- 1Department of Physics and Electronic Engineering, Tongren University, Tongren, China
- 2Jiangsu Key Laboratory of Micro and Nano Heat Fluid Flow Technology and Energy Application, School of Mathematics and Physics, Suzhou University of Science and Technology, Suzhou, China
- 3Department of Physics, Harbin Institute of Technology, Harbin, China
A nitrogen-doped diamond crystal with (111) orientation was synthesized with an NaN3 additive in the FeNi-C system at a pressure of 6.5 GPa and a temperature of 1,310°C, using the temperature gradient growth (TGG) method. Spectroscopic properties such as the absorption spectrum and the Raman spectrum as well as the Fourier transform infrared (FTIR) spectrum were studied. FTIR spectroscopy of the C-N vibrational modes at 1,344 and 1,130 cm−1 suggested a nitrogen content of 310 ppm. Its nonlinear optical (NLO) response was investigated using the Z-scan technique under the femtosecond regime. Due to the presence of nitrogen defects, the synthesized crystal performed large nonlinear absorption under both 800- and 532-nm wavelength excitations. However, intrinsic diamond only experiences nonlinear refraction under these two wavelength excitations. Its broadband NLO properties indicated that nitrogen-doped diamond crystals were suitable for the application of ultrafast optical devices.
Introduction
Owing to low thermal expansion, high breakdown voltage, high carrier mobility, high NLO coefficients, and stable color centers, diamond attracts much attention as a promising material for many applications that include quantum optical and information processing (Maurer et al., 2012; Yang et al., 2016), nonlinear all-optical switching (Aharonovich et al., 2011; Hausmann et al., 2014), and magnetic sensing (Balasubramanian et al., 2008; Rondin et al., 2014). So far, a lot of efforts have been devoted to optical nonlinearity of intrinsic diamond (type-IIa) (Dadap et al., 1991; Sheik-Bahae et al., 1994; Preuss and Stuke, 1995; Roth and Laenen, 2001; Gagarskiĭ and Prikhod’ko, 2008; Zhang et al., 2017). Due to the broadband gap (5.5 eV), type-IIa diamond just experiences nonlinear refraction attributed to the optical Kerr effect (OKE) under the visible and infrared wavelength excitation (>390 nm). In these cases, no nonlinear absorption is observed. While using ultraviolet and blue region excitation (<390 nm), both nonlinear absorption that originated from two-photon absorption and nonlinear refraction that originated from the OKE should be taken into consideration. Almeida et al. reported third-order optical nonlinearities in type-IIa diamond at the femtosecond regime (Almeida et al., 2017), performed over a broad spectral range, from 0.83 to 4.77 eV (1,500–260 nm). Low absorption losses within its large transmission window make diamond an attractive platform for on-chip photonics. Specially, intrinsic diamond also suffered multi-photon absorption under extremely high light intensity (Kozák et al., 2012; Kononenko et al., 2014; Wang et al., 2018; Kozak et al., 2019; Zukerstein et al., 2019). In addition, nanocrystalline diamond also shows strong NLO properties for the presence of grain boundaries (Preclíková et al., 2010; Trojánek et al., 2010).
The photo-physical properties that include electronic, optical, and thermal properties of diamond can be significantly changed by doping other elements into its structure. For example, insulated intrinsic diamond becomes a p-type semiconductor when doped with boron. When doped with nitrogen, spectroscopic properties such as absorption and emission spectra are easily influenced by the nitrogen vacancy (NV) center formed in the diamond (Wee et al., 2007; Subedi et al., 2019). As for optical nonlinearity, the negatively charged nitrogen vacancy defect center, NV-, in diamond has been investigated theoretically for one- and two-photon absorption properties involving the first excited state with the 3A2-to-3E transition (Lin et al., 2008). Recently, Motojima et al. reported that the effect of NV centers in single-crystal diamond on NLO effects under 800-nm, 40-fs laser pulses. Their results demonstrated that the OKE signal was strongly enhanced for the heavily implanted type-IIa diamond, and the NLO coefficient of the diamond crystal increased with the increase in nitrogen concentration (Motojima et al., 2019). It is worth noting that the enhanced NLO effect existed only near the surface region of diamond for the implanted depth of NV centers was about 30–40 nm in this case.
The nonlinear optical responses of materials are easily affected by synthetic methods, excitation conditions, and so on. Until now, fundamental studies on the third-order optical nonlinearities of nitrogen-doped diamond are still scarce. In order to clarify the modulation mechanism of NV centers on the optical nonlinearities of nitrogen-doped diamond, it is necessary to further illustrate the relationship of NLO properties and the structure of matter for diamond. Herein, we have synthesized a nitrogen-doped diamond crystal with (111) orientation under high-pressure high-temperature (HPHT). The NLO absorption responses of the synthesized diamond crystal were estimated using the Z-scan technique with two excitation wavelengths (532 and 800 nm) under the femtosecond regime. Our results have shown that nitrogen-doped diamond performed strong NLO absorption under 532- and 800-nm wavelengths, respectively. Its broadband NLO responses indicate that nitrogen-doped diamond can be a good candidate for ultrafast NLO devices such as optical limiters.
Materials and Methods
Sample Preparation and Spectroscopic Characterization
A nitrogen-doped diamond crystal with (111) orientation was synthesized with an NaN3 additive in the FeNi-C system at a pressure of 6.5 GPa and a temperature of 1,310°C, using the temperature gradient growth (TGG) method. The detailed synthetic scheme can be found in the literature (Li et al., 2018). It was a 2.05-mm-thick sample cut with its face perpendicular to the crystallographic [111] axis. Two faces of the diamond crystal were polished. The ground state absorption spectrum of the nitrogen-doped diamond crystal was recorded using a Shimadzu UV1800 spectrophotometer. The Raman spectrum and FTIR spectrum of the synthetic crystal were recorded in order to investigate crystallization quality and impurities in diamond structures. All the experiments were measured at room temperature.
Nonlinear Optical Measurements
Due to simple and single-beam measurement, the Z-scan technique is considered as a common method for measuring nonlinear optical coefficients of materials. In our Z-scan technique, the light source was a regeneratively amplified Yb:KGW fiber laser system (Light Conversion, PHAROS-SP) which produced 1-mJ pulses centered at 1,030 nm with a repetition rate of 6 kHz and 190 fs (FWHM). The optical parametric amplifier (OPA, ORPHEUS, Light Conversion) output was tuned to the wavelengths 532 and 800 nm as the test beams with 20-Hz, 190-fs laser pulses in the Z-scan technique. The spatial and temporal distribution of the pulse was nearly a Gaussian profile. The experimental setup was similar to the one in the study by Sheik-Bahae et al. (1990). The thickness of the synthetic diamond crystal is 2.05 mm, satisfying thin sample approximation in nonlinear optical experiments. The diamond crystal placed on the mobile platform was moved along the z-axis with respect to the focal point of a 200-mm focal lens. All beams were measured by using energy detectors (Rjp-765 energy probe) linked to an energy meter (Rj-7620 ENERGY RATIOMETER, laser probe). The experimental data were collected through a GPIB interface. The experimental system was calibrated using benchmark Kerr liquid CS2.
Results and Discussion
Spectroscopic Characterization
The ground state absorption spectrum of the nitrogen-doped diamond crystal is shown in Figure 1. It is well known that the intrinsic diamond exhibits the wide optical transparency range (from the ultraviolet to the far-infrared) for the broadband gap (5.5 eV) (Gagarskiĭ and Prikhod’ko, 2008). From Figure 1, the synthetic diamond crystal performs linear absorption in visible regions due to the presence of nitrogen. Apparently, the linear absorption of the 532-nm wavelength was larger than that of the 800-nm wavelength in the synthetic diamond. Considering the electronic levels of NV centers (Lin et al., 2008), the electrons can be promoted from the ground state 3A2 to excited states 3E through absorbing one photon when using 532-nm wavelength excitation. However, under 800-nm wavelength excitation, the electrons in NV centers could not be promoted from 3A2 to 3E just through absorbing one photon, consistent with its ground state absorption spectrum. The Raman spectrum of the synthesized diamond crystal is presented in Figure 2. In intrinsic diamond (IIa type), its characteristic peaks were located at 1,332.02 cm−1 (Li et al., 2018). As shown in Figure 2, the characteristic peaks of nitrogen-doped diamond are located at 1,331.75 cm−1, and there was a slight shift compared with the IIa-type diamond. Moreover, the bottom areas of the Raman spectrum were very flat, and the peak was sharp, indicating that the synthesized diamond performed at a high quality. The typical FTIR spectrum of the synthesized diamond crystal is presented in Figure 3. Compared with nitrogen-doped diamond, there were no absorption peaks located at 1,130 and 1,344 cm−1 in the FTIR spectrum of intrinsic diamond (Li et al., 2018). For the nitrogen-doped diamond crystal, the absorption peaks located at 1,130 and 1,344 cm−1 in the FTIR spectrum could be assigned to substitutional N impurity. Furthermore, the concentration of N impurity was approximately 310 ppm, which was estimated according to the following formula (Liang et al., 2005):
Open-Aperture Z-Scan
To evaluate the ultrafast NLO properties of the synthesized nitrogen-doped diamond crystal, an open-aperture Z-scan was performed under 190-fs laser pulses with 532- and 800-nm wavelengths, respectively. The energy used in the 532-nm Z-scan measurement was 200 nJ, and the beam waist was nearly 20 um, corresponding to a light intensity of 150 GW/cm2. The energy used in the 800-nm Z-scan measurement was 600 nJ, and the beam waist was nearly 30 um, corresponding to a light intensity of 207 GW/cm2. Due to the short pulse duration (190 fs) and low repetition speed (20 Hz) of our laser, the thermal effect has a negligible contribution to nonlinear absorption because the built-up time for thermal effect is rather long compared to the pulse duration (Yang and Song, 2009; Kovsh et al., 1999). It is well known that open-aperture Z-scan curves represent the NLO absorption property of materials. The open-aperture Z-scan experimental results under 532 and 800 nm are shown in Figures 4, 5, respectively. The occurrence of a valley in open-aperture Z-scan curves indicated that the synthesized nitrogen-doped diamond crystal experienced reverse saturable absorption (RSA) under both 532- and 800-nm wavelengths. It is well known that no NLO absorption is observed in intrinsic diamond under 532- and 800-nm wavelength excitation. In order to generate NLO absorption, intrinsic diamond must absorb multiple photons promoting electrons from the valence band (VB) to the conduction band (CB) under these two wavelengths for its broadband gap (5.5 eV). In these cases, extremely high light intensity is needed. In our experiments, the light intensity was controlled to a low level, and the transition of electrons from the VB to the CB could be ruled out. As a result, the NLO absorption of the synthetic diamond crystal should originate from the presence of nitrogen defects under these two wavelength excitations. When doped with nitrogen, there are many NV centers formed in the synthetic diamond crystal. Each NV center consists of a substitutional nitrogen atom and adjacent lattice vacancy. The ground and excited states are spin triplets (S = 1) with a principle zero-phonon line (ZPL) at 637 nm (1.945 eV) associated with the radiative transition from 3E to 3A2 (Ashfold et al., 2020; Ulbricht and Loh, 2018; Rogers et al., 2015). The energy levels of NV centers in the bandgap of diamond are illustrated in Figure 6. As Figure 6 shows, with the absorption of photons, electrons of the ground state 3A2 are sent to the excited state 3E. From the excited state 3E, electrons can radiatively decay to the ground state with the photon emission in the visible region or non-radiatively decay to the singlet state 1A1, followed by the radiative transition to the metastable single 1E leading to the emission band in the infrared region with the ZPL at 1042 nm (Bourgeois et al., 2005). It is noted that the excited-state energy level of the NV− is about 0.6 eV below that of the conduction band (CB). After absorbing the second photon, instead of decaying back to the ground state (radiatively or non-radiatively), the excited electron can be promoted to the CB. The time scale of the excited state 3E decaying to the ground state 3A2 or the singlet state 1A1 is nearly 10 nanoseconds (Ulbricht and Loh, 2018). In our open-aperture Z-scan measurements, a 190-fs laser pulse is too short compared to the decaying time of the 3E to the 3A2 and the 3E to the 1A1. As a result, the energy levels of the NV− can simply be two states: 3A2 and 3E. Considering the optical nonlinearity of the synthesized diamond crystal, there is a difference in the original mechanism of NLO absorption between the two wavelength (532 and 800 nm) excitations, although a strong RSA effect was observed under two experimental conditions. When using 800-nm wavelength excitation, the electrons of NV centers will be promoted from the 3A2 state to the conduction band after absorbing two photons. This absorption process is called two-photon absorption, with the participation of virtual-level states. However, when using 532-nm wavelength excitation, first, the electrons of NV centers will be promoted from the 3A2 state to the 3E state after absorbing one photon. Then the electrons of the 3E state will be promoted from the 3E state to the conduction band after absorbing another photon. The third-order nonlinearity of the synthetic diamond crystal is deduced by stepwise one-photon absorption in this case, as in a cascade absorption using a long-lived free carrier located in the excited state 3E. According to the Z-scan theory (Sheik-Bahae et al., 1990), we extracted the effective nonlinear absorption coefficient βeff through numerical simulation. The values of the effective third-order nonlinear absorption coefficient βeff under 532- and 800-nm wavelengths are summarized in Table 1. Compared with previous reports (Kononenko et al., 2014; Almeida et al., 2017; Motojima et al., 2019), NLO coefficients of the synthetic diamond crystal were comparable to those of nitrogen-implanted chemical vapor deposition (CVD) diamond and high-purity diamond. Our results showed that the nitrogen-doped diamond crystal performed broadband NLO responses, indicating that it can be used for the application of ultrafast nonlinear devices in the future.
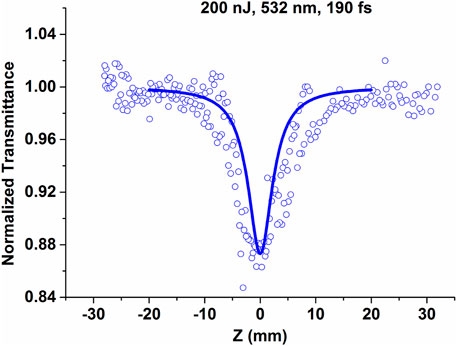
FIGURE 4. Open-aperture Z-scan curves under 532 nm with 200 nJ for the synthetic nitrogen-doped diamond crystal. The circles are the experimental data, and the solid lines are the theoretical fitting.
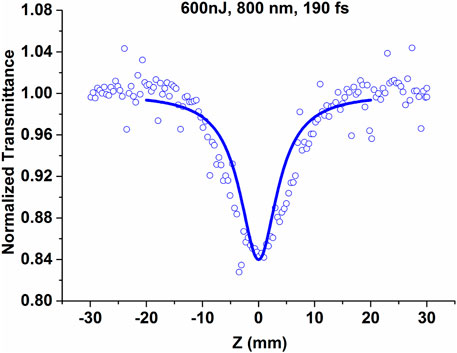
FIGURE 5. Open-aperture Z-scan curves under 800 nm with 600 nJ for the synthetic nitrogen-doped diamond crystal. The circles are the experimental data, and the solid lines are the theoretical fitting.
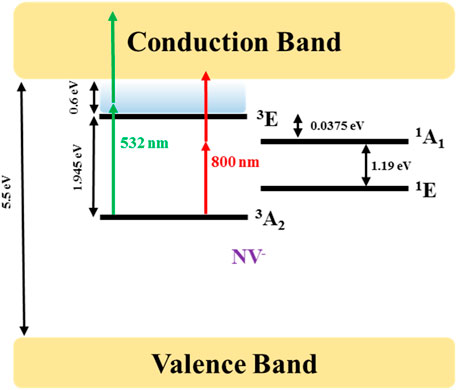
FIGURE 6. Energy levels of NV centers in the bandgap of diamond. The green and red arrow lines represent the possible transition using 532-nm excitation and 800-nm excitation, respectively.

TABLE 1. NLO absorption coefficients of diamonds under different experimental conditions from open-aperture Z-scan measurements.
Conclusion
In summary, a nitrogen-doped diamond crystal with (111) orientation was synthesized with an NaN3 additive in the FeNi-C system at a pressure of 6.5 GPa and a temperature of 1,310°C, using the temperature gradient growth (TGG) method. The FTIR and Raman spectroscopy characterized that the obtained diamond was high quality. FTIR spectroscopy of the C-N vibrational modes at 1,344 and 1,130 cm−1 suggested a nitrogen content of 310 ppm. Its NLO response was investigated using the Z-scan technique under two different wavelengths (532 and 800 nm) at the femtosecond regime. It was found that nonlinear optical absorption originated from the introduction of NV centers, which provided unique optical transitions by electronic structures in the bandgap of diamond. Our results showed that the nitrogen-doped diamond crystal performed broadband NLO responses, indicating its potential applications in future NLO devices, such as ultrafast optical power limiters.
Data Availability Statement
The original contributions presented in the study are included in the article/Supplementary Material; further inquiries can be directed to the corresponding author.
Author Contributions
ZX, YF, and YS contributed to the conception and design of the study. ZX, YL, and LC contributed to the synthesis and testing of samples. YS and CT organized the database and performed the numerical simulation. ZX wrote the first draft of the manuscript. YL and JZ wrote sections of the manuscript. All authors contributed to manuscript revision and read and approved the submitted version.
Conflict of Interest
The authors declare that the research was conducted in the absence of any commercial or financial relationships that could be construed as a potential conflict of interest.
Publisher’s Note
All claims expressed in this article are solely those of the authors and do not necessarily represent those of their affiliated organizations, or those of the publisher, the editors, and the reviewers. Any product that may be evaluated in this article, or claim that may be made by its manufacturer, is not guaranteed or endorsed by the publisher.
Acknowledgments
The authors gratefully acknowledge the support of the National Natural Science Foundation of China (grant no. 12064038), the Science and Technology Foundation of Guizhou Province, China (grant nos. ZK[2021]031 and ZK[2021]019), the Natural Science Foundation of the Guizhou Province Education Department (grant nos. KY[2018]343 and KY[2019]179), the Science and Technology Foundation of Tongren City (grant no. (2017) 47-37), the Startup Foundation for Doctoral Research (trxyDH1622), and the Open Project of the Inner Mongolia Key Lab of High-Pressure Phase Functional Materials (cfxygy202004).
References
Aharonovich, I., Greentree, A. D., and Prawer, S. (2011). Diamond Photonics. Nat. Photon 5 (7), 397–405. doi:10.1038/nphoton.2011.54
Almeida, J. M. P., Oncebay, C., Siqueira, J. P., Muniz, S. R., De Boni, L., and Mendonça, C. R. (2017). Nonlinear Optical Spectrum of diamond at Femtosecond Regime. Sci. Rep. 7, 14320. doi:10.1038/s41598-017-14748-4
Ashfold, M. N. R., Goss, J. P., Green, B. L., May, P. W., Newton, M. E., and Peaker, C. V. (2020). Nitrogen in Diamond. Chem. Rev. 120 (12), 5745–5794. doi:10.1021/acs.chemrev.9b00518
Balasubramanian, G., Chan, I. Y., Kolesov, R., Al-Hmoud, M., Tisler, J., Shin, C., et al. (2008). Nanoscale Imaging Magnetometry with diamond Spins under Ambient Conditions. Nature 455 (7213), 648–651. doi:10.1038/nature07278
Bourgeois, E., Jarmola, A., Siyushev, P., Gulka, M., Hruby, J., Jelezko, F., et al. (2005). Photoelectric Detection of Electron Spin Resonance of Nitrogen-Vacancy Centres in diamond. Nat. Commun. 6, 8577. doi:10.1038/ncomms9577
Dadap, J. I., Focht, G. B., Reitze, D. H., and Downer, M. C. (1991). Two-photon Absorption in diamond and its Application to Ultraviolet Femtosecond Pulse-Width Measurement. Opt. Lett. 16 (7), 499–501. doi:10.1364/ol.16.000499
Gagarskiĭ, S., and Prikhod’ko, K. (2008). Measuring the Parameters of Femtosecond Pulses in a Wide Spectral Range on the Basis of the Multiphoton-Absorption Effect in a Natural diamond crystal. J. Opt. Techn. 75 (3), 139–143.
Hausmann, B. J. M., Bulu, I., Venkataraman, V., Deotare, P., and Lončar, M. (2014). Diamond Nonlinear Photonics. Nat. Photon 8 (5), 369–374. doi:10.1038/nphoton.2014.72
Kononenko, V. V., Konov, V. I., Gololobov, V. M., and Zavedeev, E. V. (2014). Propagation and Absorption of High-Intensity Femtosecond Laser Radiation in diamond. Quan. Electron. 44 (12), 1099–1103. doi:10.1070/qe2014v044n12abeh015459
Kovsh, D. I., Hagan, D. J., and Stryland, E. W. V. (1999). Numerical Modeling of thermal Refraction in Liquids in the Transient Regime. Opt. Express 4 (8), 315–327. doi:10.1364/oe.4.000315
Kozak, M., Otobe, T., Zukerstein, M., Trojanek, F., and Maly, P. (2019). Anisotropy and Polarization Dependence of Multiphoton Charge Carrier Generation Rate in diamond. Phys. Rev. B 99 (10), 104305. doi:10.1103/physrevb.99.104305
Kozák, M., Trojánek, F., Dzurňák, B., and Malý, P. (2012). Two- and Three-Photon Absorption in Chemical Vapor Deposition diamond. J. Opt. Soc. Am. B 29 (5), 1141–1145. doi:10.1364/josab.29.001141
Li, Y., Li, Y., Wang, Y., Zhang, J., Song, M., She, Y., et al. (2018). Synthesis of Large diamond Single Crystals under High Pressure and High Temperature through Effective Utilization of the Synthesis Cavity. CrystEngComm 20 (29), 4127–4132. doi:10.1039/c8ce00786a
Liang, Z., Jia, X., Ma, H., Zang, C., Zhu, P., Guan, Q., et al. (2005). Synthesis of HPHT diamond Containing High Concentrations of Nitrogen Impurities Using NaN3 as Dopant in Metal-Carbon System. Diamond Relat. Mater. 14 (11-12), 1932–1935. doi:10.1016/j.diamond.2005.06.041
Lin, C.-K., Wang, Y.-H., Chang, H.-C., Hayashi, M., and Lin, S. H. (2008). One- and Two-Photon Absorption Properties of diamond Nitrogen-Vacancy Defect Centers: A Theoretical Study. J. Chem. Phys. 129 (12), 124714. doi:10.1063/1.2987717
Maurer, P. C., Kucsko, G., Latta, C., Jiang, L., Yao, N. Y., Bennett, S. D., et al. (2012). Room-temperature Quantum Bit Memory Exceeding One Second. Science 336 (6086), 1283–1286. doi:10.1126/science.1220513
Motojima, M., Suzuki, T., Shigekawa, H., Kainuma, Y., An, T., and Hase, M. (2019). Giant Nonlinear Optical Effects Induced by Nitrogen-Vacancy Centers in diamond Crystals. Opt. Express 27 (22), 32217–32227. doi:10.1364/oe.27.032217
Preclíková, J., Kromka, A., Rezek, B., and Malý, P. (2010). Laser-induced Refractive index Changes in Nanocrystalline diamond Membranes. Opt. Lett. 35 (4), 577–579. doi:10.1364/ol.35.000577
Preuss, S., and Stuke, M. (1995). Subpicosecond Ultraviolet Laser Ablation of diamond: Nonlinear Properties at 248 Nm and Time‐resolved Characterization of Ablation Dynamics. Appl. Phys. Lett. 67 (3), 338–340. doi:10.1063/1.115437
Rogers, L. J., Doherty, M. W., Barson, M. S. J., Onoda, S., Ohshima, T., and Manson, N. B. (2015). Singlet Levels of the NV−centre in diamond. New J. Phys. 17 (1), 013048. doi:10.1088/1367-2630/17/1/013048
Rondin, L., Tetienne, J.-P., Hingant, T., Roch, J.-F., Maletinsky, P., and Jacques, V. (2014). Magnetometry with Nitrogen-Vacancy Defects in diamond. Rep. Prog. Phys. 77 (5), 056503. doi:10.1088/0034-4885/77/5/056503
Roth, T., and Laenen, R. (2001). Absorption of Free Carriers in diamond Determined from the Visible to the Mid-infrared by Femtosecond Two-Photon Absorption Spectroscopy. Opt. Commun. 189 (4), 289–296. doi:10.1016/s0030-4018(01)01037-9
Sheik-Bahae, M., DeSalvo, R. J., Said, A. A., Hagan, D. J., Soileau, M., and Van Stryland, E. W. (1994). Optical Nonlinearities in diamond Proc Laser-Induced Damage in Optical Materials. Albuquerque: International Society for Optics and Photonics, 605–609.
Sheik-Bahae, M., Said, A. A., Wei, T.-H., Hagan, D. J., and Van Stryland, E. W. (1990). Sensitive Measurement of Optical Nonlinearities Using a Single Beam. IEEE J. Quan. Electron. 26 (4), 760–769. doi:10.1109/3.53394
Subedi, S. D., Fedorov, V. V., Peppers, J., Martyshkin, D. V., Mirov, S. B., Shao, L., et al. (2019). Laser Spectroscopic Characterization of Negatively Charged Nitrogen-Vacancy (NV−) Centers in diamond. Opt. Mater. Express 9 (5), 2076–2087. doi:10.1364/ome.9.002076
Trojánek, F., Žídek, K., Dzurňák, B., Kozák, M., and Malý, P. (2010). Nonlinear Optical Properties of Nanocrystalline diamond. Opt. Express 18 (2), 1349–1357. doi:10.1364/oe.18.001349
Ulbricht, R., and Loh, Z. H. (2018). Excited-state Lifetime of the NV- Infrared Transition in diamond. Phys. Rev. B 98 (9), 094309. doi:10.1103/physrevb.98.094309
Wang, Y., Zhang, W., Lin, C.-N., Ren, P., Lu, Y.-J., Shan, C., et al. (2018). Multiphoton Upconversion Emission from Diamond Single Crystals. ACS Appl. Mater. Inter. 10 (22), 18935–18941. doi:10.1021/acsami.8b07288
Wee, T.-L., Tzeng, Y.-K., Han, C.-C., Chang, H.-C., Fann, W., Hsu, J.-H., et al. (2007). Two-photon Excited Fluorescence of Nitrogen-Vacancy Centers in Proton-Irradiated Type Ib Diamond†. J. Phys. Chem. A. 111 (38), 9379–9386. doi:10.1021/jp073938o
Yang, J., and Song, Y. (2009). Direct Observation of the Transient thermal-lensing Effect Using the Phase-Object Z-Scan Technique. Opt. Lett. 34 (2), 157–159. doi:10.1364/ol.34.000157
Yang, S., Wang, Y., Rao, D. D. B., Hien Tran, T., Momenzadeh, A. S., Markham, M., et al. (2016). High-fidelity Transfer and Storage of Photon States in a Single Nuclear Spin. Nat. Photon 10 (8), 507–511. doi:10.1038/nphoton.2016.103
Zhang, B., Liu, S., Wu, X., Yi, T., Fang, Y., Zhang, J., et al. (2017). Ultrafast Dynamics of Carriers and Nonlinear Refractive index in Bulk Polycrystalline diamond. Optik 130, 1073–1079. doi:10.1016/j.ijleo.2016.11.107
Keywords: nitrogen-doped diamond, broadband NLO response, Z-scan technique, ultrafast optical devices, TGG method
Citation: Xiao Z, Fang Y, Song Y, She Y, Tian C, Zhang J, Cui L and Li Y (2021) Broadband Nonlinear Optical Response of Nitrogen-Doped Diamond. Front. Mater. 8:739448. doi: 10.3389/fmats.2021.739448
Received: 11 July 2021; Accepted: 20 August 2021;
Published: 13 September 2021.
Edited by:
Zhongquan Nie, Taiyuan University of Technology, ChinaReviewed by:
Zhongguo Li, Changshu Institute of Technology, ChinaXiao Jin, Lingnan Normal University, China
Copyright © 2021 Xiao, Fang, Song, She, Tian, Zhang, Cui and Li. This is an open-access article distributed under the terms of the Creative Commons Attribution License (CC BY). The use, distribution or reproduction in other forums is permitted, provided the original author(s) and the copyright owner(s) are credited and that the original publication in this journal is cited, in accordance with accepted academic practice. No use, distribution or reproduction is permitted which does not comply with these terms.
*Correspondence: Yong Li, bGlrYWl5b25nNkAxNjMuY29t