polymer-based blends for biomedical applications have drawn attention for their biodegradable nature (Carenza, 1992; Crescenzi et al., 1997). These blends have different material properties and can be used as hydrogels in the fields of pharmacy and biomedicine (Rosiak, 1994; Crescenzi et al., 1997; Rosiak and Ulański, 1999; Rosiak and Yoshii, 1999).
On the electromagnetic spectrum, gamma rays fall in the high ionizing energy region, which has the ability to penetrate most materials. Gamma rays are radiation-source dependent high-frequency waves. Usually, radioactive nuclides such as cesium-137 and cobalt-60, are the radioactive sources used in this technology. Electron beam involve beams of energetic electrons from electron accelerators. Industrial electron beam accelerators have been categorized with respect to energy range such as high energy (>5 MeV), medium energy (300 keV–5 MeV), and low energy (80–300 keV) (Shahidi, 2019).
Unsaturated compounds are polymerized through high ionizing energy irradiation, particularly using gamma rays and electron beams. Water soluble polymers containing vinyl groups undergo hydrogel formation when exposed to high ionizing energy irradiation (Giammona et al., 1999). These irradiations generate free radicals on the polymeric chains of the water-soluble polymers through C-H bond homolytic scission. In addition, water molecules undergo radiolysis, which creates hydroxyl radicals that further interact with polymeric chains to form macroradicals (Peppas and Mikos, 1986). Then, macroradicals generate intra- and inter-molecular interactions to form covalent bonded hydrogel networks. The irradiation crosslinked hydrogel fabrication process is usually carried out in inert atmosphere (argon or nitrogen) to avoid oxygen interaction with macroradicals in the propagation stage of polymerization. Recently, poly (acrylic acid) (Jabbari and Nozari, 2000), poly (ethylene glycol) (Kofinas et al., 1996), and poly (vinyl alcohol) (Peppas and Merrill, 1977) based hydrogels were crosslinked using high ionizing energy irradiation. Also, poly (methyl vinyl ether) was synthesized using irradiation crosslinking to form a thermosensitive hydrogel (Arndt et al., 2001). The advantage of irradiation technology for hydrogel formation is that this method can be performed in aqueous phase under mild conditions (physiological pH and room temperature).
Recently, hydrogel fabrication through gamma ray irradiation has gained enormous interest. This irradiation technique is suitable for the modification of the physical and chemical properties of polymers (Jha et al., 2010; Raza and Park, 2020). Furthermore, gamma ray irradiation has several advantages as compare to the thermal activation process such as polymerization in the absence of any extra reagent and crosslinking (Park et al., 2013). Exploiting these benefits, hydrogels prepared through gamma ray irradiation are useful for medical applications, for which even minor contamination is prohibited; these materials are employed to sterilize biomedical devices for veterinary and medical applications (Gad, 2008; Juby et al., 2012). During gamma ray irradiation, the polymers crosslink such that the backbone chains of polysaccharide polymers form chemical bonds and form a three-dimensional network structure. The irradiation method of polymers promotes quantitative changes and reproducibility in the absence of chemical additives and simultaneously allows sterilization of products (Eid et al., 2009).
Similar to free radical polymerization, electron beam and gamma polymerizations also undergo initiation, propagation, and termination steps (Hennink and Nostrum, 2002). The hydrogel formation occurs when the network achieves the critical gelation point. Hydrogel fabrication and sterilization through electron beam technology in single step is a rapid and convenient method because the crosslinking process completes in a short time at ambient temperature, there is no production of radioactive waste, and process control is easy (Rosiak, 1994; Raza et al., 2021).
In irradiation technology, chemical reactions initiate in aqueous mediums which starts generating series of reactive species due to radiolysis of water. Molecular products and radical species with different reactivities such as e-aq, H⋅, HO⋅, H2O2, H2, and H3O+ are produced as given below in equation. In argon saturated or deoxygenated polymer solutions, e-aq and HO⋅ (hydroxyl radical) govern the highest yield (Spinks and Woods, 1990). The H⋅ and HO⋅ radicals interacts quickly with polymeric chains via hydrogen removal which give rise to series of macroradicals formation depending upon polymer concentration. These macroradicals undergoes inter or intra-molecular free radical recombination reactions and produce interconnected polymer networks having permanent and stable structure (Treloar, 1975).
Figure 1 depicts applications of hydrogels in different areas. Research on the preparation of various hydrogels (ion and electrical conductive materials, and oral patches) for effective drug release using radiation crosslinking technology has been steadily undertaken. When a hydrophilic polymer is dissolved in water and irradiated with radiation, radicals are formed on the water molecules of H and OH by the radiation to attack with low bonding strength of the hydrophilic polymer. Since this is a very unstable state, radical recombination occurs between chains in which radicals are formed and crosslinking proceeds (Park et al., 2018; Jeong et al., 2020).
Table 1 shows natural and synthetic polymer based irradiation crosslinked hydrogels with irradiation types and different irradiation doses, and release of drugs. Herein, hydrogels crosslinked with both types of irradiation (electron beam and gamma ray irradiation) are discussed. Electron beam irradiation involves beam energies (usually 2.5 or 10 MeV), and parameters such as beam power, beam current and cart speed need to be adjusted for optimum crosslinking. Hafezi et al. used electron beam irradiation for the fabrication of hydrogels for drug release applications. In case of CA-O-CMCh/PAAM, irradiation dose (10–35 kGy) was used for crosslinking and doxycycline drug was loaded for release analysis. While in case of 5-HTP/Pectin, 10–50 kGy irradiation dose is utilized for tetracycline drug release. CMSP and CMSP/chitosan based hydrogels were prepared with different irradiation doses for the release of ciprofloxacin HCL and diclofenac sodium. Bacterial cellulose/acrylic acid crosslinked hydrogels were prepared at 35 or 50 kGy for the bovine serum albumin encapsulation and release. Also, Agarose and tyramine–high methoxyl content gum tragacanth based electron beam crosslinked hydrogels are prepared without involving drug loading and release.
Gamma ray irradiation involves gradual increase in dose with respect to time (k or Gy/h) to attain desired dose. Zhao et al. and Taşdelen et al. prepared different combinations of natural and synthetic polymers (HA/CS/PVA and HA/CS/HAP) using gamma irradiation for the release of cefazoline and theophylline, and 5-FU, respectively. While, Sabaghi et al. fabricated chitosan nanopartiles containing catechins and mixed with CS/PVA and crosslinked at 0, 40 and 60 kGy for drug release of catechins in different low fat and high fat simulant and studied modeling of release.
Gelatin and combination with cellulose in different forms were incorporated at lower irradiation doses and applied for methylene blue and riboflavin release analysis. Dextran/Poly (N-isopropylacrylamide) based thermo-sensitive hydrogels were irradiation crosslinked at 5 kGy and studied release pattern of ondansetron. Also, 2-hydroxyethyl methacrylate/itaconic acid copolymeric hydrogels were polymerized at 25 kGy for the drug delivery of theophylline and fenethylline hydrochloride, measured drug release kinetics and predicted drug release follows Fickian diffusion mechanism.
Advances in the preparation of hydrogels for drug release using blending of various polymers with useful properties and radiation technology are indicating future aspects of technology. However, there are still many technical problems that need to be solved. In particular, further study on crosslinking properties related to various polymers and/or polymer blending by irradiation are needed. Since radiation is a high energy state, it can induce various reactions such as crosslinking, degradation, polymerization, and grafting, depending on the characteristics of polymers. Therefore, to prepare a hydrogel for drug delivery, it is necessary to study the radiation crosslinking properties according to the radiation device type and radiation dose, and the type of polymer. However, it is difficult to optimize the conditions when preparing a hydrogel using radiation and time consuming due to the absence of basic research. From this point of view, research on the preparation of hydrogels for drug release after blending of polymers and drugs, and the preparation of hydrogels through irradiation (such as gamma ray and electron beam) and various polymers, all of which have been recently studied, could be said to be very noteworthy. The study of superior polymer crosslinking properties induced by irradiation is directly related to the preparation of hydrogels for drug release and could play a promising role in the development of effective drug-releasing hydrogels.
MR and J-OJ performed the literature survey and prepared the manuscript. SP supervised, reviewed, and revised the draft. All authors read and agreed on the final version.
This research was funded by the Korea Atomic Energy Research Institute (KAERI) grant number 523140-20.
The authors declare that the research was conducted in the absence of any commercial or financial relationships that could be construed as a potential conflict of interest.
All claims expressed in this article are solely those of the authors and do not necessarily represent those of their affiliated organizations, or those of the publisher, the editors and the reviewers. Any product that may be evaluated in this article, or claim that may be made by its manufacturer, is not guaranteed or endorsed by the publisher.
Almeida, J. F., Ferreira, P., Alves, P., Lopes, A., and Gil, M. H. (2013). Synthesis of a Dextran Based Thermo-Sensitive Drug Delivery System by Gamma Irradiation. Int. J. Biol. Macromolecules 61, 150–155. doi:10.1016/j.ijbiomac.2013.07.002
Benamer, S., Mahlous, M., Boukrif, A., Mansouri, B., and Youcef, S. L. (2006). Synthesis and Characterisation of Hydrogels Based on Poly(vinyl Pyrrolidone). Nucl. Instr. Methods Phys. Res. Section B: Beam Interactions Mater. Atoms 248, 284–290. doi:10.1016/j.nimb.2006.04.072
Bhattacharya, A. (2000). Radiation and Industrial Polymers. Prog. Polym. Sci. 25, 371–401. doi:10.1016/S0079-6700(00)00009-5
Buwalda, S. J., Boere, K. W. M., Dijkstra, P. J., Feijen, J., Vermonden, T., and Hennink, W. E. (2014). Hydrogels in a Historical Perspective: From Simple Networks to Smart Materials. J. Controlled Release 190, 254–273. doi:10.1016/J.JCONREL.2014.03.052
Carenza, M. (1992). Recent Achievements in the Use of Radiation Polymerization and Grafting for Biomedical Applications. Int. J. Radiat. Appl. Instrumentation. C. Radiat. Phys. Chem. 39, 485–493. doi:10.1016/1359-0197(92)90101-K
Crescenzi, V., Paradossi, G., Desideri, P., Dentini, M., Cavalieri, F., Amici, E., et al. (1997). New Hydrogels Based on Carbohydrate and on Carbohydrate-Synthetic Polymer Networks. Polym. Gels Networks 5, 225–239. doi:10.1016/S0966-7822(96)00051-2
Eid, M., Abdel-Ghaffar, M. A., and Dessouki, A. M. (2009). Effect of Maleic Acid Content on the thermal Stability, Swelling Behaviour and Network Structure of Gelatin-Based Hydrogels Prepared by Gamma Irradiation. Nucl. Instr. Methods Phys. Res. Section B: Beam Interactions Mater. Atoms 267, 91–98. doi:10.1016/J.NIMB.2008.11.011
Gad, Y. H. (2008). Preparation and Characterization of Poly(2-Acrylamido-2-Methylpropane-Sulfonic acid)/Chitosan Hydrogel Using Gamma Irradiation and its Application in Wastewater Treatment. Radiat. Phys. Chem. 77, 1101–1107. doi:10.1016/J.RADPHYSCHEM.2008.05.002
Giammona, G., Pitarresi, G., Cavallaro, G., Buscemi, S., and Saiano, F. (1999). New Biodegradable Hydrogels Based on a Photocrosslinkable Modified Polyaspartamide: Synthesis and Characterization. Biochim. Biophys. Acta 1428, 29–38. doi:10.1016/s0304-4165(99)00051-3
Gulrez, S. K. H., Al-Assaf, S., and Phillips, G. O. (2010). Hydrogels: Methods of Preparation. Prog. Mol. Environ. Bioeng. Anal. Model. Technol. Appl. 51, 1248–1259. doi:10.1016/j.jvs.2009.12.028
Güven, O., Şen, M., Karadağ, E., and Saraydın, D. (1999). A Review on the Radiation Synthesis of Copolymeric Hydrogels for Adsorption and Separation Purposes. Radiat. Phys. Chem. 56, 381–386. doi:10.1016/S0969-806X(99)00326-6
Hafezi Moghaddam, R., Dadfarnia, S., Shabani, A. M. H., Amraei, R., and Hafezi Moghaddam, Z. (2020). Doxycycline Drug Delivery Using Hydrogels of O-Carboxymethyl Chitosan Conjugated with Caffeic Acid and its Composite with Polyacrylamide Synthesized by Electron Beam Irradiation. Int. J. Biol. Macromolecules 154, 962–973. doi:10.1016/j.ijbiomac.2020.03.165
Hafezi Moghaddam, R., Dadfarnia, S., Shabani, A. M. H., Moghaddam, Z. H., and Tavakol, M. (2019). Electron Beam Irradiation Synthesis of Porous and Non-Porous Pectin Based Hydrogels for a Tetracycline Drug Delivery System. Mater. Sci. Eng. C 102, 391–404. doi:10.1016/j.msec.2019.04.071
Hennink, W. E., and van Nostrum, C. F. (2002). Novel Crosslinking Methods to Design Hydrogels. Adv. Drug Deliv. Rev. 54, 13–36. doi:10.1016/j.addr.2012.09.00910.1016/s0169-409x(01)00240-x
Hoare, T. R., and Kohane, D. S. (2008). Hydrogels in Drug Delivery: Progress and Challenges. Polymer 49, 1993–2007. doi:10.1016/j.polymer.2008.01.027
Jabbari, E., and Nozari, S. (2000). Swelling Behavior of Acrylic Acid Hydrogels Prepared by γ-Radiation Crosslinking of Polyacrylic Acid in Aqueous Solution. Eur. Polym. J. 36, 2685–2692. doi:10.1016/S0014-3057(00)00044-6
Jeong, J.-O., Park, J.-S., Kim, Y.-A., Yang, S.-J., Jeong, S.-I., Lee, J.-Y., et al. (2020). Gamma Ray-Induced Polymerization and Cross-Linking for Optimization of PPy/PVP Hydrogel as Biomaterial. Polymers 12, 111. doi:10.3390/polym12010111
Jha, P. K., Jha, R., Gupta, B. L., and Guha, S. K. (2010). Effect of γ-Dose Rate and Total Dose Interrelation on the Polymeric Hydrogel: A Novel Injectable Male Contraceptive. Radiat. Phys. Chem. 79, 663–671. doi:10.1016/J.RADPHYSCHEM.2009.11.010
Juby, K. A., Dwivedi, C., Kumar, M., Kota, S., Misra, H. S., and Bajaj, P. N. (2012). Silver Nanoparticle-Loaded PVA/Gum Acacia Hydrogel: Synthesis, Characterization and Antibacterial Study. Carbohydr. Polym. 89, 906–913. doi:10.1016/J.CARBPOL.2012.04.033
Arndt, K. F., Schmidt, T., and Reichelt, R. (2001). Thermo-Sensitive Poly(Methyl Vinyl Ether) Micro-Gel Formed by High Energy Radiation. Polymer (Guildf). 42, 6785–6791. doi:10.1016/S0032-3861(01)00164-1
Kofinas, P., Athanassiou, V., and Merrill, E. W. (1996). Hydrogels Prepared by Electron Irradiation of Poly(ethylene Oxide) in Water Solution: Unexpected Dependence of Cross-Link Density and Protein Diffusion Coefficients on Initial PEO Molecular Weight. Biomaterials 17, 1547–1550. doi:10.1016/0142-9612(96)89781-X
Kojima, T., Bessho, M., Furuta, M., Okuda, S., and Hara, M. (2004). Characterization of Biopolymer Hydrogels Produced by γ-ray Irradiation. Radiat. Phys. Chem. 71, 235–238. doi:10.1016/j.radphyschem.2004.04.069
Krklješ, A. N., Marinović-Cincović, M. T., Kacarevic-Popovic, Z. M., and Nedeljković, J. M. (2007). Radiolytic Synthesis and Characterization of Ag-PVA Nanocomposites. Eur. Polym. J. 43, 2171–2176. doi:10.1016/J.EURPOLYMJ.2007.03.023
Krömmelbein, C., Mütze, M., Konieczny, R., Schönherr, N., Griebel, J., Gerdes, W., et al. (2021). Impact of High-Energy Electron Irradiation on Mechanical, Structural and Chemical Properties of Agarose Hydrogels. Carbohydr. Polym. 263, 117970. doi:10.1016/j.carbpol.2021.117970
Lam, Y. L., Muniyandy, S., Kamaruddin, H., Mansor, A., and Janarthanan, P. (2015). Radiation Cross-Linked Carboxymethyl Sago Pulp Hydrogels Loaded with Ciprofloxacin: Influence of Irradiation on Gel Fraction, Entrapped Drug and In Vitro Release. Radiat. Phys. Chem. 106, 213–222. doi:10.1016/j.radphyschem.2014.07.018
Lou, C., Tian, X., Deng, H., Wang, Y., and Jiang, X. (2020). Dialdehyde-β-Cyclodextrin-Crosslinked Carboxymethyl Chitosan Hydrogel for Drug Release. Carbohydr. Polym. 231, 115678. doi:10.1016/J.CARBPOL.2019.115678
Matveev, Y., Grinberg, V. Y., and Tolstoguzov, V. B. (2000). The Plasticizing Effect of Water on Proteins, Polysaccharides and Their Mixtures. Glassy State of Biopolymers, Food and Seeds. Food Hydrocoll 14, 425–437. doi:10.1016/S0268-005X(00)00020-5
Moghaddam, R. H., Dadfarnia, S., Shabani, A. M. H., and Tavakol, M. (2019). Synthesis of Composite Hydrogel of Glutamic Acid, Gum Tragacanth, and Anionic Polyacrylamide by Electron Beam Irradiation for Uranium (VI) Removal from Aqueous Samples: Equilibrium, Kinetics, and Thermodynamic Studies. Carbohydr. Polym. 206, 352–361. doi:10.1016/J.CARBPOL.2018.10.030
Mohd Amin, M. C. I., Ahmad, N., Halib, N., and Ahmad, I. (2012). Synthesis and Characterization of Thermo- and pH-Responsive Bacterial Cellulose/acrylic Acid Hydrogels for Drug Delivery. Carbohydr. Polym. 88, 465–473. doi:10.1016/j.carbpol.2011.12.022
Nugent, M. J. D., and Higginbotham, C. L. (2007). Preparation of a Novel Freeze Thawed Poly(vinyl Alcohol) Composite Hydrogel for Drug Delivery Applications. Eur. J. Pharmaceutics Biopharmaceutics 67, 377–386. doi:10.1016/J.EJPB.2007.02.014
Park, J.-S., Kuang, J., Gwon, H.-J., Lim, Y.-M., Jeong, S.-I., Shin, Y.-M., et al. (2013). Synthesis and Characterization of Zinc Chloride Containing Poly(acrylic Acid) Hydrogel by Gamma Irradiation. Radiat. Phys. Chem. 88, 60–64. doi:10.1016/J.RADPHYSCHEM.2013.03.018
Park, J.-S., Lim, Y.-M., Baik, J., Jeong, J.-O., An, S.-J., Jeong, S.-I., et al. (2018). Preparation and Evaluation of β-Glucan Hydrogel Prepared by the Radiation Technique for Drug Carrier Applications. Int. J. Biol. Macromolecules 118, 333–339. doi:10.1016/j.ijbiomac.2018.06.068
Peppas, N. A., and Merrill, E. W. (1977). Crosslinked Poly(vinyl Alcohol) Hydrogels as Swollen Elastic Networks. J. Appl. Polym. Sci. 21, 1763–1770. doi:10.1002/app.1977.070210704
Peppas, N. A., and Mikos, A. G. (1986). Preparation Methods and Structure of Hydrogels. 1st Edition. United States: CRC Press.
Prusty, K., and Swain, S. K. (2018). Nano Silver Decorated Polyacrylamide/Dextran Nanohydrogels Hybrid Composites for Drug Delivery Applications. Mater. Sci. Eng. C 85, 130–141. doi:10.1016/J.MSEC.2017.11.028
Raza, M. A., Lim, Y.-M., Lee, S.-W., Seralathan, K.-K., and Park, S. H. (2021). Synthesis and Characterization of Hydrogels Based on Carboxymethyl Chitosan and Poly(Vinylpyrrolidone) Blends Prepared by Electron Beam Irradiation Having Anticancer Efficacy, and Applications as Drug Carrier for Controlled Release of Drug. Carbohydr. Polym. 258, 117718. doi:10.1016/j.carbpol.2021.117718
Raza, M. A., and Park, S. H. (2020). Irradiated Ch/GG/PVP-Based Stimuli-Responsive Hydrogels for Controlled Drug Release. J. Appl. Polym. Sci. 137, 49041–49114. doi:10.1002/app.49041
Ražem, D., and Katušin-Ražem, B. (2008). The Effects of Irradiation on Controlled Drug Delivery/Controlled Drug Release Systems. Radiat. Phys. Chem. 77, 288–344. doi:10.1016/j.radphyschem.2007.06.006
Rosiak, J. M., and Ulański, P. (1999). Synthesis of Hydrogels by Irradiation of Polymers in Aqueous Solution. Radiat. Phys. Chem. 55, 139–151. doi:10.1016/S0969-806X(98)00319-3
Rosiak, J. M., and Yoshii, F. (1999). Hydrogels and Their Medical Applications. Nucl. Instr. Methods Phys. Res. Section B: Beam Interactions Mater. Atoms 151, 56–64. doi:10.1016/S0168-583X(99)00118-4
Rosiak, J. (1994). Radiation Formation of Hydrogels for Drug Delivery. J. Controlled Release 31, 9–19. doi:10.1016/0168-3659(94)90246-1
Sabaghi, M., Maghsoudlou, Y., Kashiri, M., and Shakeri, A. (2020). Evaluation of Release Mechanism of Catechin from Chitosan-Polyvinyl Alcohol Film by Exposure to Gamma Irradiation. Carbohydr. Polym. 230, 115589. doi:10.1016/j.carbpol.2019.115589
Shahidi, S. (2019). “Effect of Irradiation for Producing the Conductive and Smart Hydrogels,” in Polymers and Polymeric Composites: A Reference Series,Cellulose-Based Superabsorbent Hydrogels (Cham: Springer), 625–653. doi:10.1007/978-3-319-77830-3_22
Spinks, J. W. T., and Woods, R. J. (1990). An Introduction to Radiation Chemistry. Hoboken, NJ, USA: John Wiley & Sons.
Tan, L. S., Tan, H. L., Deekonda, K., Wong, Y. Y., Muniyandy, S., Hashim, K., et al. (2021). Fabrication of Radiation Cross-Linked Diclofenac Sodium Loaded Carboxymethyl Sago Pulp/chitosan Hydrogel for Enteric and Sustained Drug Delivery. Carbohydr. Polym. Tech. Appl. 2, 100084. doi:10.1016/j.carpta.2021.100084
Taşdelen, B., Erdoğan, S., and Bekar, B. (2018). Radiation Synthesis and Characterization of Chitosan/Hyraluronic Acid/Hydroxyapatite Hydrogels: Drug Uptake and Drug Delivery Systems. Mater. Today Proc. 5, 15990–15997. doi:10.1016/j.matpr.2018.05.043
Tavakol, M., Dehshiri, S., and Vasheghani-Farahani, E. (2016). Electron Beam Irradiation Crosslinked Hydrogels Based on Tyramine Conjugated Gum Tragacanth. Carbohydr. Polym. 152, 504–509. doi:10.1016/j.carbpol.2016.07.044
Tomić, S. L., Mićić, M. M., Filipović, J. M., and Suljovrujić, E. H. (2007). Swelling and Drug Release Behavior of Poly(2-Hydroxyethyl Methacrylate/itaconic Acid) Copolymeric Hydrogels Obtained by Gamma Irradiation. Radiat. Phys. Chem. 76, 801–810. doi:10.1016/j.radphyschem.2006.05.013
Treloar, L. R. G. (1975). The Physics of Rubber Elasticity. (USA): New York, NY, USA: Oxford University Press.
Ullah, F., Othman, M. B. H., Javed, F., Ahmad, Z., and Akil, H. M. (2015). Classification, Processing and Application of Hydrogels: A Review. Mater. Sci. Eng. C 57, 414–433. doi:10.1016/J.MSEC.2015.07.053
Wan Ishak, W., Ahmad, I., Ramli, S., and Mohd Amin, M. (2018). Gamma Irradiation-Assisted Synthesis of Cellulose Nanocrystal-Reinforced Gelatin Hydrogels. Nanomaterials 8, 749–813. doi:10.3390/nano8100749
Wichterle, O., and Lím, D. (1960). Hydrophilic Gels for Biological Use. Nature 185, 117–118. doi:10.1038/185117a0
Yang, C., Xu, L., Zhou, Y., Zhang, X., Huang, X., Wang, M., et al. (2010). A Green Fabrication Approach of Gelatin/CM-Chitosan Hybrid Hydrogel for Wound Healing. Carbohydr. Polym. 82, 1297–1305. doi:10.1016/J.CARBPOL.2010.07.013
Yu, L., Dean, K., and Li, L. (2006). Polymer Blends and Composites from Renewable Resources. Prog. Polym. Sci. 31, 576–602. doi:10.1016/j.progpolymsci.2006.03.002
Zhang, W., Zhu, S., Bai, Y., Xi, N., Wang, S., Bian, Y., et al. (2015). Glow Discharge Electrolysis Plasma Initiated Preparation of temperature/pH Dual Sensitivity Reed Hemicellulose-Based Hydrogels. Carbohydr. Polym. 122, 11–17. doi:10.1016/J.CARBPOL.2015.01.007
Keywords: hydrogels, polymeric blends, irradiation crosslinking, biomedical applications, drug release systems
Citation: Raza MA, Jeong J-O and Park SH (2021) State-of-the-Art Irradiation Technology for Polymeric Hydrogel Fabrication and Application in Drug Release System. Front. Mater. 8:769436. doi: 10.3389/fmats.2021.769436
Received: 02 September 2021; Accepted: 21 October 2021;
Published: 03 November 2021.
Edited by:
Nafisa Gull, University of the Punjab, PakistanReviewed by:
Sunan Tiptipakorn, Kasetsart University, ThailandCopyright © 2021 Raza, Jeong and Park. This is an open-access article distributed under the terms of the Creative Commons Attribution License (CC BY). The use, distribution or reproduction in other forums is permitted, provided the original author(s) and the copyright owner(s) are credited and that the original publication in this journal is cited, in accordance with accepted academic practice. No use, distribution or reproduction is permitted which does not comply with these terms.
*Correspondence: Sang Hyun Park, cGFya3NoQGthZXJpLnJlLmty
†These authors have contributed equally to this work
Disclaimer: All claims expressed in this article are solely those of the authors and do not necessarily represent those of their affiliated organizations, or those of the publisher, the editors and the reviewers. Any product that may be evaluated in this article or claim that may be made by its manufacturer is not guaranteed or endorsed by the publisher.
Research integrity at Frontiers
Learn more about the work of our research integrity team to safeguard the quality of each article we publish.