- 1The State Key Laboratory Breeding Base of Basic Science of Stomatology (Hubei-MOST) and Key Laboratory for Oral Biomedicine of Ministry of Education (KLOBM), School and Hospital of Stomatology, Wuhan University, Wuhan, China
- 2KU Leuven (University of Leuven), Department of Oral Health Sciences, BIOMAT-Biomaterials Research group and UZ Leuven, University Hospitals Leuven, Leuven, Belgium
- 3Department of Endodontics, School and Hospital of Stomatology, Wuhan University, Wuhan, China
- 4Department of Dentistry, Chung Shan Medical University Hospital, Taichung City, Taiwan
- 5Institute of Oral Science, Chung Shan Medical University, Taichung City, Taiwan
This study was designed to comparatively evaluate an experimental pulp-capping agent radiopaque dicalcium silicate cement (RDSC), white-colored mineral trioxide aggregate (WMTA), iRoot BP Plus and Biodentine both ex vivo and in vivo. Fourier Transform infrared spectroscopy (FTIR) and X-ray diffraction (XRD) were utilized to analyze the phase composition of pulp-capping agents. The surface morphology and element analysis were finished by Scanning electron microscopy (SEM) and Energy Dispersive X-Ray Spectroscopy (EDX). Color stability of the cements and pulp responses induced by different cements were examined by ex-vivo human tooth culture model and in-vivo miniature pig model. Micro-computed tomography was used for quantitative evaluation of the newly formed mineralized tissue. RDSC exhibited discoloration in both models, while WMTA exhibited more visible discoloration. The occurrence of discoloration for WMTA was significantly higher than that of RDSC (p < 0.05), iRoot BP Plus and Biodentine on the 7th day, also higher (p < 0.05) than that of iRoot BP Plus and Biodentine on the 70th day. Mineralized foci were noted in all 7-days groups except the control group both ex vivo and in vivo. Intact and complete reparative dentin bridge formation which possessed tubular structures was noted in all 70-days groups in vivo. RDSC appeared to induce the thickest dentin bridge formation among all these pulp-capping agents which showed statistically significant difference to that of Biodentine (p < 0.05). With decent biological properties, RDSC may have the potential to be a pulp-capping agent recommended in the non-aesthetic area alternative to WMTA, Biodentine and iRoot BP Plus.
Introduction
For the last decades, calcium hydroxide is considered as the gold standard for pulp capping materials. In the 1990s, mineral trioxide aggregate (MTA), which possessed better survival rate than calcium hydroxide (Aeinehchi et al., 2003; Dammaschke et al., 2010; Hilton et al., 2013), was originally produced and seemed to become a new gold standard. Since then, hydraulic calcium silicate cements (hCSCs) are getting popular thanks to their biocompatibility, and odontogenic ability (Aeinehchi et al., 2003; Lee et al., 2014).
Nevertheless, no material on the market can conquer all the ideal characteristics that a pulp capping material should possess by far. MTA has been found hard to handle in clinic because of a long setting time (Chiang and Ding, 2010; Kaup et al., 2015; Guo et al., 2016). Furthermore, a potential tooth discoloration restricts the application of MTA in the aesthetic zone (Jang et al., 2013; Vallés et al., 2013; Wu et al., 2013; Marciano et al., 2014; Kang et al., 2015; Yoldaş et al., 2016; Marciano et al., 2017). Some properties of other widely used hCSCs pulp capping materials such as Biodentine (Septodont, Saint Maur des Fosses, France) and iRoot BP Plus (Innovative Bioceramix, Vancouver, Canada) are also not ideal. According to ISO 6876, the radiopacity of pulp capping material is suggested to be more than 3 mm of aluminum. Biodentine demonstrated an inadequate radiopacity which is found less radiopaque than MTA (Grech et al., 2013; Tanalp et al., 2013; Kaup et al., 2015; Elsaka et al., 2019). The setting time of iRoot BP Plus is approximately 2 h according to manufacturer’s instruction, which is far from satisfaction. Moreover, the long-term in-vivo efficacy of iRoot BP Plus is poorly investigated.
To solve the shortcomings of hCSCs based pulp capping materials, an experimental radiopaque dicalcium silicate cement (RDSC) was developed. Our previous study showed that RDSC performed a shorter setting time (24 min) than that of WMTA (Dentsply Tulsa Dental, Tulsa, OK, United States) (168 min) and a decent radiopacity (7.3 mm aluminum) was observed (Chiang and Ding, 2010). In addition, RDSC exhibited a preferable cell viability and appeared to be less cytotoxic than WMTA in MG63 and L929 cell lines, respectively, (Chiang and Ding, 2010; Wu et al., 2015). No significant differences were found between RDSC and WMTA in terms of antibacterial activity against E. faecalis (Chen et al., 2011; Wu et al., 2015) and responses of human dental pulp cells (hDPCs) (Chen et al., 2011). In the rabbit femur defect model, RDSC implants showed abundant osteoid and mineralized bone tissue between the cement-tissue interfaces. Quantitative analysis results confirmed that the RDSC implants generated more effective osteogenesis surrounding the bone defect area than WMTA implants. After 1, 3, and 6 months of implantation, RDSC performed color stability when compared with WMTA (Wu et al., 2013). However, the long-term biological efficacy of RDSC needs to be evaluated in vivo using a large animal model and its color stability should be assessed by a tooth model instead of a bone model.
The aim of this study was to evaluate the color stability and pulp repair potential of RDSC compared with WMTA, Biodentine, and iRoot BP Plus. The color stability was evaluated using an ex-vivo human tooth culture model and in-vivo miniature pig model. The histological responses of pulp tissues capped with four different materials were observed after 7 and 70 days of implantation. The null hypothesis was that (Aeinehchi et al., 2003) the color stability of RDSC shows no significant difference than the other three materials and that (Dammaschke et al., 2010) RDSC does not provoke any pulp tissue responses in both ex-vivo and in-vivo models in all observation periods.
Materials and Methods
RDSC Preparation
Tetraethyl orthosilicate (Si(OC2H5)4; Sigma-Aldrich, St. Louis, MO, United States) and calcium nitrate (Ca(NO3)2_4H2O; Showa, Tokyo, Japan) were used as precursors for dicalcium silicate, respectively, and the molar ratio of Si/Ca used in this study was 2:3. A detailed description of the powder’s fabrication using sol-gel methods has been reported (Chen et al., 2009). After sintering at 800°C for 2 h, the granules were then ball-milled for 12 h in ethyl alcohol using a centrifugal ball mill (Retsch S 100, Hann, Germany) and then dried in an oven at 60°C. Bi2O3 (Sigma-Aldrich) was added to the ground powder at 20 wt% using a conditioning mixer (ARE-250; Thinky, Tokyo, Japan). To prepare RDSC specimens, the powder was hand-mixed with distilled water in a liquid-to-powder (L/P) ratio of 0.4 ml/g.
Phase Composition and Microstructure
After mixing according to the manufacturer’s instructions, the cements were placed in a cylindrical Teflon mold to form the cylindrical specimen with a dimension of 14 mm (diameter)* 2 mm (height); the specimens were stored in an incubator at 100% relative humidity and 37°C for 1 day to set. Then, the specimens were transported into a 60°C oven to dry.
To investigate the phase composition, the specimens were ground to fine powders and then characterized with an x-ray diffractometer (XPert Pro, PANalytical, Netherlands). Fourier transform infrared spectroscopy (FTIR5700, Thermo, United States) was used to analyze the powders. Scanning electron microscopy (Zeiss SIGMA, Zeiss, Germany) was used to characterize the microstructure of the various specimens.
Ex-vivo Human Tooth Culture Model
All teeth were collected from the School and Hospital of Stomatology, Wuhan University with informed consent. With the permission of the Institutional Ethical Board of Wuhan University [no. 2017 (1)], we collected and used the human samples based on the guidelines of the National Institutes of Health. Forty-five intact human third molars with open apex extracted for orthodontic reasons were obtained from healthy young patients (15 to 20 years old). The surgery procedure was described previously (Pedano et al., 2020; Téclès et al., 2008). In brief, The teeth were collected immediately after the surgery and transported in centrifuge tubes which contained 10 ml of α-minimum essential medium (α-MEM) supplemented with 1% penicillin-streptomycin and 0.75 μg/ml amphotericin B. After removing remained gingiva, periodontal ligament, and the dental sac with sterilized instruments, teeth were then rinsed with phosphate buffer saline (PBS). A class-I cavity was prepared using a 1.0 mm-diameter carbide bur at high speed with copious cooling. The diameter of the cavity in the enamel layer was approximately 4 mm. Then, the bur was kept working until the penetration to the pulp cavity. Finally, a puncture point was prepared in diameter of approximately 1.0 mm. Afterwards, the cavity was irrigated with sterile saline. Sterile cotton pellets were applied with light pressure for drying. Three commercial pulp capping agents were prepared according to the manufacturer’s instructions and applied at a thickness of approximately 2 mm while no pulp capping agent was applied in the exposed site of control group (Figure 1A). The teeth were divided into 5 groups: 1) RDSC, 2) WMTA, 3) Biodentine, 4) iRoot BP Plus, 5) a negative control (n = 3 for each group). Finally, restoration was completed by glass ionomer and resin. All the samples were then taken into the laboratory and rinsed with sterile PBS for 5 times. The tooth culture medium was α-MEM containing 1% penicillin-streptomycin, 0.75 μg/ml amphotericin B, 10% fetal bovine serum and 2 mM b-glycerophosphate. Then, the samples were hung in the 12-well culture plates and immersed in 4 ml tooth culture medium which was refresh every day. After culture for 1, 7 or 14 days, teeth were rinsed by ddH2O. Pulp capping materials were exposed. Two independent observers who were blinds to the materials evaluated the discoloration of teeth. If the results of two independent observers were different. The teeth would be evaluated by third veteran observer and the evaluation of the third evaluator should prevail. All observers passed the consistency check before the study. Then, the occlusal surfaces and the cervical part of the teeth were photographed.
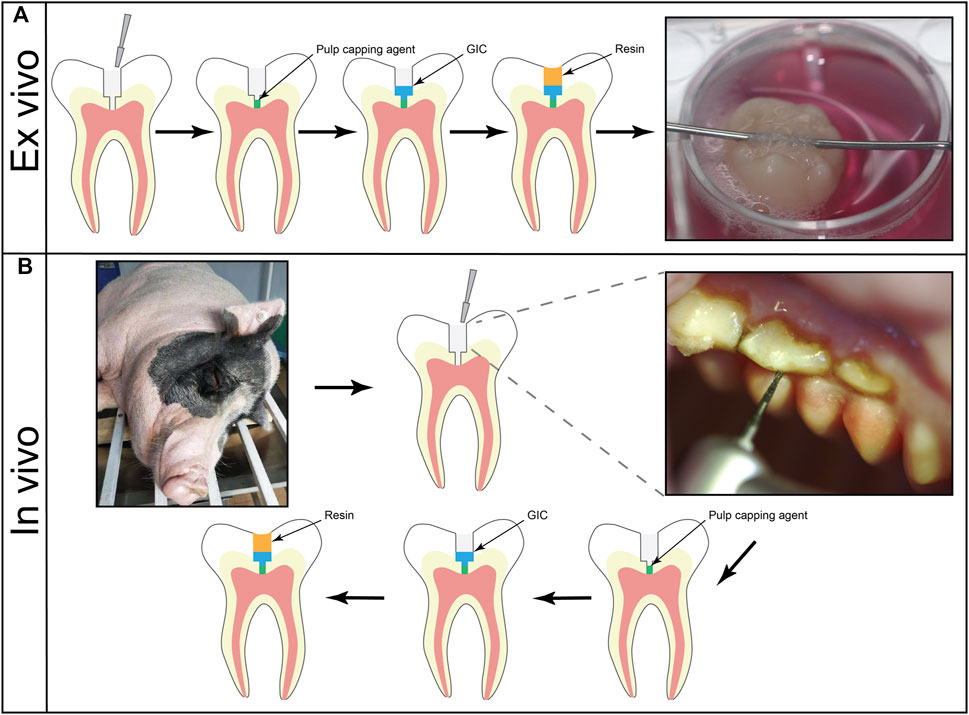
FIGURE 1. (A) Schematic of pulp-capping procedure of ex vivo human tooth culture model. The class-I cavities were prepared on the occlusal surface. After the application of pulp capping agents, restoration was finished by glass ionomer cement and light-curable restorative resin. (B) Schematic of pulp-capping procedure of in vivo miniature pig tooth culture model. Class-I cavities were made on the occlusal surfaces. The cavities were capped by different agents. A layer of glass ionomer cement was implemented before restoration of light-curable resin.
Histological Assessment in Ex-Vivo Human Tooth Culture Model
After culturing, 15 teeth in 7 days groups were immersed in 4% paraformaldehyde (PFA) for 2 days for fixation. The teeth were then decalcified using 10% EDTA and embedded in paraffin blocks. Subsequently, the teeth were sectioned into slices at a thickness of 5 μm, followed by staining of hematoxylin and eosin solution (Servicebio, WuHan, China) and modified Masson’s Trichrome stain kit (maxim, Fu Zhou, China) for the identification of collagen.
In-Vivo Miniature Pig Model
The use of animal model was approved by the Animal Ethics Committee in Wuhan University (protocol00279848). Three female minipigs at the age of 36 months with intact permanent dentitions were used. Each of them was raised in a single cell and fed twice a day with ad libitum access to water.
Before the experiment, the dentition of each miniature pig was scanned using computed tomography to ensure that its teeth were healthy and fully developed. Premolars and molars on the right side were scheduled for 7 days observation group while the ones on the left were for 70 days. Specifically, 70-days group was operated on the first day. After 63 days, 7-days group was scheduled. Then, the animals were sacrificed on the 70th day. Teeth were divided into four material groups: for day 7, 1) RDSC (n = 6, 5 premolars and 1 M), 2) WMTA (n = 6, 5 premolars and 1 M), 3) Biodentine (n = 7, 5 premolars and 2 M), 4) iRoot BP Plus (n = 6, 4 premolars and 2 M); for day 70, 1) RDSC (n = 7, 5 premolars and 2 M), 2) WMTA (n = 5, 4 premolars and 1 M), 3) Biodentine (n = 8, 6 premolars and 2 M), 4) iRoot BP Plus (n = 6, 4 premolars and 2 M), as detailed in Table 1.

TABLE 1. The occurrence rates of discoloration for different materials using in-vivo miniature pig model. Means with different small letters in the same column indicate a significant difference (p < 0.05).
General anesthesia was conducted intramuscularly using Zoletil 50 (0.1–0.15 mg/kg; Virbac, Fort Worth, TX, United States). Under local anesthesia by 2% lidocaine, class-I cavities were prepared on the occlusal surfaces. The diameter of the cavities and exposure site was 3.0 and 1.0 mm, respectively. After rinsing with sterile saline, sterile wet cotton pellets were applied gently to control the hemorrhage. Pulp-capping agents were prepared according to the manufacturers’ instructions. 2 mm thick pulp-capping agents were applied, followed by a layer of glass ionomer cement (ShangChi, Changshu, China). Finally, the cavities were restored with a universal and light-curable restorative composites (Herculite Precis, Kerr, CA, United States) (Figure 1B). Soft diet was then provided for the animals.
After euthanasia, the mandible and maxilla of the miniature pigs were separated, and photographs were taken before 4% PFA fixation. The teeth were then removed individually and immersed in the 4% PFA at 4°C for 2 days.
Micro-Computed Tomography
Teeth were scanned using a μCT scanner (Skyscan 1,176, Bruker Micro-CT, Belgium) with the following parameters: 82 kV/277 μA X-ray source, 0.1 cuprum filter, pixel size of 18 μm, averaging frame of 1, rotation step of 0.5, random movement of 50 and 360°rotation around the vertical axis. The raw data were reconstructed using NRecon software (Bruker Micro-CT).
For quantitative analysis, we used Dataviewer software and CTAn software (Bruker Micro-CT) to measure the thickness of newly formed mineralized tissue (from exposure side to material side) using the full-axial slices dataset. The maximum thickness of newly formed mineralized tissue was recorded. The continuity of mineralized tissue was classified as either “complete”, “partial” or “none” in accordance with both micro-CT and corresponding histological section.
Histological Assessment in Miniature Pig Model
The histological procedures were applied as described in the ex-vivo human tooth culture model. The histological responses were evaluated using digital pathology slide scanner (panoramic midi, 3DHISTECH, Budapest, Hungary).
Statistical Analysis
The occurrence of tooth discoloration was statistically compared using a Chi-square test of independence. One-way analysis of variance statistical analysis was used to evaluate the significance of the differences between the mean values. In all cases, the results were considered statistically significant at a p-value less than 0.05.
Results
Phase Composition and Microstructure
For all specimens, the broad IR absorption band corresponding to O-H stretching vibration extended over a wide wave-number range around 3435 cm−1. Two sharp peaks at 1,628 and 1,473 cm−1 are detected as O-H bending vibration band. Two peaks at 931 and 1,001 cm−1 are ascribed to Si-O stretching vibration (Figure 2A).
The major diffraction peaks at 2θ 27°and 33°were attributed to the bismuth oxide phase (filled circle). For RDSC, two peaks at 2θ 32°were ascribed to the dicalcium silicate phase (filled diamond). For WMTA, Biodentine and iRoot BP plus, two peaks at 2θ 32°and one peak at 2θ 34°were attributed to the tricalcium silicate phase (opened diamond). For WMTA, Biodentine, peak at 2θ 29°were attributed to calcium carbonate phase (filled inverted triangle). For iRoot BP plus, two peaks at 2θ 28°and 31°were ascribed to zirconium oxide (open square) (Figure 2B).
The SEM micrographs of the four cements are shown in Figure 3. All cement specimens had an appearance with entangled particles and exhibited several micropores. The micropores with a near spherical shape were uniformly and separately distributed. However, it seems that WMTA and Biodentine had more micropores than RDSC and iRoot BP plus (Figures 3A–D). The EDX analysis shows that all specimens contain calcium and silicon (Figures 3E–H). In RDSC and WMTA, bismuth was detected due to the introduction of bismuth oxide as radiopacifer (Figures 3E,F). In Biodentine and iRoot BP, zirconium was detected due to the introduction of zirconium oxide as radiopacifer (Figures 3G,H). Aluminum was detected in WMTA (Figure 3F).
Color Stability
In ex-vivo human tooth culture model, RDSC shows no obvious discoloration on day 1 (Figure 4A) and slight discoloration on day 7 (Figures 4E,I). For in-vivo miniature pig model, RDSC shows discoloration on day 7 and it becomes more visible on day 70 (Figures 4M,Q). WMTA exhibites an obvious discoloration in both models, especially on day 70 (Figures 4B,F,J,N,R). No obvious discoloration can be seen at the cervical part of all ex-vivo groups. Biodentine and iRoot BP Plus merely show discoloration except for one tooth in 70 days group (Figures 4C,D,G,H,K,L,O,P,S,T). The occurrences of discoloration for different materials using in vivo miniature pig model were presented in Table 1. At day 7, the discoloration occurrence for WMTA is 100%, which is significantly higher than that of RDSC (33.3%) (p < 0.05), iRoot BP Plus 0% (p < 0.05) and Biodentine (p < 0.05). For 70 days groups, WMTA also show a significantly higher occurrence rate 80% than that of RDSC (57.1%), iRoot BP Plus (16.7%) and Biodentine (12.5%) (p < 0.05).
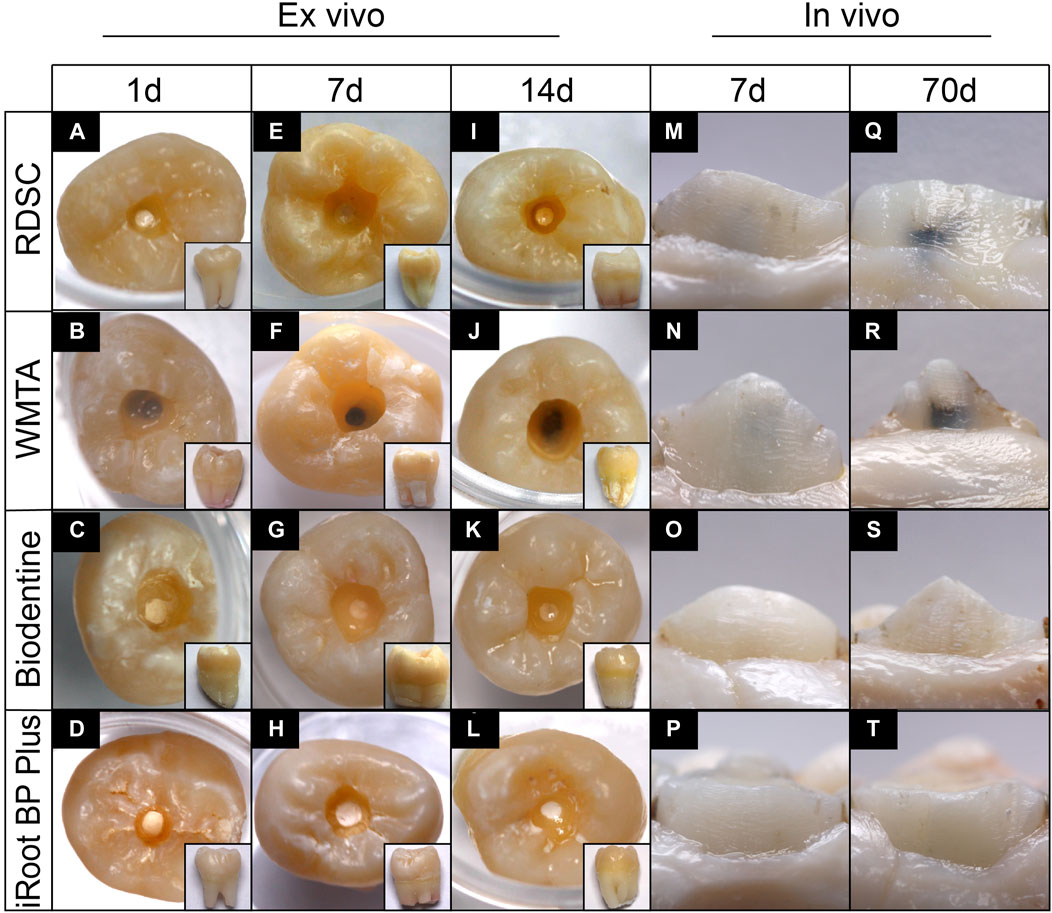
FIGURE 4. Color stability assessment in ex-vivo human tooth culture model and in-vivo miniature pig model. Teeth were capped for 1, 7 or 14-days ex-vivo (A–L) and 7 or 70 days in-vivo (M–T).
Histological Assessment in Ex-Vivo Human Tooth Culture Model
The histological micrographs of pulps after 7 days capping with RDSC, WMTA, Biodentine, iRoot BP Plus and negative control (no capping agent) are shown in Figure 5. In RDSC group, mineral foci are observed underneath the exposed area (arrows in Figure 5A) which would lead to the future formation of a mineralized bridge covering/healing the exposed region. Some of them contained sequestered cells showing an osteodentin aspect. Masson’s staining showed blue-stained area around the mineralized foci which represented the accumulation of collagen fibers. The other pulp-capping agents (Figures 5B–D) also show similar results as RDSC groups. Among them, Biodentine group generates the minimum size of mineral foci (Figure 5C). The control group with no pulp-capping materials exhibits a normal pulp morphology, no necrosis occurred (Figure 5E).
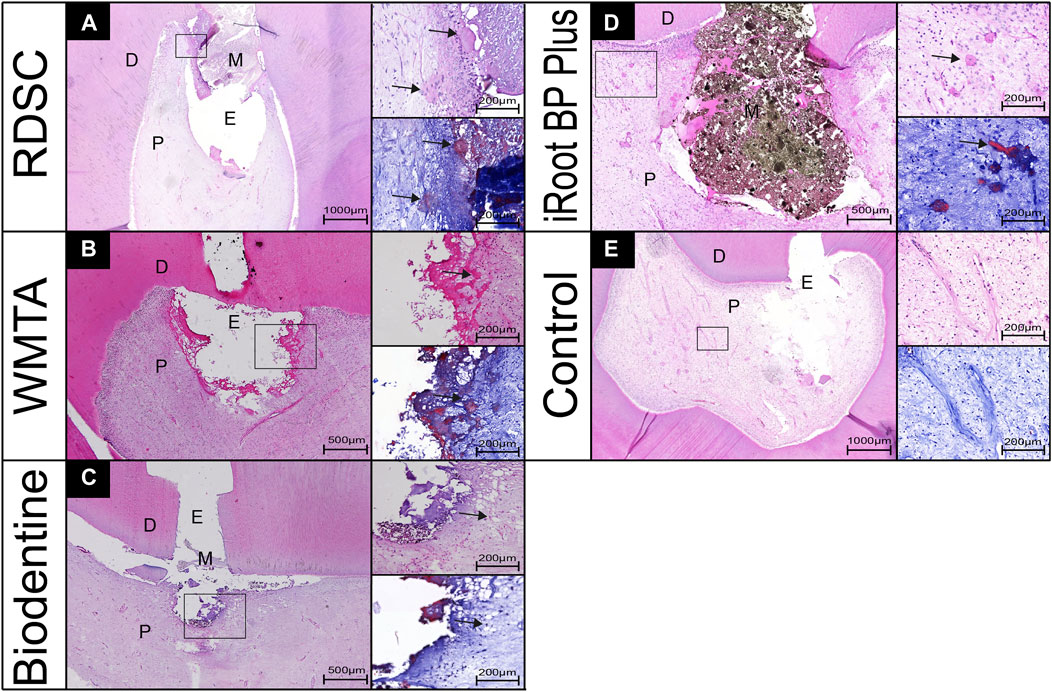
FIGURE 5. Pulp-tissue response of representative teeth after 7 days in the human tooth-culture model, with histological micrographs of pulps that were capped by RDSC (A), WMTA (B), Biodentine (C), iRoot BP Plus (D), or the control (E). (D, dentin; M, materials; E, exposure sites; P, pulp tissue, arrows: mineral foci).
μCT of Pulp Tissue for In-Vivo Miniature Pig Model
Three-dimensional μCT reconstructions of teeth capped with different materials for seven or 70 days are presented in Figure 3. RDSC can be distinctly distinguished from the dentin and high-intensive area is noted underneath the RDSC on day 7 (the arrow in Figure 6A). Similar results are found in WMTA and Biodentine group (arrows in Figures 6B,C). Histological micrographs identify that they are just accumulation of cells and collagen fibers or residual dentin (Figure 7). In contrast to other three materials, Biodentine is not distinct in the μCT photographs (Figure 6C). No high-intensive area is found in the iRoot BP Plus group on day 7. All teeth capped for 70 days exhibite radiopaque layer underneath the materials (arrows in Figures 6E–I). The radiopaque layer is confirmed to be reparative dentin by histological assay (the arrow in Figure 6J). The thickness of the reparative dentin bridge is measured, and RDSC appears to induce the thickest dentin bridge formation (353 μm) among all pulp-capping agents tested in which MTA is 205 mm; iRoot BP Plus is 194 mm; and Biodentine is 124 mm (p < 0.05) (Figure 4K). All teeth in 70 days group are identified as “complete” and confirmed to be reparative dentin (Table 2). Therefore, the exposure sites are all completely sealed.
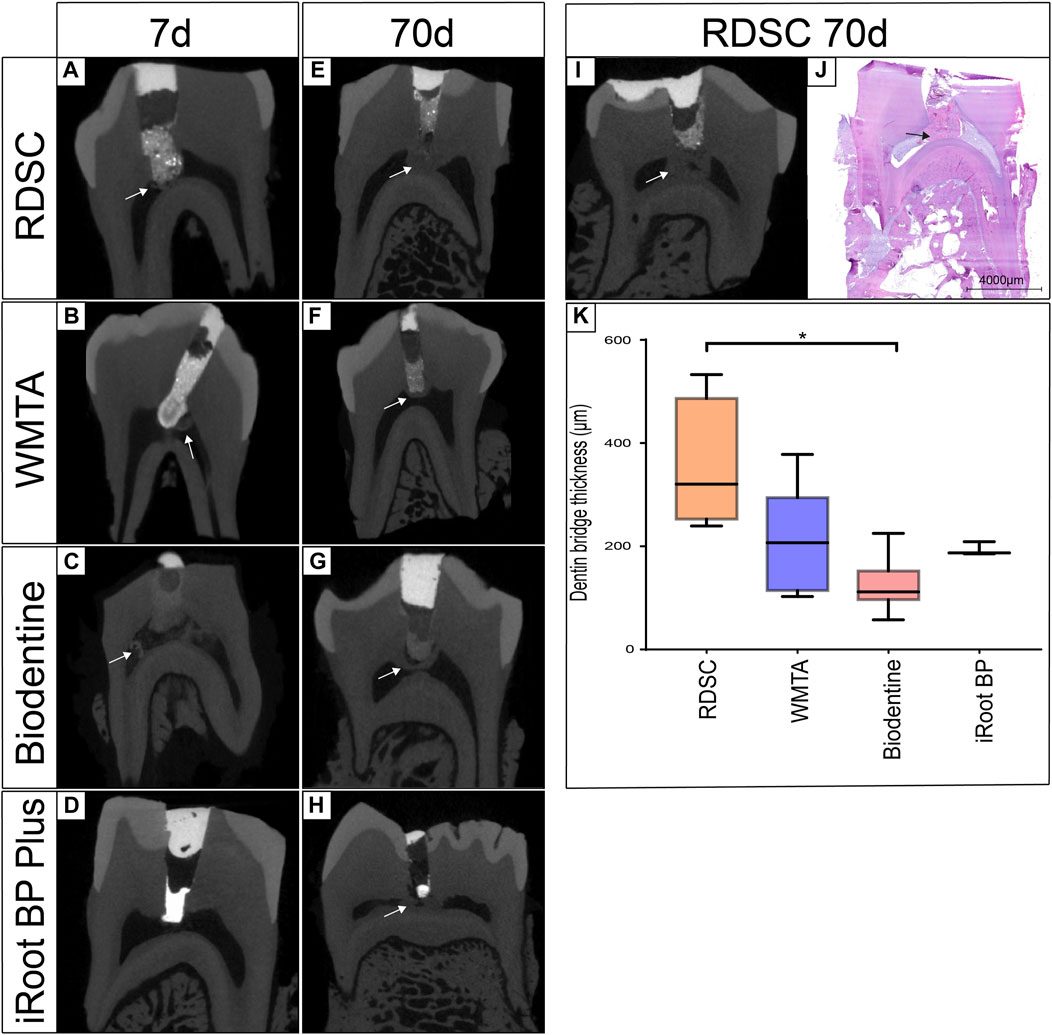
FIGURE 6. Three-dimensional micro-CT reconstruction of teeth capped for 7 or 70 days in the in-vivo miniature pig model by RDSC (A,E,I), WMTA (B,F), Biodentine (C,G) or iRoot BP Plus (D,H). The hard tissue bridge was confirmed to be reparative dentin bridge by histological assay (J). The dentin bridge thickness of each 70 days group was compared by three-dimensional micro-CT reconstruction (K) (* indicates a significant difference, p < 0.05).
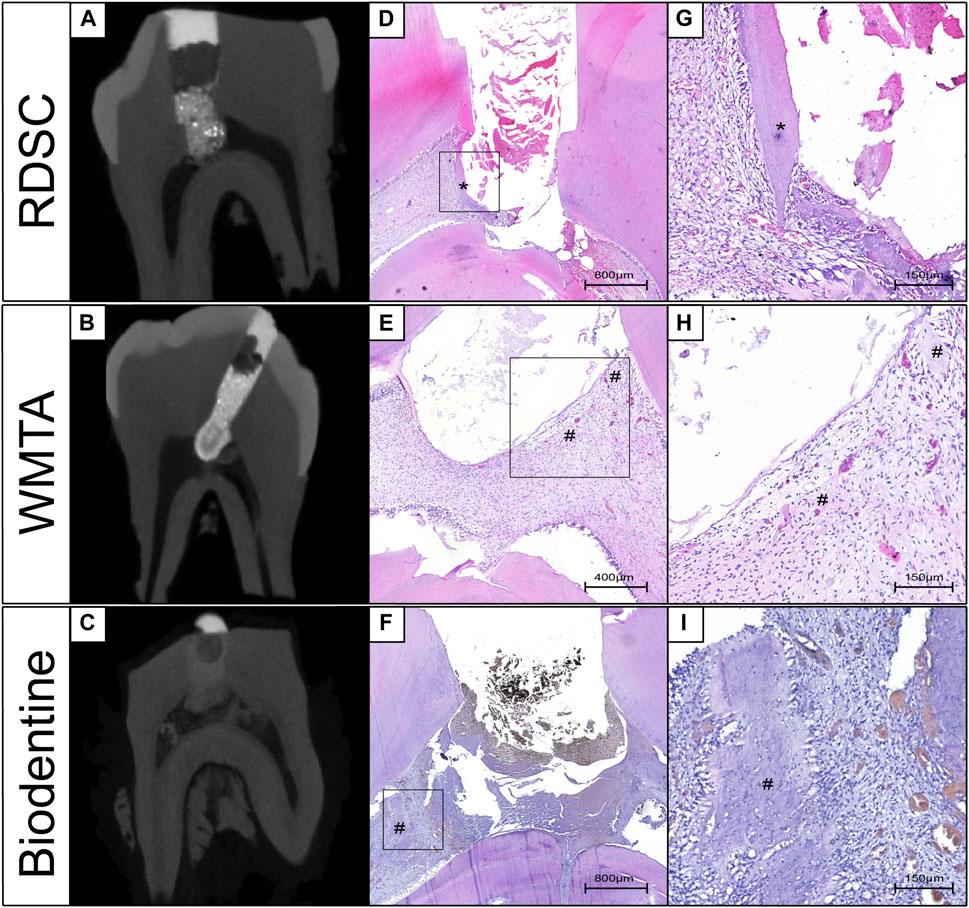
FIGURE 7. Corresponding photographs of Micro-CT and histological assessments in the 7 days in-vivo miniature pig model. The high-intensive area in RDSC groups is the residual dentin (A,D,G). Accumulation of cells and collagen fibers was noted at the high-intensive area of WMTA and Biodentine groups (B–F,H,I) (*: residual dentin, #: Accumulation of cells and collagen fibers).
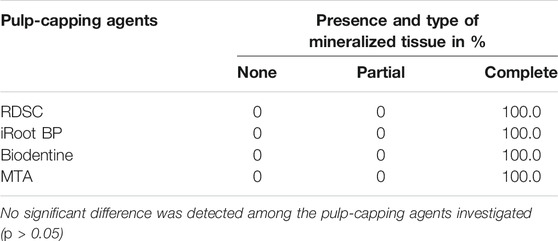
TABLE 2. The continuity of mineralized tissue in 70-days in-vivo miniature pig model. No significant difference was detected among the pulp-capping agents investigated (p > 0.05)
Histological Assessment in Miniature Pig Model
The histological micrographs of pulps after 7 and 70 days capping with RDSC, WMTA, Biodentine, iRoot BP Plus and negative control (no capping agent) were shown in Figure 8. The result of 7 days group is in consistent with that of ex-vivo human tooth model that showed mineralized foci formation (arrows in Figures 8A–D). Moreover, the sizes and amounts of mineralized foci are bigger and larger than those in ex-vivo human tooth model. In RDSC and WMTA groups, accumulation of collagen fibers is noted (# in Figures 8A,B). Intact and complete reparative dentin bridge formation which possessed tubular structures were noted underneath the exposure site (*) in RDSC groups and the layer next to the pulp is blue-stained and the distant part is red-stained (Figure8E). The reparative dentin bridge facing the material side is disorganized with pores inside. However, the opposite part of the reparative dentin bridge is well-organized with tubular structures (Figure 6E). Similar results could be observed for other cements (Figures 8F–H). Mineral foci are also found close to the dentin bridges (arrows) in the RDSC, Biodentine, iRoot BP Plus groups (Figures 8E,G,H).
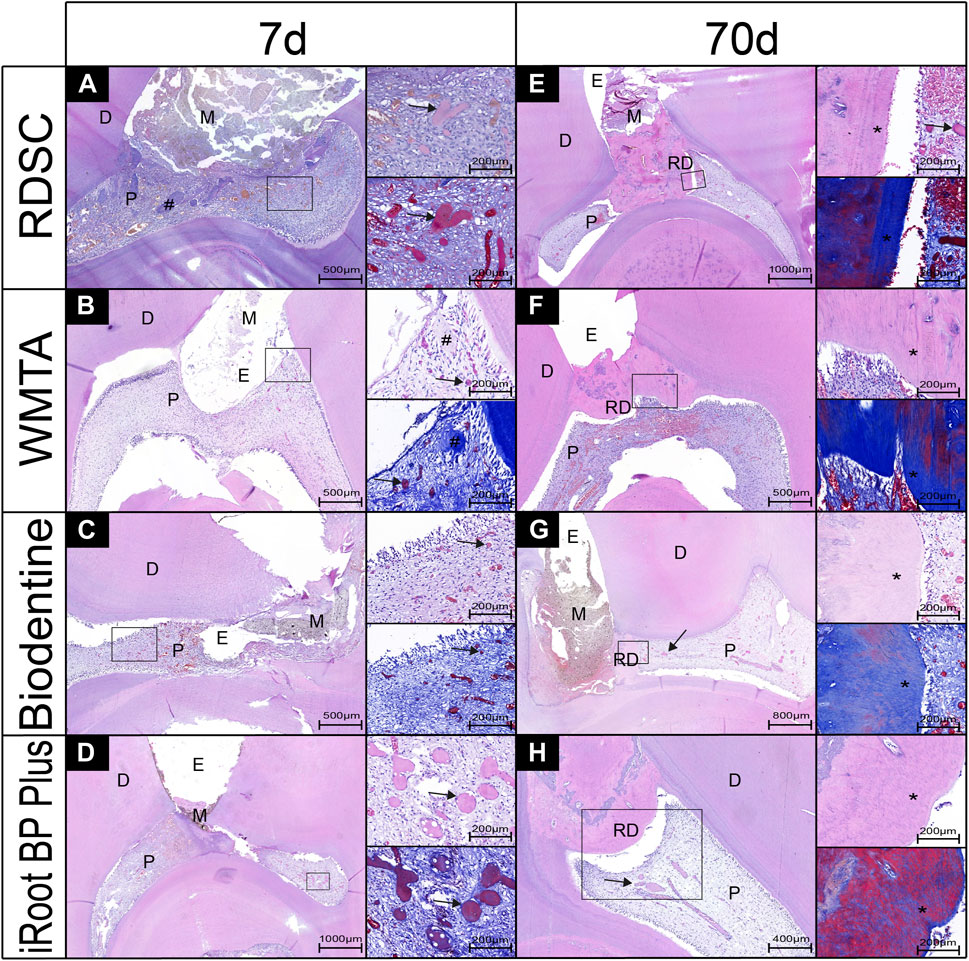
FIGURE 8. Pulp-tissue response of representative teeth after seven or 70 days in the in-vivo miniature pig model, with histological micrographs of pulps that were capped by RDSC (A,E), WMTA (B,F), Biodentine (C,G), iRoot BP Plus (D,H). (D, dentin; M, materials; E, exposure sites; RD, reparative dentin; P, pulp tissue, arrows: mineral foci, #: collagen fibers; *: tubular structures).
Discussion
In general, the discoloration incidence of RDSC was slightly lower than that of WMTA. Therefore, the null hypothesis (Aeinehchi et al., 2003) the color stability of RDSC shows no significant different than the other three materials was rejected. RDSC promoted the formation of mineralized foci and facilitated reparative dentin formation. We can reject the second null hypothesis that RDSC does not provoke any pulp tissue response in both ex vivo and in vivo models in all periods.
Although the color stability of RDSC was better than that of WMTA, it was still far from satisfaction, especially when compared with those of Biodentine and iRoot BP Plus. RDSC and WMTA both contained approximately 20% bismuth oxide (Camilleri, 2008; Kang et al., 2015), while Biodentine and iRoot BP Plus used zirconium oxide and tantalum oxide as alternative radiopacifiers, respectively (Jiang et al., 2014; Kaup et al., 2015). Bismuth oxide was probably the dominant cause of tooth discoloration. Clinical condition (light-exposed and oxygen-free) may cause the dissociation of bismuth oxide into dark-colored crystals of metallic bismuth and oxygen (Vallés et al., 2013; Kang et al., 2015). Once the discoloration products formed, they can spread out through dentinal tubules (Jang et al., 2013; Marciano et al., 2014; Kang et al., 2015; Marciano et al., 2017). In this case, the reduction of discoloration incidence in in vivo experimentation from 7 to 70 days in case of MTA may be due to the individual variation of the pulp chamber. Only one sample in 70 days MTA group exhibited no discoloration and it happened to be a molar with a deep position of pulp chamber which may lead to less light exposure. Zirconium oxide was proved to possessed better color stability than bismuth oxide in both in-vitro and ex-vivo assays (8, 11, 13). Unfortunately, the radiopacity of zirconium oxide was inferior to that of bismuth oxide, larger proportion is required to provide similar radiopacity to bismuth oxide which thus may result in the deterioration in physical and chemical properties of the cement (10), that may explain the long setting time of iRoot BP Plus. In the present study, it is relatively hard to distinguish the Biodentine from the dentin in the micro-CT graphs. Above all, the balance of the parameters is necessary to meet the need of individual clinical case. RDSC is a good candidate as a pulp capping agent in non-aesthetic region.
As recommended by ISO 7045-2018, use non-rodent mammals of one species of such an age that their dentition contains intact permanent teeth with closed mature apices. Minipigs have been widely used as a pulp capping model for decades due to similar anatomical and physiological characteristics to human (Tziafa et al., 2014; Tziafa et al., 2015; Tziafas et al., 2017; Li et al., 2018; Pedano et al., 2020). Nonetheless, pulp responses in animals cannot fully represent those in humans (Emara et al., 2018). Hence an ex vivo human tooth culture model was implemented. In a previous study, both MTA and calcium hydroxide could induce the formation of mineralized foci which possessed dentin sialoprotein and nestin positive sequestered cells (Téclès et al., 2008). It is proved that pulp tissues cultured in this human tooth model for 4 weeks still have viable cells that behave and express mesenchymal stem cell markers (Pedano et al., 2019). The application of materials can be easily done in clinical ways by this model which might lead to a higher reproducibility and accuracy. Moreover, human teeth are simpler to acquire.
The aim of treatment after pulp exposure is to promote the pulp tissue healing and facilitate the formation of reparative dentin to preserve the pulp vitality and health (Aguilar and PJJoe, 2011). Dental pulp stem cells have been demonstrated to play an important part in the regeneration and repair of the pulp injury (MJJodr, 1979; Gronthos et al., 2000). Therefore, calcium and silicon release has widely been shown to play a key role in migration, proliferation and differentiation of human stem cells (Bunpetch et al., 2019). Moreover, Ca and Si have also been noticed to facilitate osteoblast proliferation and collagen production (Valerio et al., 2004). A similar increase in alkaline phosphatase activity, deposition of mineralized nodules, and up-regulation of markers for odontoblastic differentiation was detected in MTA and Biodentine (Chang et al., 2014). It was also found that Alkaline materials such as calcium hydroxide or tricalcium silicate released growth factors such as TGF-β1 via collagen degradation (Huang et al., 2018).
In the present study, mineralized foci were noted along with the accumulation of collagen fibers in all the pulp capping groups in ex vivo human tooth culture model (Figure3). This result was accordance with previous studies (Pedano et al., 2020; Téclès et al., 2008; Laurent et al., 2012; Jeanneau et al., 2017) and the present in vivo study (Figure8). Although some non-organized hard tissues with small hole defects were formed distantly to pulp, Intact and complete reparative dentin bridge formation which possessed tubular structures were noted in all 70 days groups next to the pulp tissue that provide a complete defense (Figures 8E–H). Since dentin was considered as a protection of pulp, the thickness and continuity of dentin were two important factors. Previous research has proven that Odontoblast numbers beneath cavities restored with different restoration materials decreased when remaining dentin thickness (RCT) was below 0.5 mm compared to the one above 0.5 mm (About PEM et al., 2001). In this study, the average dentin bridge thickness of each group was in the range of 100–400 μm and this result was correlated to previous studies (Tziafa et al., 2014; Tziafa et al., 2015) (Oguntebi et al., 1995). RDSC induced the thickest reparative dentin among these materials, which may be due to the large constituent ratio of dicalcium silicate and silicate ions. Silicate act as an effective apatite nucleator in the apatite precipitation (Ding et al., 2009). Moreover, Dicalcium silicate reacts slowly (Peterson et al., 2005) which may lead to a more prolonged calcium ion release than tricalcium silicate, which can also explain the mineral foci formation of RDSC is less than that of iRoot BP Plus on day 7 in the ex-vivo human culture model that lacks calcium source from the vascular system.
In conclusion, RDSC possesses promising dentinogenic property and may have the potential to be a pulp capping agent recommended alternative to WMTA, Biodentine and iRoot BP Plus in the non-aesthetic area. Further studies such as randomized clinical trials should be implemented to validate the clinical applications.
Data Availability Statement
The original contributions presented in the study are included in the article/Supplementary Material, further inquiries can be directed to the corresponding authors.
Ethics Statement
The studies involving human participants were reviewed and approved by the Institutional Ethical Board of Wuhan University. Written informed consent to participate in this study was provided by the participants’ legal guardian/next of kin. The animal study was reviewed and approved by Animal Ethics Committee in Wuhan University.
Author Contributions
W-TK contributed to data acquisition, analysis, and interpretation, drafted, and critically revised the article. XL contributed to the design of the study and the revise of article. F-YC helped performed the in vivo experiment. Y-JW helped performed the ex vivo experiment. ZC contributed to conception and data analysis. S-JD contributed to the synthesis of RDSC and revised the article. LZ contributed to conception, design, data analysis, critically revised the article.
Funding
This research was granted from National Natural Science Foundation of China (81771064, 81974148) to LZ.
Conflict of Interest
The authors declare that the research was conducted in the absence of any commercial or financial relationships that could be construed as a potential conflict of interest.
Publisher’s Note
All claims expressed in this article are solely those of the authors and do not necessarily represent those of their affiliated organizations, or those of the publisher, the editors and the reviewers. Any product that may be evaluated in this article, or claim that may be made by its manufacturer, is not guaranteed or endorsed by the publisher.
References
About Pem, I., Franquin, J.-C., Remusat, M., and Smith, A. J. The Effect of Cavity Restoration Variables on Odontoblast Cell Numbers and Dental Repair. J. Dentistry 2001 29, 109–117. doi:10.1016/s0300-5712(00)00067-1
Aeinehchi, M., Eslami, B., Ghanbariha, M., and Saffar, A. S. (2003). Mineral Trioxide Aggregate (MTA) and Calcium Hydroxide as Pulp-Capping Agents in Human Teeth: a Preliminary Report. Int. Endod. J. 36 (3), 225–235. doi:10.1046/j.1365-2591.2003.00652.x
Aguilar, P., and PJJoe, L. (2011). Vital Pulp Therapy in Vital Permanent Teeth with Cariously Exposed Pulp: a Systematic Review. J. Endodontics 37 (5), 581–587. doi:10.1016/j.joen.2010.12.004
Bunpetch, V., Zhang, X., Li, T., Lin, J., Maswikiti, E., Wu, Y., et al. (2019). Silicate-based Bioceramic Scaffolds for Dual-Lineage Regeneration of Osteochondral Defect. Biomaterials 192, 323–333. doi:10.1016/j.biomaterials.2018.11.025
Camilleri, J. (2008). Characterization of Hydration Products of mineral Trioxide Aggregate. Int. Endod. J. 41 (5), 408–417. doi:10.1111/j.1365-2591.2007.01370.x
Chang, S., Lee, S., Ann, H., Kum, K., and Kim, E. (2014). Effects of Calcium Silicate Endodontic Cements on Biocompatibility and Mineralization-Inducing Potentials in Human Dental Pulp Cells. J. Endodontics 40 (8), 1194–1200. doi:10.1016/j.joen.2014.01.001
Chen, C. C., Ho, C. C., Chen, C. H., and Ding, S. J. (2009). Physicochemical Properties of Calcium Silicate Cements for Endodontic Treatment. J. endodontics 35 (9), 1288–1291. doi:10.1016/j.joen.2009.05.036
Chen, C. C., Shie, M. Y., and Ding, S. J. (2011). Human Dental Pulp Cell Responses to New Calcium Silicate-Based Endodontic Materials. Int. Endod. J. 44 (9), 836–842. doi:10.1111/j.1365-2591.2011.01890.x
Chiang, T. Y., and Ding, S. J. (2010). Comparative Physicochemical and Biocompatible Properties of Radiopaque Dicalcium Silicate Cement and mineral Trioxide Aggregate. J. endodontics 36 (10), 1683–1687. doi:10.1016/j.joen.2010.07.003
Dammaschke, T., Leidinger, J., and Schäfer, E. (2010). Long-term Evaluation of Direct Pulp Capping-Treatment Outcomes over an Average Period of 6.1 Years. Clin. Oral Invest. 14 (5), 559–567. doi:10.1007/s00784-009-0326-9
Ding, S-J., Shie, M-Y., and Wang, C-Y. (2009). Novel Fast-Setting Calcium Silicate Bone Cements with High Bioactivity and Enhanced Osteogenesis In Vitro. J. Mater. Chem. 19 (8), 1183. doi:10.1039/b819033j
Elsaka, S. E., Elnaghy, A. M., Mandorah, A., and Elshazli, A. H. (2019). Effect of Titanium Tetrafluoride Addition on the Physicochemical and Antibacterial Properties of Biodentine as Intraorfice Barrier. Dental Mater. : official Publ. Acad. Dental Mater. 35 (1), 185–193. doi:10.1016/j.dental.2018.11.019
Emara, R., Elhennawy, K., and Schwendicke, F. (2018). Effects of Calcium Silicate Cements on Dental Pulp Cells: A Systematic Review. J. dentistry 77, 18–36. doi:10.1016/j.jdent.2018.08.003
Grech, L., Mallia, B., and Camilleri, J. (2013). Investigation of the Physical Properties of Tricalcium Silicate Cement-Based Root-End Filling Materials. Dental Mater. : official Publ. Acad. Dental Mater. 29 (2), e20–28. doi:10.1016/j.dental.2012.11.007
Gronthos, S., Mankani, M., Brahim, J., Robey, P., and Shi, S. (2000). Postnatal Human Dental Pulp Stem Cells (DPSCs) In Vitro and In Vivo. Proc. Natl. Acad. Sci. 97 (25), 13625–13630. doi:10.1073/pnas.240309797
Guo, Y.-j., Du, T.-f., Li, H.-b., Shen, Y., Mobuchon, C., and Hieawy, A. (2016). Physical Properties and Hydration Behavior of a Fast-Setting Bioceramic Endodontic Material. BMC oral health 16, 23. doi:10.1186/s12903-016-0184-1
Hilton, T. J., Ferracane, J. L., Mancl, L., and Northwest Practice-Based Res, C. (2013). Comparison of CaOH with MTA for Direct Pulp Capping: A PBRN Randomized Clinical Trial. J. Dent Res. 92 (S7), 16S–22S. doi:10.1177/0022034513484336
Huang, X., Camba, J., Gu, L., Bergeron, B., Ricucci, D., Pashley, D., et al. (2018). Mechanism of Bioactive Molecular Extraction from Mineralized Dentin by Calcium Hydroxide and Tricalcium Silicate Cement. Dental Mater. 34 (2), 317–330. doi:10.1016/j.dental.2017.11.010
Jang, J. H., Kang, M., Ahn, S., Kim, S., Kim, W., Kim, Y., et al. (2013). Tooth Discoloration after the Use of New Pozzolan Cement (Endocem) and mineral Trioxide Aggregate and the Effects of Internal Bleaching. J. endodontics 39 (12), 1598–1602. doi:10.1016/j.joen.2013.08.035
Jeanneau, C., Laurent, P., Rombouts, C., Giraud, T., and About, I. (2017). Light-cured Tricalcium Silicate Toxicity to the Dental Pulp. J. Endod. 43 (12), 2074–2080. doi:10.1016/j.joen.2017.07.010
Jiang, Y., Zheng, Q., Zhou, X., Gao, Y., and Huang, D. (2014). A Comparative Study on Root Canal Repair Materials: a Cytocompatibility Assessment in L929 and MG63 Cells. TheScientificWorldJournal 2014, 463826. doi:10.1155/2014/463826
Kang, S. H., Shin, Y. S., Lee, H. S., Kim, S. O., Shin, Y., Jung, I. Y., et al. (2015). Color Changes of Teeth after Treatment with Various mineral Trioxide Aggregate-Based Materials: an Ex Vivo Study. J. endodontics 41 (5), 737–741. doi:10.1016/j.joen.2015.01.019
Kaup, M., Schäfer, E., and Dammaschke, T. (2015). An In Vitro Study of Different Material Properties of Biodentine Compared to ProRoot MTA. Head Face Med. 11, 16. doi:10.1186/s13005-015-0074-9
Laurent, P., Camps, J., and About, I. (2012). Biodentine(TM) Induces TGF-Beta1 Release from Human Pulp Cells and Early Dental Pulp Mineralization. Int. Endod. J. 45 (5), 439–448. doi:10.1111/j.1365-2591.2011.01995.x
Lee, J.-B., Park, S.-J., Kim, H.-H., Kwon, Y.-S., Lee, K.-W., and Min, K.-S. (2014). Physical Properties and Biological/odontogenic Effects of an Experimentally Developed Fast-Setting α-tricalcium Phosphate-Based Pulp Capping Material. BMC oral health 14, 87. doi:10.1186/1472-6831-14-87
Li, X., Pedano, M. S., Camargo, B., Hauben, E., De Vleeschauwer, S., and Chen, Z. (2018). Experimental Tricalcium Silicate Cement Induces Reparative Dentinogenesis. Dental Mater. 34 (9), 1410–1423. doi:10.1016/j.dental.2018.06.016
Marciano, M. A., Costa, R. M., MondelliCamilleri, R. F. L., Guimarães, B. M., and Duarte, M. A. H. (2014). Assessment of Color Stability of white mineral Trioxide Aggregate Angelus and Bismuth Oxide in Contact with Tooth Structure. J. endodontics 40 (8), 1235–1240. doi:10.1016/j.joen.2014.01.044
Marciano, M. A., CostaCamilleri, R. M., Matsumoto, M. A., Guimarães, B. M., and Duarte, M. A. H. (2017). Zinc Oxide Inhibits Dental Discoloration Caused by White Mineral Trioxide Aggregate Angelus. J. endodontics 43 (6), 1001–1007. doi:10.1016/j.joen.2017.01.029
MJJodr, F. (1979). Cellular Mechanics of Dentinal Bridge Repair Using 3H-Thymidine. J. Dental Res. 58, 2198–2206. doi:10.1177/002203457905800411011
Oguntebi, B. R., Heaven, T., Clark, A. E., and Pink, F. E. Quantitative Assessment of Dentin Bridge Formation Following Pulp-Capping in Miniature Swine. Journal Endodontics 1995 21, 79–82. doi:10.1016/S0099-2399(06)81100-3
Pedano, M. S., Li, X., Camargo, B., Hauben, E., Vleeschauwer, S. D., Yoshihara, K., et al. (2020). Injectable Phosphopullulan-Functionalized Calcium-Silicate Cement for Pulp-Tissue Engineering: An In-Vivo and Ex-Vivo Study. Dental Mater. : official Publ. Acad. Dental Mater. 36 (4), 512–526. doi:10.1016/j.dental.2020.01.011
Pedano, M. S., Li, X., Jeanneau, C., Ghosh, M., Yoshihara, K., Landuyt, K. V., et al. (2019). Survival of Human Dental Pulp Cells after 4-week Culture in Human Tooth Model. J. dentistry 86, 33–40. doi:10.1016/j.jdent.2019.05.023
Peterson, V., Neumann, D., and Livingston, R. (2005). Hydration of Tricalcium and Dicalcium Silicate Mixtures Studied Using Quasielastic Neutron Scattering. Journal Physical Chemistry. B 109 (30), 14449–14453. doi:10.1021/jp052147o
Tanalp, J., Kazandağ, M. K., Dölekoğlu, S., and Kayahan, M. B. (2013). Comparison of the Radiopacities of Different Root-End Filling and Repair Materials. TheScientificWorldJournal 2013, 594950. doi:10.1155/2013/594950
Téclès, O., Laurent, P., Aubut, V., and About, I. (2008). Human Tooth Culture: a Study Model for Reparative Dentinogenesis and Direct Pulp Capping Materials Biocompatibility. J. Biomed. Mater. Res. B, Appl. Biomater. 85 (1), 180–187. doi:10.1002/jbm.b.30933
Tziafa, C., Koumpia, E. K., Papadimitriou, S., and Tziafas, D. (2015). Dentinogenic Activity of Biodentine in Deep Cavities of Miniature Swine Teeth. J. endodontics 41 (7), 1161–1166. doi:10.1016/j.joen.2015.03.018
Tziafa, C., Koumpia, E. K., Papadimitriou, S., and Tziafas, D. (2014). Dentinogenic Responses after Direct Pulp Capping of Miniature Swine Teeth with Biodentine. J. endodontics 40 (12), 1967–1971. doi:10.1016/j.joen.2014.07.021
Tziafas, D., Kodonas, K., Gogos, C., Tziafa, C., and Papadimitriou, S. (2017). Dentine-pulp Tissue Engineering in Miniature Swine Teeth by Set Calcium Silicate Containing Bioactive Molecules. Arch. Oral Biol. 73, 230–236. doi:10.1016/j.archoralbio.2016.10.023
Valerio, P., Pereira, M., Goes, A., and Leite, M. (2004). The Effect of Ionic Products from Bioactive Glass Dissolution on Osteoblast Proliferation and Collagen Production. Biomaterials 25 (15), 2941–2948. doi:10.1016/j.biomaterials.2003.09.086
Vallés, M., Mercadé, M., Sindreu, F. D., Bourdelande, J. L., and Roig, M. (2013). Influence of Light and Oxygen on the Color Stability of Five Calcium Silicate-Based Materials. J. endodontics 39 (4), 525–528. doi:10.1016/j.joen.2012.12.021
Wu, B. C., Huang, S. C., and Ding, S. J. (2013). Comparative Osteogenesis of Radiopaque Dicalcium Silicate Cement and White-Colored Mineral Trioxide Aggregate in a Rabbit Femur Model. Materials (Basel, Switzerland) 6 (12), 5675–5689. doi:10.3390/ma6125675
Wu, B. C., Wei, C. K., Hsueh, N. S., and Ding, S.-J. (2015). Comparative Cell Attachment, Cytotoxicity and Antibacterial Activity of Radiopaque Dicalcium Silicate Cement and white-coloured mineral Trioxide Aggregate. Int. Endod. J. 48 (3), 268–276. doi:10.1111/iej.12310
Keywords: dicalcium silicate cement, entire tooth culture, miniature pig, direct pulp capping (DPC), reparative dentin formation
Citation: Kuo W-T, Li X, Chen F-Y, Wang Y-J, Chen Z, Ding S-J and Zhang L (2021) Experimental Pulp-Capping Agent Radiopaque Dicalcium Silicate Cement Facilitates Dentinogenesis. Front. Mater. 8:738430. doi: 10.3389/fmats.2021.738430
Received: 08 July 2021; Accepted: 29 September 2021;
Published: 20 October 2021.
Edited by:
Tiantian Kong, Shenzhen University, ChinaCopyright © 2021 Kuo, Li, Chen, Wang, Chen, Ding and Zhang. This is an open-access article distributed under the terms of the Creative Commons Attribution License (CC BY). The use, distribution or reproduction in other forums is permitted, provided the original author(s) and the copyright owner(s) are credited and that the original publication in this journal is cited, in accordance with accepted academic practice. No use, distribution or reproduction is permitted which does not comply with these terms.
*Correspondence: Shinn-Jyh Ding, c2pkaW5nQGNzbXUuZWR1LnR3; Lu Zhang, bHV6aGFuZzIwMTJAd2h1LmVkdS5jbg==