- The State Key Laboratory Breeding Base of Basic Science of Stomatology (Hubei-MOST) and Key Laboratory of Oral Biomedicine, Ministry of Education, School of Stomatology, Wuhan University, Wuhan, China
Staphylococcus aureus (S. aureus) is the most common cause of hospital and community-acquired infections. The current clinical treatment is limited by the emergence of drug-resistant strains. We previously developed a chimeric ClyC that effectively inhibited S. aureus strains. Nonetheless, an efficient delivery system to provide sustained release of ClyC to infected site is needed. Thus, we engineered a chimeric ClyC loaded alginate hydrogel (ClyC-AH) to improve the therapeutic outcomes against S. aureus. ClyC-AH retained the stability and activity of ClyC while providing a sustained release of ClyC and a continuous antibacterial effect against S. aureus. Compared to ClyC alone, the use of ClyC-AH was relatively safe, as there was no significant cytotoxicity to BHK-21 cells at a ClyC concentration≤250 μg/ml. Furthermore, in a S. aureus infected mouse model of osteomyelitis, ClyC-AH reduced bacterial burden in the femur and surrounding tissues, with a reduction of 2 log10 (CFU/ml) in viable bacterial number. Based on these results, hydrogel-delivered chimeric lysin ClyC provides a promising future in the S.aureus targeting therapy.
Introduction
Staphylococcus aureus (S. aureus) is a Gram-positive pathogen that primarily colonizes on the nostrils, skin, and mucosal membranes of human (Lowy, 1998; Becker and Bubeck Wardenburg, 2015). S. aureus is the main conditional pathogen contributing to hospital and community-acquired infections, leading to infectious diseases such as osteomyelitis (Favero, 2003; Lindsay and Holden, 2004).
Osteomyelitis is a challenging infectious medical condition for orthopaedic surgeons (Lew and Waldvogel, 2004; Maffulli et al., 2016). Approximately 44% of the osteomyelitis are caused by S. aureus (Kremers et al., 2015), through hematogenous infection or the spread of a contiguous source. Treatment for osteomyelitis mainly include use of antibiotics and surgical debridement (Conterno and Turchi, 2013). However, S. aureu could directly invade and colonize the osteocyte lacuno-canicular network (Maffulli et al., 2016; Urish and Cassat, 2020; Gimza and Cassat, 2021), which might bring difficulty to anti-infectious treatment. In addition, the pathogenic characteristics of S. aureu, including biofilm formation, transmission of drug-resistant genes, and intracellular survival (Pantosti et al., 2007; Davies and Davies, 2010; Munita and Arias, 2016), further limit the therapeutic outcomes. Thus, finding novel antibacterial agents against S. aureus has captured the attention of scientists in recent years.
Endolysins are enzymes encoded by bacteriophages, which specifically target and degrade the peptidoglycan externally, to cleave bacteria (Haddad Kashani et al., 2018; Gondil et al., 2020b). Endolysins have strong bactericidal activity and low susceptibility to resistance. Thus, they are emerging as a viable alternative to antibiotics (Abdelrahman et al., 2021). Some endolysins are successfully applied in clinical trials. CF-301 has showed impressive results in treating patients with S.aureus bloodstream infections and endocarditis. Furthermore, CF-301 has been used in phase 3 clinical trials, indicating the potential effect of endolysins on bacterial infections (Jun et al., 2017; Fowler et al., 2020).
ClyC, a novel chimeric lysin, has specificity and robust killing efficacy against drug-resistant and susceptible S. aureus and other staphylococcal, the effect of which could be further enhanced with calcium. Also, ClyC protected mice from lethal systemic S. aureus infection (Li et al., 2021). However, the effect of endolysins are limited by its instability and short half-life in vivo, as well as the cytotoxicity tested in vitro (Resch et al., 2011; Seijsing et al., 2018). Thus, a suitable delivery mechanism is required to prevent ClyC from degradation, enhance the biocompatibility, and facilitate ClyC entry into the infection sites (Pinto et al., 2021).
Alginate is a linear copolymer derived from brown seaweed that contains repeating units of β-1,4-linked D-mannuronic acid and L-guluronic acid residues. Alginate hydrogels are widely used in wound dressing and bone and cardiac tissue engineering due to their biocompatibility, low cytotoxicity, low immunogenicity, and biodegradability (Ruvinov and Cohen, 2016; Varaprasad et al., 2020). They are ideal materials for the delivery of protein drugs because they can be prepared under mild pH and temperature conditions (Lee and Mooney, 2012). Antibacterial agents have been encapsulated in alginate hydrogel in order to target specific pathogens and reduce infections. LysMR-5 incorporated into alginate-chitosan nanoparticles recently exhibited enhanced bactericidal activity against S. aureus in vitro, but its stability was not assessed in that study (Kaur et al., 2020).
In this study, we designed ClyC loaded alginate hydrogel (ClyC-AH) to treat osteomyelitis caused by S. aureus (Figure 1). Through the encapsulation process, the stability and bactericidal activity of endolysin were optimized. We hypothesized that the ClyC-AH could sustainably release ClyC, which process the property of ClyC remained stable and the cytotoxicity reduced. Furthermore, we tested the efficacy of ClyC-AH in a S. aureus infected osteomyelitis mouse model. The change of bacteria colony forming unit (CFU) was tested to demonstrate the therapeutic potential of the ClyC-AH.
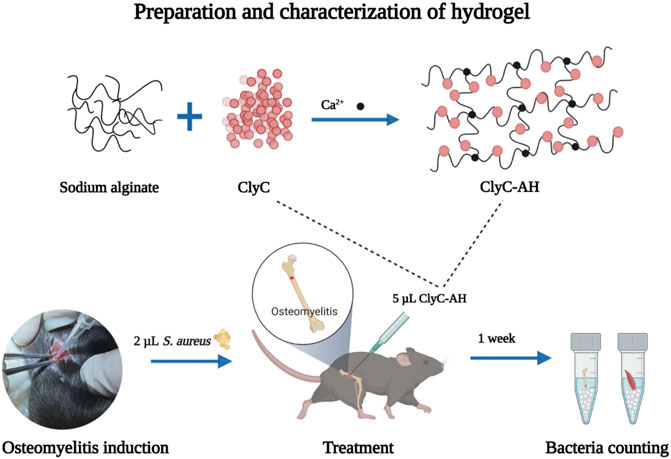
FIGURE 1. The overall experiment design of the study. 2 µL S. aureus was injected into the marrow cavity for creating an osteomyelitis model. A hydrogel loaded with ClyC was synthesized. ClyC would target and cleave the cell wall conserved domain, leading to lysis. ClyC resulted in infection reduction.
Materials and Methods
Preparation of ClyC-AH
The chimeric lysin, ClyC, was expressed as described previously (Li et al., 2021). Concentration of ClyC was determined by BCA assay and visualized by SDS-PAGE. The ClyC protein solution was freeze-dried and then stored at −80°C until use. An optimized ClyC-AH formulation was prepared using a one-step procedure at room temperature as proposed by Deng and Feng (Deng et al., 2015; Feng et al., 2020), with slight modification. Briefly, sodium alginate was dissolved in distilled water and magnetically stirred for 3 h until it completely dissolved. Freeze-dried ClyC was mixed with 0.68% calcium chloride solution (Hushi, Shanghai, China). Then, the mixture was added into 1.5% alginate solution at a ratio of 1:1 (v/v) and homogenized. The gelation time was measured by the inversion tube method at room temperature. In brief, 400 μL ClyC-AH was taken in a 1.5-ml Eppendorf tubes and incubated at room temperature. The gelation time was confirmed by inverting the tube every 5 s until the gel stopped flowing. Each solution was filtered separately through a sterile 0.22-μm membrane into a sterile container.
Scanning Electron Microscopy
Hydrogel alone and ClyC-AH were separately immersed in Tris buffer at room temperature until reached swelling equilibrium, cut into sections (10 mm × 7 mm × 5 mm). The samples were lyophilized using the LyoBeta 6 PL (Telstar, Spain) instrument, coated with gold (Hummer VI; Technic Inc., Anaheim, CA, United States). The microstructure of the sample was evaluated using the SEM (SU8010, Hitachi, Japan) at an accelerating voltage of 3 kv.
Cytotoxicity Test
The toxicity of ClyC released from hydrogel was evaluated using the CCK-8 assay. Alginate hydrogels with different ClyC concentrations (0, 31.25, 62.5, 125, 250, 500, and 1,000 μg/ml) were incubated in equal-volume Dulbecco’s modified Eagle’s medium (DMEM; Sigma, United States) at 37°C. After 24 and 48 h, the whole medium was collected. To investigate the cytotoxicity of the ClyC in the harvested medium, Baby hamster Syrian kidney (BHK)-21 cells were used. The cells were cultured in DMEM supplemented with 10% fetal bovine serum and 1% penicillin/streptomycin at 37°C under 5% CO2. BHK-21 cells were seeded into 96-well at a density of 1 × 104 cells/well. After 24 h, the cell culture medium was exchanged with the harvested medium (100 µL) and the same concentration of soluble ClyC as a control. After incubation for 24 h, the cell viability was measured by CCK-8 assay. The normal cell culture medium without ClyC was used as a positive control (PC), while medium without ClyC and BHK-21 cells served as a negative control (NC). The relative cell viability was determined by using the formula: %cytotoxicity = [1- (ODsample–ODNC)/(ODPC–ODNC)] × 100%.
Determination of the Amount of ClyC Released From Alginate Hydrogels
To investigate the release amount of ClyC from alginate hydrogels, the ClyC-AH (250 µg/ml, 400 µL) was incubated in Tris buffer (100 µL in 1.5-ml Eppendorf tubes) at 37°C. At predesignated time intervals (1, 3, 6, 10, 14, 24, 36, 48, and 72 h). At each timepoint, the supernatant was sampled, and the same volume of fresh Tris buffer was added. The amount of released ClyC from alginate hydrogels at each time point was determined using the BCA method and calculated based on the standard curve. The structural integrity of ClyC after release was examined using SDS-PAGE analysis. The ClyC released from hydrogel was suspended in SDS-PAGE loading buffer, destabilized by boiling for 10 min, processed and analyzed by SDS-PAGE, following standard protocol. Free native ClyC was also processed similarly and analyzed along with the test sample as a reference.
The Anti-Bacterial Activity Assays of ClyC Released From Alginate Hydrogels
The bacteria strain used in the study was S. aureus T23. The inoculum was prepared by culturing S.aureus T23 overnight in lysogeny broth (LB) medium with shaking at 37°C, washing three times in Tris buffer, by centrifugation, and adjusting the optical density at OD600 (Synergy H1). A preliminary assessment of the anti-bacterial effect of ClyC released from hydrogel was conducted via turbidity reduction experiment and inhibition zone method. For turbidity reduction experiment, the decrease of samples in OD600 was monitored by a Synergy H1 microplate reader every 1 min for 10 min at 37°C. For the inhibition zone method, 10 μL ClyC and ClyC-AH were spotted onto agar plates overlaid with T23 and incubated overnight at 37°C. Bactericidal activity was assessed by clear zones on the agar plates. Comparison of bactericidal activity of different timepoint sample was done by measuring colony count. Samples from each timepoint (50 µL) incubated with 50 µL T23 inoculum at 37°C for 1 h, serial dilution, and plating on lysogeny broth agar. Free ClyC solutions (125 μg/ml) incubated at 37°C were also prepared at each timepoint.
In vivo Treatment of Osteomyelitis With ClyC-AH
This study was approved by the Ethics Committee for Animal Research, Wuhan University, China (No. 2019A79). C57BL/6 mice were housed in 12-h light/dark cycles and freely provided food and water. The mice were assigned to four groups (bacteria infected, bacteria infected with hydrogel alone, bacteria infected with soluble ClyC alone, and bacteria infected with ClyC-AH groups; n = 5 or 6 per group). Briefly, after anesthetized, the skin on the right knee of mice was shaved and sterilized with 75% alcohol. A lateral incision was made, and the muscle was bluntly dissected to expose the femur. The proximal end of the femur was perforated using a high-speed drill with a 0.5-mma sterile round bur (Cassat and Skaar, 2013; Klopfenstein et al., 2021). T23 (3 × 106 cell CFU/mL in 2 μL of PBS) was inoculated into the medullary cavity of the femur using 10 μL-pipet tips and then injected 5 μL of different treatments. For animals receiving hydrogel alone, the hydrogel was pipetted over the hole to polymerize. For animals receiving soluble ClyC, the concentration of ClyC was equal to that of ClyC-AH. Then the wound was double-stitched by surgical silk suture.
After 1 week, all mice were sacrificed. The skin on the right hind leg was disinfected with 75% alcohol. After making a lateral incision, the femur and surrounding tissue were separated, weighed, and placed in 1.5-ml microcentrifuge tubes (with saline). Following dissection and removal, all samples were maintained on ice. The tissue and femur samples were subsequently homogenized using the MD100 by bead beating (5,000 rpm, 20 s) (NewZongKe, China) (Johnson et al., 2018). Water bath sonication was used to create single-cell bacterial suspensions after homogenization (sonicate 10 min, vortex 30 s). Homogenates were serially diluted, plated on Baird-Parker agar, and incubated overnight at 37°C. Colonies were acounted, normalized to sample weight, and transformed using the formula CFU = log10 (1 + X) to avoid negative values.
Statistics Analysis
Experimental data were expressed as mean ± standard deviation. Statistical analyses were conducted using Prism v 8.0.2 (GraphPad Software, United States). Statistical comparisons between two groups were made with Student’s t test. ANOVA for multivariate parametric data with a Tukey’s post hoc test or a Kruskal–Wallis test with Dunn’s post hoc test for nonparametric data. p-value of <0.05 was considered as statistically significant.
Result
Characterization of the ClyC-AH
ClyC-AH were prepared simply by mixing 1.5% alginate solution and 0.68% calcium chloride solution-containing ClyC, which was the most common method to prepare the hydrogel from the sodium alginate solution (Lee and Mooney, 2012). From a macroscopic view, there was no significant difference between ClyC-AH and alginate hydrogel without ClyC, which were both transparent and colorless (Figure 2A). The morphology of the samples was observed using SEM. The surface morphology of hydrogel alone and ClyC-AH was similar, which showed porous structure with good continuity. There were tiny pores observed in the structure which was thought to be beneficial for the sustain ClyC release (Figure 2C). The gelation time of ClyC-hydrogel was about 20 s, short but sufficient for injection into the lesion (Figure 2B). Meanwhile, it was conducive for the rapid fixation of ClyC-AH in the lesion.
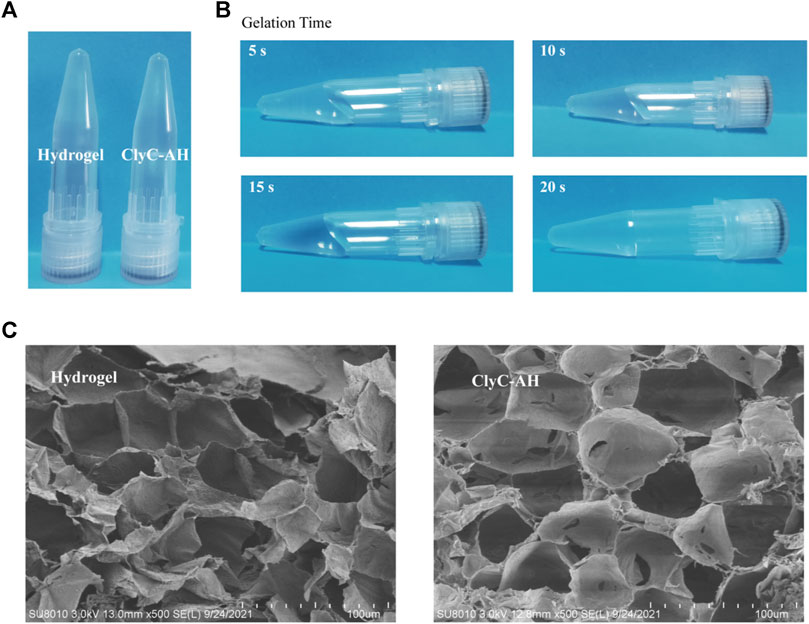
FIGURE 2. Characterization of the ClyC-AH. (A) Images of the hydrogel and ClyC-AH at room temperature; (B) Gelation time showing the solution-gel transition of ClyC-AH; (C) Surface morphologies of hydrogel and ClyC-AH by SEM.
ClyC-AH Reduced ClyC Cytotoxicity
Considering that high concentration of ClyC could cause cytotoxicity in the local infection, we loaded ClyC into alginate hydrogels. The results showed that the cytotoxicity of ClyC-AH was reduced compared with soluble ClyC (Figure 3). At 24 h after incubation, with the concentration of ClyC up to 250 μg/ml, the cytotoxicity of ClyC-AH showed no significant difference among different concentrations. Soluble ClyC alone had toxic effect on BHK-21 cells. Compared with soluble ClyC, ClyC-AH significantly improved cell viability. It could be explained by the slow release of ClyC from alginate hydrogels. The results suggested that the blank and loaded 250 μg/ml ClyC-AH were not cytotoxic to BHK-21 cells.
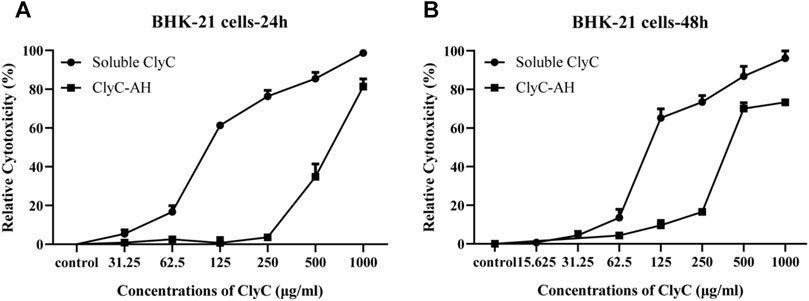
FIGURE 3. Cytotoxicity of ClyC-AH and free ClyC to BHK-21 cells. Firstly, DMEM incubated with ClyC-AH (0, 31.25, 62.5, 125, 250, 500, and 1,000 µg/ml) for 24 h (A) and 48 h (B). Then cells were co-cultured with incubated DMEM and different concentrations of free ClyC (0, 15.625, 31.25, 62.5, 125, 250, 500 and 1,000 µg/ml) for 24 h. The relative viability was calculated by the change of OD450 by CCK-8 assay.
In vitro Release of ClyC From Alginate Hydrogels
The amount of ClyC released from the alginate hydrogels are showed in Figure 4A. After 72 h, the cumulative released amount of ClyC from alginate hydrogels was approximately 23 ± 0.45%. To test the structural integrity of ClyC released from the alginate hydrogels, SDS-PAGE analysis was used and showed a single band corresponding to the molecular weight of free ClyC, as could be seen in Figure 4B. No additional band below the ClyC from the alginate hydrogels band, proving that ClyC was not hydrolyzed or degraded during the process of hydrogel gelation and release.
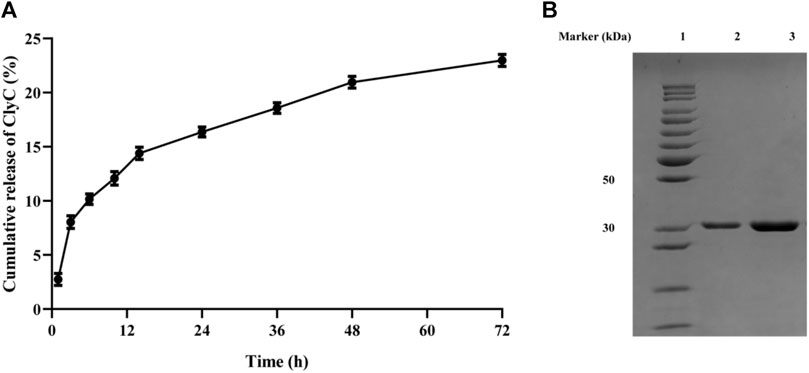
FIGURE 4. In vitro release of ClyC from hydrogel at 37°C in Tris buffer. (A) The curve of percent released ClyC at different time intervals. Mean ± SD, n = 3; (B) SDS-PAGE of free ClyC and ClyC released from hydrogel to determine the structural integrity of endolysin after encapsulation. Lane 1: Standard Protein Marker. Lane 2: ClyC released from the hydrogel. Lane 3: ClyC free native ClyC.
The Hydrogel Maintains ClyC Antibacterial Activity in vitro
As reported before, ClyC had highly anti-bacterial against S.aureus and showed enhanced bactericidal activity in the presence of calcium. In the turbidity reduction experiment, the OD600 values of T23 bacterial suspensions reduced rapidly (Figure 5A). In support, clear zones could also be seen in the plates after dipping ClyC and ClyC-AH on the lawn of S. aureus (Figure 5B), which showed that the released ClyC retained bactericidal activity. The bactericidal activity against S.aureus T23, by ClyC released from the hydrogel after different time intervals, was compared. The results showed that the ClyC released from hydrogel had high bactericidal activity within 24 h. After incubation in 37°C incubator for 24 h, ClyC could lead to a reduction of 2.89 log10 in viable cell numbers. Moreover, after incubation for 72 h, there was still a 1.14 log10 reduction in viable cells, indicating a therapeutic effect for acute infection (Figure 5C).
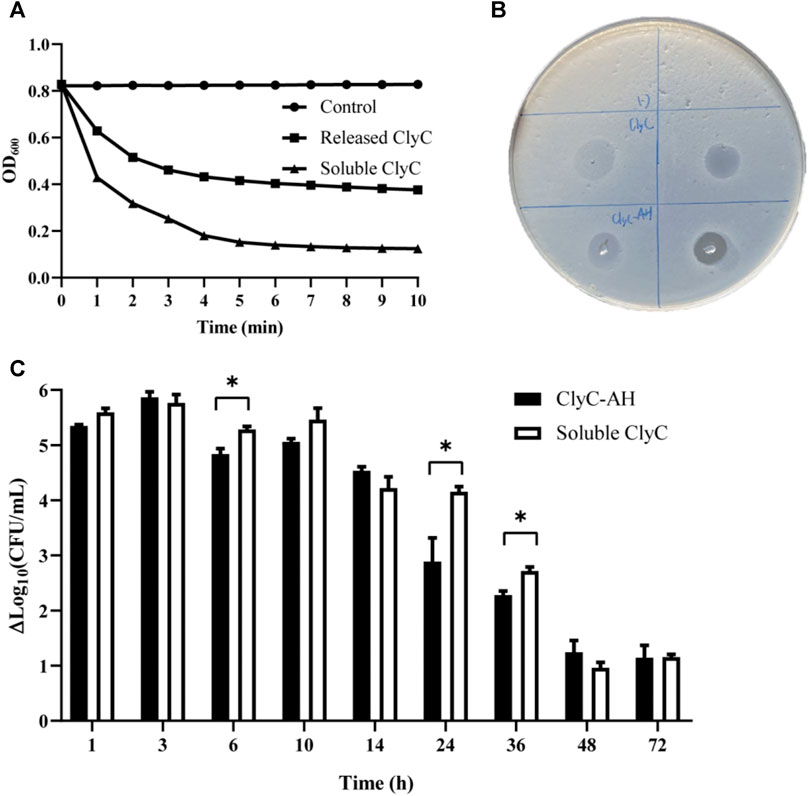
FIGURE 5. The bactericidal effect of blank hydrogel, ClyC-AH (250 µg/ml), and free ClyC (125 µg/ml) was quantified by incubating the S. aureus T23. (A) Turbidity reduction experiment, the lytic activity of ClyC and released ClyC after incubated 3 h in 37°C incubator; (B) inhibition zone assay result showing ClyC and ClyC-AH activity against S. aureus T23; (C) Briefly, S. aureus T23 cells at a cell density of approximately 108 CFU/ml were incubated with different time of sample for 1 h at 37°C. The lytic potential was assessed based on a decrease in bacterial log count compare with blank hydrogel after 1 h of treatment. And the calculated result of the reduction of log10 in viable cell number (ΔLog10 CFU/mL). Student’s t test, Mean ± SD, n = 3 per group, *p < 0.05.
In vivo Treatment of Osteomyelitis With ClyC-AH
The osteomyelitis mouse model was established to evaluate the ability of ClyC-AH to inhibit S. aureus infection in vivo. ClyC-AH was injected into the medullary cavity using 10 μL-pipet tips and subsequently transformed into hydrogels which allow stable injection into the cavity without leakage. The number of bacteria CFU in the femur and surrounding tissue was measured 7 days after surgery to evaluate the severity of osteomyelitis. Both the infection-only and hydrogel-only groups had high number of bacteria in the femur and surrounding tissue (Figure 6), indicating alginate hydrogels alone had no effect against S. aureus. The number of bacteria of the soluble ClyC group was significantly reduced in the surrounding tissue (Figure 6B). For the ClyC-AH group, the amount of bacteria was significantly reduced compared with the infection-only group or the hydrogel-only group both in the femur and surrounding tissue (Figure 6). In summary, these results demonstrated that ClyC-AH decreased S. aureus infections, the effect of which was comparable with that of soluble ClyC.
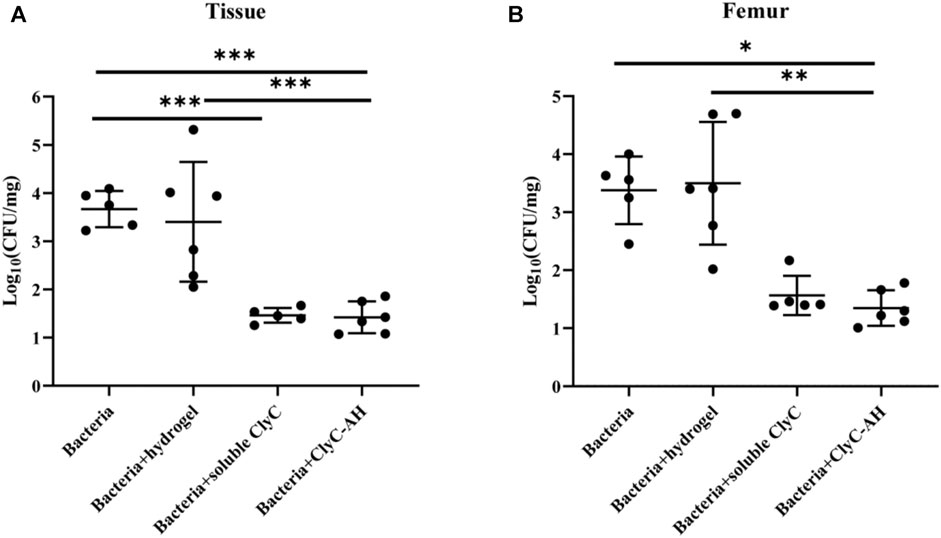
FIGURE 6. ClyC-AH reduced bacteria in the infected femur. After 7 days, S. aureus T23 recovery from (A) femur bone (B) tissue surrounding the femur. Error bars represent standard deviations. *p < 0.05, **p < 0.01, ***p < 0.001. Kruskal–Wallis test with Dunn’s multiple comparisons test for A. ANOVA with Tukey’s post hoc test for B. Mean ± SD, n = 5–6 per group.
Discussion
Bacterial infections associated with the outbreak of human diseases have developed into multidrug-resistant forms due to abuse of antibiotics, necessitating the research and development of new antibacterial agents (Otto, 2012). In this study, we loaded ClyC into the alginate hydrogel, which retained ClyC stability and activity while providing sustained release. Recently, some studies on the encapsulation of endolysin have been reported (Hathaway et al., 2015; Bai et al., 2019; Pinto et al., 2021). Chitosan nanoparticles loaded with the Cpl-1 pneumococcal endolysin showed low cytotoxicity to lung epithelial cell lines and reduced bacterial colonization in the lungs of mice (Gondil et al., 2020a). LysRODI encapsulated in pH-sensitive liposomes reduced S. aureus counts by 2log units upon incubation at pH 5 (Portilla et al., 2020).
The delivery system enhanced the bioavailability and safety of the encapsulated protein. In vitro release studies revealed that ClyC-AH exhibited biphasic release. There was an initial rapid release, followed by slow and sustained release, which could maintain the effective ClyC concentration and avoid the rapid degradation of ClyC in vivo. The CCK-8 assay also showed that the ClyC-AH exhibited low cytotoxicity, indicating biocompatibility of ClyC-AH.
It is important to stably encapsulate and deliver ClyC without any adverse effects on its structure and anti-bacterial activity. SDS-PAGE analysis determined the structural integrity of ClyC. It could be explained that proteins could be incorporated into alginate hydrogel under relatively mild conditions, which was also reported by Kaur (Kaur et al., 2020). The hydrogel retained the anti-bacterial activity of ClyC against S. aureus long-term in vitro. The anti-bacterial activity of ClyC released from ClyC-AH and ClyC solutions against S. aureus was not significantly different at most time points, but the lytic speed of ClyC released from ClyC-AH tends to be slightly slower. This result might be explained by the fewer amount of ClyC released from ClyC-AH at the beginning. Sodium alginate solution can be sol-gel converted by crosslinking some divalent cations (i.e., Ca2+). (Lee and Mooney, 2012). Interestingly, ClyC showed improved activity in the presence of calcium, with up to 9 Log10 S. aureus cell reduction within 20 min in the presence of Ca2+, which could explain the stable ClyC activity in the hydrogel.
There was no evidence of endolysin resistance, which was still a potential problem. Researchers had demonstrated a synergetic approach to bacterial treatment by a combination of endolysin and antibiotics (Daniel et al., 2010; Letrado et al., 2018). In our previous studies, we showed that penicillin G and ClyC had a synergistic effect, reducing the MIC of ClyC for planktonic and sessile S. aureus (Li et al., 2021). It was beneficial to reduce the working concentration of ClyC in the lesion and weaken potential safety hazards. Next, we will further optimize ClyC-AH by combining with antibiotics to broaden the bactericidal spectrum and decrease the working concentration of ClyC.
Furthermore, results of this study demonstrated antibacterial activity of ClyC-AH against S. aureus in vivo. In a S. aureus infected mouse model, ClyC-AH significantly reduced bacterial burden compared to the hydrogel-only group. In contrast, mice treated with hydrogel showed no differences in bacterial levels in the femur and surrounding tissue compared to the bacteria-infected control group. This demonstrated that ClyC had antibacterial activity against S. aureus infection. However, our results presented one-week observation of mouse osteomyelitis model treatment, which was not enough to make a detailed conclusion. There is a need to extend the experimental period to evaluate the effect of ClyC hydrogel on bone healing and local inflammation.
This study demonstrated that ClyC-AH was successfully prepared by crosslinking Ca2+, with no adverse effect on the structure of ClyC improve the anti-bacterial activity of ClyC. The delivery system could improve the biocompatibility, stability, and prolonged half-life of ClyC. Conclusively, ClyC-AH could be a promising candidate for prevention and treatment of infection caused by S. aureus.
Data Availability Statement
The raw data supporting the conclusions of this article will be made available by the authors, without undue reservation.
Ethics Statement
The animal study was reviewed and approved by the Ethics Committee for Animal Research, Wuhan University.
Author Contributions
YuL, QY and FY designed the project. FY, YiL and XW performed the experiments and analyzed the data. FY drafted the initial manuscript. All authors reviewed the manuscript and read the proof.
Funding
This work was supported by National Youth Natural Science Foundation of China (No. 81901045).
Conflict of Interest
The authors declare that the research was conducted in the absence of any commercial or financial relationships that could be construed as a potential conflict of interest.
Publisher’s Note
All claims expressed in this article are solely those of the authors and do not necessarily represent those of their affiliated organizations, or those of the publisher, the editors and the reviewers. Any product that may be evaluated in this article, or claim that may be made by its manufacturer, is not guaranteed or endorsed by the publisher.
References
Abdelrahman, F., Easwaran, M., Daramola, O. I., Ragab, S., Lynch, S., Oduselu, T. J., et al. (2021). Phage-Encoded Endolysins. Antibiotics 10 (2), 124. doi:10.3390/antibiotics10020124
Bai, J., Yang, E., Chang, P.-S., and Ryu, S. (2019). Preparation and Characterization of Endolysin-Containing Liposomes and Evaluation of Their Antimicrobial Activities against Gram-Negative Bacteria. Enzyme Microb. Tech. 128, 40–48. doi:10.1016/j.enzmictec.2019.05.006
Becker, R. E., and Bubeck Wardenburg, J. (2015). Staphylococcus aureus and the Skin: a Longstanding and Complex Interaction. Skinmed 13 (2), 111–120.
Cassat, J. E., and Skaar, E. P. (2013). Recent Advances in Experimental Models of Osteomyelitis. Expert Rev. Anti-infective Ther. 11 (12), 1263–1265. doi:10.1586/14787210.2013.858600
Conterno, L. O., and Turchi, M. D. (2013). Antibiotics for Treating Chronic Osteomyelitis in Adults. Cochrane Database Syst. Rev. 9, Cd004439. doi:10.1002/14651858.CD004439.pub3
Daniel, A., Euler, C., Collin, M., Chahales, P., Gorelick, K. J., and Fischetti, V. A. (2010). Synergism between a Novel Chimeric Lysin and Oxacillin Protects against Infection by Methicillin-Resistant Staphylococcus aureus. Antimicrob. Agents Chemother. 54 (4), 1603–1612. doi:10.1128/aac.01625-09
Davies, J., and Davies, D. (2010). Origins and Evolution of Antibiotic Resistance. Microbiol. Mol. Biol. Rev. 74 (3), 417–433. doi:10.1128/mmbr.00016-10
Deng, B., Shen, L., Wu, Y., Shen, Y., Ding, X., Lu, S., et al. (2015). Delivery of Alginate-Chitosan Hydrogel Promotes Endogenous Repair and Preserves Cardiac Function in Rats with Myocardial Infarction. J. Biomed. Mater. Res. 103 (3), 907–918. doi:10.1002/jbm.a.35232
Feng, J., Wu, Y., Chen, W., Li, J., Wang, X., Chen, Y., et al. (2020). Sustained Release of Bioactive IGF-1 from a Silk Fibroin Microsphere-Based Injectable Alginate Hydrogel for the Treatment of Myocardial Infarction. J. Mater. Chem. B 8 (2), 308–315. doi:10.1039/c9tb01971e
Fowler, V. G., Das, A. F., Lipka-Diamond, J., Schuch, R., Pomerantz, R., Jáuregui-Peredo, L., et al. (2020). Exebacase for Patients with Staphylococcus aureus Bloodstream Infection and Endocarditis. J. Clin. Invest. 130 (7), 3750–3760. doi:10.1172/jci136577
Gimza, B. D., and Cassat, J. E. (2021). Mechanisms of Antibiotic Failure during Staphylococcus aureus Osteomyelitis. Front. Immunol. 12, 638085. doi:10.3389/fimmu.2021.638085
Gondil, V. S., Dube, T., Panda, J. J., Yennamalli, R. M., Harjai, K., and Chhibber, S. (2020a). Comprehensive Evaluation of Chitosan Nanoparticle Based Phage Lysin Delivery System; a Novel Approach to Counter S. Pneumoniae Infections. Int. J. Pharmaceutics 573, 118850. doi:10.1016/j.ijpharm.2019.118850
Gondil, V. S., Harjai, K., and Chhibber, S. (2020b). Endolysins as Emerging Alternative Therapeutic Agents to Counter Drug-Resistant Infections. Int. J. Antimicrob. Agents 55 (2), 105844. doi:10.1016/j.ijantimicag.2019.11.001
Haddad Kashani, H., Schmelcher, M., Sabzalipoor, H., Seyed Hosseini, E., and Moniri, R. (2018). Recombinant Endolysins as Potential Therapeutics against Antibiotic-Resistant Staphylococcus aureus: Current Status of Research and Novel Delivery Strategies. Clin. Microbiol. Rev. 31 (1). doi:10.1128/cmr.00071-17
Hathaway, H., Alves, D. R., Bean, J., Esteban, P. P., Ouadi, K., Mark Sutton, J., et al. (2015). Poly(N-isopropylacrylamide-co-allylamine) (PNIPAM-Co-ALA) Nanospheres for the Thermally Triggered Release of Bacteriophage K. Eur. J. Pharmaceutics Biopharmaceutics 96, 437–441. doi:10.1016/j.ejpb.2015.09.013
Johnson, C. T., Wroe, J. A., Agarwal, R., Martin, K. E., Guldberg, R. E., Donlan, R. M., et al. (2018). Hydrogel Delivery of Lysostaphin Eliminates Orthopedic Implant Infection by Staphylococcus aureus and Supports Fracture Healing. Proc. Natl. Acad. Sci. USA 115 (22), E4960–e4969. doi:10.1073/pnas.1801013115
Jun, S. Y., Jang, I. J., Yoon, S., Jang, K., Yu, K.-S., Cho, J. Y., et al. (2017). Pharmacokinetics and Tolerance of the Phage Endolysin-Based Candidate Drug SAL200 after a Single Intravenous Administration Among Healthy Volunteers. Antimicrob. Agents Chemother. 61 (6), e02629. doi:10.1128/aac.02629-16
Kaur, J., Kour, A., Panda, J. J., Harjai, K., and Chhibber, S. (2020). Exploring Endolysin-Loaded Alginate-Chitosan Nanoparticles as Future Remedy for Staphylococcal Infections. AAPS PharmSciTech 21 (6), 233. doi:10.1208/s12249-020-01763-4
Klopfenstein, N., Cassat, J. E., Monteith, A., Miller, A., Drury, S., Skaar, E., et al. (2021). Murine Models for Staphylococcal Infection. Curr. Protoc. 1 (3), e52. doi:10.1002/cpz1.52
Kremers, H. M., Nwojo, M. E., Ransom, J. E., Wood-Wentz, C. M., Melton, L. J., and Huddleston, P. M. (2015). Trends in the Epidemiology of Osteomyelitis. J. Bone Jt. Surg. 97 (10), 837–845. doi:10.2106/jbjs.N.01350
Lee, K. Y., and Mooney, D. J. (2012). Alginate: Properties and Biomedical Applications. Prog. Polym. Sci. 37 (1), 106–126. doi:10.1016/j.progpolymsci.2011.06.003
Letrado, P., Corsini, B., Díez-Martínez, R., Bustamante, N., Yuste, J. E., and García, P. (2018). Bactericidal Synergism between Antibiotics and Phage Endolysin Cpl-711 to Kill Multidrug-Resistant Pneumococcus. Future Microbiol. 13 (11), 1215–1223. doi:10.2217/fmb-2018-0077
Lew, D. P., and Waldvogel, F. A. (2004). Osteomyelitis. The Lancet 364 (9431), 369–379. doi:10.1016/s0140-6736(04)16727-5
Li, X., Wang, S., Nyaruaba, R., Liu, H., Yang, H., and Wei, H. (2021). A Highly Active Chimeric Lysin with a Calcium-Enhanced Bactericidal Activity against Staphylococcus aureus In Vitro and In Vivo. Antibiotics 10 (4), 461. doi:10.3390/antibiotics10040461
Lindsay, J. A., and Holden, M. T. G. (2004). Staphylococcus aureus : Superbug, Super Genome? Trends Microbiol. 12 (8), 378–385. doi:10.1016/j.tim.2004.06.004
Lowy, F. D. (1998). Staphylococcus aureusInfections. N. Engl. J. Med. 339 (8), 520–532. doi:10.1056/nejm199808203390806
Maffulli, N., Papalia, R., Zampogna, B., Torre, G., Albo, E., and Denaro, V. (2016). The Management of Osteomyelitis in the Adult. The Surgeon 14 (6), 345–360. doi:10.1016/j.surge.2015.12.005
Munita, J. M., and Arias, C. A. (2016). Mechanisms of Antibiotic Resistance. Microbiol. Spectr. 4 (2), 481–511. doi:10.1128/microbiolspec.VMBF-0016-2015
Otto, M. (2012). MRSA Virulence and Spread. Cell Microbiol 14 (10), 1513–1521. doi:10.1111/j.1462-5822.2012.01832.x
Pantosti, A., Sanchini, A., and Monaco, M. (2007). Mechanisms of Antibiotic Resistance in Staphylococcus aureus. Future Microbiol. 2 (3), 323–334. doi:10.2217/17460913.2.3.323
Pinto, A. M., Silva, M. D., Pastrana, L. M., Bañobre-López, M., and Sillankorva, S. (2021). The Clinical Path to Deliver Encapsulated Phages and Lysins. FEMS Microbiol. Rev. 19, 1–29. doi:10.1093/femsre/fuab019
Portilla, S., Fernández, L., Gutiérrez, D., Rodríguez, A., and García, P. (2020). Encapsulation of the Antistaphylococcal Endolysin LysRODI in pH-Sensitive Liposomes. Antibiotics 9 (5), 242. doi:10.3390/antibiotics9050242
Pugliese, G., and Favero, M. S. (2003). Outbreaks of Community-Associated Methicillin-Resistant Staphylococcus aureus Skin Infections. Infect. Control. Hosp. Epidemiol. 24 (10), 787. doi:10.1086/infeconthospepid.24.10.787
Resch, G., Moreillon, P., and Fischetti, V. A. (2011). A Stable Phage Lysin (Cpl-1) Dimer with Increased Antipneumococcal Activity and Decreased Plasma Clearance. Int. J. Antimicrob. Agents 38 (6), 516–521. doi:10.1016/j.ijantimicag.2011.08.009
Ruvinov, E., and Cohen, S. (2016). Alginate Biomaterial for the Treatment of Myocardial Infarction: Progress, Translational Strategies, and Clinical Outlook. Adv. Drug Deliv. Rev. 96, 54–76. doi:10.1016/j.addr.2015.04.021
Seijsing, J., Sobieraj, A. M., Keller, N., Shen, Y., Zinkernagel, A. S., Loessner, M. J., et al. (2018). Improved Biodistribution and Extended Serum Half-Life of a Bacteriophage Endolysin by Albumin Binding Domain Fusion. Front. Microbiol. 9, 2927. doi:10.3389/fmicb.2018.02927
Urish, K. L., and Cassat, J. E. (2020). Staphylococcus aureus Osteomyelitis: Bone, Bugs, and Surgery. Infect. Immun. 88 (7), e00932. doi:10.1128/iai.00932-19
Keywords: Staphylococcus aureus, MRSA, osteomyelitis, anti-bacterial activity, bacteriophage lysin, alginate hydrogel
Citation: Yao F, Wu X, Liao Y, Yan Q and Li Y (2021) Smart Chimeric Lysin ClyC Loaded Alginate Hydrogel Reduces Staphylococcus aureus Induced Bone Infection. Front. Mater. 8:763297. doi: 10.3389/fmats.2021.763297
Received: 23 August 2021; Accepted: 04 October 2021;
Published: 22 October 2021.
Edited by:
Yijing Liu, Huazhong University of Science and Technology, ChinaReviewed by:
Qianqian Liu, Wuhan Institute of Technology, ChinaQianqian Ni, Nanjing University, China
Copyright © 2021 Yao, Wu, Liao, Yan and Li. This is an open-access article distributed under the terms of the Creative Commons Attribution License (CC BY). The use, distribution or reproduction in other forums is permitted, provided the original author(s) and the copyright owner(s) are credited and that the original publication in this journal is cited, in accordance with accepted academic practice. No use, distribution or reproduction is permitted which does not comply with these terms.
*Correspondence: Qi Yan, eWFucWlAd2h1LmVkdS5jbg==; Yuhong Li, MTAwNDgwOTM3MkB3aHUuZWR1LmNu
†These authors have contributed equally to this work