- 1Department of Electrical Engineering and Information Systems, Graduate School of Engineering, The University of Tokyo, Tokyo, Japan
- 2Center for Spintronics Research Network, Graduate School of Engineering, The University of Tokyo, Tokyo, Japan
Thin films of BaFe1-xMxO3 (M = Hf, Zr, and Ce; 0.0 ≤ x ≤ 0.75) were fabricated using pulsed laser deposition and their magnetic properties were investigated. X-ray diffraction analysis indicated that oxygen-deficient BaFeOx (x < 3.0) with a monoclinic structure was formed when the deposition was conducted using a non-substituted target (x = 0.0). The as-grown BaFeOx films were converted into fully oxidized BaFeO3 with a perovskite structure by low-temperature oxidation in an ozone atmosphere. In contrast, the as-deposited films of Hf, Zr, and Ce-substituted films exhibited a perovskite structure, and their crystallinity did not change after low-temperature ozone annealing. The magnetic transition temperature Tc of the BaFeO3 film was 115 K, whereas the substituted BaFeO3 films showed ferromagnetic behavior even at 300 K. These results can be attributed to the weakening of the antiferromagnetic super-exchange coupling among Fe ions owing to the lattice expansion in the substituted BaFeO3. In addition, the magnetization of the films was found to increase with the decreasing ionic ratio of Fe4+/Fe3+, suggesting that the inherent carrier-induced ferromagnetic interaction is dominant in the films.
Introduction
Oxide materials with unusual valence ions have attracted much attention for a long time because of their intriguing electrical and magnetic properties (Watanabe, 1957; Chen et al., 2012). In this study, we focused on perovskite-type BaFeO3 with unusually high-valence Fe4+ ions. Barium ferrite, BaFeO3-δ, has various crystal structures depending on the values of the oxygen vacancy δ. For decades, a number of studies have been conducted on the crystal structures and magnetic properties of oxygen-defective BaFeO3-δ (K. Mori et al., 2007, S. Mori 1966). However, owing to the large ionic radius of Ba, the synthesis of bulk BaFeO3 (BFO) with a perovskite structure was not successful until recently. It has also recently been shown that fully oxidized BaFeO3 can be obtained from BaFeOx (x < 3.0) using a low-temperature oxidation procedure (Hayashi et al., 2011). The authors reported that the obtained BaFeO3 is an A-type spiral magnet with a Curie temperature (Tc) of 111 K. Likewise, Chakraverty et al. reported the successful growth of epitaxial BaFeO3 films by pulsed laser deposition (PLD) followed by low-temperature oxidation treatment (Chakraverty et al., 2013). They reported that the Ms and Tc values for the films are 3.2 μB/formula unit and 115 K, respectively. Thus, the Tc value of the BaFeO3 films and bulk BaFeO3 is far below room temperature. Enhancing the Tc of BaFeO3 is crucial from an applied viewpoint. Recently, it has been reported that the Tc of BaFeO3 can be elevated by the substitution of Fe by other elements with larger ionic ratios, such as Zr and Sn (Matsui et al., 2005; Matsui et al., 2008; Kanatani et al., 2010; Shinoda et al., 2014). However, there are few systematic studies on substituted BaFeO3 in the literature. In this study, we fabricated thin films of Hf-, Zr-, and Ce-substituted BaFeO3 films and investigated their magnetic properties.
Experimental
Thin films of BaFe1-xMxO3 (BFMO) (M = Hf, Zr, and Ce; 0.0 ≤ x ≤ 0.75) were fabricated by PLD followed by a low-temperature oxidation process. PLD targets were prepared via a standard solid-state reaction. A SrTiO3 (STO) (001) single-crystal plate was used as the substrate. During the film deposition, the substrate temperature and oxygen pressure were maintained at 700°C and under 0.1 Pa, respectively. The as-grown films were annealed at 200 °C in an O3 atmosphere for 3 h. The crystalline structures of the films were analyzed by X-ray diffraction (XRD) using an X-ray diffractometer (Empyrean system, Malvern Panalytical) with a Cu Kα source (λ = 0.154 nm) operated at 40 kV and 40 mA. The magnetic properties of the films were measured using a superconducting quantum interference device (MPMS, Quantum Design) over 10–300 K. To obtain detailed information about the oxidation states of the films, X-ray photoelectron spectroscopy (XPS) was conducted using an XPS system (JPS-9010 MC, JEOL) with a monochromatic Al Kα radiation source (1,486.7 eV). Optical absorption measurements were performed using a UV-Vis spectrometer (V-670, JASCO).
Results and Discussion
The out-of-plane XRD patterns of the films are shown in Figure 1A–E. As shown in the black data in Figure 1A, upon using a non-substituted barium ferrite target, the resulting as-grown film comprises (001)-oriented single-crystalline BaFeOx with a pseudocubic lattice parameter of 4.12 Å. After annealing in O3, the BaFeOx phase is converted to a (001)-oriented, single-crystalline, and cubic perovskite-type BaFeO3 phase with a lattice parameter of 3.99 Å, as shown in the red data in Figure 1A. Figure 1B shows the XRD patterns of the as-grown and annealed BaFe0.5Hf0.5O3 films. Unlike in the case of pristine BaFeO3, the as-grown film shows a cubic phase, and no obvious change in the crystal structure is observed after annealing in O3, indicating that the cubic perovskite phase is stabilized by the substitution of Hf4+. This result is consistent with the fact that the Gibbs formation energy of BaHfO3 is smaller than that of BaFeO3 (https://materialsproject.org/materials/mp-19035/, https://materialsproject.org/materials/mp-998552/). As shown in Figure 1C, clear Laue fringes are observed even for the as-grown film, which suggests the high quality of the films. The lattice parameter of the as-grown and annealed films are 4.19 Å and 4.18 Å, respectively. The slightly smaller lattice parameter of the annealed film was due to oxidation. It should be noted that Ce- or Zr-substituted BaFeO3 films with a cubic perovskite structure can also be fabricated by PLD without O3 annealing as shown in Figure 1D. As Figure 1E shows, the (002) peak shifts to a lower angle with an increase in the substitution level x. This result indicates the lattice expansion of films by the substitution of M4+ for Fe4+. Figure 1F shows the dependence of lattice parameters of as-grown BFMO films on the substitution level x. The lattice parameter obeys Vegard’s law for M = Hf, Zr, and Ce. In addition, as clearly shown in Figures 1D,F, the lattice parameter increases upon increasing the ionic radius of the substituted ions [Hf4+ (0.78 Å), Zr4+ (0.84 Å), and Ce4+ (0.97 Å)]. These results suggest that the M4+ ions substitute the Fe4+ ions at the B-site of the perovskite structure in the films. As shown in the reciprocal space map (RSM) of Figure 1G, all the films are relaxed because of the large lattice mismatch (5.2–8.6%) between the film and the STO substrate.
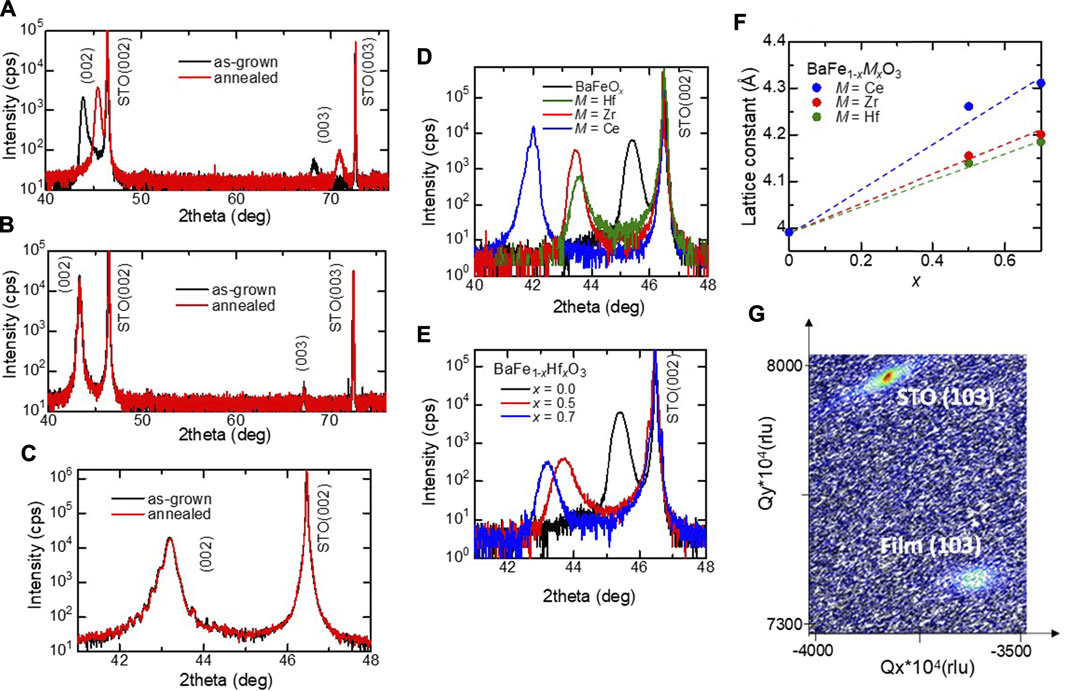
FIGURE 1. (A) XRD 2θ–ω patterns for the BaFeOx (as-grown) and BaFeO3 (annealed) films grown on the STO (001) substrate. Open and closed circles denote the peaks of BaFeOx and BaFeO3, respectively. (B) XRD patterns of as-grown and annealed BaFe0.5Hf0.5O3 films. (C) XRD patterns around (002) reflection of as-grown and annealed BaFe0.5Hf0.5O3 films. (d) XRD patterns around the (002) reflection of BaFe0.5M0.5O3 (M = Hf, Zr, and Ce) films. (E) XRD patterns around the (002) reflection of BaFe1-xHfxO3 films. (F) Lattice parameters as a function of the substitution level x for the BFMO films. (G) Reciprocal space mapping of the BaFe0.5Hf0.5O3 thin film around the (103) Bragg reflection.
We found that the optical properties of the BFMO films were peculiar compared to the solid-solution system. Figure 2A shows the optical transmission spectra of the BaFe1-xHfxO3 films. The increased absorption of BaFeO3 in the visible region is attributed to O2p–Fe3d charge-transfer transitions, as suggested by first-principles calculations (Li et al., 2012; Mizumaki et al., 2015). Figure 2A shows the Tauc plots of the optical absorption of the BaFe1-xHfxO3 films. The bandgap energy of the BFO films is estimated to be 1.8 eV, which is close to the previously reported value (Chakraverty et al., 2013). The tails observed in the lower energy region for x = 0–0.5, in the Tauc plots can be attributed to defects in the films. As shown in Figure 2C, Eg exhibits a bowing behavior. The relation between the bandgap and the substitution level x can be described by the following equation based on Vegard’s law (Cardona 1963; Androulidaki et al., 2006):
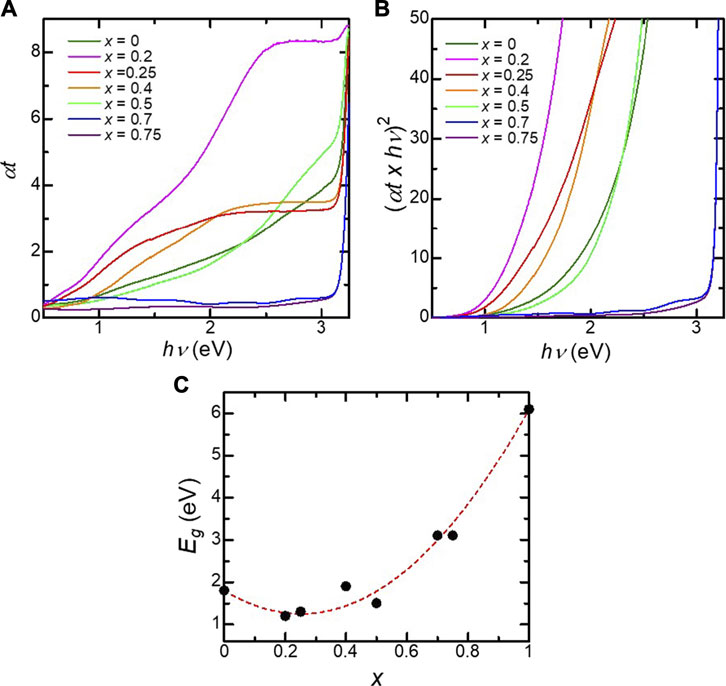
FIGURE 2. (A) Optical absorption (αt) as a function of photon energy for the BaFe1-xHfxO3 films. (B) Tauc plot for the BaFe1-xHfxO3 films. (C) Band gap energy (Eg) as a function of the Hf content x in the BaFe1-xHfxO3 films. The value of Eg for x = 1.0 is the datum from ref. [21].
Eg(BaFe1-xHfxO3) = xEg(BaHfO3) + (1−x)Eg(BaFeO3) –bgx(1−x) (1)
where bg is the so-called bowing parameter, and Eg(BaFe1-xHfxO3), Eg(BaHfO3), and Eg(BaFeO3) are the bandgaps of BaFe1-xHfxO3, BaHfO3Kim et al. (2017), and BaFeO3, respectively. The dependence of the optical bandgap on x is well fitted with bg = 8.7 eV, as shown by the dotted curve in Figure 2C. This value is considerably larger than those reported for other alloy oxide systems such as (Ni, Mg)O Deng et al. (2012), (Fe, Mg)O Seki et al. (2014) and (Zn, Cd)O Zhu et al. (2008), suggesting a large band offset in the valence and conduction bands in the BFMO films.
XPS measurements were performed to validate the valence of the Fe ions in the films. The Fe-2p core level XPS spectra of the BaFeOx and BMFO films are shown in Figure 3A. The 2p1/2 and 2p3/2 peaks of the BFMO films are located at 727.0 and 709.9 eV, respectively. These peak positions are nearly the same for all BFMO films. However, the 2p1/2 and 2p3/2 peaks can be seen at 729.9 and 711.3 eV, respectively, both of which are slightly higher than those of the BFMO films, suggesting the different valence states of Fe between the BaFeOx and BFMO. As Figure 3B shows, when a Gaussian fitting method is used, the 2p3/2 peak of the BaFeOx film is deconvoluted into two components at 711.7 and 709.9 eV, which can be assigned to Fe3+ and Fe2+, respectively (Temesghen and Sherwood, 2002; Li et al., 2021). The ionic ratio of Fe3+/Fe2+ is calculated to be 13.3, indicating that most of Fe ions in the BaFeOx films are in a 3 + state. However, as shown in Figure 3C, the Fe 2p3/2 peak of the BFMO films consists of two major components at 711.7 and 709.6 eV, which can be assigned to Fe3+ and Fe4+, respectively (Bocquet et al., 1992; Lombardi et al., 2019). Thus, Fe ions in the BFMO films are in the mixed valence state of Fe4+/Fe3+, which strongly affects the magnetic properties of the films, as discussed later. The ionic ratio of Fe4+/Fe3+ in the annealed film (Fe4+/Fe3+ ∼ 2.0–2.5) was larger than that of the as-grown films (Fe4+/Fe3+ ∼ 1.5–1.7), suggesting the oxidation of the film after annealing in O3.
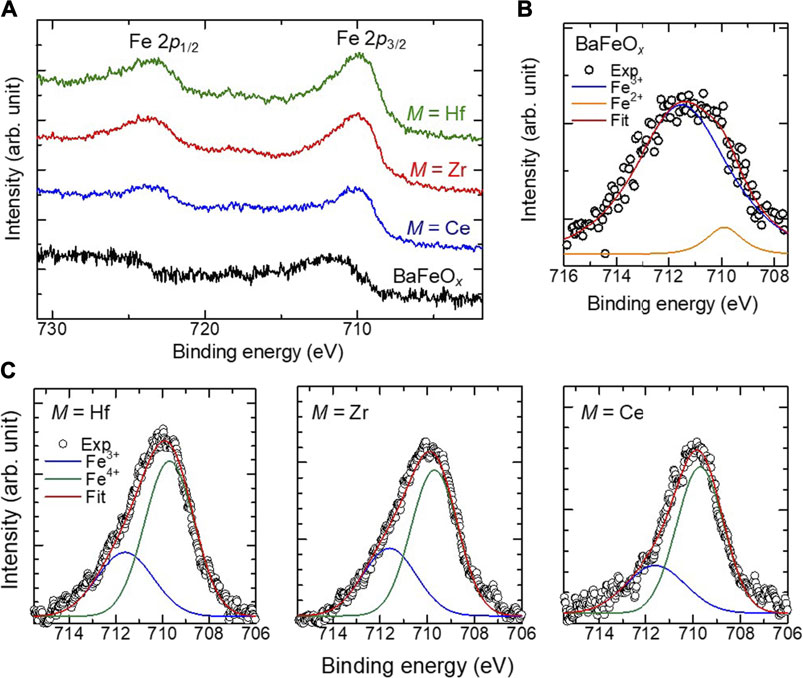
FIGURE 3. (A) XPS spectrum of the Fe 2p level of the as-grown BaFeOx and BaFeMO3 thin films. (B) A fitted XPS spectrum of the Fe level 2p3/2 level of the BaFeOx thin film. (C) Fitted spectra of the Fe 2p3/2 level of the BaFeMO3 films.
The results of the magnetic measurements are shown in Figure 4. As shown in Figure 4A, the Tc of the BFO film is estimated to be 115 K, which agrees well with the reported value for the bulk BFO (111 K) (Hayashi et al., 2011). As shown in the inset of Figure 4A, the saturation magnetization of the BFO film is ×1.0 10–3 emu/cm3 at 10 K. The magnetic properties were found to change drastically upon substitution. Figure 4B shows the temperature dependence of the magnetization of the BFMO films measured under a persistent magnetic field of 1 kOe. There was no significant downward trend in the magnetization of any of the films. This result provides evidence that the Curie temperature TC of the films is well above 300 K. High temperature measurements are scheduled using the MPMS oven option to determine the value of TC for BFMO films. As shown in Figure 4C, we confirmed that all the BFMO films displays ferromagnetic behaviors with coercive field of ∼20–30 Oe at 300 K. Figure 4D shows the magnetic field H-dependence of the magnetization M of the BaFe1-xCexO3 films at room temperature (300 K). It can be clearly seen that all these compositions exhibited room-temperature ferromagnetic behavior. This enhanced magnetization could be attributed to the lowered antiferromagnetic (AFM) coupling among Fe ions in the substituted films, as explained later. The magnetic properties of BFMO films are explained in terms of the coexistence of ferromagnetic double-exchange and antiferromagnetic super-exchange interactions. Figure 4E shows the dependence of the saturation magnetization on the ionic ratio Fe4+/Fe3+, which was calculated from the results of Gaussian fittings in the XPS analysis. As shown in Figure 4E, the saturation magnetization tends to decrease with an increase in the Fe4+/Fe3+ ratio. This result indicates that the Fe4+/Fe3+ mixed valence state enhances the ferromagnetism in the films owing to the double exchange mechanism. However, super-exchange coupling among Fe ions should also be considered. According to Harrison’s relation, the super-exchange interaction J is expressed as J ∝ 1/r14, where r is the distance between Fe ions, while the double exchange interaction depends significantly less on r (Harrison 1989). Therefore, AFM coupling is drastically reduced and FM interaction becomes more prominent by substituting Fe4+ with M4+ with larger ionic radii in the films, resulting in the observed ferromagnetism at room temperature.
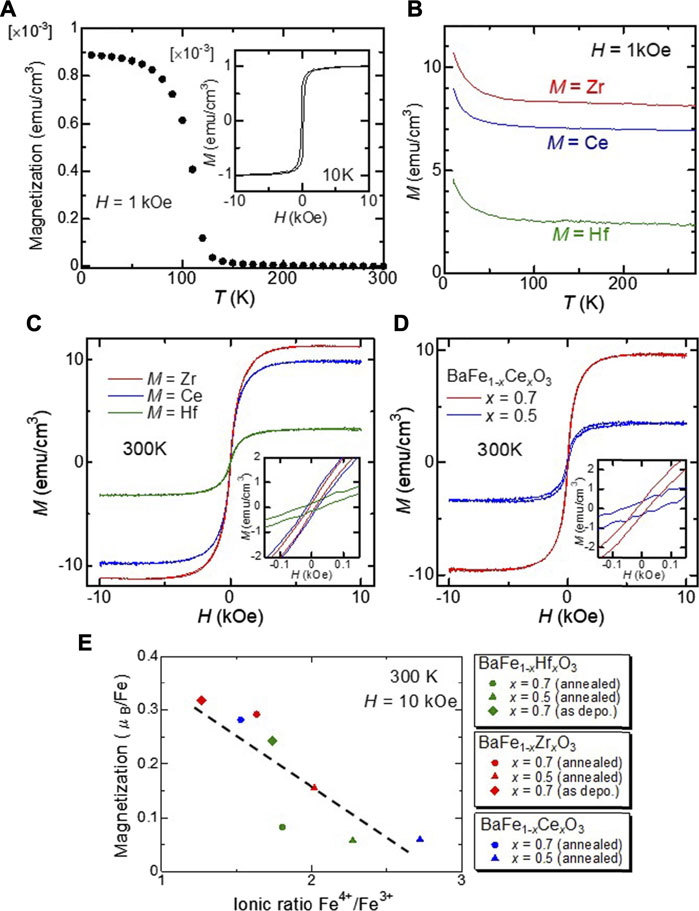
FIGURE 4. (A) Temperature dependence of the magnetization for the BaFeO3 film under a magnetic field of 10 kOe. Inset shows the magnetization as a function of magnetic field at 10 K. (B) Temperature dependence of the magnetization for the BFMO films under the magnetic field of one kOe. (C) The magnetization of the BaFe0.3M0.7O3 films as a function of the magnetic field at 300 K. Inset shows magnified M–H curves of the films. (D) The magnetization of the BaFe1-xCexO3 films with x = 0.5 and 0.7 as a function of the magnetic field at 300 K. Inset shows magnified M–H curves of the films. (E) Dependence of the saturation magnetization of the BFMO films on the Fe4+/Fe3+ ratio.
In summary, thin films of Hf, Zr, and Ce-substituted BaFeO3 epitaxial thin films were fabricated using PLD. The highly oriented epitaxial thin films of BFMO films with a cubic perovskite structure were found to grow without annealing treatment in contrast to the pure BaFeO3 film. The bandgap of the films can be varied over a wide range owing to the large bandgap bowing effect. XPS measurements revealed that the Fe ions in the BFMO films were in the mixed valence state of Fe4+/Fe3+. The BaFeO3 film was a ferromagnet with a Tc of 115 K. On the other hand, ferromagnetic behaviors were observed at room temperature for the BFMO films. The saturation magnetization of the BFMO films increased with an increase in the lattice parameters and ionic ratio of Fe4+/Fe3+ in the films. The ferromagnetic behaviors of the BFMO films could be explained by the double exchange interaction and lowered AFM coupling among Fe ions caused by the substitution of Fe3+ by M4+ with a larger ionic ratio. Our findings indicate the potential of BFMO films as room-temperature ferromagnetic semiconductors.
Data Availability Statement
The original contributions presented in the study are included in the article/supplementary material, further inquiries can be directed to the corresponding author.
Author Contributions
MS: Investigation, Validation, Writing - review andamp; editing. HY: Validation, Writing - review andamp; editing. HT: Methodology, Funding acquisition, Supervision, Project administration. MS: Conceptualization, Investigation, Methodology, Validation, Writing-original draft, Funding acquisition.
Funding
This research was supported by the Institute for AI and Beyond at the University of Tokyo, and JSPS KAKENHI Grant Number JP18K18850.
Conflict of Interest
The authors declare that the research was conducted in the absence of any commercial or financial relationships that could be construed as a potential conflict of interest.
Publisher’s Note
All claims expressed in this article are solely those of the authors and do not necessarily represent those of their affiliated organizations, or those of the publisher, the editors and the reviewers. Any product that may be evaluated in this article, or claim that may be made by its manufacturer, is not guaranteed or endorsed by the publisher.
References
Androulidaki, M., Pelekanos, N. T., Tsagaraki, K., Dimakis, E., Iliopoulos, E., Adikimenakis, A., et al. (2006). Energy Gaps and Bowing Parameters of InAlGaN Ternary and Quaternary Alloys. Phys. Stat. Sol. (C) 3, 1866–1869. doi:10.1002/pssc.200565280
Bocquet, A. E., Fujimori, A., Mizokawa, T., Saitoh, T., Namatame, H., Suga, S., et al. (1992). Electronic Structure ofSrFe4+O3and Related Fe Perovskite Oxides. Phys. Rev. B 45, 1561–1570. doi:10.1103/physrevb.45.1561
Cardona, M. (1963). Optical Properties of the Silver and Cuprous Halides. Phys. Rev. 129, 69–78. doi:10.1103/physrev.129.69
Chakraverty, S., Matsuda, T., Ogawa, N., Wadati, H., Ikenaga, E., Kawasaki, M., et al. (2013). BaFeO3 Cubic Single Crystalline Thin Film: A Ferromagnetic Insulator. Appl. Phys. Lett. 103 (1), 142416. doi:10.1063/1.4824210
Chen, W.-T., Saito, T., Hayashi, N., Takano, M., and Shimakawa, Y. (2012). Ligand-hole Localization in Oxides with Unusual Valence Fe. Sci. Rep. 2 (1), 449. doi:10.1038/srep00449
Deng, J., Mortazavi, M., Medhekar, N. V., and Liu, J. Z. (2012). Band Engineering of Ni1−xMgxO Alloys for Photocathodes of High Efficiency Dye-Sensitized Solar Cells. J. Appl. Phys. 112 (1), 123703. doi:10.1063/1.4769210
Hayashi, N., Yamamoto, T., Kageyama, H., Nishi, M., Watanabe, Y., Kawakami, T., et al. (2011). BaFeO3: A Ferromagnetic Iron Oxide. Angew. Chem. 123, 12755–12758. doi:10.1002/ange.201105276
Kanatani, H., Matsui, T., Hirao, N., Yamamoto, H., Baba, Y., Kume, H., et al. (2010). Improvement of Magnetic and Dielectric Properties of BaFeO3−δ Thin Films by Sn Substitution A. J. Appl. Phys. 107 (1), 09E312. doi:10.1063/1.3360208
Kim, Y. M., Park, C., Ha, T., Kim, U., Kim, N., Shin, J., et al. (2017). High-k Perovskite Gate Oxide BaHfO3. APL Mater. 5 (1), 016104. doi:10.1063/1.4974864
Li, H., Yamahara, H., Tabata, H., and Seki, M. (2021). Epitaxial Thin Films of Room-Temperature Ferromagnetic Semiconductor Based on Fe2TiO5–FeTi2O5 Solid Solution. Appl. Phys. Lett. 119 (1), 022402. doi:10.1063/5.0055324
Li, Z., Laskowski, R., Iitaka, T., and Tohyama, T. (2012). First-principles Calculation of Helical Spin Order in Iron Perovskite SrFeO3 and BaFeO3. Phys. Rev. B 85 (1), 134419. doi:10.1103/physrevb.85.134419
Lombardi, J., Yang, L., Pearsall, F. A., Farahmand, N., Gai, Z., Billinge, S. J. L., et al. (2019). Stoichiometric Control over Ferroic Behavior in Ba(Ti1-xFex)O3 Nanocrystals. Chem. Mater. 31, 1318–1335. doi:10.1021/acs.chemmater.8b04447
Matsui, T., Sato, R., and Tsuda, H. (2008). Valence State and Spatial Distribution of Fe Ions in Ferromagnetic Ba(Fe1−xZrx)O3−δ Single-crystal Films on SrTiO3 Substrates. J. Appl. Phys. 103 (1), 07E304. doi:10.1063/1.2836709
Matsui, T., Taketani, E., Fujimura, N., Tsuda, H., and Morii, K. (2005). Enhancement of Ferromagnetic Ordering in Dielectric BaFe1−xZrxO3−δ (x=0.5–0.8) Single-crystal Films by Pulsed Laser-Beam Deposition. J. Appl. Phys. 97 (1), 10M509. doi:10.1063/1.1850860
Mizumaki, M., Fujii, H., Yoshii, K., Hayashi, N., Saito, T., Shimakawa, Y., et al. (2015). Electronic Structure of BaFeO3 Studied by X-ray Spectroscopy. Phys. Status Solidi C 12, 818–821. doi:10.1002/pssc.201400252
Mori, K., Kamiyama, T., Kobayashi, H., Otomo, T., Nishiyama, K., Sugiyama, M., et al. (2007). Mixed Magnetic Phase in 6H-type BaFeO3−δ. J. Appl. Cryst. 40, s501–s505. doi:10.1107/s0021889807001653
Mori, S. (1966). Phase Transformation in Barium Orthoferrate, BaFeO3-X. J. Am. Ceram. Soc. 49, 600–605. doi:10.1111/j.1151-2916.1966.tb13176.x
Seki, M., Takahashi, M., Adachi, M., Yamahara, H., and Tabata, H. (2014). Fabrication and Characterization of Wüstite-Based Epitaxial Thin Films: P -type Wide-gap Oxide Semiconductors Composed of Abundant Elements. Appl. Phys. Lett. 105 (1), 112105. doi:10.1063/1.4896316
Shinoda, R., Iwase, A., and Matsui, T. (2014). Magneto-Dielectric Properties of Epitaxial Ba(Fe0.5Sn0.5)O3−δ Thin Films on (001) SrTiO3 Substrates by Pulsed Laser Deposition. Mater. Trans. 55, 637–639. doi:10.2320/matertrans.m2013429
Temesghen, W., and Sherwood, P. (2002). Analytical Utility of Valence Band X-ray Photoelectron Spectroscopy of Iron and its Oxides, with Spectral Interpretation by Cluster and Band Structure Calculations. Anal. Bioanal. Chem. 373, 601–608. doi:10.1007/s00216-002-1362-3
Watanabe, H. (1957). Magnetic Properties of Perovskites Containing Strontium I. Strontium-Rich Ferrites and Cobaltites. J. Phys. Soc. Jpn. 12, 515–522. doi:10.1143/jpsj.12.515
Keywords: pulsed laser deposition, perovskite oxide, ferromagnetic oxide, barrium ferrite, Fe4+-containing perovskites
Citation: Sakuda M, Yamahara H, Tabata H and Seki M (2021) Control of Magnetic Properties of Barium Ferrite Thin Films With Unusual Valence Fe. Front. Mater. 8:732676. doi: 10.3389/fmats.2021.732676
Received: 29 June 2021; Accepted: 26 August 2021;
Published: 09 September 2021.
Edited by:
Ahmad Gholizadeh, Damghan University, IranReviewed by:
Saket Asthana, Indian Institute of Technology Hyderabad, IndiaVipul Srivastava, Lovely Professional University, India
Copyright © 2021 Sakuda, Yamahara, Tabata and Seki. This is an open-access article distributed under the terms of the Creative Commons Attribution License (CC BY). The use, distribution or reproduction in other forums is permitted, provided the original author(s) and the copyright owner(s) are credited and that the original publication in this journal is cited, in accordance with accepted academic practice. No use, distribution or reproduction is permitted which does not comply with these terms.
*Correspondence: Munetoshi Seki, m-seki@ee.t.u-tokyo.ac.jp