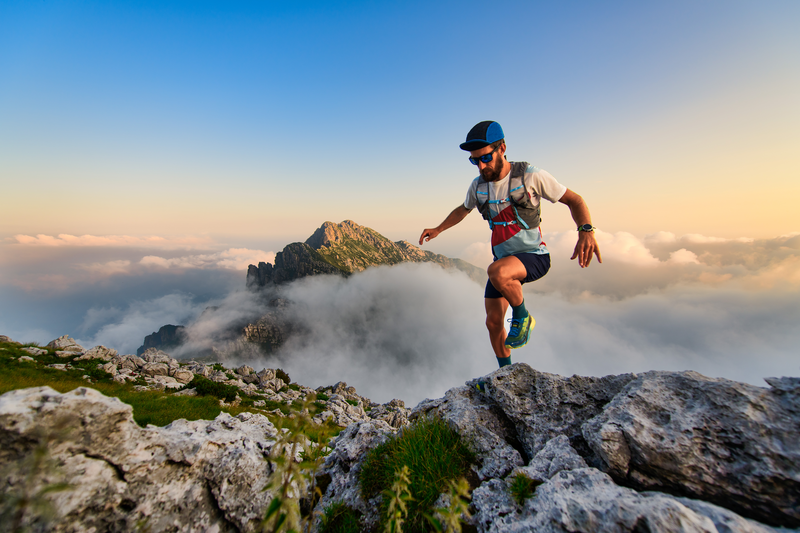
94% of researchers rate our articles as excellent or good
Learn more about the work of our research integrity team to safeguard the quality of each article we publish.
Find out more
MINI REVIEW article
Front. Mater. , 24 May 2021
Sec. Carbon-Based Materials
Volume 8 - 2021 | https://doi.org/10.3389/fmats.2021.695485
This article is part of the Research Topic Innovative Synthetic Methodology for Next Carbon Nanoarchitectures: Towards Sustainable Applications View all 4 articles
As a product generated from incomplete combustion, soot is harmful to people’s health and the environment. In recent decades, much attention has been paid to the control of soot generation in combustion systems. Efforts to reduce soot emissions depend on a basic understanding of the physical and chemical pathways from fuel to soot particles in flames. At the same time, flame synthesis method has become an alternative method for the preparation of carbon nanomaterials because of its advantages of low cost and mass production. Carbon-based materials can be synthesized within the sooting zones in flames. The research of soot formation mechanism in flames can provide support for the synthesis of carbon nanomaterials. In this paper, the effects of additives, temperature, and fuel type on soot formation characteristics and soot nanostructure in diffusion flames are reviewed. The deficiencies and prospects are put forward for future research.
Soot generated from the burning of hydrocarbon fuel is a known pollutant. The emission of fine soot particles brings severe environmental problems. As shown in Figure 1, the physical and chemical processes of soot formation are very complex, including the formation of precursors, soot nucleation, soot surface growth and agglomeration, and soot oxidation (Richter and Howard, 2000). With carbon as the main component, soot is also a kind of carbon material. Carbon nanomaterials (CNM) are widely used in various fields including storage of hydrogen and natural gas (Mykhailiv et al., 2017), electronics (Olariu et al., 2017), and manufacture of batteries, fuel cell devices, hyper-lubricants and sensors due to exceptional mechanical, thermal and electrical properties of CNM. The synthesis of CNM requires three inputs: a heat source, a carbon source and a catalyst. Recently flame synthesis method is generally accepted for the preparation of CNM (Merchan-Merchan et al., 2010). Flame synthesis method has the advantages of high energy efficiency and low cost, and can produce CNM rapidly in large quantities. It provides an effective way for the commercial production of carbon nanomaterials.
So far, many researches have been carried out on soot morphology and formation mechanism in flames. The formation of soot in flames has a lot in common with the synthesis of carbon nanomaterials by flame method. The growth of single-wall carbon nanotubes in the post-flame gases can be described by the formation of small discrete particles with a progression toward carbon nanotubes inception and growth in the upper region of the flame (Merchan-Merchan et al., 2010). This formation pathway is very similar to that encountered in soot formation. In flames carbon-based materials and related nanostructures can be synthesized within the sooting zones. Understanding the formation mechanism of soot is not only helpful for reducing the emission of soot and its adverse effects on human health and environment, but also good for producing carbon-based products under controlled conditions.
In this review, the latest research progress on the effects of additives, fuel type and flame temperature on the morphology evolution and nanostructure of soot in diffusion flames is introduced. The formation mechanism of soot in diffusion flames is also discussed.
Additives affect soot formation mainly through dilution effect, thermal effect and chemical effect. Studies over the past few decades have provided important information on the effect of H2 and CO addition (Dearden and Long, 1968; Du et al., 1995; Gülder et al., 1996; Guo et al., 2006, Guo et al., 2009). However, most of the previous studies did not take account of soot nanostructure. In recent years, much attention has been paid on the nanostructure of soot. Dai et al. (Dai et al., 2020) studied the effect of CO addition in the ethylene diffusion flame. The results indicated that CO chemically promoted soot formation and the dilution effect of CO addition monotonically reduced soot formation. Lin et al. (Lin et al., 2020) investigated soot formation in laminar co-flow ethylene diffusion flames with different H2 addition ratios by Fourier transform infrared spectroscopy (FTIR) and field emission scanning electron microscopy (FESEM). The results show that the addition of H2 promotes the formation and growth of soot precursors. A distinct and dense fine fiber network structure of soot was found at the upper part of the ethylene flame, demonstrating that the oxidation of soot in the hydrogen-doped ethylene flame was enhanced with abundant soot branches oxidized and more straight chain soot produced. Li et al. (Li et al., 2021) used the method of thermophoretic sampling and transmission electron microscopy (TEM) to study the effect of CO/H2/N2 addition on the morphological evolutions and nanostructures of soot in ethylene diffusion flames. Compared with N2 addition, the addition of CO/H2 caused greater fringe length, smaller fringe tortuosity and inter-fringe spacing for soot particles, resulting in more orderly and compact lattice structure. CO addition promoted the production of the key intermediate of acetylene. On the other hand, CO addition enriched H radical dominantly through CO + OH = CO2 + H, promoting the formation and surface growth of soot. Besides, the soot nanoparticles were more graphitized in the H2-doped flame with the enhancement of C2H2 production and the increase of pyrene concentration. In the flame tip region, the enhancement of oxidation contributed to the decrease of the fringe lengths of the particles.
In addition to the above common additives, the effects of some special additives on soot formation are also studied. Ying et al. (Ying and Liu, 2018) suggested that the soot particles in n-butanol-doped ethylene inverse diffusion flames with the dilution of CO2 possessed larger fringe tortuosity, shorter fringe length, and higher oxidation reactivity. Before too long, Ying et al. (Ying and Liu, 2019) studied soot properties in ethylene inverse diffusion flames added with water. Results illustrated that soot nanostructure had an increased carbonization degree resulting from larger fringe length, lower fringe tortuosity and smaller interlayer spacing of soot when H2O is doped. Alrefaai et al. (Alrefaai et al., 2018) investigated the characteristics of soot in diesel flames doped with dicyclopentadiene. It was found that the addition of dicyclopentadiene caused the increase of the content of hydrogen, oxygen and aliphatic groups, the enhancement of the reactivity, and the diminishment of the content of aromatic groups for soot particles. A similar phenomenon was found by Botero et al. (Botero et al., 2019). Salamanca et al. (Salamanca et al., 2020) studied the impact of three cyclic fuels, namely cyclohexene (C6H10), cyclopentene (C5H8), and methylcyclohexane (C7H14), on the nanostructure of soot in n-heptane (C7H16) diffusion flames. The fringe tortuosity of soot was increased in n-heptane flames with cyclopentene addition, indicating that cyclopentene could be used to incorporate curvature in different carbonaceous structures to modify their properties. The formation of soot nanoparticles was enhanced with the addition of cyclic fuels because the addition of cyclic fuels results in quicker formation of the first aromatic ring than in pure aliphatic flames. The double-bond in the cyclic fuels had a more significant effect than the methyl substitution. The five-membered rings played a crucial role in the formation of incipient carbon nanoparticles. Earlier, Wang et al. (Wang et al., 2013) found that the relative content of aliphatic C-H groups was an important factor affecting the soot oxidation reactivity, and its importance was higher than that of C-OH and C=O groups. The addition of oxygenated compounds yields more curved and disordered nanostructures of soot (Abboud et al., 2018).
In sum, the additives can change the concentration of intermediate species and radicals (e.g. H, OH, benzene) important for soot generation. These intermediate species and radicals are closely related to the nanostructure of soot (e.g., fringe length, interlayer spacing, fringe tortuosity). The above results are helpful for the modification of the structure of carbon nanomaterials produced in flames.
Temperature is an important factor not only for the nucleation and growth of soot particles, but also for the production of carbon nanomaterials. Understanding the effect of temperature on soot formation is beneficial for the development of the technology (flame method) of carbon nanomaterials production.
The high pyrolysis rate of fuel and the rapid formation of polycyclic aromatic hydrocarbons (PAHs) are mainly caused by high initial temperature (Xu et al., 2018). High initial temperature also promotes the nucleation process of soot (Mahmoud et al., 2019). The effect of gas preheat temperature on soot formation was investigated by Qi et al. (Qi et al., 2021). The soot temperature increased with higher gas preheat temperature, leading to more violent soot formation and oxidation. Chu et al. (Chu et al., 2020) investigated the effects of different reactant temperatures on soot nanostructure. Three temperatures (300, 473, and 673 K) were applied. The results showed that increasing the temperature promoted soot aggregation and generated more mature primary particles. When the reactant temperature was relatively high (673 K), soot had more curved nanostructures. The deceleration of soot surface growth could be explained by surface aging, which was characterized by the increase in curved nanostructures. In addition, the dehydrogenation process, which contributes to soot maturity and graphitic shell formation, is sensitive to flame temperature (Kholghy et al., 2016). More than 100 polycyclic aromatic hydrocarbons (PAHs) cluster together to form liquid-like particles in flames with temperatures greater than 1,350 K (Dobbins, 2002). To improve the understanding of soot formation process, much attention has been paid to soot morphology and internal nanostructure by utilizing advanced measurement methods in recent years. Improving the accuracy of measurement is very important. For example, the acquisition of Raman spectrum for sample characterization can be interfered by the fluorescence of PAHs contained in volatile organic fraction (VOF). VOF refers to the organic fractions (PAHs or saturated/unsaturated hydrocarbons), which are prone to be volatile when heated under inert atmosphere, loosely attached to the surface of soot. Liu et al. (Liu et al., 2021) revealed that VOF could be effectively removed from the soot surface when the devolatilization temperature was 650°C.
In general, adjusting the amount and type of diluents in streams is the main method to change the temperature of diffusion flames. As a result, the effect of dilution is likely to interfere with the effect of temperature (Wang and Chung, 2019). The relative influence of temperature and dilution has been identified in earlier research (Guo et al., 2002). Since CO and N2 have similar thermal and transport properties, the addition of them has similar thermal and dilution effects (Guo et al., 2009). Higher flame temperature promotes the carbonization of soot particles and the formation of PAHs with higher planarity which reduces steric hindrance and allows the PAHs to stack (Martin et al., 2019). Raj et al. (Raj, 2019) suggested that the conversion of a planar PAH to a curved one through ring addition at an armchair site had a rapid rate at temperatures below 2,000 K. The temperature effect on soot formation in laminar diffusion flames was investigated by Davis et al. (Davis et al., 2020). The curvatures of the PAHs were reduced with the increase in flame temperature. The layer stacking of PAHs was more efficient with higher content of stacked fringes so that the PAHs became more polarizable and geometrically uniform. The curved PAHs that form young soot particles converted to graphitic structures forming mature soot aggregates. In low temperature flames, large amorphous particles with short fringe length and high fringe tortuosity were produced. In high temperature flames, soot aggregates with long fringe length and low fringe tortuosity were produced. Chu et al. (Chu et al., 2019) studied the properties of soot particles in co-flow diffusion flames with different oxygen concentrations. The results showed that soot particles along the flame centerline were oxidized into dense flocculent and fibrous mesh structures when the flame temperature was increased. The flame temperature increased sharply with the increase in flame heights, leading to the soot aggregates being oxidized to loose chain-like agglomerates. Mao et al. (Mao et al., 2017) conducted a series of ReaxFF molecular dynamics simulations and the results suggested that the PAHs developed into incipient soot particles by physical nucleation at 400 K and the chemical nucleation dominated when the temperature was 2,500 K.
All in all, temperature is an important parameter for entire soot formation process. High initial temperature results in high pyrolysis rate of fuel. Thermal effects of the additives change the flame temperature which influences the formation of PAHs and the nanostructure of soot particles. Yet further study remains to be conducted for the functional relationship between temperature and soot morphology parameters.
The significant amount of CO with the existence of H2 is a prerequisite factor for the growth of carbon nanomaterials (Hall et al., 2011). Different pyrolysis products generated from different fuels can affect soot nanostructures. In general, acetylene and aromatics make soot more graphitic. The influence of oxygen-containing functional groups on soot morphology depends on their locations in the molecules (Westbrook et al., 2006; Barrientos et al., 2013; Das et al., 2015). The effect of nitrogen-containing compounds on soot formation was investigated by Montgomery et al. (Montgomery et al., 2021). Amines had lower sooting tendencies than the structurally analogous hydrocarbons and oxygenates.
Xu et al. (Xu et al., 2020) found that cyclopentane, with a five-membered ring in the molecule, generated more soot than cyclohexane in counterflow diffusion flames because more odd carbon radicals (cyclopentadienyl and allyl, which are efficient aromatic precursors) were produced in cyclopentane flames. The aliphatic bond between the aromatic sub-units enhances the clustering of PAHs and decreases the dissociation effect due to increasing temperature (Iavarone et al., 2017). Influence of oxygenated compounds concentration on sooting propensity was studied by Abboud et al. (Abboud et al., 2017). The light extinction measurement (LEM) was used to measure the soot volume fraction. Compared with biodiesel, soot derived from surrogate diesel flames displayed less ordered graphite-like structure and contained more amorphous carbon. It was also found that adding oxygenated compounds to a diesel surrogate fuel reduced the tendency of soot production. In another study, similar sooting tendency was found in diesel flames with the addition of oxygenated compounds by Wei et al. (Wei et al., 2020). Barrientos et al. (Barrientos et al., 2015) found that shorter alkyl chain length suppressed the formation of soot and promoted the generation of less ordered soot structure. Botero et al. (Botero et al., 2016) studied the characteristics of soot particles generated from different fuels including heptane, toluene, the mixture of heptane and toluene and a commercial gasoline in laminar diffusion flames. It was found that the primary soot particles generated from the heptane flame were the smallest and the soot produced from the toluene flame showed longer, less curved fringes and more stacked layers. The properties of soot particles generated in n-decane and RP-3 kerosene flames were compared by Abdalla et al. (Abdalla et al., 2020). The results showed that soot generated from RP-3 kerosene had smaller inter-planar spacing and fewer layers. Fuels with lower decomposition temperatures can more easily generate enough local heat to complete the reactions in the synthesis process and create materials of high porosity and good crystallinity, and also prevent particle agglomeration (Parauha et al., 2021).
Thus, fuel molecular structures such as the length of the carbon chain, H/C ratio, the fuel unsaturation and the cyclic ring structure play a vital role to the sooting tendency and soot nanostructure of a combustion system. Also, the role of fuel type is important for the flame synthesis of carbon nanomaterials. In the design of combustion-based synthesis systems, the presence of suitable hydrocarbons must be taken into account to ensure the quality and repeatability of the resulting products. The results of the effect of fuel type on soot formation is valuable for the choice of the appropriate fuel to produce carbon-based materials.
In this review, the effects of additives, temperature and fuel type on the evolution of soot nanostructure and morphology are introduced. The formation of soot is a complex physical and chemical process. Although a lot of research progress has been made in the field of soot formation, there are still some unsolved problems and challenges. Further research is needed to deeply clarify the formation mechanism of soot, which can not only achieve reduction of combustion emissions, but also provide theoretical support for regulating and controlling the production of carbon nanomaterials since soot formation in flames is very similar to the synthesis of carbon nanomaterials by flame method. More effort should be made to develop the modeling capabilities of soot formation and carbon nanomaterials production. Effective measurement techniques and sampling methods need to be further developed so that more detailed information and parameters of soot particles can be obtained accurately, which is beneficial to the precise control of carbon nanomaterials synthesis in flames.
JX proposed the conception of the study and edited the article. GY drafted the manuscript. JC and ZG checked the article.
This work was supported by the National Natural Science Foundation of China (grant number 51706107) and the Natural Science Foundation of Jiangsu Province (grant number BK20181385).
The authors declare that the research was conducted in the absence of any commercial or financial relationships that could be construed as a potential conflict of interest.
Abboud, J., Schobing, J., Legros, G., Bonnety, J., Tschamber, V., Brillard, A., et al. (2017). Impacts of Oxygenated Compounds Concentration on Sooting Propensities and Soot Oxidative Reactivity: Application to Diesel and Biodiesel Surrogates. Fuel 193, 241–253. doi:10.1016/j.fuel.2016.12.034
Abboud, J., Schobing, J., Legros, G., Matynia, A., Bonnety, J., Tschamber, V., et al. (2018). Impacts of Ester’s Carbon Chain Length and Concentration on Sooting Propensities and Soot Oxidative Reactivity: Application to Diesel and Biodiesel Surrogates. Fuel 222, 586–598. doi:10.1016/j.fuel.2018.02.103
Abdalla, A. O. G., Ying, Y., Jiang, B., He, X., and Liu, D. (2020). Comparative Study on Characteristics of Soot from N-Decane and RP-3 Kerosene normal/inverse Diffusion Flames. J. Energ. Inst. 93, 62–75. doi:10.1016/j.joei.2019.04.008
Alrefaai, M. M., Guerrero Peña, G. D. J., Raj, A., Stephen, S., Anjana, T., and Dindi, A. (2018). Impact of Dicyclopentadiene Addition to Diesel on Cetane Number, Sooting Propensity, and Soot Characteristics. Fuel 216, 110–120. doi:10.1016/j.fuel.2017.11.145
Barrientos, E. J., Lapuerta, M., and Boehman, A. L. (2013). Group Additivity in Soot Formation for the Example of C-5 Oxygenated Hydrocarbon Fuels. Combustion and Flame 160, 1484–1498. doi:10.1016/j.combustflame.2013.02.024
Barrientos, E., Maricq, M., Boehman, A., and Anderson, J. (2015). “Impact of Ester Structures on the Soot Characteristics and Soot Oxidative Reactivity of Biodiesel,” in SAE 2015 World Congress, Detroit, MI, April 14, 2015. doi:10.4271/2015-01-1080
Botero, M. L., Chen, D., González-Calera, S., Jefferson, D., and Kraft, M. (2016). HRTEM Evaluation of Soot Particles Produced by the Non-premixed Combustion of Liquid Fuels. Carbon 96, 459–473. doi:10.1016/j.carbon.2015.09.077
Botero, M. L., Sheng, Y., Akroyd, J., Martin, J., Dreyer, J. A. H., Yang, W., et al. (2019). Internal Structure of Soot Particles in a Diffusion Flame. Carbon 141, 635–642. doi:10.1016/j.carbon.2018.09.063
Chu, C., Naseri, A., Mitra, T., Dadsetan, M., Sediako, A., and Thomson, M. J. (2021). The Effect of Elevated Reactant Temperatures on Soot Nanostructures in a Coflow Diffusion Ethylene Flame. Proc. Combustion Inst. 38, 2525–2532. doi:10.1016/j.proci.2020.06.348
Chu, H., Han, W., Cao, W., Tao, C., Raza, M., and Chen, L. (2019). Experimental Investigation of Soot Morphology and Primary Particle Size along Axial and Radial Direction of an Ethylene Diffusion Flame via Electron Microscopy. J. Energ. Inst. 92, 1294–1302. doi:10.1016/j.joei.2018.10.005
Dai, W., Yan, F., Xu, L., Zhou, M., and Wang, Y. (2020). Effects of Carbon Monoxide Addition on the Sooting Characteristics of Ethylene and Propane Counterflow Diffusion Flames. Fuel 271, 117674. doi:10.1016/j.fuel.2020.117674
Das, D. D., McEnally, C. S., and Pfefferle, L. D. (2015). Sooting Tendencies of Unsaturated Esters in Nonpremixed Flames. Combustion Flame 162, 1489–1497. doi:10.1016/j.combustflame.2014.11.012
Davis, J., Molnar, E., and Novosselov, I. (2020). Nanostructure Transition of Young Soot Aggregates to Mature Soot Aggregates in Diluted Diffusion Flames. Carbon 159, 255–265. doi:10.1016/j.carbon.2019.12.043
Dearden, P., and Long, R. (1968). Soot Formation in Ethylene and Propane Diffusion Flames. J. Appl. Chem. 18, 243–251. doi:10.1002/jctb.5010180805
Dobbins, R. A. (2002). Soot Inception Temperature and the Carbonization Rate of Precursor Particles. Combustion Flame 130, 204–214. doi:10.1016/S0010-2180(02)00374-7
Du, D. X., Axelbaum, R. L., and Law, C. K. (1995). Soot Formation in Strained Diffusion Flames with Gaseous Additives. Combustion Flame 102, 11–20. doi:10.1016/0010-2180(95)00043-6
Gülder, Ö. L., Snelling, D. R., and Sawchuk, R. A. (1996). Influence of Hydrogen Addition to Fuel on Temperature Field and Soot Formation in Diffusion Flames. Symp. (International) Combustion 26, 2351–2358. doi:10.1016/S0082-0784(96)80064-6
Guo, H., Liu, F., Smallwood, G. J., and Gülder, Ö. L. (2002). A Numerical Study of the Influence of Transport Properties of Inert Diluents on Soot Formation in a Coflow Laminar Ethylene/air Diffusion Flame. Proc. Combustion Inst. 29, 2359–2365. doi:10.1016/S1540-7489(02)80287-5
Guo, H., Liu, F., Smallwood, G. J., and Gülder, Ö. L. (2006). Numerical Study on the Influence of Hydrogen Addition on Soot Formation in a Laminar Ethylene-Air Diffusion Flame. Combustion and Flame 145, 324–338. doi:10.1016/j.combustflame.2005.10.016
Guo, H., Thomson, K. A., and Smallwood, G. J. (2009). On the Effect of Carbon Monoxide Addition on Soot Formation in a Laminar Ethylene/air Coflow Diffusion Flame. Combustion and Flame 156, 1135–1142. doi:10.1016/j.combustflame.2009.01.006
Hall, B., Zhuo, C., Levendis, Y. A., and Richter, H. (2011). Influence of the Fuel Structure on the Flame Synthesis of Carbon Nanomaterials. Carbon 49, 3412–3423. doi:10.1016/j.carbon.2011.04.036
Iavarone, S., Pascazio, L., Sirignano, M., De Candia, A., Fierro, A., de Arcangelis, L., et al. (2017). Molecular Dynamics Simulations of Incipient Carbonaceous Nanoparticle Formation at Flame Conditions. Combustion Theor. Model. 21, 49–61. doi:10.1080/13647830.2016.1242156
Kholghy, M. R., Veshkini, A., and Thomson, M. J. (2016). The Core-Shell Internal Nanostructure of Soot - A Criterion to Model Soot Maturity. Carbon 100, 508–536. doi:10.1016/j.carbon.2016.01.022
Li, Q., Wang, L., Yan, Z., Liu, H., and Huang, Z. (2021). Effects of CO/H2/N2 Addition on the Soot Morphology and Nanostructure in Laminar Co-flow Ethylene Diffusion Flame. J. Energ. Inst. 95, 8–17. doi:10.1016/j.joei.2020.12.001
Lin, Y., Zhu, B., Chen, J., Wu, J., Lu, K., Gu, M., et al. (2020). Study of Soot Functional Groups and Morphological Characteristics in Laminar Coflow Methane and Ethylene Diffusion Flames with Hydrogen Addition. Fuel 279, 118474. doi:10.1016/j.fuel.2020.118474
Liu, P., Ahmad, H., Mei, B., Jiang, S., You, B., and Li, Y. (2021). Effects of Devolatilization Temperature on Chemical Structure and Oxidation Reactivity of Soot Sampled from a Coflow Diffusion Ethylene Flame. Fuel 293, 120424. doi:10.1016/j.fuel.2021.120424
Mahmoud, N. M., Yan, F., and Wang, Y. (2019). Effects of Fuel Inlet Boundary Condition on Aromatic Species Formation in Coflow Diffusion Flames. J. Energ. Inst. 92, 288–297. doi:10.1016/j.joei.2018.01.007
Mao, Q., van Duin, A. C. T., and Luo, K. H. (2017). Formation of Incipient Soot Particles from Polycyclic Aromatic Hydrocarbons: A ReaxFF Molecular Dynamics Study. Carbon 121, 380–388. doi:10.1016/j.carbon.2017.06.009
Martin, J. W., Bowal, K., Menon, A., Slavchov, R. I., Akroyd, J., Mosbach, S., et al. (2019). Polar Curved Polycyclic Aromatic Hydrocarbons in Soot Formation. Proc. Combustion Inst. 37, 1117–1123. doi:10.1016/j.proci.2018.05.046
Merchan-Merchan, W., Saveliev, A. V., Kennedy, L., and Jimenez, W. C. (2010). Combustion Synthesis of Carbon Nanotubes and Related Nanostructures. Prog. Energ. Combustion Sci. 36, 696–727. doi:10.1016/j.pecs.2010.02.005
Montgomery, M. J., Zhu, J., Pfefferle, L. D., and McEnally, C. S. (2021). Amines Have Lower Sooting Tendencies Than Analogous Alkanes, Alcohols, and Ethers. Combustion Flame 227, 335–345. doi:10.1016/j.combustflame.2021.01.016
Mykhailiv, O., Zubyk, H., and Plonska-Brzezinska, M. E. (2017). Carbon Nano-Onions: Unique Carbon Nanostructures with Fascinating Properties and Their Potential Applications. Inorg. Chim. Acta 468, 49–66. doi:10.1016/j.ica.2017.07.021
Olariu, M., Arcire, A., and Plonska-Brzezinska, M. E. (2017). Controlled Trapping of Onion-like Carbon (OLC) via Dielectrophoresis. J. Elec. Materi. 46, 443–450. doi:10.1007/s11664-016-4870-1
Parauha, Y. R., Sahu, V., and Dhoble, S. J. (2021). Prospective of Combustion Method for Preparation of Nanomaterials: A challenge. Mater. Sci. Eng. B 267, 115054. doi:10.1016/j.mseb.2021.115054
Qi, S., Sun, Z., Wang, Z., Liu, Y., He, Y., Liu, S., et al. (2021). Effects of Gas Preheat Temperature on Soot Formation in Co-flow Methane and Ethylene Diffusion Flames. Proc. Combustion Inst. 38, 1225–1232. doi:10.1016/j.proci.2020.08.034
Raj, A. (2019). Structural Effects on the Growth of Large Polycyclic Aromatic Hydrocarbons by C2H2. Combustion Flame 204, 331–340. doi:10.1016/j.combustflame.2019.03.027
Richter, H., and Howard, J. B. (2000). Formation of Polycyclic Aromatic Hydrocarbons and Their Growth to Soot-A Review of Chemical Reaction Pathways. Prog. Energ. Combustion Sci. 26, 565–608. doi:10.1016/S0360-1285(00)00009-5
Salamanca, M., Botero, M. L., Martin, J. W., Dreyer, J. A. H., Akroyd, J., and Kraft, M. (2020). The Impact of Cyclic Fuels on the Formation and Structure of Soot. Combustion Flame 219, 1–12. doi:10.1016/j.combustflame.2020.04.026
Wang, L., Song, C., Song, J., Lv, G., Pang, H., and Zhang, W. (2013). Aliphatic C-H and Oxygenated Surface Functional Groups of Diesel in-cylinder Soot: Characterizations and Impact on Soot Oxidation Behavior. Proc. Combustion Inst. 34, 3099–3106. doi:10.1016/j.proci.2012.07.052
Wang, Y., and Chung, S. H. (2019). Soot formation in laminar counterflow flames. Prog. Energy Combust. Sci. 74, 152–238. doi:10.1016/j.pecs.2019.05.003
Wei, J., Zeng, Y., Pan, M., Zhuang, Y., Qiu, L., Zhou, T., et al. (2020). Morphology Analysis of Soot Particles from a Modern Diesel Engine Fueled with Different Types of Oxygenated Fuels. Fuel 267, 117248. doi:10.1016/j.fuel.2020.117248
Westbrook, C. K., Pitz, W. J., and Curran, H. J. (2006). Chemical Kinetic Modeling Study of the Effects of Oxygenated Hydrocarbons on Soot Emissions from Diesel Engines. J. Phys. Chem. A. 110, 6912–6922. doi:10.1021/jp056362g
Xu, L., Yan, F., and Wang, Y. (2020). A Comparative Study of the Sooting Tendencies of Various C5-C8 Alkanes, Alkenes and Cycloalkanes in Counterflow Diffusion Flames. Appl. Energ. Combustion Sci. 1-4, 100007. doi:10.1016/j.jaecs.2020.100007
Xu, L., Yan, F., Zhou, M., Wang, Y., and Chung, S. H. (2018). Experimental and Soot Modeling Studies of Ethylene Counterflow Diffusion Flames: Non-monotonic Influence of the Oxidizer Composition on Soot Formation. Combustion Flame 197, 304–318. doi:10.1016/j.combustflame.2018.08.011
Ying, Y., and Liu, D. (2019). Effects of Water Addition on Soot Properties in Ethylene Inverse Diffusion Flames. Fuel 247, 187–197. doi:10.1016/j.fuel.2019.03.034
Keywords: soot, nanostructure, formation characteristics, diffusion flame, carbon nanomaterials
Citation: Xi J, Yang G, Cai J and Gu Z (2021) A Review of Recent Research Results on Soot: The Formation of a Kind of Carbon-Based Material in Flames. Front. Mater. 8:695485. doi: 10.3389/fmats.2021.695485
Received: 15 April 2021; Accepted: 10 May 2021;
Published: 24 May 2021.
Edited by:
Xingtao Xu, National Institute for Materials Science, JapanReviewed by:
Lichao Ge, Hohai University, ChinaCopyright © 2021 Xi, Yang, Cai and Gu. This is an open-access article distributed under the terms of the Creative Commons Attribution License (CC BY). The use, distribution or reproduction in other forums is permitted, provided the original author(s) and the copyright owner(s) are credited and that the original publication in this journal is cited, in accordance with accepted academic practice. No use, distribution or reproduction is permitted which does not comply with these terms.
*Correspondence: Jianfei Xi, amlhbmZlaXhpQG5qbnUuZWR1LmNu; Zhongzhu Gu, Z3V6aG9uZ3podUBuam51LmVkdS5jbg==
Disclaimer: All claims expressed in this article are solely those of the authors and do not necessarily represent those of their affiliated organizations, or those of the publisher, the editors and the reviewers. Any product that may be evaluated in this article or claim that may be made by its manufacturer is not guaranteed or endorsed by the publisher.
Research integrity at Frontiers
Learn more about the work of our research integrity team to safeguard the quality of each article we publish.