- 1Department of Agricultural Sciences, University of Naples Federico II, Portici, Italy
- 2Department of Pharmacy, University of Salerno, Fisciano, Italy
- 3Interdepartmental Research Center for Biomaterials at the University of Salerno, Fisciano, Italy
Zinc (II) cation is an environmentally friendly metal, less expensive, easy to dispose of, and managed. Highly engineered symmetric systems can be built using zinc (II) atoms as the metal nodes of hybrid organic-inorganic supramolecular structures. In biological contexts, luminescent zinc-based nanoprobes are in growing demand. Specifically, they are currently employed to detect biologically and environmentally relevant analytes, in therapeutic drug delivery, and for bioimaging and diagnostic techniques monitoring aspects of cellular functions. This review will provide a systematic and consequential approach to zinc-based nanoprobes, including zinc-based MOFs and other zinc-based organized nanoparticles. A progression from detecting the biological target to the intracellular sensing/marking/carriage has been followed. A selection of significant cutting-edge articles collected in the last five years has been discussed, based on the structural pattern and sensing performance, with special notice to living cell bioimaging as the most targeted and desirable application.
Introduction
The term nanoprobe refers generically to any chemical or biological technique that deals with nano-quantities. A nanoparticle is defined as a particle of matter between 1 and 100 nm in diameter, sometimes larger (up to 500 nm) in only two directions. Chemists and biochemists define fluorescence “nanoprobes” as the nanosized materials able to provide a fluorescence response in the presence of a specific substrate or analyte. Responsive nanosized materials, even multi-purpose designed, are currently employed to detect biologically and environmentally relevant analytes in therapeutic drug delivery and bioimaging and diagnostic techniques. Due to the size of the particles, nanoprobes provide a permeation function in intracellular compartments or living cell micro-environments.
In recent years, researchers were inspired by the variety of structures and behaviors of low molecular weight complexes (Caruso et al., 2009; Caruso et al., 2011; Caruso et al., 2013; Quintard and Rodriguez, 2014; Abu-Dief and Mohamed, 2015; Panunzi et al., 2018b) in designing and developing novel organic-inorganic hybrid materials with targeted properties (Chen et al., 2000; Hosomi et al., 2016; Diana et al., 2019a; Diana et al., 2019b; Diana et al., 2019c; Dey et al., 2019; Liu et al., 2020). The materials named coordination polymers (CPs) are hybrid organic-inorganic supramolecular structures self-assembled from metal nodes and/or metal clusters (metal particles smaller than 1 nm) and organic ligands. Highly engineered symmetric systems can be built using metal nodes, organic spacers, and even solvent molecules by repeating the coordination frame in one, two, or three dimensions. Metal-organic frameworks (MOFs) are a subgroup of the CPs class, with tridimensional crystalline regularity producing specific nano- (<2 nm) or meso (2–50 nm) porous structures. Their voids are functional cavities that can be selectively exploited. Due to the unique structural ductility, such nano-assembled compounds encompass one of the most versatile classes of hybrid materials, causing a dramatic impact on the scientific literature. Endless combination possibilities, limitless structures and functionalities are obtained by the deliberate design and selection of organic and inorganic units (Borbone et al., 2015).
CPs and/or MOFs nanoparticles, micelles, supramolecular nano-assemblies, polymer vesicles are some of the most important macromolecular nano-assemblies (Panunzi et al., 2019a). Their functional properties include fluid storage (Lin et al., 2017; Tsivadze et al., 2019) and separation (Barnett et al., 2019; Iacomi et al., 2019; Lin et al., 2020), water purification (Zhao X. et al., 2018; Gao Q. et al., 2019; Li J. et al., 2020), catalysis (Zhang Y. et al., 2018; Aljammal et al., 2019; Kang et al., 2019), electrochemical (Landi et al., 2014; Wang D.-G. et al., 2020; Xie X.-X. et al., 2020) biological and medical applications (Chowdhury, 2017; Zhuang et al., 2017; Cui et al., 2018; Cai et al., 2019), energy conversion (Zak et al., 2017; Kaur et al., 2019; Griffin and Champness, 2020; Liu et al., 2020). Finally, nanosized CPs showed considerable potential in luminescence sensing and monitoring (Kempahanumakkagari et al., 2018; Griffin and Champness, 2020; Marchenko et al., 2020). Nanoprobes that exhibit luminescence properties are the perfect candidates for monitoring biological parameters, bio-analytes, environmental pollutants, and manufacturing biosensors and markers for living cells. Compared to other recent reviews in the literature (Yang et al., 2019; Liu et al., 2020), this review presents an overview of the most relevant examples from the last 5 years of Zn-MOFs designed for metals, ions and small molecules with biological and environmental relevance, and Zn-MOFs and Zn-NPs targeted for living cell imaging and drug vectors. A selection of innovative and significant articles has been discussed, based on the structural model and sensing performance, with emphasis on living cell bioimaging.
Luminescent Nanomaterials for Biological Target Sensing
Luminescence is a phenomenon involving spontaneous emission of radiation from an excited state generated by photonic excitation, by chemical/electrochemical reactions, or by applying mechanical stress. The mechanism involves electronic transitions from the ground to an excited state by absorption of a photon, followed by a return to the ground state with the resultant emission of a photon. Radiative or non-radiative relaxation processes can be involved. In the fluorescence mechanism, a transition occurs from the singlet excited state (S1) to the ground state (S0) with a short-excited state lifetime. In the phosphorescence mechanism, intersystem crossing (ISC) occurs from the singlet excited (S1) to the excited triplet state (T1). The forbidden photon-emitting transition to the ground state (S0) has a long-excited state lifetime.
A biosensor must, by definition, include some biomolecular interaction able to give rise to an output signal. Fluorescent dyes have been the most widely used in bio-applications, due to their structures with delocalized electrons and even aromatic rings. The energy gap between the lowest unoccupied molecular orbital (LUMO) and the highest occupied molecular orbital (HOMO) is mainly in the visible light range. Traditional fluorescent organic dyes were used in living cells (Jun et al., 2012; Herpoldt et al., 2017; Kim et al., 2018) with some disadvantages such as photobleaching, low brightness, and scarce permeability. Biology and nanotechnology both inhabiting the same length scale are designed to move synergically, but the properties of the material can be dramatically altered when one or more dimensions are reduced to the nanoscale.
Among the many methods developed for the sensitive detection of biological molecules, luminescence signal detection has advantageous properties over other methods. From a synthetic point of view, the PL response can be preserved and even enhanced judiciously turning the macro-scaled into nano-scaled probes. On the other hand, the easy-to-operate PL signal guarantee a low expansive, real-time, in situ analysis. Finally, the mimic behavior of the nanoprobes into the living cell benefits from the non-invasiveness proper of the biomolecules (Liu et al., 2021). As will be discussed in the next section, the tailored composition of nanosized hybrid probes can represent a value-added in the design of the new generation of PL smart tools.
Luminescence in Hybrid Supramolecular Materials
Density function theory (DFT), as a theoretical method for multi-electron system calculation (Gentile et al., 2018; Tawfik et al., 2019; Schira and Latouche, 2020), has been successfully applied in predicting the nature of luminescence also in hybrid materials (Chai et al., 2012; Causa et al., 2013; Işık Büyükekşi et al., 2019; Di Palma et al., 2020). In this case, the complex mechanism of luminescence involves metal and/or ligand-centered transitions. Emission can arise from metal-centered (MC) transitions due to d and f orbitals of the metal, ligand-centered (LC) transitions due to the organic frameworks, charge transfer processes including ligand-to-metal charge transfer (LMCT), metal-to ligand charge transfer (MLCT), ligand-to-ligand charge transfer (LLCT), and metal-to-metal charge transfer (MMCT) (Lamansky et al., 2001; Castellano et al., 2006; You and Nam, 2012; Quah et al., 2015; Jia et al., 2016; Alam et al., 2019).
Luminescence can be achieved by selecting metal cations and organic molecular frameworks coordinated to the metal centers and entangled solvents. A luminescence response is expected to form the complex because of the interaction of metal ions (or clusters) and conjugated ligand moieties. On the other hand, sensing ability arises from the presence of guest molecules which establish analyte-probe interactions due to sites present in the ligand. Sensing involves alteration in the luminescence pattern, affecting the maximum and/or intensity of the emission band of the organic frameworks. In sensing applications, supramolecular interactions of the probe with the analyte could provide a gradual enhancement or quenching in the emission or a luminescence turn-off/turn-on pattern. Luminescence sensing takes place through different mechanistic pathways, mostly Förster resonance energy transfer (FRET), Dexter electron exchange (DEE) process and photo-induced electron transfer (PET) (Lakowicz, 2013; Nakabayashi and Ohta, 2015; Steinegger et al., 2020). FRET is a non-radiative energy transfer from a donor to an acceptor molecule with the effect of fluorescence reduction of the donor and enhancement of the acceptor; DEE involves quenching of donor’s fluorescence by the transmission of excited electron of the donor molecule to acceptor molecule via non-radiative pathway; PET is similarly a quenching process due to transmission of an excited electron from donor to acceptor molecule via a non-radiative pathway. In Figure 1 is shown an example of upconversion efficiency enhanced by FRET within host–guest metal–organic frameworks consisting of encapsulated high quantum yielding guest molecules. The structures reported in all the figures have been readapted using Mercury software (Macrae et al., 2020).
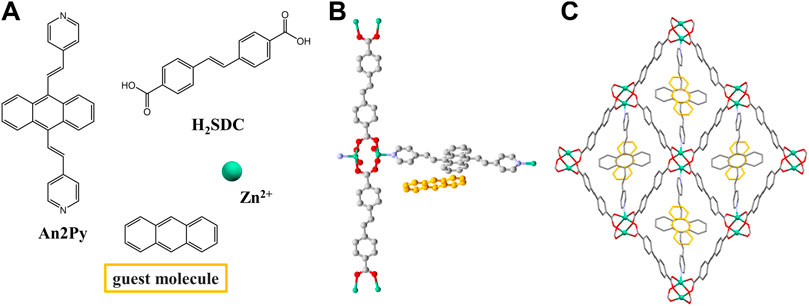
FIGURE 1. (A) Chemical structure of the ligand trans, trans-9,10-bis(4-pyridylethenyl)anthracene (An2Py), trans,trans-4,4′ stilbenedicarboxylic acid and anthracene. (B) Coordination environment of An2Py (C) a view of MOF containing the ligand with encapsulated anthracene (orange) guest along axis a. Readapted from (Quah et al., 2015).
Luminescence can be due to conformational and electronic changes of the organic part; in addition, the metal centers can claim a role. Metal-centered electronic transitions are involved in emission properties, mostly with lanthanide and actinide metal cations, which show weak f–f transitions. On the other hand, other transition metals can be utilized in the synthesis of hybrid luminescent compounds. Strong coordination ability and variable coordination number and geometries are desirable properties of many complexes of first-row transition metals. Among them, closed-shell metal ions with d0 or d10 electron configurations play a special role due to the deactivation of d-d transitions.
In constructing complexes and aggregates based on the closed-shell zinc (II) cation, the emission is mainly due to LC transitions and/or to LLC transitions. Because zinc (II) ion has no optical signature due to the absence of d–d electronic transition, LMCT are rare and involve s or p empty orbitals of the metal (Christie, 1994). However, even in poorly emissive ligands, fluorescence enhancement can be achieved upon zinc (II) coordination to stabilize the excited state (Kwok et al., 2015) through a conformational constrain of the organic part. Furthermore, zinc (II) cation often causes a CHEF (chelation enhanced fluorescence) (Hancock, 2013; Panunzi et al., 2018a; Diana et al., 2018; Patra et al., 2018; Panunzi et al., 2019b) effect in the ligands, and therefore it is a valid building block in the building of highly emissive architectures.
In addition to the great variability of structures and a convenient optical pattern, zinc (II) is an environmentally friendly metal. As opposed to rare earth metals, zinc is less expensive, easy to dispose of, and managed. For this reason, “green” zinc (II) metal cation meets the sustainability requirements that are in growing demand in biological contexts.
From Zinc-Based Coordination Polymers to Nanoparticles: The Aim and the Scope of the Review
Metal ions are present everywhere in living or non-living species so that they play significant roles in many environmental, medical, and industrial purposes. Some metals are essential to chemical, enzymatic, biological processes. Some of them cause health risks and hazards. Other small molecules, such as H2S, sulfur oxides, carbon oxides, chromates and dichromates, NH3, nitrogen oxides, methane, nitroaromatic compounds, volatile organic compounds (VOCs, as chlorofluorocarbons, hydrocarbons, fluorocarbons), can be dispersed in air and/or in water as common toxic and hazardous pollutants. Moreover, the specific detection of bio-relevant molecules such as trichloroacetic acid, antibiotics, histidine, or cellular pH and temperature parameters is a diagnostic tool.
The development of chemosensors selectively trapping environmental and biological relevant analytes have some definite advantages over conventional time-consuming and expensive spectrophotometric and electroanalytical techniques. Specifically, luminescence sensing offers simple naked-eye perception and real-time responses so that fluorescence chemo and bio-sensing is a rapidly growing field. Many macromolecular hybrid sensing systems have been reported in recent years; among them, several examples of responsive CPs have been explored. From the first work in 1997 (Petrochenkova et al., 1997) to recent years, more than 4,000 works have been published in CPs. The construction of CPs has been a hot topic in sensing of anions, metal cations (Zhao S.-N. et al., 2018; Qu et al., 2018; Pamei and Puzari, 2019; Xie S. et al., 2020; Wang Y. et al., 2020; Razavi and Morsali, 2020), organic solvents (Li and Song, 2011; Xiao et al., 2015; Roales et al., 2017; Kustov et al., 2019; Wang et al., 2019) and gases (Liu et al., 2018), present in environmental and industrial samples, and for medical and biological applications. CPs are rightly placed among the emerging luminescence sensors for common pollutants and biological targets due to the highly modulable structural and optical features, predictable mechanisms, and selective responses. In addition, the use of zinc (II) d10 metal nodes guarantee eco-compatibility and low-cost.
In the last 5 years, macro-sized Zn (II) based CPs were found to be successfully employable in sensing metals, small organic molecules, anions, and cations (Jia et al., 2016; Park et al., 2016; Gu et al., 2017; Zhang et al., 2017; Fan et al., 2020a). Lessons learned from the mono and bi-dimensional macroscopic tools aid the construction of highly engineered three-dimensional nano-architectures, able to cell permeation. MOFs and other nanosized bioengineered probes, highly symmetric structures held by supramolecular interactions, are designed to detect metals and small molecules, carry drugs for cancer therapy, and monitor/mark specific cells or subcellular parameters (Kang et al., 2017). They can take advantage of the employ of the eco-friendly and versatile zinc (II) ion in their architectures. Zinc-based nanoprobes are among the recent advanced tools for sensing of biological relevant entities and parameters and for understanding cellular functions in medical diagnostic. The structural versatility of the zinc coordination core can be exploited in building tailored nanosized architecture. On the other hand, the desired PL properties of the nano-tools can be modulated by the optically innocent “clip approach” (Diana and Panunzi, 2021) due to zinc cation. Finally, in the live observations cells and tissues can be both deeply penetrated and preserved thanks to the small non-toxic zinc ion.
This review will provide a systematic and consequential approach to the zinc-based nanoprobes, including zinc-based MOFs (abbreviated Zn-MOFs) and other zinc-based nanoparticles (abbreviated Zn-NPs), The progression of the review will start from the targeted biological systems pursuing the nanosized intracellular sensors/markers/carriers. A selection of significant cutting-edge articles about zinc (II) nanoprobes collected in the last 5 years will be discussed, based on their structural pattern and sensing performance. Synthetic novelty, study completeness, selective response, symmetric pattern, and theoretical deepening will be underlined, with special notice to living cell bioimaging as the most targeted and desirable application.
We review the literature organizing the discussion in the following sections:
Zn-MOFs for biological targets and environmental pollutants sensing;
Zn-MOFs and other Zn-NPs for living cell determinations.
Our final aim will be to highlight the recent challenges and goals in luminescent zinc nanoprobes, bringing the layman closer to this topic and stimulating the research to novel working hypotheses.
Zinc-Metal-Organic Frameworks for Biological Targets Sensing
Due to their unique porous crystalline structure, high surface area, and versatile functionalities, MOFs are considered ideal candidates for selective sensing (Fan et al., 2020b; Roy et al., 2020; Lei et al., 2021; Li et al., 2021).
The sensing properties can be tuned depending on the binding affinity of the metal cation, the design and chelation ability of the linker, the number and size of available open sites, the coordination pattern, the symmetry and the crystalline arrangement, the potential interactive free sites. The common design idea in the construction of LMOFs is the employ of rigid organic ligands with a π-conjugated system and specific functions. The formation of the MOF architecture involves metal-ligand bonds able to produce a rigid structure undergoing a decrease in the vibration and rotation of the ligands. The resulting non-radiative relaxation and decay improve the emission.
In designing a fluorescence sensor, attention must be paid to the host-guest interactions and the electronic structure of the host frames. The symmetry of the scaffold and the introduction of specific functions leads to selective sensing properties. On the other hand, the nature of the metal ions should be generally considered, as it affects both the chemo and physical properties of the sensor and the sensing specificity and mechanism.
The fluorescence sensing by LMOFs can result in fluorescence enhancement or quenching due to the molecular interactions between the LMOFs and analytes. In some cases, the detected target analyte interferes with the ligand’s electronic structure, thereby reducing the energy transfer from the ligand to the central metal ions. Therefore, the sensing process results in a decrease in fluorescence intensity. In other cases, a fluorescence enhancement can be obtained due to the activation of an emissive channel in bonding the target analyte. In this case, the sensing process involves originally weak emissive MOFs, which become strong emissive LMOFs after detection.
The zinc (II) d10 cation is a “unique individual” in the MOFs building. When employed as a metal node of LMOFs, zinc (II) ion can both retain the fluorescence of the linkers or activate their emission channel, easily affording strong luminescent LMOFs due to constraining effect on the ligands (CHEF). In the closed-shell cation, the lowest energy excited states are mainly LCT (ligand charge transfer) transitions and/or LL'CT (ligand to ligand charge transfer) transitions. Thus, zinc ions can freeze a favourable emissive configuration of the ligand, with scarce electronic contributes. Due to its wide range of architecture, porosity, and functionality, Zn-MOFs can represent unique LMOFs, and potential candidates in the fields of storage, drug delivery, and sensing (Jiang et al., 2017; Zhao et al., 2019).
In Zinc-Metal-Organic Frameworks for Biological Targets Sensing, we will report the most relevant examples of novel Zn-MOFs used as sensors for biological targets. Specifically, iron and other metal cations, both essential for life (as Cu2+) and toxic/mutagens (as Cd2+), hazardous analytes such as Cr (VI) oxides and nitroaromatic compounds, environmental organic pollutants, such as organic dyes, pesticides, antibiotics, and finally analytical parameter such as pH have been considered in this section. Our discussion will be articulated based on structural similarities and differences in the targeted analytes and the sensing mode. The most representative structures have been reported in Figures 2, 3.
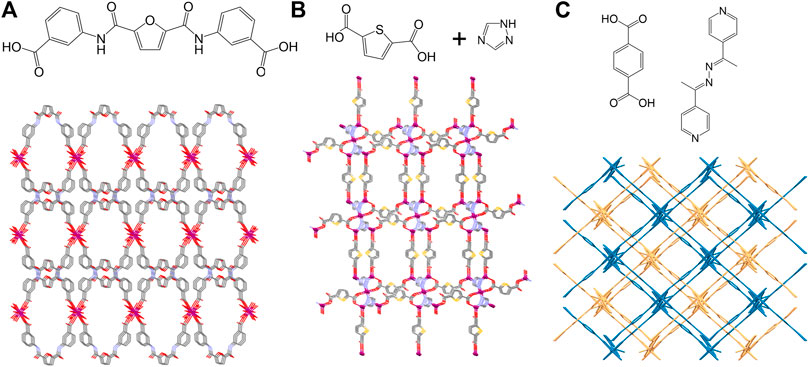
FIGURE 2. Some representative structures of Zn-MOFs for biologically and environmentally relevant analytes. (A) Ligand and 3D structure of Zn (II) MOF BIPT-1 viewed along the c axis, form ref (Qu et al., 2018). (B) Ligands and 3D framework of Zn (II) MOF along the a axis, form ref (Zou et al., 2018). (C) Ligands and the two-fold interpenetration TMU-16, form ref (Farahani and Safarifard, 2019).
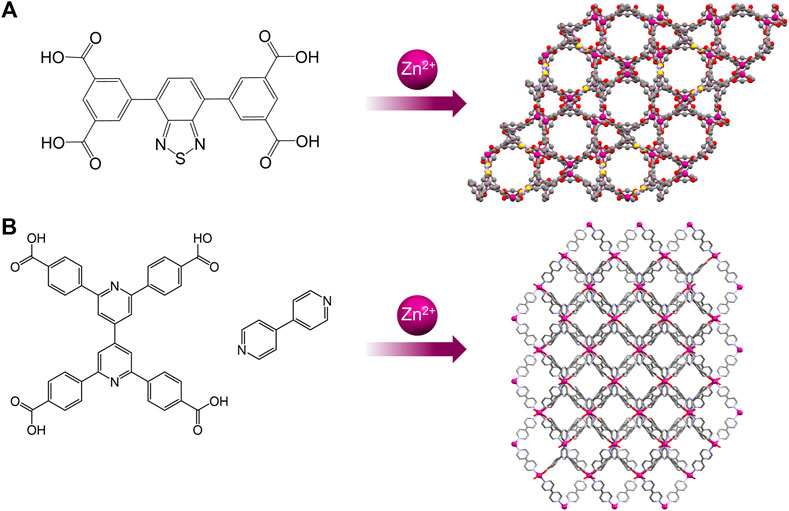
FIGURE 3. Some representative structures of Zn-MOFs for pH and temperature measurement. (A) H4L tetracarboxylic acid ligand and the MOF ZJU-21 framework viewed along the c direction, from ref (Zhao et al., 2017); (B) ligands and 3D framework representation of the Zn MOF viewed along the c direction, form ref (Yang et al., 2020).
Iron cation is a relevant biological target. As an essential trace element in the human body, it plays a crucial role in biological processes such as oxygen transport, cell metabolism, and electron transfer processes in DNA and RNA syntheses. In the same year (2018), two researchers groups produced two different LMOFs for the detection of iron. Huan Yang and coworkers (Wang et al., 2018) produced a one-dimensional zinc MOF based on 5-(5-norbonene-2,3-dicarboximide) isophthalic acid) with encapsulated Tb3+ and Eu3+ cations. The composite luminescent (Tb3+)@Zn-MOF undergoes selective and linear luminescence quenching effect upon addition of Fe(III) cation and low detection limit (LOD = 7.5 × 10−6 M). Analogously, a selective and reversible quenching response to Fe (III) cation was obtained by employing a LMOF based on Li+ and Zn2+ and the quadridentate carboxylate ligand 5-[bis(4-carboxybenzyl)amino]isophthalic acid by Guangshan Zhu and coworkers (Ren et al., 2018).
Many cases are known of Zn MOFs for simultaneous detection of iron and other analytes. In some cases, the other analyte is a metal cation. In 2017 Xin Liu and coworkers (Lv et al., 2017) produced a Zn LMOF based on TPOM = tetrakis (4-pyridyloxymethylene) methane and H2ndc = 2,6-naphthalenedicarboxylic acid with Eu3+ encapsulated cations, able to detect Fe(III) and Cr(VI) ions with high selectivity, scarce interference, and short response time. In 2018, Xia Li and coworkers (Zhang M.-N. et al., 2018) assembled three MOFs based on Zn(II), Cd(II) and Cu(II) and the ligands dpdc = 3,3′-diphenyldicarboxylate and btb = 1,4-bis(1,2,4-triazol-1-yl)butane. The Zn MOF can act as a bi-functional chemosensor for Fe3+ and Al3+ ions. In 2019 Vahid Safarifard and coworkers (Farahani and Safarifard, 2019) synthesized a bipyridyl ligand containing a bridging azine group to fabricate the Zn MOF named TMU-16. The MOF displays high luminescence emission and can detect Fe(III) (quenching response) and Cd(II) (enhancement response) ions with high selectivity and sensitivity (LOD = 0.2 and 0.5 μM, respectively) and short response time (<1 min). The MOF also gives a luminescence response toward a small molecule such as CH2Cl2. Wei-Yin Sun and coworkers in 2018 (Liu et al., 2018) presented six MOFs):[Cd (L1) (oba)]·DMF (1), [Ni3(L1)2(BPT)2(H2O)4] (2), [Zn2 (L1)2-(HBPT)2]·H2O (3), [Ni(L1) (BPTC)0.5(H2O)2] (4), [Ni2(μ2-O) (L2)3(Hoba)2-(H2O)2] (5), and [Ni2(L2)3(BPTC) (H2O)2]·6H2O (6) [H2oba = 4,4’-oxybis-(benzoic acid), H3BPT = biphenyl-3,4’,5-tricarboxylic acid, H4BPTC = biphenyl-3,3’,5,5’-tetracarboxylic acid, DMF = N,N-dimethylformamide] derived from 1H-imidazol-4-yl-containing ligands 1,3-di (1H-imidazol-4-yl)benzene (L1) and 4,4’-di (1H-imidazol-4-yl)biphenyl (L2) with varied carboxylate ligands. Zn MOF (3) shows highly efficient quenching for detecting Fe (III) cation and acetone molecules.
Several other efficient zinc-based LMOFs for the detection of small hazardous analytes are known. Specifically, Cr2O72-, CrO42-, and nitroaromatic compounds are potent pollutants of aqueous solutions, widely used in industrial applications and highly carcinogenic (Ostrowski et al., 1999; Levina et al., 2005; Zhitkovich, 2011). The ability of LMOFs to detect hazardous analytes can be precious. Several recent examples of Zn MOFs detecting nitro derivatives are known. Ming Ho and coworkers in 2017 (Zhang et al., 2017) produced a series of chiral 3d-4f heterometallic interpenetrating Zn MOFs based on a multidentate terpyridyl carboxylic acid ligand. The Eu (III) based compound proved to be a luminescent sensor to detect nitrobenzene molecules with high sensitivity. Xiangyang Qin and coworkers in 2019 (Lu et al., 2019) prepared a Zn MOF using benzene-1,3-dicarboxylic acid (H2BDC) and 1,4-bis(2-methyl-imidazole-yl)-propane (bip) which behaves as a sensitive luminescent probe for quenching detection of nitroaromatics (NACs), especially 2,4,6-trinitrophenol (TNP). In the same year, T. Thonhauser and coworkers (Jensen et al., 2019) examined the quenching mechanism undergoing a Zn2 (bpdc)2 (bpee) MOF, (bpdc = 4,40-biphenyldicarboxylate; bpee = 1,2-bipyridylethene), upon nitroaromatic molecule adsorption by ab initio simulation. Sheng-Yong You and coworkers in 2020 (Li L. et al., 2020) obtained a two-fold interpenetrating pillar-layer Zn MOF from thiophene-2,5-dicarboxylic acid (H2tda) and 4,4’-azobispyridine (azopy) as a chemosensor for nitroaniline detection. The fluorescence resonance energy transfer (FRET) process resulted in dominating the luminescence quenching response of the MOF.
Multifunctional MOF sensors able to selectively detect both biologically relevant and hazardous analytes can be versatile tools. For example, in several cases, iron can be detected by multiple function Zn MOFs together with nitroaromatic compounds or/and Cr2O72- and CrO42- anions.
Xiao Zhang and co-workers in 2019 (Zhuang et al., 2019) synthesized a Zn MOF with a 3-fold interpenetrating 3D framework based on t pt = 2,4,6-tri (pyridin-4-yl)-1,3,5-triazine and H2tda = 2,5-thiophene dicarboxylic acid acting as a very sensitive probe for Fe(III) ions and 2,4,6-Trinitriphenol detection by fluorescence quenching behavior and visible color change in the presence of iron. Very recently, Lirong Yang and coworkers (Yang et al., 2021) reported three MOFs by hydrothermal reactions with 440,400-nitrilotribenzoic acid (H3NTB), 1,10-phenanthroline (phen) and Zn(II), Mn(II) and Cu(II) ions, respectively. The Zn-MOF exhibits a dual fluorescent response toward nitrobenzene and Fe(III) ion.
Suna Wang and coworkers in 2018 (Chen et al., 2018) produced two LMOFs based on S-containing carboxylate acid and N-donor ligands, {[Zn(L) (bpp)]·DMF}n (1) and {[Zn(L) (bpe)]·DMF}n (2) (where L = 2,2'-[benzene-1,3-diylbis (methanediylsulfanediyl)]dibenzoic acid, bpp = 1,3-bis(4-pyridyl)propane, bpe = 1,2-Bis (4-pyridyl) ethylene, DMF = N,N-Dimethylformamide), with selectivity for Fe3+ and Cr2O72- in DMF. In the same year He-Gen Zheng and coworkers (Xiao et al., 2018) produced three Zn MOFs, {ZnL (chd)}n (1), {[Zn(L)0.5 (oba)]·DMF·H2O}n (2) and {[Zn(L)0.5 (sdb)]·H2O}n (3) [where L = E,E-2,5-dihexyloxy-1,4-bis-(2-pyridin-vinyl)-benzene; H2chd = 1,4-cyclohexanedicarboxylic acid, H2oba = 4,4’-oxybisbenzoic acid, H2sdb = 4,4’-sulfonyldibenzoic acid], able to selective sensing still for Fe3+ and Cr2O72-. Xian-He Bu and coworkers in 2020 (Yin et al., 2020) used the MOF [Zn (dptz) (BDC) (H2O)]n [1, dptz = 3,6-di (1H-pyrazol-4-yl)-1,2,4,5-tetrazine, H2BDC = terephthalic acid] for the same sensing analyte, and so Tuoping Hu and coworkers in 2019 (Gao L. et al., 2019) starting from three 3D metal–organic frameworks (MOFs), namely, {[Cd2(HL) (4,4-bipy) (H2O)]n_H2O} (1), {Zn2(HL) (phen)}n (2) and {Zn2(HL) (2,2-bipy)}n (3) obtained from the V-pattern multi-carboxylic acid ligand H5L (H5L = 3,5-di (2’,5’-dicarboxylphenyl)benzoic acid) and Cd(II)/Zn(II) salts.
The multi-responsive tools attract the interest of both researchers and technicians. Some Zn MOFs are multi-responsive probes for sensing biologically sensible targets. Zhong-Feng Shi and coworkers in 2020 produced (Xu et al., 2020) the LMOF [Zn2 (4,4’-nba)2 (1,4-bib)2]n (1) where 4,4’-H2nba = 3-nitro-4,4’-biphenyl dicarboxylic acid and 1,4-bib = 1,4-bis(imidazole-1-ylmethyl)-benzene, which can be used as a sensitive multi-responsive luminescent sensor for detecting of Fe3+, Cr2O72- and CrO42- anions in water and for sensing of nitrobenzene in ethanol. LOD values reach 1.76, 3.25, 3.8 and 0.19 mM, respectively. Another Zn MOF acting as a multi-response chemo-sensor was proposed in 2018 by Shao-Wei Zhang and coworkers (Zou et al., 2018). The MOF {[(CH3)2NH2]2 [Zn5 (TDA)4 (TZ)4].4DMF}n (1) (H2TDA = thiophene-2,5-dicarboxylic acid and HTZ = 1H-1,2,4-Triazole) based on Zn5(COO)2 (TZ)4 pentametallic clusters and thiophene-2,5-dicarboxylic acid was synthesized and post-functionalization via cation exchange with Eu3+ and Tb3+ afforded two other lanthanide MOFs: Eu3+@1 and Tb3+@1. The MOFs were utilized as selective and sensitive turn-off luminescent sensors for aniline, benzaldehyde in DMF and Cr2O72- and CrO42- anions in water.
In some cases, Zn MOFs are designed to be strongly selective toward a highly specific small molecule. Bing-Hui Wanga and Bing Yan in 2019 (Wang and Yan, 2019) produced a Zn MOF based on fluorescein (FS) dianion dye functionalized ([Zn2IJTipa)2IJOH)]·3NO3·12H2O). The MOF was designed to effectively detect trichloroacetic acid (TCA), a carcinogen metabolite in human urine. Jian-Zhong Cui and coworkers in 2018 (Cui et al., 2018) produced an anionic Zn MOF {[(CH3)2NH2][Zn(FDA) (BTZ)2]} n (1) (H2FDA = furan-2,5-dicarboxylic acid and HBTZ = 1H-benzotriazole) undergoing post-synthetic cation exchange with Cu2+, Co2+, and Ni2+. The probe works as a turn-off luminescent sensor sensitively and selectively detecting acetylacetone, a widely utilized volatile and flammable analytical reagent, with LOD = 0.647 μmol/L.
The interactions and the structural pattern in MOFs make them ideal candidates for detecting volatile organic compounds upon adsorption of the guest molecules. Yao Li and coworkers in 2020 (Guo et al., 2020) synthesized CNT@ZnSnO3 hollow boxes via hydrothermal reaction by using the Zn-MOF(ZIF-8) as the Zn source and carbon nanotubes (CNTs). CNT@ZnSnO3 demonstrated a large specific surface area (up to 45.73 m2/g), highly responsive to ethanol up to 166 at 240°C at the concentration of 100 ppm. Patima Nizamidin and coworkers in 2019 (Tuergong et al., 2019) proposed a series of Zn MOFs, [Zn2 (bdc)2 (dpNDI)]n (where bdc = terephthalic acid and dpNDI = N,N’-di (4-pyridyl)-1,4,5,8-naphthalenediimide), moulded in optically transparent membranes grown on a tin-diffused glass substrate. They display a large pore size (380 nm), uniform surface and lower refractive index. The films display a selective adsorption response to meta-xylene gas over interferents such as toluene, styrene and benzene gases, harmful and poisonous to the human body and the environment.
Zn MOFs responsive towards biologically harmful molecules, which are environmental organic pollutants, such as organic dyes, pesticides, antibiotics, are highly required sensing probes. Many industrial processes generate a large amount of wastewater containing organic dyes (Gupta and Suhas, 2009). Many commonly used dyes are toxic, and mutagens and their monitoring and removal are essential for water quality and the ecosystem. In 2017 Ruiping Deng and coworkers (Guo et al., 2017) reported a Zn MOF namely [Zn2 (btb)2 (bbis)](Me2NH2)2·6DMF, built from bbis = bis(4-benzylimidazol-ylphenyl)sulfone and H3btb = 4,4’,4’’-benzene-1,3,5-triyltribenzoic acid exhibiting a post-synthetic cation exchange with Eu3+, Tb3+, Dy3+ and Sm3+. The LMOF was found responsive in selective separation of methylene blue. Wen-Li Guo and coworkers in 2018 (Qu et al., 2018) and Lun Zhao and coworkers in 2019 (Zhao et al., 2019) presented two multifunctional systems able to sense iron and a dye. Specifically, in (Qu et al., 2018), a 2D Zn MOF, ZnL (H2O)3]_3H2O_DMF (BIPT-1_3H2O_DMF) has been synthesized by using bis-(3-carboxy-phenyl)furan-2,5-dicarboxamide (H2L) exhibiting high selectivity and sensitivity fluorescence quenching toward to Fe3+ ions in water and selectively adsorbing cationic dyes like methylene blue. In (Zhao et al., 2019) a Zn MOF, {[Zn2L2 (bibp)2]⋅H2O}n (L = N,N0-bis(4-carbozylbenzyl)-4-aminotoluene) presenting an 8-fold interpenetrating diamond networks can sensitively detect Fe3+ ions in water and selectively adsorbing methyl orange.
A Zn MOF for sensing of dichloronitroaniline pesticide and nitrofuran antibiotics in water was realized by Xiutang Zhang and coworkers in 2020 (Fan L. et al., 2020). A 3D self-penetrated framework, {[Zn (tptc)0.5 (bimb)]H2O}n (NUC-6), was built from 1,3-bis (imidazol-1-ylmethyl)benzene (bimb) and π-conjugated aromatic p-terphenyl-2,2”,5”,5”’-tetracarboxylate acid (H4tptc). The LOD was measured as 116 ppb for DCN pesticide, 16 ppb for NFT antibiotic, and 12 ppb for NTZ antibiotic. In the same year, Jarugu Narasimha Moorthy and coworkers (Mukhopadhyay et al., 2020) published a Zn-MOF (Zn-DBC), with ca. 27% solvent-accessible void volume from a tetracarboxylic acid linker based on a twisted dibenzo (g,p) chrysene core, 2,7,10,15-tetrakis [2,6-dimethyl-4-(α-carboxy)methoxyphenyl]-dibenzo (g,p) chrysene (H4DBC). The Zn-MOF shows sensing ability toward hazardous “quat” dicationic herbicide containing a diquaternary bipyridyl motif, with LOD< 2.8 ppm in water.
Still, in 2020, Qinhe Pan and coworkers in (Qin et al., 2020) designed a Zn MOF (HNU-50) for the detection and extraction of U (VI) as a potential environmental pollutant. The amide groups on N-pyridin-4-ylpyridine-4-carboxamide ligands and two uncoordinated carboxyl oxygen atoms on pyromellitic acid ligands provide potential uranium-binding sites allowing fluorescence sensitive and selective detection of U(VI) with LOD = 1.2 × 10–8 M for U(VI).
As a fundamental parameter for life, pH plays a pivotal role in many biological processes, so accurate monitoring of pH is highly required. Yulin Yang and coworkers in 2018 (Xing et al., 2018) produced a biocompatible Zn MOF as a drug delivery carrier with a high loading ability of 5-FU drug molecules. The Zn MOF [Zn-cpon-1 with the (3,6)-connected rtl 3D topological network constructed by semirigid 5-(4’-carboxyphenoxy)nicotinic acid (H2cpon)] was verified as a pH-responsive dual-emission tool, the controllable releasing being triggered via the thermal and pH dual stimulus. In 2020, En-Qing Gao and coworkers (Yang et al., 2020) produced a three-dimensional Zn MOF from a bipyridyl-tetracarboxylic ligand composed of 4-fold interpenetrated diamond frameworks (Figure 3). The free basic N sites make the probe pH-responsive. A reversible on-off fluorescence transition in the narrow pH range of 5.4–6.2 can be used for precise pH monitoring. In addition, the pH-responsive Zn MOF shows sensing performance towards 3-nitropropionic acid, a major mycotoxin in moldy sugar cane. Xiu-Li Wang and coworkers in 2019 (Lin et al., 2019) fabricated a dual-responsive Zn MOF, Zn-MOF@SWCNT composite with single-wall carbon nanotubes (SWCNTs). Zn-MOF@SWCNT exhibited a selective fluorescence quenching response to Fe (III) ions and a relationship between pH value and fluorescence intensity in the pH range from 1 to 13.
Temperature is a basic physical parameter of science, and its accurate measurement is crucial in scientific and technological contexts. A ratiometric fluorescence sensor can measure emission intensities at two or more wavelengths to accurately detect changes to the local environment/analyte. Several promising ratiometric Zn (II) LMOFs have been developed. Guodong Qian and coworkers in 2017 (Zhao et al., 2017) employed a tetracarboxylic acid ligand containing a highly polarized benzothiadiazole moiety to construct the luminescent porous Zn MOF ZJU-21 (Figure 3), which can absorb the luminescent dye 4-[p-(dimethylamino)styryl]-1-methylpyridinium (DMASM) affording the dual-emitting MOF*dye composite ZJU-21*DMASM. The emission color of the resulting composite can be modulated based on the amount of dye, and the intensity ratio of the two emissions can be used as a ratiometric probe for temperature measurement. Very recently, Banglin Chen and coworkers (Wang et al., 2021) synthesized two isostructural NbO-type Zn MOFs, where two similar tetracarboxylate ligands, 5,5'-(pyrazine-2,5-diyl) diisophthalic acid (H4PZDDI) and 5,5'-(pyridine-2,5-diyl) diisophthalic acid (H4PDDI), with pyridine or pyrazine moieties were used as the organic linkers. By absorbing pyridinium hemicyaninedye 4-[p-(dimethylamino) styryl]-1-methylpyridinium (DSM) and Eu3+ different composites were obtained, specifically DSM@ZnPZDDI and DSM@ZJU-56, and Eu3+@nPZDDI and Eu3+@ZJU-56. The europium adducts Eu3+@ZnPZDDI and Eu3+@ZJU-56 proved to be ratiometric luminescence thermometry with large sensing temperature range and higher sensitivities.
Zinc-Metal-Organic Frameworks and Other Nano-Enginereed Zinc-Based Tools for Living Cell Determinations
Fluorescence microscopy is the preferred choice by biologists owing to its high sensitivity, rapid response, and spatio-temporal resolution. Moreover, the possibility of a non-destructive analysis at the molecular level is a relevant added value. A wide array of fluorescent probes is available for use in fluorescence microscopy. Nanotechnology is a rapidly developing field with various applications in science and technology (Jun 2021). Nanosized fluorescent probes for bioimaging and living cell determination were recently discussed in several excellent reviews (Mittal and Banerjee, 2016; Zhao et al., 2016; Hemmer et al., 2017; Yang et al., 2019; Parmar et al., 2020). These fluorogenic probes include nanomaterial-based probes, (Nicewarner-Pena et al., 2001; Kamat, 2002; Singh and Lillard, 2009; Mittal and Banerjee, 2016; Macairan et al., 2020), from quantum dots and carbon nanomaterials to organic and inorganic nanoparticles for multimodal bioimaging and targeted therapy (Yang et al., 2019), and near-infrared emitting nanoparticles for imaging, thermal sensing, and photodynamic therapy (Hemmer et al., 2017). Another group of nanosized organic-inorganic hybrid materials, highly symmetric, used in fluorescence analysis are LMOFs. They are used as markers to detect biomolecules and therapeutic applications (antimicrobial, antiplatelet, drug delivery, and photothermal therapy) (Singh et al., 2020). Owing to their unique structures and properties, such as targeted symmetry, high surface area and density of active sites, tuneable pore size, various MOF-based sensing platforms have been reported for living cell detection and subcellular units. On the other hand, fluorescent NPs are commonly used to visualize the spatio-temporal dynamics of cells in various biomedical applications (Singh et al., 2017).
Recently, there has been considerable interest in developing nanoprobes based on bio-compatible cations together with organic scaffolds. The employ of metal nodes of zinc (II) ion in building novel engineered tools for bioimaging is quite recent. In many cases, such hybrid organic-inorganic nanoprobes meet the requirement of good cell penetration without perturbing the cell physiology. Most Zn-MOF and Zn-NPs were studied for their sensing ability. Moreover, several zinc-based MOFs and nanoprobes have been designed as a specific therapeutical tool against cancer cell proliferation.
In this section, we summarize the progress made during the past 5 years in the field of zinc-based nanoprobes acting in living cells, with an emphasis on the advantages of each specified system. Specifically, sensing performance toward toxic ions and small molecules in living cells, anticancer drug (such as 5-fluorouracil) delivery, anti-viability and anti-proliferation ability, efficient cellular uptake by high optical penetrating power at the deep tissue level to target cancer cells in bioimaging will be discussed. Some relevant structures of the most recent examples of Zn-MOFs and other highly engineered nanosized in vivo zinc tools are reported in Figure 4.
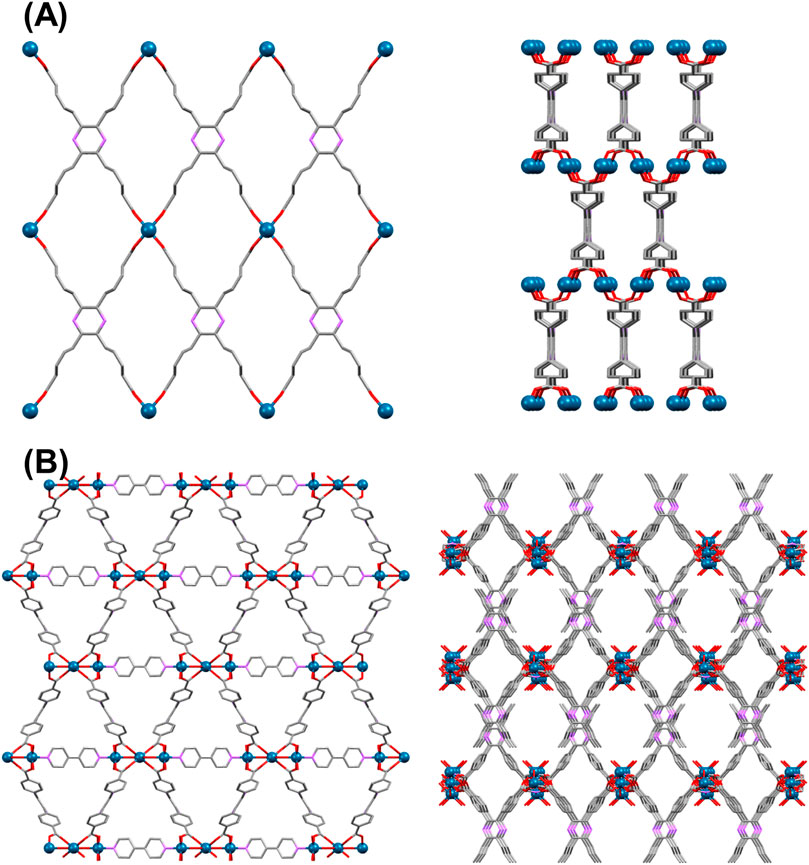
FIGURE 4. Some representative structures of Zn-MOFs for living cell determinations. (A) by Hua Yang (Hu et al., 2020); (B) by Wei-Ping Zhang (Wu et al., 2021).
The sensing performance of Zn-MOFs already discussed in Zinc-Metal-Organic Frameworks for Biological Targets Sensing, finds the more desirable bioengineered application in the living cell imaging (Martynov et al., 2018; Macairan et al., 2020).
Xiaoyan Liu and coworkers in 2018 (Pan et al., 2018) produced a Zn-MOF based on L = 2,5-bis (phenylamino)-1,4-benzenedicarboxylic acid named Zn2(L)2 (DMF)2H2O for sensing applications in a living cell. The probe exhibited fluorescence enhancement for cadmium (II) ion and fluorescence quenching sensing in presence of nitrobenzene with LOD = 0.12 mM for Cd2+ and 1.19 mg/ml for nitrobenzene. To explore whether Zn-MOF can be used for cell imaging, its toxicity to living cells was investigated. It was found that the cellular viability of HepG2 remains over 94% in the presence of 4.0 mg/ml Zn-MOF and that the cytotoxicity in DMF results in more than 88% of cells surviving at 8.0 mg/ml Zn-MOF. The Zn-MOF sensing ability toward Cd2+ was tested in real water samples and in HepG2 living cells, where an obvious yellow fluorescence was observed in the presence of the adduct probe-Cd2+.
In addition to sensing performance, several Zn-MOFs tailored as therapeutic tools were recently proposed. In 2020 Hua Yang and coworkers (Hu et al., 2020) presented a novel Zn-LMOF named [Zn2 (OH)2 (H2TCPP)](DMF)3 was synthesized by using the rigid symmetric 2,3,5,6-tetrakis (4-carboxyphenyl)pyrazine ligand and zinc (II) crosslinkers obtaining a sea-type net 3D structure. The MOF can selectively detect picric acid in solution with a quenching effect. Moreover, the Zn-MOF’s inhibitory effect on various cancer cell lines (HeLa, CHO, HepG2, U251, MDA-MB-435 S and BEAS-2B) was detected via CCK-8 (Cell counting kit-8) method. The influence on the U251 human glioma cells invasion, migration and proliferation was detected with the transwell method. In the same year, Xiao-Ming Han and coworkers (Peng et al., 2020) produced a Zn-MOF named [Zn2 (cipa) (H2O)2] (DMF) from 5,5’-carbonyldiisophthalic acid (H4cipa) in a mixed DMF/water solvent (Figure 5). The treatment activity against the Mg63 osteosarcoma cell line was evaluated, and the mechanism was proposed. Real-time RT-PCR (reverse transcription-polymerase chain reaction) assay was used to measure the relative expression levels of the autophagic genes under treatment. Due to the porous size-selectivity, an interesting application as a heterogeneous catalyst for forming C–C bonds of carbonyl compounds was also detected. Interestingly, catalytic activity can accompany the therapeutic purpose or be an integral part of it. Still, in 2020, a catalytic activity (for aromatic aldehydes cyanation) was detected for a Zn-MOF obtained by Wei-Ping Zhang and coworkers in (Wu et al., 2021). The MOF was obtained from a rigid V-shaped ligand 2,6-di (2’,5’-dicarboxylphenyl)pyridine in DMF/water solvent and named [Zn3(L) (OH)2(H2O)4](DMF)5. The reverse transcription-polymerase chain reaction (PT-PCR) was used to determine cylindromatosis (CYLD) and miR-130 relative expression in bladder cancer cells. The treatment ability of the compound on bladder cancer cells was assessed and the mechanism explored by evaluating the level of autophagic programmed cell death by western blot.
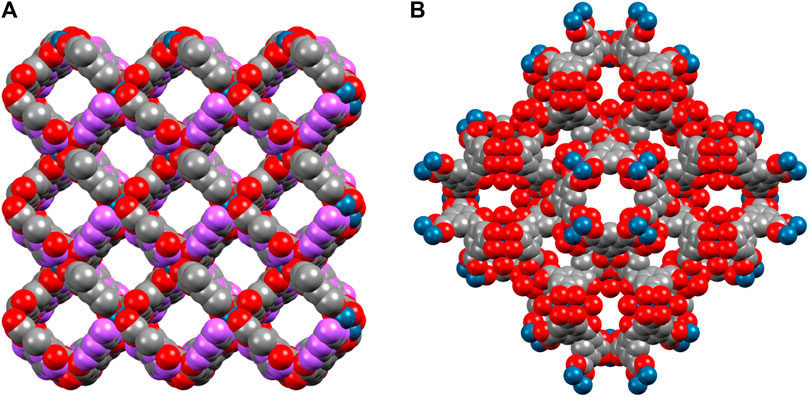
FIGURE 5. Perspective view of the channels and cages formed within the three-dimensional skeleton of (A) ZnMOF for drug release by Xiao-Tao Xin and Jia-Ze Cheng, 2018 (Xin and Cheng, 2018); (B) ZnMOF for drug release and anticancer activity by Xiao-Ming Han, 2020 (Peng et al., 2020).
The employ of LMOFs as drug-delivering nanoprobes could be the application with the strongest biomedical impact. Suhui Wu and coworkers in 2020 (Yan et al., 2020) affords a new Zn-MOF [Zn3(OH)2(H2tccp)2 (bpy)2](H2O)3 (DMF)3 from ligand 2,3,5,6-tetrakis (4-carboxyphenyl)pyrazine (H4tcpp) and 4,4’-bipyridine (bpy) (Figure 6). The anticancer drug 5-fluorouracil (5-Fu) resulted in being captured into the nanopores preferentially with a loading capacity of 30.7% from the drug loading experiment. At the same time, the 5-fluorouracil was controlled to release in the artificial human body with a phosphate-buffered saline solution. The anti-viability and anti-proliferation activity of 5-Fu@1a vs. CoC1 ovarian cancer cells was carried out by Cell Counting Kit-8 (CCK-8) assay and clone formation assay. 5-Fu@1a showed an excellent anticancer effect in a caspase-dependent manner, causing CoC1 ovarian cancer apoptotic death. Another 5-Fu carrier was designed and studied in 2018 by Xiao-Tao Xin and Jia-Ze Cheng (Xin and Cheng, 2018) which produced a Zn-MOF, [Zn(BTC) (HME)](DMAc) (H2O) (where H3BTC = 1,3,5-benzenetricarboxylic acid, HME = protonated melamine, DMAc = N,N-dimethylacetamide) (Figure 5). Due to many N-donor sites and suitable pore size, the de-solvated MOF was a moderate loading carrier of 5-Fu molecules. The cytotoxicity was evaluated using MTT assays against human oral squamous cell carcinoma (SCC-251 and HSC-4).
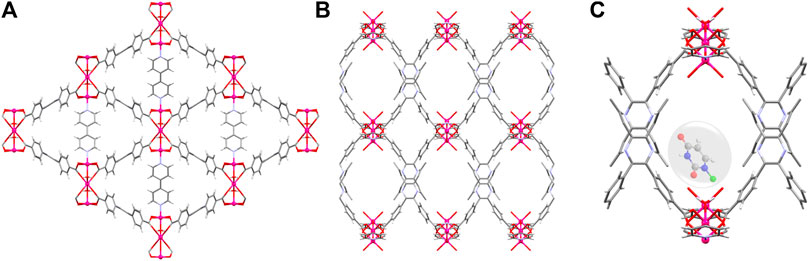
FIGURE 6. Zn-MOF 5-Fu@1a by (Yan et al., 2020). (A) The one-dimensional triangular channels along b axis with the coordinated bpy ligand. (B) The one-dimensional rhombic channels along the c axis. (C) The 5-Fu molecule’s advantageous binding site.
Huwei Liu and coworkers in 2017 (Qi et al., 2017) proposed a functional MOF modified with antibodies to combine cell recognition and targeted cell capture with controllable drug delivery. A ZnMOF-COOH assembled in situ forms a platform for further functionalization (Figure 7). The derivatization of surface acid groups to give peptide linkages offers a strategy for immobilizing antibodies on the MOF. The resulting Zn-MOF core acts as a selective tumor cells trapper. Due to the peculiar structure, the Zn-MOF is able to deliver and to release drugs in specific conditions and in response to external stimuli. An interesting engineered application of Zn-MOF as an antibacterial tool was designed by Chunsheng Wu and coworkers in 2020 (Chen et al., 2020) by using a zeolitic imidazolate frameworks-8 (ZIF-8) to build a cell-in-shell structure onto living cells. The in-situ encapsulation process involved cell death depending on the zinc (II) salt employed in forming the MOF. The ZIF-8 coating could engineer the cell division, suppressing cell budding and growth. After the removal of the MOF shell, the cell growth could recover. This coating method was applied to yeast cells and can be used in the treatment of bacteria cells.
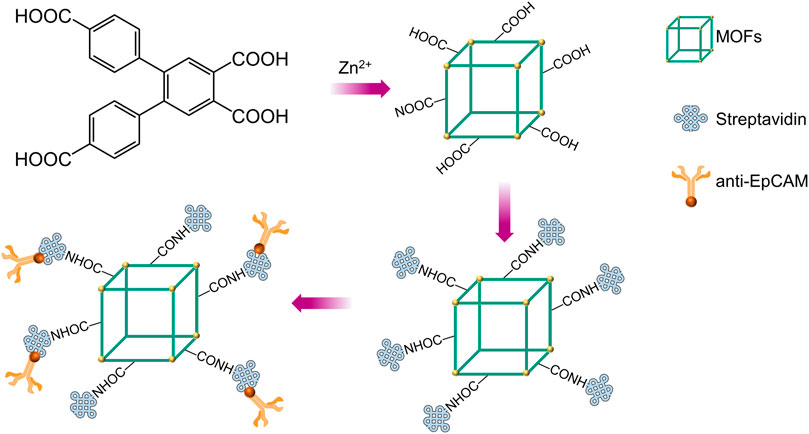
FIGURE 7. Schematic Illustrating the Synthetic Strategy for fabricating a MOF-Based Immunotrapper (Qi et al., 2017).
Nanoprobes for bioimaging selectively e specifically monitoring tumor cells, are highly required. Upconverting nanoparticles (UCNPs) are nanoscaled particles exhibiting photon upconversion. In this process, two or more incident photons of low energy are absorbed and converted into one emitted photon with higher energy. Typically, emission occurs in the visible region of the electromagnetic spectrum. UCNPs are known for their applications in bioimaging, biosensing, and nanomedicine thanks to their highly efficient cellular uptake, high optical penetrating power, and little background noise at the deep tissue level. Yu Tang and coworkers in 2017 (Cai et al., 2017) produced a core-shell MOF-based nanocomposite UCNPs/MB@ZIF-8@ catalase (UCNPs = upconverting nanoparticles; MB = methylene blue; ZIF = zeolitic imidazolate framework) useful in bioimaging and NIR/H2O2-responsive in photodynamic therapy vs. hypoxic tumor cells. The nanoporous MOF shell prevents aggregation of photosensitizers and acts as an efficient, self-sufficient oxygen gas acceptor.
The employ of fluorescence AIE (aggregation induced enhancement) active markers state exhibit stronger emission in the concentred solution (Caruso et al., 2018; Diana and Panunzi, 2021) than in diluted ones. AIE undergoing probes can solve problems due to fluorescence quenching of the aggregates in aqueous physiologic media and are very useful in bioimaging (Fan et al., 2020b; Ying et al., 2021). Dongdong Sun and coworkers in 2019 (Zhang et al., 2019) fabricated gold nanoprisms (AuNPR) conjugated to phenanthroline derivatives functionalized tetraphenylethene (TPE) with aggregation induced enhancement (AIE) of fluorescence. The probe was stabilized with target peptide aptamers via Au-S bonds (Au-Apt-TPE). The free nitrogen atoms in Au-Apt-TPE chelate zinc (II) ions (Au-Apt-TPE@Zn) which was used for selectively monitoring early-stage apoptotic cells. Au-Apt-TPE@Zn showed deep penetration and photothermal therapy against SGC-7901 human gastric carcinoma cells growth under NIR irradiation. Another AIE-based system was developed in 2018 by Yun Yan and coworkers (Wei et al., 2018). A fluorescent vesicle based on triarylamine carboxylate AIE molecules (TPA-1) interacts with zinc (II) ion, and forms the complex Zn (TPA-1)2, which further self-assembles into vesicles. The vesicles are cell imaging probes as tested in Hela cells.
Nanotechnology based on MOF NPs is a valid strategy to improve the efficacy of cell-mediated drugs delivery for cancer therapy. Dong-Pyo Kim and coworkers in 2021 (Ha et al., 2021) employed Janus carrier cells coated with MOFs by asymmetrical immobilizing the nanoparticles of a Zn-MOF with cytotoxic enzymes encapsulated on the surface of carrier cells (Figure 8). Retaining the biological and structural features of the living cells, the MOF-coated Janus cells have the same binding ability as the regular cells to their microenvironment. Still, they are protected from the cytotoxic drug contained in MOFs. When a chemotherapeutic protein of proteinase K is released from the MOF nanoparticles in an acid environment, the composite MOF-Janus probe can eliminate three-dimensional (3D) tumor spheroids. Still, in 2021 Mosad A. El-ghamry and coworkers (El-Ghamry and El-Shafiy, 2021) produced several complexes from Co(II), Ni(II), Cu(II), Zn(II), and Fe(III) and the ligand 3-(1-methyl-4-hydroxy-2-oxo-1,2-dihydroquinolin-3-yl)-2-nitro-3-oxopropanoic acid (H3L). The antimicrobial properties of the ligand showed enhancement upon coordination, and the antitumor activity was evaluated against the Hepatocellular carcinoma cell line (HepG-2 cells). The Zn (II) complex exhibits bio-efficiency over other complexes. Nanosized Zn (II) complex exhibited IC50 value (2.90 μg/ml) smaller than the standard antitumor drug cis-platin (IC50 ∼ 3.27 μg/ml) towards HepG-2 cells.
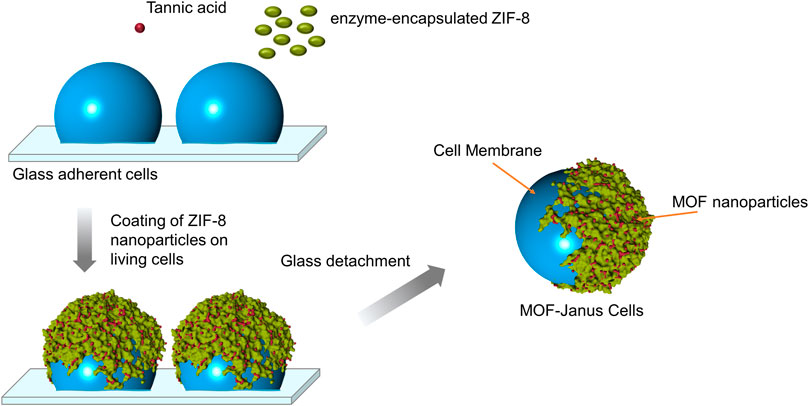
FIGURE 8. Schematic Illustration of the development of MOF-Janus cell-based drug delivery system (Ha et al., 2021).
Peptide nanoprobes and DNA functionalized nanomaterials showed great potential in biomedical applications. In 2016 mingjun Zhang and coworkers (Fan et al., 2016) designed tryptophan–phenylalanine dipeptide (Trp-Phe DNPs) self-assemblies through Zn (II) coordination in nanoparticles (Figure 9) emitting the visible range rather than in the ultraviolet (as for the neat peptide), and therefore employable in bioimaging. The probe resulted in photostable and biocompatible. Trp-Phe DNPs functionalized with the MUC1 aptamer and doxorubicin can target cancer cells and can be used in bioimaging and monitoring drug release. In 2020 Shengfeng Wang and coworkers (Wang S. et al., 2020) explored the use of nanoscale zeolitic imidazolate framework-8 (ZIF-8) as a building block for DNA-based nanoprobe. Specifically, they designed a DNAzyme embedded molecular beacon (DMB) to functionalize ZIF-8. Upon endocytosis, ZIF-8 was disintegrated to release DMB for target mRNA detection. The co-released zinc (II) cation acts as an effective cofactor to activate the embedded DNAzyme for mRNA regulation.
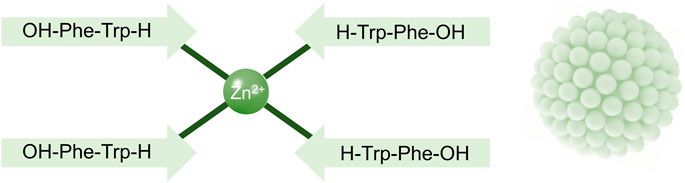
FIGURE 9. Fluorescent dipeptide nanoparticles DNPs obtained from Trp-Phe dipeptides and Zn (II) by Mingjun Zhang (Fan et al., 2016).
Rare examples of Zn-MOFs in the effective fabrication of nano-engineered biomedical devices and supplies are known. They are a very interesting check of the technological potential of Zn-MOFs. Yongan Wang and coworkers (Yao et al., 2021) fabricated a biocompatible and degradable Zn-MOF named ZIF-8@MeHA-MNs by encapsulating zeolitic imidazolate frameworks-8 (ZIF-8) into a photo-crosslinked methacrylated hyaluronic acid (MeHA) hydrogel and formed into microneedles (MNs) by using the molding method (Figure 10). MNs array loaded with active can produce remarkable therapeutic effects benefiting from the large contact area between the MNs patch and the wound. Due to the zinc ion gradually released from the Zn-MOF, the MNs array presents antibacterial activity and steadily releases the active ingredients. The low molecular weight hyaluronic acid (HA) generated by hydrolysis of MeHA is advantageous for tissue regeneration and neovascularization. Live/Dead cell staining of NIH-3T3 cell lines was examined.
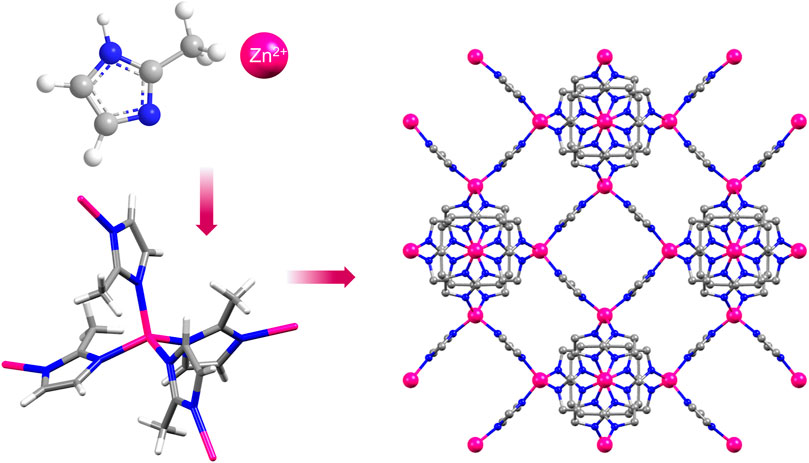
FIGURE10. Biocompatible and degradable ZIF-8, by Yongan Wang (Yao et al., 2021).
Conclusions and Perspectives
The review summarizes the recent achievements of zinc-based luminescent nanoprobes. During the past 5 years, the development of nanoprobes able to cell permeation has undergone rapid growth.
The wide application of nanotechnology is moved by the growing demand of biomedical imaging techniques. In the continuous efforts to develop novel efficient and specific nanoprobes, the medical application of Zn nanoparticles has expanded horizons in the field of diagnosis, monitoring, and therapeutic delivery. Highly bioengineered structures can be built using zinc (II) atoms in hybrid organic-inorganic supramolecular structures. In the search for such hybrid nano-organised tools with unique photophysical properties, zinc (II) cation claims a role in designing novel chemo and biosensors, markers, and drug carriers. The non-toxic, abundant, and inexpensive zinc (II) metal cation offers several benefits in building versatile CPs structures, MOFs, and other NPs. The scale and the nature of cell and tissues is meaningful and imposes some structural, optical, and biological constraints that zinc ion can satisfy. The most relevant examples of Zn MOFs and Zn-NPs from the last 5 years (2016–2021) were collected and discussed with a systematic and consequential approach. A progression from detecting the biological target to the intracellular sensing/marking/carriage has been followed. A selection of significant cutting-edge articles has been discussed, based on the structural pattern and sensing performance, with special notice to living cell bioimaging as the most targeted and desirable application. In short, the review displays an overview, from the latest Zn-MOFs designed for metals, ions, and small molecules with biological and environmental relevance, to the Zn-MOFs and Zn-NPs targeted for living cell imaging and drug carriers. We trust that the short but comprehensive approach can put foundations for new projects in the biosensing technological frontier. Finally, we believe and expect that zinc-based nanoprobes will be developed by researchers in near future, with the aim of combining highly biocompatible systems with deeply permeation PL instruments for treatment, targeting and diagnosis of cancer.
Author Contributions
RD, LS, and SC: conceptualization. RD and LS: writing—original draft preparation. BP and SC: writing—review and editing. SP and BP: supervision. All authors contributed to manuscript revision, read, and approved the submitted version.
Conflict of Interest
The authors declare that the research was conducted in the absence of any commercial or financial relationships that could be construed as a potential conflict of interest.
Publisher’s Note
All claims expressed in this article are solely those of the authors and do not necessarily represent those of their affiliated organizations, or those of the publisher, the editors, and the reviewers. Any product that may be evaluated in this article, or claim that may be made by its manufacturer, is not guaranteed or endorsed by the publisher.
References
Abu-Dief, A. M., and Mohamed, I. M. A. (2015). A Review on Versatile Applications of Transition Metal Complexes Incorporating Schiff Bases. Beni-Suef Univ. J. Basic Appl. Sci. 4, 119–133. doi:10.1016/j.bjbas.2015.05.004
Alam, P., Climent, C., Alemany, P., and Laskar, I. R. (2019). "Aggregation-induced Emission" of Transition Metal Compounds: Design, Mechanistic Insights, and Applications. J. Photochem. Photobiol. C: Photochem. Rev. 41, 100317. doi:10.1016/j.jphotochemrev.2019.100317
Aljammal, N., Jabbour, C., Thybaut, J. W., Demeestere, K., Verpoort, F., and Heynderickx, P. M. (2019). Metal-organic Frameworks as Catalysts for Sugar Conversion into Platform Chemicals: State-Of-The-Art and Prospects. Coord. Chem. Rev. 401, 213064. doi:10.1016/j.ccr.2019.213064
Barnett, B. R., Gonzalez, M. I., and Long, J. R. (2019). Recent Progress towards Light Hydrocarbon Separations Using Metal-Organic Frameworks. Trends Chem. 1, 159–171. doi:10.1016/j.trechm.2019.02.012
Borbone, F., Caruso, U., Palma, S. D., Fusco, S., Nabha, S., Panunzi, B., et al. (2015). High Solid State Photoluminescence Quantum Yields and Effective Color Tuning in Polyvinylpyridine Based Zinc(II) Metallopolymers. Macromol. Chem. Phys. 216, 1516–1522. doi:10.1002/macp.201500120
Cai, H.-J., Shen, T.-T., Zhang, J., Shan, C.-F., Jia, J.-G., Li, X., et al. (2017). A Core-Shell Metal-Organic-Framework (MOF)-based Smart Nanocomposite for Efficient NIR/H2O2-responsive Photodynamic Therapy against Hypoxic Tumor Cells. J. Mater. Chem. B 5, 2390–2394. doi:10.1039/c7tb00314e
Cai, H., Huang, Y.-L., and Li, D. (2019). Biological Metal-Organic Frameworks: Structures, Host-Guest Chemistry and Bio-Applications. Coord. Chem. Rev. 378, 207–221. doi:10.1016/j.ccr.2017.12.003
Caruso, U., Diana, R., Panunzi, B., Roviello, A., Tingoli, M., and Tuzi, A. (2009). Facile Synthesis of New Pd(II) and Cu(II) Based Metallomesogens from Ligands Containing Thiophene Rings. Inorg. Chem. Commun. 12, 1135–1138. doi:10.1016/j.inoche.2009.09.006
Caruso, U., Panunzi, B., Roviello, A., Tingoli, M., and Tuzi, A. (2011). Two Aminobenzothiazole Derivatives for Pd(II) and Zn(II) Coordination. Inorg. Chem. Commun. 14, 46–48. doi:10.1016/j.inoche.2010.09.027
Caruso, U., Panunzi, B., Roviello, A., and Tuzi, A. (2013). Fluorescent Metallopolymers with Zn(II) in a Schiff Base/phenoxide Coordination Environment. Inorg. Chem. Commun. 29, 138–140. doi:10.1016/j.inoche.2012.11.037
Caruso, U., Panunzi, B., Diana, R., Concilio, S., Sessa, L., Shikler, R., et al. (2018). AIE/ACQ Effects in Two DR/NIR Emitters: a Structural and DFT Comparative Analysis. Molecules 23, 1947. doi:10.3390/molecules23081947
Castellano, F. N., Pomestchenko, I. E., Shikhova, E., Hua, F., Muro, M. L., and Rajapakse, N. (2006). Photophysics in Bipyridyl and Terpyridyl Platinum(II) Acetylides. Coord. Chem. Rev. 250, 1819–1828. doi:10.1016/j.ccr.2006.03.007
Causà, M., D’Amore, M., Garzillo, C., Gentile, F., and Savin, A. (2013). “The Bond Analysis Techniques (ELF and Maximum Probability Domains) Application to a Family of Models Relevant to Bio-Inorganic Chemistry,” in Applications of Density Functional Theory to Biological and Bioinorganic Chemistry. Editors M. V. Putz, and D. M. P. Mingos. (Berlin, Heidelberg: Springer Berlin Heidelberg), 119–141. doi:10.1007/978-3-642-32750-6_4
Chai, W.-X., Lin, J., Song, L., Qin, L.-S., Shi, H.-S., Guo, J.-Y., et al. (2012). Three Iodometalate Organic-Inorganic Hybrid Materials Based on Methylene Blue Cation: Syntheses, Structures, Properties and DFT Calculations. Solid State. Sci. 14, 1226–1232. doi:10.1016/j.solidstatesciences.2012.06.002
Chen, L. X., Jäger, W. J. H., Gosztola, D. J., Niemczyk, M. P., and Wasielewski, M. R. (2000). Ionochromic Effects and Structures of Metalated Poly(p-Phenylenevinylene) Polymers Incorporating 2,2'-Bipyridines. J. Phys. Chem. B 104, 1950–1960. doi:10.1021/jp993365i
Chen, Z., Mi, X., Wang, S., Lu, J., Li, Y., Li, D., et al. (2018). Two Novel Penetrating Coordination Polymers Based on Flexible S-Containing Dicarboxylate Acid with Sensing Properties towards Fe3+ and Cr2O72- Ions. J. Solid State. Chem. 261, 75–85. doi:10.1016/j.jssc.2018.02.008
Chen, W., Kong, S., Lu, M., Chen, F., Cai, W., Du, L., et al. (2020). Comparison of Different Zinc Precursors for the Construction of Zeolitic Imidazolate Framework-8 Artificial Shells on Living Cells. Soft matter 16, 270–275. doi:10.1039/c9sm01907c
Chowdhury, M. A. (2017). Metal-organic-frameworks for Biomedical Applications in Drug Delivery, and as MRI Contrast Agents. J. Biomed. Mater. Res. 105, 1184–1194. doi:10.1002/jbm.a.35995
Christie, R. M. (1994). Pigments, Dyes and Fluorescent Brightening Agents for Plastics: An Overview. Polym. Int. 34, 351–361. doi:10.1002/pi.1994.210340401
Cui, J., Ren, S., Sun, B., and Jia, S. (2018). Optimization Protocols and Improved Strategies for Metal-Organic Frameworks for Immobilizing Enzymes: Current Development and Future Challenges. Coord. Chem. Rev. 370, 22–41. doi:10.1016/j.ccr.2018.05.004
Dey, N., Biswakarma, D., and Bhattacharya, S. (2019). Metal Complex as an Optical Sensing Platform for Rapid Multimodal Recognition of a Pathogenic Biomarker in Real-Life Samples. ACS Sustain. Chem. Eng. 7, 569–577. doi:10.1021/acssuschemeng.8b04107
Di Palma, G., Kirtman, B., Gentile, F. S., Platonenko, A., Ferrari, A. M., and Dovesi, R. (2020). The VN2 Negatively Charged Defect in diamond. A Quantum Mechanical Investigation of the EPR Response. Carbon 159, 443–450. doi:10.1016/j.carbon.2019.12.031
Diana, R., and Panunzi, B. (2021). Zinc (II) and AIEgens: The “Clip Approach” for a Novel Fluorophore Family. A Review. Molecules 26, 4176. doi:10.3390/molecules26144176
Diana, R., Caruso, U., Concilio, S., Piotto, S., Tuzi, A., and Panunzi, B. (2018). A Real-Time Tripodal Colorimetric/fluorescence Sensor for Multiple Target Metal Ions. Dyes Pigm. 155, 249–257. doi:10.1016/j.dyepig.2018.03.055
Diana, R., Panunzi, B., Concilio, S., Marrafino, F., Shikler, R., Caruso, T., et al. (2019a). The Effect of Bulky Substituents on Two π-Conjugated Mesogenic Fluorophores. Their Organic Polymers and Zinc-Bridged Luminescent Networks. Polymers 11, 1379. doi:10.3390/polym11091379
Diana, R., Panunzi, B., Shikler, R., Nabha, S., and Caruso, U. (2019b). Highly Efficient Dicyano-Phenylenevinylene Fluorophore as Polymer Dopant or Zinc-Driven Self-Assembling Building Block. Inorg. Chem. Commun. 104, 145–149. doi:10.1016/j.inoche.2019.04.007
Diana, R., Panunzi, B., Tuzi, A., and Caruso, U. (2019c). Two Tridentate Pyridinyl-Hydrazone Zinc(II) Complexes as Fluorophores for Blue Emitting Layers. J. Mol. Struct. 1197, 672–680. doi:10.1016/j.molstruc.2019.07.098
El‐Ghamry, M. A., and El‐Shafiy, H. (2021). Synthesis and Structural Characterization of Nano‐sized Metal Complexes of 3‐(1‐methyl‐4‐hydroxy‐2‐oxo‐1, 2‐dihydroquinolin‐3‐yl)‐2‐nitro‐3‐oxopropanoic Acid. XRD, thermal, 3D Modeling, and Antitumor Activity Studies. Appl. Organomet. Chem. 35, e6206. doi:10.1002/aoc.6206
Fan, Z., Sun, L., Huang, Y., Wang, Y., and Zhang, M. (2016). Bioinspired Fluorescent Dipeptide Nanoparticles for Targeted Cancer Cell Imaging and Real-Time Monitoring of Drug Release. Nat. Nanotech 11, 388–394. doi:10.1038/nnano.2015.312
Fan, C., Wang, L., Xu, C., Wu, R., Li, N., Zhang, D., et al. (2020a). Synthesis, Structure Diversity, and Dye Adsorption and Luminescent Sensing Properties of Zinc (II) Coordination Polymers Based on 1,3,5-Tris(1-Imidazolyl)benzene and 1,3-Bis(1-Imidazolyl)toluene. J. Solid State. Chem. 288, 121445. doi:10.1016/j.jssc.2020.121445
Fan, C., Zhang, X., Li, N., Xu, C., Wu, R., Zhu, B., et al. (2020b). Zn-MOFs Based Luminescent Sensors for Selective and Highly Sensitive Detection of Fe3+ and Tetracycline Antibiotic. J. Pharm. Biomed. Anal. 188, 113444. doi:10.1016/j.jpba.2020.113444
Fan, L., Wang, F., Zhao, D., Peng, Y., Deng, Y., Luo, Y., et al. (2020c). A Self‐penetrating and Chemically Stable Zinc (Ii)‐organic Framework as Multi‐responsive Chemo‐sensor to Detect Pesticide and Antibiotics in Water. Appl. Organomet. Chem. 34, e5960. doi:10.1002/aoc.5960
Farahani, Y. D., and Safarifard, V. (2019). Highly Selective Detection of Fe3+, Cd2+ and CH2Cl2 Based on a Fluorescent Zn-MOF with Azine-Decorated Pores. J. Solid State. Chem. 275, 131–140. doi:10.1016/j.jssc.2019.04.018
Gao, L., Zhang, J., Zhai, L., Liang, J., Liang, J., Niu, X., et al. (2019a). Fluorescent Sensing Properties of Cd(II)/Zn(II) Metal-Organic Frameworks Based on 3,5-Di(2′,5′-Dicarboxylphenyl)benozoic Acid. Polyhedron 164, 90–95. doi:10.1016/j.poly.2019.02.043
Gao, Q., Xu, J., and Bu, X.-H. (2019b). Recent Advances about Metal-Organic Frameworks in the Removal of Pollutants from Wastewater. Coord. Chem. Rev. 378, 17–31. doi:10.1016/j.ccr.2018.03.015
Gentile, F. S., Salustro, S., Desmarais, J. K., Ferrari, A. M., D'arco, P., and Dovesi, R. (2018). Vibrational Spectroscopy of Hydrogens in diamond: a Quantum Mechanical Treatment. Phys. Chem. Chem. Phys. 20, 11930–11940. doi:10.1039/c8cp00596f
Griffin, S. L., and Champness, N. R. (2020). A Periodic Table of Metal-Organic Frameworks. Coord. Chem. Rev. 414, 213295. doi:10.1016/j.ccr.2020.213295
Gu, T.-Y., Dai, M., Young, D. J., Ren, Z.-G., and Lang, J.-P. (2017). Luminescent Zn(II) Coordination Polymers for Highly Selective Sensing of Cr(III) and Cr(VI) in Water. Inorg. Chem. 56, 4668–4678. doi:10.1021/acs.inorgchem.7b00311
Guo, M., Liu, S., Guo, H., Sun, Y., Guo, X., and Deng, R. (2017). The Mixed-Ligand Strategy to Assemble a Microporous Anionic Metal-Organic Framework: Ln3+ post-functionalization, Sensors and Selective Adsorption of Dyes. Dalton Trans. 46, 14988–14994. doi:10.1039/c7dt02506h
Guo, R., Wang, H., Tian, R., Shi, D., Li, H., Li, Y., et al. (2020). The Enhanced Ethanol Sensing Properties of CNT@ZnSnO3 Hollow Boxes Derived from Zn-MOF(ZIF-8). Ceramics Int. 46, 7065–7073. doi:10.1016/j.ceramint.2019.11.198
Gupta, V. K., and Suhas, (2009). Application of Low-Cost Adsorbents for Dye Removal - A Review. J. Environ. Manage. 90, 2313–2342. doi:10.1016/j.jenvman.2008.11.017
Ha, L., Choi, K. M., and Kim, D.-P. (2021). Interwoven MOF-Coated Janus Cells as a Novel Carrier of Toxic Proteins. ACS Appl. Mater. Inter. 13, 18545–18553. doi:10.1021/acsami.1c01927
Hancock, R. D. (2013). The Pyridyl Group in Ligand Design for Selective Metal Ion Complexation and Sensing. Chem. Soc. Rev. 42, 1500–1524. doi:10.1039/c2cs35224a
Hemmer, E., Acosta-Mora, P., Méndez-Ramos, J., and Fischer, S. (2017). Optical Nanoprobes for Biomedical Applications: Shining a Light on Upconverting and Near-Infrared Emitting Nanoparticles for Imaging, thermal Sensing, and Photodynamic Therapy. J. Mater. Chem. B 5, 4365–4392. doi:10.1039/c7tb00403f
Herpoldt, K.-L., Loynachan, C. N,, and Stevens, M. M. (2017). 3.29 Nanomaterials for Biological Sensing. Comprehensive Biomaterials 2nd edn. Editors P. Ducheyne. (Oxford: Elsevier).
Hosomi, T., Masai, H., Fujihara, T., Tsuji, Y., and Terao, J. (2016). A Typical Metal-Ion-Responsive Color-Tunable Emitting Insulated π-Conjugated Polymer Film. Angew. Chem. Int. Ed. 55, 13427–13431. doi:10.1002/anie.201603160
Hu, Y.-J., Li, Y.-M., Zheng, K., Zhang, W.-H., Liu, Y.-L., and Yang, H. (2020). Construction of a Zn(II)-containing MOF for Highly Selective Detection of Picric Acid and Inhibition of Human Glioma Cell Growth. J. Mol. Struct. 1202, 127359. doi:10.1016/j.molstruc.2019.127359
Iacomi, P., Formalik, F., Marreiros, J., Shang, J., Rogacka, J., Mohmeyer, A., et al. (2019). Role of Structural Defects in the Adsorption and Separation of C3 Hydrocarbons in Zr-Fumarate-MOF (MOF-801). Chem. Mater. 31, 8413–8423. doi:10.1021/acs.chemmater.9b02322
Işık Büyükekşi, S., Orman, E. B., Acar, N., Altındal, A., Özkaya, A. R., and Şengül, A. (2019). Electrochemical, Photovoltaic and DFT Studies on Hybrid Materials Based on Supramolecular Self-Assembly of a Ditopic Twisted Perylene Diimide with Square-Planar Platinum(II)- And/or Palladium(II)-2,2′:6′,2″-terpyridyl Complex Ions. Dyes Pigm. 161, 66–78. doi:10.1016/j.dyepig.2018.09.022
Jensen, S., Tan, K., Lustig, W., Kilin, D., Li, J., Chabal, Y. J., et al. (2019). Quenching of Photoluminescence in a Zn-MOF Sensor by Nitroaromatic Molecules. J. Mater. Chem. C 7, 2625–2632. doi:10.1039/c8tc06281a
Jia, J., Xu, J., Wang, S., Wang, P., Gao, L., Chai, J., et al. (2016). Layer-structured Coordination Polymers Based on 5-(1h-Tetrazol-5-Yl)isophthalic Acid: Structure, Sensitization of Lanthanide(iii) Cations and Small-Molecule Sensing. CrystEngComm 18, 7126–7134. doi:10.1039/c6ce00883f
Jiang, Y., Sun, L., Du, J., Liu, Y., Shi, H., Liang, Z., et al. (2017). Multifunctional Zinc Metal-Organic Framework Based on Designed H4TCPP Ligand with Aggregation-Induced Emission Effect: CO2 Adsorption, Luminescence, and Sensing Property. Cryst. Growth Des. 17, 2090–2096. doi:10.1021/acs.cgd.7b00068
Jun, B.-H., Kang, H., Lee, Y.-S., and Jeong, D. H. (2012). Fluorescence-based Multiplex Protein Detection Using Optically Encoded Microbeads. Molecules 17, 2474–2490. doi:10.3390/molecules17032474
Kamat, P. V. (2002). Photophysical, Photochemical and Photocatalytic Aspects of Metal Nanoparticles. J. Phys. Chem. B 106, 7729–7744. doi:10.1021/jp0209289
Kang, M. S., Singh, R. K., Kim, T.-H., Kim, J.-H., Patel, K. D., and Kim, H.-W. (2017). Optical Imaging and Anticancer Chemotherapy through Carbon Dot Created Hollow Mesoporous Silica Nanoparticles. Acta Biomater. 55, 466–480. doi:10.1016/j.actbio.2017.03.054
Kang, Y.-S., Lu, Y., Chen, K., Zhao, Y., Wang, P., and Sun, W.-Y. (2019). Metal-organic Frameworks with Catalytic Centers: From Synthesis to Catalytic Application. Coord. Chem. Rev. 378, 262–280. doi:10.1016/j.ccr.2018.02.009
Kaur, H., Sundriyal, S., Pachauri, V., Ingebrandt, S., Kim, K.-H., Sharma, A. L., et al. (2019). Luminescent Metal-Organic Frameworks and Their Composites: Potential Future Materials for Organic Light Emitting Displays. Coord. Chem. Rev. 401, 213077. doi:10.1016/j.ccr.2019.213077
Kempahanumakkagari, S., Vellingiri, K., Deep, A., Kwon, E. E., Bolan, N., and Kim, K.-H. (2018). Metal-organic Framework Composites as Electrocatalysts for Electrochemical Sensing Applications. Coord. Chem. Rev. 357, 105–129. doi:10.1016/j.ccr.2017.11.028
Kim, H. M., Kim, D. M., Jeong, C., Park, S. Y., Cha, M. G., Ha, Y., et al. (2018). Assembly of Plasmonic and Magnetic Nanoparticles with Fluorescent Silica Shell Layer for Tri-functional SERS-Magnetic-Fluorescence Probes and its Bioapplications. Sci. Rep. 8 (1), 1–10. doi:10.1038/s41598-018-32044-7
Kustov, L. M., Isaeva, V. I., Přech, J., and Bisht, K. K. (2019). Metal-organic Frameworks as Materials for Applications in Sensors. Mendeleev Commun. 29, 361–368. doi:10.1016/j.mencom.2019.07.001
Kwok, R. T. K., Leung, C. W. T., Lam, J. W. Y., and Tang, B. Z. (2015). Biosensing by Luminogens with Aggregation-Induced Emission Characteristics. Chem. Soc. Rev. 44, 4228–4238. doi:10.1039/c4cs00325j
Lakowicz, J. R. (2013). Principles of Fluorescence Spectroscopy. New York: Springer science & business media.
Lamansky, S., Djurovich, P., Murphy, D., Abdel-Razzaq, F., Lee, H.-E., Adachi, C., et al. (2001). Highly Phosphorescent Bis-Cyclometalated Iridium Complexes: Synthesis, Photophysical Characterization, and Use in Organic Light Emitting Diodes. J. Am. Chem. Soc. 123, 4304–4312. doi:10.1021/ja003693s
Landi, G., Fahrner, W. R., Concilio, S., Sessa, L., and Neitzert, H. C. (2014). Electrical Hole Transport Properties of an Ambipolar Organic Compound with Zn-Atoms on a Crystalline Silicon Heterostructure. IEEE J. Electron. Devices Soc. 2, 179–181. doi:10.1109/jeds.2014.2346584
Lei, Z., Zhou, J., Liang, M., Xiao, Y., and Liu, Z. (2021). Aggregation-Induced Emission of Au/Ag Alloy Nanoclusters for Fluorescence Detection of Inorganic Pyrophosphate and Pyrophosphatase Activity. Front. Bioeng. Biotechnol. 8, 1530. doi:10.3389/fbioe.2020.628181
Levina, A., and Lay, P. A. (2005). Mechanistic Studies of Relevance to the Biological Activities of Chromium. Coord. Chem. Rev 249 (3), 281–298. doi:10.1016/j.ccr.2004.02.017
Li, Y., and Song, D. (2011). Syntheses, Structures and Luminescent Properties of Decorated Lanthanide Metal-Organic Frameworks of (E)-4,4′-(ethene-1,2-diyl)dibenzoic Acids. CrystEngComm 13, 1821–1830. doi:10.1039/c0ce00750a
Li, J., Wang, H., Yuan, X., Zhang, J., and Chew, J. W. (2020a). Metal-organic Framework Membranes for Wastewater Treatment and Water Regeneration. Coord. Chem. Rev. 404, 213116. doi:10.1016/j.ccr.2019.213116
Li, L., Zou, J.-Y., and You, S.-Y. (2020b). A Luminescent Pillar-Layer Zn(II) Metal-Organic Framework for the Ultrasensitive Detection of Nitroaniline. Inorg. Chim. Acta 509, 119703. doi:10.1016/j.ica.2020.119703
Li, X., Xie, S., Hu, Y., Xiang, J., Wang, L., Li, R., et al. (2021). AIEgen Modulated Per-Functionalized Flower-like IRMOF-3 Frameworks with Tunable Light Emission and Excellent Sensing Properties. Chem. Commun. 57, 2392–2395. doi:10.1039/d0cc08403d
Lin, Y., Kong, C., Zhang, Q., and Chen, L. (2017). Metal-Organic Frameworks for Carbon Dioxide Capture and Methane Storage. Adv. Energ. Mater. 7, 1601296. doi:10.1002/aenm.201601296
Lin, H.-Y., Tian, Y., Liang, S., Cui, Z.-W., Liu, G.-C., and Wang, X.-L. (2019). A Multi-Responsive Luminescent Sensor Based on Flexible and Ultrastable Zn-MOF@SWCNT Hybrid Nanocomposite Film. Polyhedron 160, 68–73. doi:10.1016/j.poly.2018.12.025
Lin, R.-B., Xiang, S., Zhou, W., and Chen, B. (2020). Microporous Metal-Organic Framework Materials for Gas Separation. Chem 6, 337–363. doi:10.1016/j.chempr.2019.10.012
Liu, Z.-Q., Chen, K., Zhao, Y., Kang, Y.-S., Liu, X.-H., Lu, Q.-Y., et al. (2018). Structural Diversity and Sensing Properties of Metal-Organic Frameworks with Multicarboxylate and 1H-Imidazol-4-Yl-Containing Ligands. Cryst. Growth Des. 18, 1136–1146. doi:10.1021/acs.cgd.7b01572
Liu, J.-Q., Luo, Z.-D., Pan, Y., Kumar Singh, A., Trivedi, M., and Kumar, A. (2020). Recent Developments in Luminescent Coordination Polymers: Designing Strategies, Sensing Application and Theoretical Evidences. Coord. Chem. Rev. 406, 213145. doi:10.1016/j.ccr.2019.213145
Liu, J., Wu, M., Zhang, R., and Xu, Z. P. (2021). Oxygen-derived Free Radicals: Production, Biological Importance, Bioimaging, and Analytical Detection with Responsive Luminescent Nanoprobes. VIEW n/a, 20200139. doi:10.1002/VIW.20200139
Lu, L., He, J., Wang, J., Wu, W.-P., Li, B., Singh, A., et al. (2019). Luminescent Sensing and Photocatalytic Degradation in a New 3D Zn(II)-based Highly Luminescent Metal−Organic Framework. J. Mol. Struct. 1179, 612–617. doi:10.1016/j.molstruc.2018.10.099
Lv, R., Li, H., Su, J., Fu, X., Yang, B., Gu, W., et al. (2017). Zinc Metal-Organic Framework for Selective Detection and Differentiation of Fe(III) and Cr(VI) Ions in Aqueous Solution. Inorg. Chem. 56, 12348–12356. doi:10.1021/acs.inorgchem.7b01822
Macairan, J. R., Zhang, I., Clermont‐Paquette, A., Naccache, R., and Maysinger, D. (2020). Ratiometric pH Sensing in Living Cells Using Carbon Dots. Part. Part. Syst. Charact. 37, 1900430. doi:10.1002/ppsc.201900430
Macrae, C. F., Sovago, I., Cottrell, S. J., Galek, P. T. A., Mccabe, P., Pidcock, E., et al. (2020). Mercury 4.0: from Visualization to Analysis, Design and Prediction. J. Appl. Cryst. 53, 226–235. doi:10.1107/s1600576719014092
Marchenko, R. D., Lysova, A. A., Samsonenko, D. G., Dybtsev, D. N., and Potapov, A. S. (2020). Synthesis, Structural Diversity, Luminescent Properties and Antibacterial Effects of Cadmium(II) and Silver(I) Coordination Compounds with Bis(1,2,3-Benzotriazol-1-Yl)alkanes. Polyhedron 177, 114330. doi:10.1016/j.poly.2019.114330
Martynov, V. I., Pakhomov, A. A., Deyev, I. E., and Petrenko, A. G. (2018). Genetically Encoded Fluorescent Indicators for Live Cell pH Imaging. Biochim. Biophys. Acta (Bba) - Gen. Subj. 1862, 2924–2939. doi:10.1016/j.bbagen.2018.09.013
Mittal, A. K., and Banerjee, U. C. (2016). “Current Status and Future Prospects of Nanobiomaterials in Drug Delivery,” in Nanobiomaterials in Drug Delivery. Editors A. M. Grumezescu (Amsterdam, Netherland: Elsevier), 147–170. doi:10.1016/b978-0-323-42866-8.00005-8
Mukhopadhyay, A., Jindal, S., Savitha, G., and Moorthy, J. N. (2020). Temperature-Dependent Emission and Turn-Off Fluorescence Sensing of Hazardous "Quat" Herbicides in Water by a Zn-MOF Based on a Semi-rigid Dibenzochrysene Tetraacetic Acid Linker. Inorg. Chem. 59, 6202–6213. doi:10.1021/acs.inorgchem.0c00307
Nakabayashi, T., and Ohta, N. (2015). Sensing of Intracellular Environments by Fluorescence Lifetime Imaging of Exogenous Fluorophores. Anal. Sci. 31, 275–285. doi:10.2116/analsci.31.275
Nicewarner-Pena, S. R., Freeman, R. G., Reiss, B. D., He, L., Peña, D. J., Walton, I. D., et al. (2001). Submicrometer Metallic Barcodes. Science 294, 137–141. doi:10.1126/science.294.5540.137
Ostrowski, S. R., Wilbur, S., Chou, C.-H. S. J., Pohl, H. R., Stevens, Y.-W., Allred, P. M., et al. (1999). Agency for Toxic Substances and Disease Registry's 1997 Priority List of Hazardous Substances. Latent Effects-Carcinogenesis, Neurotoxicology, and Developmental Deficits in Humans and Animals. Toxicol. Ind. Health 15, 602–644. doi:10.1191/074823399678847005
Pamei, M., and Puzari, A. (2019). Luminescent Transition Metal-Organic Frameworks: An Emerging Sensor for Detecting Biologically Essential Metal Ions. Nano-Struct. Nano-Objects 19, 100364. doi:10.1016/j.nanoso.2019.100364
Pan, Y., Wang, J., Guo, X., Liu, X., Tang, X., and Zhang, H. (2018). A New Three-Dimensional Zinc-Based Metal-Organic Framework as a Fluorescent Sensor for Detection of Cadmium Ion and Nitrobenzene. J. Colloid Interf. Sci. 513, 418–426. doi:10.1016/j.jcis.2017.11.034
Panunzi, B., Concilio, S., Diana, R., Shikler, R., Nabha, S., Piotto, S., et al. (2018a). Photophysical Properties of Luminescent Zinc(II)-Pyridinyloxadiazole Complexes and Their Glassy Self-Assembly Networks. Eur. J. Inorg. Chem. 2018, 2709–2716. doi:10.1002/ejic.201800344
Panunzi, B., Diana, R., Concilio, S., Sessa, L., Shikler, R., Nabha, S., et al. (2018b). Solid-state Highly Efficient Dr Mono and Poly-Dicyano-Phenylenevinylene Fluorophores. Molecules 23, 1505. doi:10.3390/molecules23071505
Panunzi, B., Diana, R., and Caruso, U. (2019a). A Highly Efficient white Luminescent Zinc (II) Based Metallopolymer by RGB Approach. Polymers (Basel) 11, 1712. doi:10.3390/polym11101712
Panunzi, B., Diana, R., Concilio, S., Sessa, L., Tuzi, A., Piotto, S., et al. (2019b). Fluorescence pH-dependent Sensing of Zn(II) by a Tripodal Ligand. A Comparative X-ray and DFT Study. J. Lumin. 212, 200–206. doi:10.1016/j.jlumin.2019.04.052
Park, M. K., Lim, K. S., Park, J. H., Song, J. H., Kang, D. W., Lee, W. R., et al. (2016). Two- and Three-Dimensional Zn(ii) Coordination Polymers Constructed from Mixed Ligand Systems: Interpenetration, Structural Transformation and Sensing Behavior. CrystEngComm 18, 4349–4358. doi:10.1039/c6ce00155f
Parmar, B., Bisht, K. K., Rachuri, Y., and Suresh, E. (2020). Zn(ii)/Cd(ii) Based Mixed Ligand Coordination Polymers as Fluorosensors for Aqueous Phase Detection of Hazardous Pollutants. Inorg. Chem. Front. 7, 1082–1107. doi:10.1039/c9qi01549c
Patra, L., Das, S., Gharami, S., Aich, K., and Mondal, T. K. (2018). A New Multi-Analyte Fluorogenic Sensor for Efficient Detection of Al3+and Zn2+ions Based on ESIPT and CHEF Features. New J. Chem. 42, 19076–19082. doi:10.1039/c8nj03191f
Peng, T., Jia, P., Wang, R., Feng, H., and Han, X.-M. (2020). A Ketone-Functionalized Zn-MOF for Solvent-free Cyanosilylation of Aldehyde and Treatment Activity against Osteosarcoma Trough Increasing Mg63 Cells Autophagy. J. Coord. Chem. 73, 2235–2249. doi:10.1080/00958972.2020.1819538
Petrochenkova, N., Mirochnik, A., Kulikov, A., and Karasev, V. (1997). Structure and Fluorescence Properties of Coordination Polymers of Europium (III) with Pyromellitic Acid. Russ. J. Coord. Chem. C/C Koordinatsionnaia Khimiia 23, 817–819.
Qi, X., Chang, Z., Zhang, D., Binder, K. J., Shen, S., Huang, Y. Y. S., et al. (2017). Harnessing Surface-Functionalized Metal-Organic Frameworks for Selective Tumor Cell Capture. Chem. Mater. 29, 8052–8056. doi:10.1021/acs.chemmater.7b03269
Qin, X., Yang, W., Yang, Y., Gu, D., Guo, D., and Pan, Q. (2020). A Zinc Metal-Organic Framework for Concurrent Adsorption and Detection of Uranium. Inorg. Chem. 59, 9857–9865. doi:10.1021/acs.inorgchem.0c01072
Qu, T.-G., Hao, X.-M., Wang, H., Cui, X.-G., Chen, F., Wu, Y.-B., et al. (2018). A Luminescent 2D Zinc(II) Metal-Organic Framework for Selective Sensing of Fe(III) Ions and Adsorption of Organic Dyes. Polyhedron 156, 208–217. doi:10.1016/j.poly.2018.09.039
Quah, H. S., Chen, W., Schreyer, M. K., Yang, H., Wong, M. W., Ji, W., et al. (2015). Multiphoton Harvesting Metal-Organic Frameworks. Nat. Commun. 6, 7954–7957. doi:10.1038/ncomms8954
Quintard, A., and Rodriguez, J. (2014). Iron Cyclopentadienone Complexes: Discovery, Properties, and Catalytic Reactivity. Angew. Chem. Int. Ed. 53, 4044–4055. doi:10.1002/anie.201310788
Razavi, S. A. A., and Morsali, A. (2020). Metal Ion Detection Using Luminescent-MOFs: Principles, Strategies and Roadmap. Coord. Chem. Rev. 415, 213299. doi:10.1016/j.ccr.2020.213299
Ren, G., Li, Z., Li, M., Liang, Z., Yang, W., Qiu, P., et al. (2018). A Hexanuclear Cluster Based Metal-Organic Framework for Fe3+ Sensing. Inorg. Chem. Commun. 91, 108–111. doi:10.1016/j.inoche.2018.03.016
Roales, J., Moscoso, F., Gámez, F., Lopes-Costa, T., Sousaraei, A., Casado, S., et al. (2017). Preparation of Luminescent Metal-Organic Framework Films by Soft-Imprinting for 2,4-Dinitrotoluene Sensing. Materials 10, 992. doi:10.3390/ma10090992
Roy, E., Nagar, A., Chaudhary, S., and Pal, S. (2020). Advanced Properties and Applications of AIEgens-Inspired Smart Materials. Ind. Eng. Chem. Res. 59, 10721–10736. doi:10.1021/acs.iecr.0c01869
Schira, R., and Latouche, C. (2020). DFT and Hybrid-DFT Calculations on the Electronic Properties of Vanadate Materials: Theory Meets Experiments. New J. Chem. 44, 11602–11607. doi:10.1039/d0nj02316g
Singh, R., and Lillard, J. W. (2009). Nanoparticle-based Targeted Drug Delivery. Exp. Mol. Pathol. 86, 215–223. doi:10.1016/j.yexmp.2008.12.004
Singh, R. K., Patel, K. D., Leong, K. W., and Kim, H.-W. (2017). Progress in Nanotheranostics Based on Mesoporous Silica Nanomaterial Platforms. ACS Appl. Mater. Inter. 9, 10309–10337. doi:10.1021/acsami.6b16505
Singh, R. K., Kurian, A. G., Patel, K. D., Mandakhbayar, N., Lee, N.-H., Knowles, J. C., et al. (2020). Label-Free Fluorescent Mesoporous Bioglass for Drug Delivery, Optical Triple-Mode Imaging, and Photothermal/Photodynamic Synergistic Cancer Therapy. ACS Appl. Bio Mater. 3, 2218–2229. doi:10.1021/acsabm.0c00050
Steinegger, A., Wolfbeis, O. S., and Borisov, S. M. (2020). Optical Sensing and Imaging of pH Values: Spectroscopies, Materials, and Applications. Chem. Rev. 120, 12357–12489. doi:10.1021/acs.chemrev.0c00451
Tawfik, S. A., Isayev, O., Stampfl, C., Shapter, J., Winkler, D. A., and Ford, M. J. (2019). Efficient Prediction of Structural and Electronic Properties of Hybrid 2D Materials Using Complementary DFT and Machine Learning Approaches. Adv. Theor. Simul. 2, 1800128. doi:10.1002/adts.201800128
Tsivadze, A. Y., Aksyutin, O. E., Ishkov, A. G., Knyazeva, M. K., Solovtsova, O. V., Men'shchikov, I. E., et al. (2019). Metal-organic Framework Structures: Adsorbents for Natural Gas Storage. Russ. Chem. Rev. 88, 925–978. doi:10.1070/rcr4873
Tuergong, M., Nizamidin, P., Yimit, A., and Simayi, R. (2019). In Situ growth and Optical Gas Adsorption Performance of Zn(ii) Metal-Organic Framework Membranes at Room Temperature. Analyst 144, 4887–4896. doi:10.1039/c9an00278b
Wang, B.-H., and Yan, B. (2019). A Dye@MOF Crystalline Probe Serving as a Platform for Ratiometric Sensing of Trichloroacetic Acid (TCA), a Carcinogen Metabolite in Human Urine. CrystEngComm 21, 4637–4643. doi:10.1039/c9ce00924h
Wang, Y., Huang, R., Zhang, J., Cheng, G., and Yang, H. (2018). Lanthanide(Tb3+, Eu3+)-Functionalized a New One Dimensional Zn-MOF Composite as Luminescent Probe for Highly Selectively Sensing Fe3+. Polyhedron 148, 178–183. doi:10.1016/j.poly.2018.04.013
Wang, P.-L., Xie, L.-H., Joseph, E. A., Li, J.-R., Su, X.-O., and Zhou, H.-C. (2019). Metal-Organic Frameworks for Food Safety. Chem. Rev. 119, 10638–10690. doi:10.1021/acs.chemrev.9b00257
Wang, D.-G., Liang, Z., Gao, S., Qu, C., and Zou, R. (2020a). Metal-organic Framework-Based Materials for Hybrid Supercapacitor Application. Coord. Chem. Rev. 404, 213093. doi:10.1016/j.ccr.2019.213093
Wang, S., Ouyang, L., Deng, G., Deng, Z., and Wang, S. (2020b). DNA Adsorption on Nanoscale Zeolitic Imidazolate Framework-8 Enabling Rational Design of a DNA-Based Nanoprobe for Gene Detection and Regulation in Living Cells. RSC Adv. 10, 31012–31021. doi:10.1039/d0ra06218a
Wang, Y., Zhang, X., Zhao, Y., Zhang, S., Li, S., Jia, L., et al. (2020c). Three Novel Zn-Based Coordination Polymers: Synthesis, Structure, and Effective Detection of Al3+ and S2− Ions. Molecules 25, 382. doi:10.3390/molecules25020382
Wang, S., Gong, M., Han, X., Zhao, D., Liu, J., Lu, Y., et al. (2021). Embedding Red Emitters in the NbO-type Metal-Organic Frameworks for Highly Sensitive Luminescence Thermometry over Tunable Temperature Range. ACS Appl. Mater. Inter. 13, 11078–11088. doi:10.1021/acsami.1c00635
Wei, Y., Wang, L., Huang, J., Zhao, J., and Yan, Y. (2018). Multifunctional Metallo-Organic Vesicles Displaying Aggregation-Induced Emission: Two-Photon Cell-Imaging, Drug Delivery, and Specific Detection of Zinc Ion. ACS Appl. Nano Mater. 1, 1819–1827. doi:10.1021/acsanm.8b00226
Wu, Y.-Y., Fan, H.-Q., Chen, H.-J., Jiang, L.-X., and Zhang, W.-P. (2021). A Zn(II)-MOF with Suitable Pore Surroundings for Cyanosilylation Reaction and Protective Effect on Bladder Cancer Cells by Regulating miR-130 and CYLD. J. Inorg. Organomet. Polym. 31, 520–527. doi:10.1007/s10904-020-01694-4
Xiao, Y.-J., Liu, F.-H., Zhao, L., and Su, Z.-M. (2015). An Unprecedented Stable Self-Penetrating Metal-Organic Framework with Solvent-dependent Luminescence Properties. Inorg. Chem. Commun. 59, 32–35. doi:10.1016/j.inoche.2015.06.012
Xiao, Z.-Z., Han, L.-J., Wang, Z.-J., and Zheng, H.-G. (2018). Three Zn(ii)-Based MOFs for Luminescence Sensing of Fe3+ and Cr2O72− Ions. Dalton Trans. 47, 3298–3302. doi:10.1039/c7dt04659f
Xie, S., Liu, Q., Zhu, F., Chen, M., Wang, L., Xiong, Y., et al. (2020a). AIE-active Metal-Organic Frameworks: Facile Preparation, Tunable Light Emission, Ultrasensitive Sensing of Copper(ii) and Visual Fluorescence Detection of Glucose. J. Mater. Chem. C 8, 10408–10415. doi:10.1039/d0tc00106f
Xie, X.-X., Yang, Y.-C., Dou, B.-H., Li, Z.-F., and Li, G. (2020b). Proton Conductive Carboxylate-Based Metal-Organic Frameworks. Coord. Chem. Rev. 403, 213100. doi:10.1016/j.ccr.2019.213100
Xin, X.-T., and Cheng, J.-Z. (2018). A Mixed-Ligand Approach for Building a N-Rich Porous Metal-Organic Framework for Drug Release and Anticancer Activity against Oral Squamous Cell Carcinoma. J. Coord. Chem. 71, 3565–3574. doi:10.1080/00958972.2018.1528499
Xing, K., Fan, R., Wang, F., Nie, H., Du, X., Gai, S., et al. (2018). Dual-Stimulus-Triggered Programmable Drug Release and Luminescent Ratiometric pH Sensing from Chemically Stable Biocompatible Zinc Metal-Organic Framework. ACS Appl. Mater. Inter. 10, 22746–22756. doi:10.1021/acsami.8b06270
Xu, T.-Y., Li, J.-M., Han, Y.-H., Wang, A.-R., He, K.-H., and Shi, Z.-F. (2020). A New 3D Four-fold Interpenetrated Dia-like Luminescent Zn(ii)-Based Metal-Organic Framework: the Sensitive Detection of Fe3+, Cr2O72−, and CrO42− in Water, and Nitrobenzene in Ethanol. New J. Chem. 44, 4011–4022. doi:10.1039/c9nj06056a
Yan, Z., Li, X., Fan, Q., Bai, H., Wu, S., Zhang, Z.-F., et al. (2020). A Water-Stable and Biofriendly Zn-MOF with Pyrazine Decorated Pores as 5-Fu Delivery System to Induce Human Ovarian Cancer Cells Apoptosis and Abrogate Their Growth. J. Mol. Struct. 1204, 127477. doi:10.1016/j.molstruc.2019.127477
Yang, Y., Wang, L., Wan, B., Gu, Y., and Li, X. (2019). Optically Active Nanomaterials for Bioimaging and Targeted Therapy. Front. Bioeng. Biotechnol. 7, 320. doi:10.3389/fbioe.2019.00320
Yang, S.-L., Liu, W.-S., Li, G., Bu, R., Li, P., and Gao, E.-Q. (2020). A pH-Sensing Fluorescent Metal-Organic Framework: pH-Triggered Fluorescence Transition and Detection of Mycotoxin. Inorg. Chem. 59, 15421–15429. doi:10.1021/acs.inorgchem.0c02419
Yang, H., Qi, D., Chen, Z., Cao, M., Deng, Y., Liu, Z., et al. (2021). A Zn-Based Metal-Organic Framework as Bifunctional Chemosensor for the Detection of Nitrobenzene and Fe3+. J. Solid State. Chem. 296, 121970. doi:10.1016/j.jssc.2021.121970
Yao, S., Chi, J., Wang, Y., Zhao, Y., Luo, Y., and Wang, Y. (2021). Zn‐MOF Encapsulated Antibacterial and Degradable Microneedles Array for Promoting Wound Healing. Adv. Healthc. Mater. 10, 2100056. doi:10.1002/adhm.202100056
Yin, J.-C., Li, N., Qian, B.-B., Yu, M.-H., Chang, Z., and Bu, X.-H. (2020). Highly Stable Zn-MOF with Lewis Basic Nitrogen Sites for Selective Sensing of Fe3+ and Cr2O72− Ions in Aqueous Systems. J. Coord. Chem. 73, 2718–2727. doi:10.1080/00958972.2020.1830976
Ying, X.-D., Chen, J.-X., Tu, D.-Y., Zhuang, Y.-C., Wu, D., and Shen, L. (2021). Tetraphenylpyrazine-Based Luminescent Metal-Organic Framework for Chemical Sensing of Carcinoids Biomarkers. ACS Appl. Mater. Inter. 13, 6421–6429. doi:10.1021/acsami.0c20893
You, Y., and Nam, W. (2012). Photofunctional Triplet Excited States of Cyclometalated Ir(iii) Complexes: beyond Electroluminescence. Chem. Soc. Rev. 41, 7061–7084. doi:10.1039/c2cs35171d
Zak, P. P., Lapina, V. A., Pavich, T. A., Trofimov, A. V., Trofimova, N. N., and Tsaplev, Y. B. (2017). Luminescent Materials for Modern Light Sources. Russ. Chem. Rev. 86, 831–844. doi:10.1070/rcr4735
Zhang, X., Chen, C., Liu, X., Gao, P., and Hu, M. (2017). Series of Chiral Interpenetrating 3d-4f Heterometallic MOFs: Luminescent Sensors and Magnetic Properties. J. Solid State. Chem. 253, 360–366. doi:10.1016/j.jssc.2017.06.020
Zhang, M.-N., Fan, T.-T., Wang, Q.-S., Han, H.-L., and Li, X. (2018a). Zn/Cd/Cu- Frameworks Constructed by 3,3′-diphenyldicarboxylate and 1,4-Bis(1,2,4-Triazol-1-Yl)butane: Syntheses, Structure, Luminescence and Luminescence Sensing for Metal Ion in Aqueous Medium. J. Solid State. Chem. 258, 744–752. doi:10.1016/j.jssc.2017.12.005
Zhang, Y., Yang, X., and Zhou, H.-C. (2018b). Synthesis of MOFs for Heterogeneous Catalysis via Linker Design. Polyhedron 154, 189–201. doi:10.1016/j.poly.2018.07.021
Zhang, W., Ding, X., Cheng, H., Yin, C., Yan, J., Mou, Z., et al. (2019). Dual-targeted Gold Nanoprism for Recognition of Early Apoptosis, Dual-Model Imaging and Precise Cancer Photothermal Therapy. Theranostics 9, 5610–5625. doi:10.7150/thno.34755
Zhao, D., Cui, Y., Yang, Y., and Qian, G. (2016). Sensing-functional Luminescent Metal-Organic Frameworks. CrystEngComm 18, 3746–3759. doi:10.1039/c6ce00545d
Zhao, D., Yue, D., Jiang, K., Cui, Y., Zhang, Q., Yang, Y., et al. (2017). Ratiometric Dual-Emitting MOF⊃dye Thermometers with a Tunable Operating Range and Sensitivity. J. Mater. Chem. C 5, 1607–1613. doi:10.1039/c6tc05203g
Zhao, S.-N., Wang, G., Poelman, D., and Voort, P. (2018a). Luminescent Lanthanide MOFs: a Unique Platform for Chemical Sensing. Materials 11, 572. doi:10.3390/ma11040572
Zhao, X., Wang, Y., Li, D. S., Bu, X., and Feng, P. (2018b). Metal-Organic Frameworks for Separation. Adv. Mater. 30, 1705189. doi:10.1002/adma.201705189
Zhao, C., Zhao, L., Meng, L., Liu, X., and liu, C. (2019). A Zn-MOF with 8-fold Interpenetrating Structure Constructed with N,N′-bis(4-carbozylbenzyl)-4-aminotoluene Ligands, Sensors and Selective Adsorption of Dyes. J. Solid State. Chem. 274, 86–91. doi:10.1016/j.jssc.2019.03.014
Zhitkovich, A. (2011). Chromium in Drinking Water: Sources, Metabolism, and Cancer Risks. Chem. Res. Toxicol. 24, 1617–1629. doi:10.1021/tx200251t
Zhuang, J., Young, A. P., and Tsung, C. K. (2017). Integration of Biomolecules with Metal-Organic Frameworks. Small 13, 1700880. doi:10.1002/smll.201700880
Zhuang, X., Zhang, X., Zhang, N., Wang, Y., Zhao, L., and Yang, Q. (2019). Novel Multifunctional Zn Metal-Organic Framework Fluorescent Probe Demonstrating Unique Sensitivity and Selectivity for Detection of PA and Fe3+ Ions in Water Solution. Cryst. Growth Des. 19, 5729–5736. doi:10.1021/acs.cgd.9b00704
Keywords: zinc (II) ion, fluorescence, nanoprobes, sensing, supramolecular structures
Citation: Diana R, Sessa L, Concilio S, Piotto S and Panunzi B (2021) Luminescent Zn (II)-Based Nanoprobes: A Highly Symmetric Supramolecular Platform for Sensing of Biological Targets and Living Cell Imaging. Front. Mater. 8:750247. doi: 10.3389/fmats.2021.750247
Received: 30 July 2021; Accepted: 04 October 2021;
Published: 19 October 2021.
Edited by:
Milana C Vasudev, University of Massachusetts Dartmouth, United StatesReviewed by:
Run Zhang, The University of Queensland, AustraliaRajendra Kumar Singh, Institute of Tissue Regeneration Engineering (ITREN), South Korea
Copyright © 2021 Diana, Sessa, Concilio, Piotto and Panunzi. This is an open-access article distributed under the terms of the Creative Commons Attribution License (CC BY). The use, distribution or reproduction in other forums is permitted, provided the original author(s) and the copyright owner(s) are credited and that the original publication in this journal is cited, in accordance with accepted academic practice. No use, distribution or reproduction is permitted which does not comply with these terms.
*Correspondence: Lucia Sessa, bHVjc2Vzc2FAdW5pc2EuaXQ=; Simona Concilio, c2NvbmNpbGlvQHVuaXNhLml0
†These authors have contributed equally to this work and share corresponding authorship