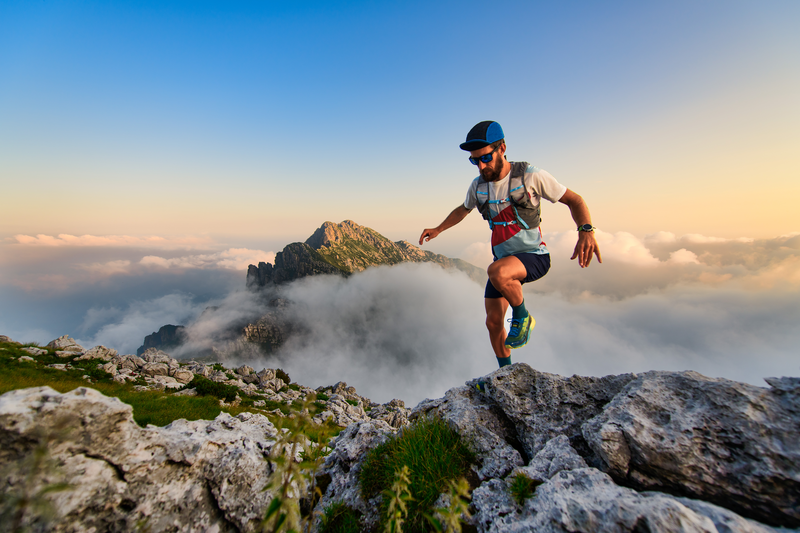
94% of researchers rate our articles as excellent or good
Learn more about the work of our research integrity team to safeguard the quality of each article we publish.
Find out more
ORIGINAL RESEARCH article
Front. Mater. , 17 June 2021
Sec. Biomaterials
Volume 8 - 2021 | https://doi.org/10.3389/fmats.2021.682844
Background: The short half-life of TRAIL (tumor necrosis factor–related apoptosis-inducing ligand) greatly limits its clinical application. This study was aimed to improve its potency on liver fibrosis through PEG (polyethylene glycol) modification prolonging the half-life of TRAIL.
Methods: PEG, TRAIL, and the chemically synthesized complex PEG-TRAIL were used to treat 3T3 and LX-2 cells and liver fibrotic mice. In vitro, cell viability, apoptosis, and fibrosis were investigated using CCK-8 (cell counting kit-8) assay, flow cytometry, and Western blotting, respectively. In vivo, Sirius red staining, immunohistochemistry, and α-SMA (α-smooth muscle actin)/TUNEL (terminal deoxynucleotidyl transferase dUTP [2'-deoxyuridine 5'-triphosphate] nick end labeling) double-labeling immunofluorescence (IF) were performed after various treatments for liver fibrotic mice. The fibrotic liver was subjected to DR4 (death receptor 4)/TRAIL double-labeling IF to assess the retention of TRAIL enhanced by PEGylation.
Results: The cells treated with PEG-TRAIL showed lower cell viability, higher apoptosis level, and stronger anti-fibrotic effect compared with PEG or TRAIL treatment. In vivo, PEGylated TRAIL exhibited a longer circulation than TRAIL did. Compared with TRAIL treatment, PEG-TRAIL caused a significant reduction of α-SMA and a markedly increase of apoptotic aHSCs. PEGylation is more likely to prolong the retention of TRAIL in circulation and enhance the possibility to target aHSCs and DR4-positive (DR4+) cells in the liver.
Conclusion: PEG-TRAIL presents better anti-fibrotic and proapoptotic effects, for which, the prolonged circulation half-life in vivo may account. The PEG-TRAIL may serve as a new clinical therapeutic for liver fibrosis in the future.
Liver fibrosis, a serious chronic liver disorder characterized by the superabundant extracellular matrix (ECM) accumulation, which induces various liver injury and then leads to substantial morbidity and mortality (Bansal et al., 2011; Hasenfuss et al., 2014). Despite tremendous efforts have been made in experimental and clinical studies (Beljaars et al., 1999; Poynard et al., 2000; Inagaki et al., 2003), no satisfactory anti-fibrosis drug has been developed for managing hepatic fibrosis clinically until now because of the poor drug effectiveness caused by short half-life and weak liver target ability, as well as the toxic side effects caused by the drug accumulation in nontarget tissues or cells in vivo (Beaussier et al., 2005). Thus, exploring the targeted and long-term drug delivery system has become an important strategy for hepatic fibrosis therapy, which is the key to make drug delivery specifically targeting at the specific populations of hepatic cells (Li et al., 2012). Particularly, activated hepatic stellate cells (aHSCs) are the currently preferred targeted cells in anti-liver fibrotic therapy, and a variety of targeted drug delivery systems for HSCs have been explored and attempted for treating hepatic fibrosis in recent years (Sato et al., 2008; Bansal et al., 2011).
TRAIL (tumor necrosis factor (TNF)–related apoptosis-inducing ligand) is an important member of TNF superfamily. It is upregulated in aHSCs and has been proposed as a favorable candidate for therapy of many diseases because it can specifically induce cellular apoptosis of some tumor cells, aHSCs, and virus-infected hepatocytes by activating caspase-3 (Holoch and Griffith, 2009; Xu et al., 2016). Based on this, focusing the application of TRAIL genes is likely to provide a feasible technology to treat liver fibrosis. For example, Xu et al. (2016) have revealed that TRAIL-induced HSC apoptosis is a potential critical mechanism of blocking and reversing liver fibrosis. Although TRAIL triggers apoptosis in various specific cell types, most normal cells can escape from TRAIL-induced apoptosis (Yoon and Gores, 2002; Hellwig and Rehm, 2012).
Previous studies have confirmed the poor pharmacokinetics of TRAIL characterized by the half-life of TRAIL (∼3 min to 1.1 h in rats or mice and ∼23 min to 30 min in nonhuman primates) (Kelley et al., 2001; Kim et al., 2011c; Lim et al., 2011), which has been a major obstacle for the preclinical or clinical application of TRAIL. The latest clinical research studies revealed that TRAIL-based cancer therapy including recombinant TRAIL or agonistic monoclonal antibodies against death receptors failed to show clinical efficacy in phase II clinical studies (Lemke et al., 2014; Holland, 2014), which is mainly because of the short plasma half-life of recombinant TRAIL in the human body (Lemke et al., 2014). Therefore, the short half-life of TRAIL in vivo that indirectly enhances the resistance of aHSCs to TRAIL is also a main hindrance in enabling the clinically therapeutic application of TRAIL as a powerful tool in treating liver fibrosis.
To prolong the activity and in vivo half-life of targeting drugs, various multiple particulate systems have been developed, including liposomal systems (Martinez-Lostao et al., 2010), nanoparticles (Kim et al., 2011b; Chu et al., 2020; Wang et al., 2021), microspheres (Kim et al., 2011c), and polymeric complexes (Hu et al., 2015). Of which, PEGylation and fusion with human serum albumin are two of most common protocols (AlQahtani et al., 2019). Especially, PEGylation is a novel delivery system utilized recently (Wang et al., 2018), which is a process involving the covalent attachment of polyethylene glycol (PEG) polymer chains to varying biomolecules, such as small molecules, proteins, peptides, and antibody fragments. Generally, the formed PEGylated analogs usually present better pharmaceutical and therapeutic properties (Harris and Chess, 2003; Veronese and Pasut, 2005; Wang et al., 2018). Typically, the serum half-life of PEGylated protein is significantly prolonged via adjusting the size of conjugated PEG (Kim et al., 2011a). In the present study, to address the poor clinical potency of recombinant TRAIL in hepatic fibrosis clinical studies, we utilized PEG to modify TRAIL to form the PEG-TRAIL delivery system and explored anti-fibrotic potency of this novel delivery with a view toward improving anti-fibrotic and apoptosis inductive effects both in vitro and in vivo (Supplementary Figure S1). Related mechanistic studies were also implemented to reveal the potential regulatory mechanism of PEG-TRAIL in the development of liver fibrosis. This study offers a novel approach for addressing the poor clinical efficacy of cytokine preparation caused by short half-life and may provide a novel drug delivery method for clinical anti-liver fibrosis therapy.
The recombinant human TRAIL was purchased from Cloud Clone Co., Ltd, United States (#RPA139hu01). Its amino acid (AA) sequence (168 AAs) is as follows: VRERGPQRVAAHITGTRGRSNTLSSPNSKNEKALGRKINSWESSRSGHSFLSNLHLRNGE-LVIHEKGFYYIYSQTYFRFQEEIKENTKNDKQMVQYIYKYTSYPDPILLMKSARNSCWSKDAEYGLYSIYQGGIFELKENDRIFVSVTNEHLIDMDHEASFFGAFLVG. For more information, please check the official website: https://www.cloud-clone.us/protein/Tumor-Necrosis-Factor-Related-Apoptosis-Inducing-Ligand-TRAIL-21277.htm. The mPEG5000-ALD was purchased from Shanghai Ziqi Biotech (Shanghai, China). Sodium cyanoborohydride and sodium acetate anhydrous were purchased from Sinopharm Chemical Reagent Co., Ltd (Shanghai, China). Acetic acid glacial was purchased from Shanghai Heqi Chemical Technology Co., Ltd. Human LX-2 cells and mouse 3T3 cells were provided by the Cell Bank of Chinese Academy of Sciences (Shanghai, China). All animal procedures were approved by the Ethics Committee of Shanghai East Hospital, Tongji University.
According to the methods mentioned in the literature (Chae et al., 2010; Kim et al., 2011c), PEG-TRAIL was prepared. Simply, 500 μl of TRAIL (2.56 nmol), 446.4 μl of mPEG-ALD (179 nmol), and 1.66 ml of sodium cyanoborohydride (20 mmol) were added to a flask that contained 5 ml sodium acetate buffer solution (pH = 5). The final solution was stirred for 12 h at 4°C and then dialyzed in 3.5 kDa dialysis bag against distilled water for 24 h, during which the distilled water was changed every ∼1 h. Western blot assay was used to confirm the successful synthesis of PEG-TRAIL.
Human LX-2 cells were cultured in Dulbecco’s Modified Eagle’s Medium (DMEM) containing 2 % FBS and 1X glutamin (Millipore, MA, United States). The purpose of designing the complex is to treat human hepatic fibrosis, but before its application in clinics, there is a long road. In addition to human cells, animals like rats and mice are still inevitably needed. Therefore, we decide to choose another cell type 3T3, which is a type of fibroblast cells that resemble the activated HSCs. Before the decision, the amino acid sequences of recombinant mouse TRAIL and recombinant human TRAIL were aligned using BLAST (https://blast.ncbi.nlm.nih.gov/Blast.cgi), and a 71 % identity was found between them (data not shown). Meantime, we noticed that some other researchers also used rhTRAIL in the treatment of mice (Marcuzzi et al., 2012). Subsequently, preliminary experiment was performed to validate the proapoptotic and anti-fibrotic effects of rhTRAIL on mouse 3T3 cells. Based on the eventual positive results, 3T3 cell line was chosen throughout the study. Mouse 3T3 cells were cultured in DMEM supplied with 10 % fetal bovine serum (FBS, Gibco, United States). All cells were cultured in a humidified incubator at 37°C containing 5 % CO2. To investigate the anti-fibrosis impacts of PEG-TRAIL, the cells were pretreated with TGF-β1 (2 ng/ml) for 48 h. Following which the cells were, respectively, treated with PEG, TRAIL, and PEG-TRAIL at the concertation of 0, 0.031, 0.063, 0.125, 0.25, and 0.5 μg/ml for 12 h. After the cells were harvested, the cell viability was determined by cell counting kit-8 (CCK-8) assay.
Cells were plated in 96-well plates at 5 × 103 cells per well and then transferred into a humidified incubator containing 5 % CO2 at 37°C. Cell viability was evaluated by adding CCK-8 reagent (Dojindo Molecular Technologies, Kimamoto, Japan) to each well. After further incubation for 4 h, the absorbance at 450 nm was detected by a microplate reader (Bio-Rad Laboratories, Inc., Hercules, CA, United States).
Prior to the apoptosis assay, the cells were treated with serum starvation for 48 h. Then the cells were cultured in the complete DMEM and harvested by centrifugation at 1000 rpm for 5 min. After being rinsed in precooled phosphate buffer saline (PBS), the cells were stained with propidium iodide and Annexin V-FITC (BD Pharmingen, United States) according to the protocol. Apoptotic cells were analyzed and quantified by a flow cytometry (FCM, BD Pharmingen).
Male C57BL/6 mice were obtained from Shanghai SLAC Laboratory Animal Co. Ltd. The liver fibrosis model was established according to our previous report (Li Q. et al., 2017). Simply, the mice were subcutaneously injected with camellia oil containing 20 % CCl4 and during the molding of liver fibrosis using CCl4 for 8 weeks. Mice with liver fibrosis were randomly divided into 3 groups (five mice in each group) and then intravenously injected with 1 μg/ml PEG, TRAIL, or PEG-TRAIL dissolved in 0.5 ml PBS twice a week. Four weeks later, the animals were anesthetized with ketamine (80 mg/kg) and xylazine (10 mg/kg) and sacrificed by cervical dislocation. The liver tissues were collected and stored at −20°C in refrigerator for further analysis. Animal experiments were conducted in the Department of Gastroenterology, Shanghai East Hospital, Tongji University. All animal procedures were approved by the Tongji University Ethical Institutional Committee of Animal Care and Use.
Rats were randomly divided into two groups (four rats in each group). Each animal was administered with the equivalent of 1 μg/ml TRAIL or corresponding to PEG-TRAIL. Samples were injected into the tail vein in a total volume of 0.5 ml. After 15 min, 30 min, 1 h, 2 h, 4 h, 8 h, 12 h, 24 h, and 48 h of sample injection, where the time points were set based on the literature (Kim et al., 2011c), the rats were anesthetized with intraperitoneal pentobarbital (50 mg/kg) and blood samples of 0.15–0.2 ml were obtained from the caudal artery. The ELISA kit (MLBIO, Shanghai, China) was used to detect the serum rhTRAIL (recombinant human TRAIL) levels. After the measurement, all rats were euthanized by CO2 exposure (approximately 50 %) and confirmed dead with criteria, including lack of breathing (for ∼10 min), pulse (for ∼10 min), and corneal reflex according to the AVMA (American Veterinary Medical Association) guidelines.
Paraffin-embedded liver tissues were sliced into 4 μm sections. For α-SMA IHC, the sections were successively deparaffinized, dehydrated, and treated with 3 % H2O2, and then were blocked with normal goat serum (Biofavor, Wuhan, China) at 37°C for 30 min. After washing with PBS, the sections were incubated with the primary α-SMA antibody at 4°C overnight and then incubated with fluorescence-labeled secondary antibody at 37°C for 1 h. Finally, the sections were rinsed with PBS and then stained with hematoxylin.
Collected liver tissues were permeabilized in 0.2 % Triton X-100 for 10 min and blocked in 10 % BSA for 1 h. For immunofluorescence double staining of α-SMA/TUNEL, the sections were incubated with the anti–α-SMA antibody (1:100 dilution; ab5831; Abcam) at 4°C overnight, followed by incubation with the corresponding secondary antibodies. TUNEL was conducted using a commercial kit (Calbiochem, San Diego, CA, United States). The sections were examined using an Olympus Inverted System (Olympus, Tokyo, Japan).
Sirius red staining was performed as the previous report (Marconi et al., 2013).
The protein was extracted from liver tissues or in vitro cells using RIPA buffer and then quantified using the BCA protein assay kit (Pierce, United States) in accordance with the manufacturer’s protocol. Following which the protein was separated by SDS-PAGE and loaded on to nitrocellulose membrane (Millipore, Bedford, MA, United States). After blocking with 5 % skim milk in TBST buffer, the membranes were incubated with the primary antibody against TRAIL (ab9959, Abcam), p53 (ab1431, Abcam), caspase-3 (ab13847, Abcam), tBid (truncated BH3 interacting domain death agonist; ab10640, Abcam), DR4 (death receptor 4; ab209412, Abcam), DR5 (death receptor 5; ab8416, Abcam), uPA (urokinase plasminogen activator; sc-59727, Santa Cruz) or PAI-1 (plasminogen activator inhibitor type-1; sc-5297, Santa Cruz), β-crystallin (ab13496, Abcam), α-SMA (α-smooth muscle actin; ab5831, Abcam), collagen I (ab34710, Abcam), and collagen III (ab7778, Abcam) or GAPDH (glyceraldehyde-3-phosphate dehydrogenase; ab8245; Abcam) at 4°C overnight, and subsequently incubated with the secondary antibodies at 25°C for 2 h. The protein bands were visualized using ECL-chemiluminescent kit (ECLplus, Thermo Fisher Scientific) and quantitated using ImageJ software (WS Rasband, ImageJ, NIH, Bethesda, MD, United States) normalized with GAPDH.
All data were calculated from at least three independent experiments and showed as mean ± standard deviations (SD). The differences among groups were analyzed by the Student’s t test or one-way analysis of variance (ANOVA). p values < 0.05 were considered significant difference in all comparisons. All results were analyzed using SPSS 18.0 software (SPSS, Chicago, IL, United States).
To verify whether the complex PEG-TRAIL was successfully prepared, Western blot assay was performed to determine the protein level and molecular weight of TRAIL and the synthesized PEG-TRAIL. As shown in Figure 1A, the molecular weight of PEG-TRAIL and TRAIL was 26 kDa and 19 kDa, respectively, indirectly suggesting the successful synthesis of PEG-TRAIL. Based on three independent tests, the synthesis efficiency of PEG-TRAIL was about 51 %. Subsequently, we investigated the effect of PEG-TRAIL on the viability and apoptosis of aHSCs by CCK-8 assay and flow cytometry. The viability of both types of cells was significantly inhibited when the concentration of PEG-TRAIL exceeded 0.125 μg/ml (p < 0.01), identifying that 0.125 μg/ml is the efficient concentration of PEG-TRAIL (Figure 1B). For 3T3 cells, PEG-TRAIL and TRAIL exerted an efficient inhibitive effect on cell viability relative to PEG (p < 0.01) and no significant difference was found between these two groups, suggesting that PEG had no or extremely low cytotoxicity. For LX-2 cells, PEG-TRAIL had more power to suppress the cell viability at a certain concentration (< 0.5 μg/ml) than TRAIL alone did (p < 0.01). Besides, no strong suppression of cell viability was found between PEG-TRAIL and TRAIL at most concentrations, suggesting that PEG-TRAIL did not exhibit better long-circulating effect than TRAIL in vitro. The result of apoptosis analysis is shown in Figures 1C,D, and we observed a significant increase of apoptotic rate in both PEG-TRAIL–treated and TRAIL-treated cells compared with the PEG group (p < 0.01) and no obvious difference existed between them. This finding was consistent with the results of CCK-8 assay. Besides, this proapoptotic effect of TRAIL and PEG-TRAIL was more pronounced in LX-2 cells, which might be caused by species specificity of rhTRAIL (Figure 1D). Overall, PEGylation showed slightly enhanced ability to reduce cell viability and enhance apoptosis by contrast with TRAIL in vitro.
FIGURE 1. PEG-TRAIL attenuates the viability and promotes apoptosis of 3T3 and LX-2 cells. (A) Successful synthesis of PEG-TRAIL was verified with Western blotting. (B) Cell viability of 3T3 and LX-2 cells treated with PEG, TRAIL, and PEG-TRAIL was measured with CCK-8 assay. (C) Cell apoptosis of 3T3 and LX-2 cells treated with PEG, TRAIL, and PEG-TRAIL was detected by flow cytometry assay. (D) Statistical result of cell apoptosis assay. *p < 0.05 and **p < 0.01 compared with PEG; #p < 0.05 and ##p < 0.01 compared with TRAIL.
The expression of some important proteins, including p53, cleaved caspase-3, tBid, DR4, DR5, uPA, and PAI-1, that are involved in TRAIL-induced apoptotic signaling pathways, were determined using Western blot assay. As a result, both PEG-TRAIL and TRAIL treatment contributed to a significant enhancement of the proapoptotic proteins (p53, caspase-3, tBid, DR4, DR5, and PAI-1) expression (p < 0.05, p < 0.01), whereas a well-marked inhibition of antiapoptotic protein (uPA) expression compared to PEG treatment (p < 0.05, p < 0.01, Figure 2A). The effect of PEG-TRAIL on the above multiple genes expression was more pronounced than TRAIL-treated cells (p < 0.05 or p < 0.01, Figure 2A). Similarly, we also observed an apparent reduction of fibrotic markers, including β-crystallin, α-SMA and collagen I, and collagen III, that are linked to the severity of liver fibrosis, in 3T3 and LX-2 cells after treatment with TRAIL or PEG-TRAIL to various extents (p < 0.05, p < 0.01), and the impact of PEG-TRAIL was more obvious relative to TRAIL in LX-2 cells (p < 0.05, p < 0.01, Figure 2B), which documented that the anti-fibrotic effect of PEG-TRAIL on activated human HSCs was greater than that of TRAIL alone. More visual results were presented in Figure 2C. Generally, the above findings further verified that PEGylation enhances the anti-fibrosis effect of TRAIL.
FIGURE 2. PEG-TRAIL alters the protein expression of apoptosis- and fibrosis-related genes in 3T3 and LX-2 cells. (A) The relative protein level of p53, cleaved caspase-3, tBid, DR4, DR5, uPA, and PAI-1 normalized to GAPDH; (B) The relative protein level of β-crystallin, α-SMA, collagen I, and collagen III normalized to GAPDH; (C) Representative blots for each protein measured with Western blotting from triplicate results. *p < 0.05 and **p < 0.01 compared with PEG; #p < 0.05 and ##p < 0.01 compared with TRAIL.
The pharmacokinetics of TRAIL and PEG-TRAIL were evaluated through the serum TRAIL of rats, and the corresponding results are shown in Figure 3. Initially, TRAIL showed a satisfactory result that its content was higher than PEG-TRAIL within 1 h. But the active TRAIL was found to be removed from the rat serum very rapidly, with a circulating half-life (t1/2) of 1.07 ± 0.06 h, whereas PEG-TRAIL had a t1/2 value of 8.01 ± 0.98 h. In addition, the AUCinf values (% h) of PEG-TRAIL in blood were also observed to be much greater than that of TRAIL. Taken together, the above findings revealed a better long-circulating effect of PEG-TRAIL than TRAIL in vivo.
FIGURE 3. Serum concentrations of rhTRAIL at the designed time points and the corresponding pharmacokinetic parameters (half-life) used in anesthetized rats.
Next, we explored the anti-fibrosis and apoptosis inductive effects of PEG-TRAIL in vivo by establishing mice models of hepatic fibrosis. After liver fibrosis was induced and intravenously treated with equal amount of PEG, TRAIL, and PEG-TRAIL, respectively, for 4 weeks later, liver tissues were collected. As shown in the images of Sirius red staining, the liver tissues in PEG-TRAIL group showed significantly decreased positive areas of collagen deposition staining compared with TRAIL, which indicated that PEG-TRAIL had more power to alleviate hepatic fibrosis (Figure 4A). α-SMA is a biomarker of hepatic fibrosis, and its expression was studied by immunohistochemistry and Western blotting. Clearly, α-SMA expression was notably reduced by TRAIL and PEG-TRAIL compared with PEG-treated group, and particularly, PEG-TRAIL caused the lowest expression of α-SMA (Figure 4A). In consistency with immunohistochemistry, similar result was also confirmed by the Western blot analysis (p < 0.01, Figure 4B). In the result of α-SMA/TUNEL double-labeling, we found that PEG-TRAIL caused more apoptotic aHSCs (α-SMA+/TUNEL+) than either PEG or TRAIL did (p < 0.01, Figures 4C,D), confirming the stronger apoptosis inductive effect of PEG-TRAIL. These manifestations revealed that the satisfactory anti-fibrosis of PEG-TRAIL might be due to the fact that PEGylation improved the long-circulating activity of TRAIL for aHSCs. Overall, PEG-TRAIL has the ability to enhance the anti-fibrosis and apoptosis inductive effects of TRAIL on aHSCs in vivo.
FIGURE 4. PEG-TRAIL enhances antifibrotic and proapoptotic effects of TRAIL in hepatic fibrosis mice models. (A) Sirius red staining and α-SMA IHC of liver tissues after treatment with PEG, TRAIL, or PEG-TRAIL. (B) Western blotting showing the protein expression level of α-SMA in the liver tissues after treatment with PEG, TRAIL, and PEG-TRAIL. (C,D) TUNEL/α-SMA double-labeling IF of liver tissues after treatment with PEG, TRAIL, or PEG-TRAIL, and the corresponding quantitative apoptosis rate of aHSCs in liver tissues (C). Arrows indicate TUNEL+/α-SMA+ cells. Scale bar: 20 μm. **p < 0.01, compared with PEG; ##p < 0.01, compared with TRAIL.
To explore the potential anti-fibrosis mechanism of PEG-TRAIL in vivo, we also performed DR4/TRAIL-R1 IF double staining. As shown in Figure 5A, it is obvious that the DR4+ (DR4-positive)/TRAIL+ (TRAIL-positive) cells in PEG-TRAIL–treated liver tissues were significantly more than those in PEG and TRAIL groups, demonstrating that PEG-TRAIL has longer retention time in the liver so that DR4+ cells can be targeted by TRAIL with greater possibility in relative to free TRAIL (Figure 5A). Then we quantitatively analyzed the intensity in the liver and found that the percentage of DR4+/TRAIL+ cells in DR4+ cells in PEG-TRAIL was approximately 56 % and 35 % more than that in PEG- or TRAIL-treated livers (p < 0.01, Figure 5B). Together, it can be concluded that PEG modification enhances the antifibrosis and apoptosis inductive effects of TRAIL by extending the circulating time of TRAIL in the serum and retaining more TRAIL in the liver of hepatic fibrotic mice.
FIGURE 5. PEG-TRAIL enhances the long-circulating efficiency of TRAIL in hepatic fibrosis mice models. (A) TRAIL/TRAIL-R1 double-labeling IF of the livers after treatment with PEG, TRAIL, or PEG-TRAIL. (B) A histogram showing the percentage of DR4+/TRAIL+ cells in DR4+ cells in liver tissues after treatment with PEG, TRAIL, or PEG-TRAIL. **p < 0.01, compared with PEG; ##p < 0.01, compared with TRAIL.
Hepatic fibrosis is a major world health problem, which is caused by the chronic liver injury (Bansal et al., 2011; Hasenfuss et al., 2014). Credible study has confirmed the importance of aHSCs in the hepatic fibrogenesis and its apoptosis is a significant mechanism for liver fibrosis improvement (Friedman, 2003). Therefore, inhibiting HSCs activation or promoting their apoptosis is an available method for treating liver fibrosis. aHSCs are associated with the regulation of various apoptosis-related molecules, including neural growth factor receptor (NGFR), NF-κB, Fas/FasL, and Bcl2/Bax. Natural killer (NK) cells were found to exhibit a strong capacity in inducing aHSCs apoptosis via NKG2DRAE1 and TRAIL/DR5 pathways (Tacke and Zimmermann, 2014), as well as an outstanding ability to alleviate liver fibrosis by targeting aHSCs in a NKG2D- and TRAIL-dependent manner (Glässner et al., 2012). Considering the important role of TRAIL in the induction of aHSC apoptosis, targeting TRAIL-mediated death may provide a promising anti-fibrotic strategy for fibrotic diseases therapy. However, unmodified recombinant TRAIL is of a relatively weak cell lethality and has an extremely short half-life (Yoon and Gores, 2002; Kim et al., 2011c; Hellwig and Rehm, 2012). Although recombinant human TRAIL has been investigated as an anticancer drug by several studies, its clinical efficacy never got along very well mainly owing to the short half-life (Jean-Charles et al., 2011; Lemke et al., 2014), which greatly limits its application in drug development.
In the current study, to enhance the pharmaceutical property of TRAIL against liver fibrosis, we chemically synthesized the complex PEG-TRAIL and performed series of biochemical experiments to evaluate the anti-fibrosis activity and fibrosis-targeting efficacy of PEG-TRAIL in vitro and in vivo. In vitro, PEG-TRAIL exerted a better ability to suppress the cell viability at a relatively low concentration by contrast with TRAIL in LX-2 but not in 3T3 cells, suggesting the strong affinity of PEG-TRAIL to aHSCs. Besides, PEG-TRAIL was also found to exhibit extremely low cytotoxicity in vitro. In fact, PEGylation is a novel approved technology and more than twenty PEGylated therapies are being explored in clinic, recently (Swierczewska et al., 2015). Considering our result and prior report, we can make sure that PEGylation is a safe and effective approach to enhance the delivery efficiency of protein drug (Figure 1B). Consistent with cell viability data, TRAIL and PEG-TRAIL induced a well-marked enhancement of both 3T3 and LX-2 cells apoptosis compared with PEG treatment, which can be observed from an obvious increase of cell apoptosis rate and the significant upregulation of proapoptotic protein expression and inhibition of antiapoptotic protein expression (Figures 1C,D, 2A,C). Also, downregulation of the main fibrotic markers (β-crystallin, α-SMA and collagen I, and collagen III), which are the main characteristics of PEG-TRAIL–treated cells (Gregorio et al., 2017), is considered to be the result of TRAIL-induced cell apoptosis and recovery from fibrosis in vitro (Figures 2A–C). What is noteworthy is that the proapoptotic and anti-fibrosis effects of PEG-TRAIL were more pronounced in LX-2 cells than in 3T3 cells, characterized by the significant increase of cell apoptosis and the well-marked reduction of fibrotic-related protein expression, compared with 3T3 cells. These findings further identify the stronger anti-fibrosis and apoptosis inductive ability of PEG-TRAIL than free TRAIL.
According to a previous study, we have known that TRAIL has a very short half-life and can be quickly cleared in vivo because of renal clearance (Xiang et al., 2004). In our in vivo study, we first examined the circulation time of PEG-TRAIL in the blood of rats. Here, the reason for selecting rats is because rats have more blood than mice, which is convenient for repeated blood test and measuring related index. The results showed that PEGylation could significantly improve the pharmacokinetic profile of TRAIL by prolonging its half-life time (Figure 3), which was highly in agreement with the result of one previous research (Kim et al., 2011c). According to the literature review by Harris et al. (2001), in addition to increasing the size and molecular weight of rhTRAIL, PEGylation may also alter the physicochemical properties of rhTRAIL, including changes in conformation, steric hindrance, changes in electrostatic binding properties, hydrophobicity, local lysine basicity, and pI (the pH at which a protein’s charge is neutral). These physical and chemical changes not only reduce systemic clearance by a number of mechanisms, including decreases in renal clearance, proteolysis, and opsonisation (macrophage uptake), and influence the binding affinity of the therapeutic protein to cellular receptors, resulting in changes in the bioactivity of the agent, but also delay the translocation of rhTRAIL into extravascular tissue and returning to the blood circulation again by diffusion (Yamaoka et al., 1994). Therefore, rhTRAIL without modification quickly ran into the circulation system, reaches its peak concentration in artery, while PEG-rhTRAIL did it relatively slow, causing the temporarily higher level of rhTRAIL in serum in 0.25 ∼ 1 h after intravenous injection.
In a previous large-scale clinical trial for liver fibrosis treatment, the safety and availability of TRAIL have been confirmed; however, the usage of TRAIL has to be terminated at the phase II of clinical trials due to the short half-life and poor efficacy (Oh et al., 2016). Herein, the half-life of TRAIL was prolonged significantly through PEGylation, which addresses a huge defect of TRAIL in clinical application and suggests that it is likely to become a new strategy to ameliorate liver fibrosis in the future. Besides, we also found that PEG-TRAIL treatment could effectively alleviate liver fibrosis in the mice animal model, as reflected in the decline of Sirius red staining intensity, as well as the decrease of α-SMA expression and α-SMA-positive cells. As we know, the intensity of Sirius red staining indirectly represents the collagen content that is the main component of ECM in liver tissues (Mahmud et al., 2007); thus, the images of Sirius red staining revealed the reduction of ECM accumulation and the improvement of liver injury under the treatment of PEG-TRAIL (Figures 4A,B). Upon the liver injury, a large number of HSCs would be activated and then exhibit an ability to enhance the expression of α-SMA and production of ECM (Sato et al., 2003; Higashi et al., 2017). Besides, it has been verified that there is a direct association between the number of α-SMA–positive cells and the reduction of fibrogenesis (Iimuro et al., 2003). Considering the reduction of ECM along with the decrease of α-SMA–positive cells in this study, we speculate that the decreased expression of collagen may have resulted from the suppression of HSCs activation induced by PEG-TRAIL. Not surprisingly, the following α-SMA/TUNEL IF double staining revealed that long circulation of PEG-TRAIL may result in the increased number of TRAIL halted on aHSCs of the liver compared with free TRAIL (Figures 4C,D). We believed it was one of the potential mechanisms for PEG-TRAIL exerting greater anti-fibrosis and apoptosis inductive ability in the animal models of liver fibrosis.
Under physiological conditions, TRAIL exerts its function by directly triggering cell apoptotic signaling in aHSCs via binding to its receptors, including DR4 and DR5. For example, the sensitivity of cancer cells would shift from one TRAIL death receptor to another upon DR4 internalization under the treatment of anticancer drugs (Bychkov et al., 2014). In the present study, the recombinant TRAIL was modified with PEG. To investigate whether the interaction between TRAIL and its receptor is associated with the inhibitive effect of PEG-TRAIL on liver fibrosis, DR4/TRAIL-R1 double IF staining was implemented. We found that the number of DR4+/TRAIL+ cells in PEG-TRAIL group was significantly greater than those in the groups only treated with TRAIL (Figure 5), which indicated that the PEGylation did notimpair TRAIL functions, but rather make it retained in the circulation for a long time, making it possible that more TRAIL molecules anchoring at their receptors on aHSCs. In particular, TRAIL-DR5 interaction was not included in the current study because TRAIL/DR5 is a well-established signal axis for aHSC apoptosis. However, the exact role of the internalized TRAIL/DR4 complex in the process of aHSCs remains unclear. Based on the finding that a higher concentration of TRAIL is necessary for DR4-mediated apoptosis (Macfarlane et al., 2005; Arabpour, 2016), we can confirm the involvement of TRAIL/DR4 interaction in aHSCs apoptosis that was not well documented in previous reports, which may provide more valuable information about the DR4-mediated signaling.
Liposomes, synthetic vesicles that consist of phospholipid membranes, are able to transport drug molecules across cell membranes and have been broadly used as a drug delivery system in basic and clinical experiments. Although further research about dosage regimen needs to be done, the existing evidence has proven that they seem to be the first choice for drug delivery systems for various diseases (Beltrán-Gracia et al., 2019). Lipid nanoparticles are a relatively new colloidal drug delivery system, and their kinetic stability and rigid morphology are major advantages over liposomes. But the liposomes or the nanoparticles can be PEGylated to further extend their circulating time and enhance their stability (Shen et al., 2018). However, researchers have raised some concerns about the safety of PEGylation because PEG itself shows toxicity at high parenteral doses and/or causes potential immunogenicity issues (Webster et al., 2009; Shiraishi and Yokoyama, 2019). Therefore, there is still a long road for PEGylation’s application in clinical use.
It is likely that all the cells that express TRAIL receptors have the access to be targeted by TRAIL. For example, one study has proven that apoptosis of pro-inflammatory Th17 cells is induced by PEGylated rhTRAIL in experimental rheumatoid arthritis mouse models (Park et al., 2017). Increased Th17 cells are a type of well-documented risk factors in liver fibrosis nowadays (Li X. et al., 2017; Milosavljevic et al., 2018; Zhou et al., 2019). However, in the present study, we can only focus on one of the key target cells which are believed to directly trigger liver fibrosis due to the limited funds and time. More detailed information about the mechanism that PEGylated rhTRAIL effectively alleviates liver fibrosis is worth further exploration.
In this study, a PEG modified complex PEG-TRAIL was synthesized and used for the treatment of hepatic fibrosis, and it exerted better anti-fibrosis and apoptosis inductive effects mainly through prolonging the half-life time of TRAIL. Based on these, we believe that PEGylation is an effective method to improve the clinical efficacy of cytokine preparation with short half-life. Overall, in spite of the potential renal toxicity and immunogenicity issues of PEGylation, PEG-TRAIL presents promising prospect in the therapy of liver fibrosis, and clinical transformation of TRAIL-based therapies for liver fibrosis in future is now expected.
The raw data supporting the conclusions of this article will be made available by the authors, without undue reservation.
The animal study was reviewed and approved by the Tongji University Ethical Institutional Committee of Animal Care and Use.
QL and HL conceived and designed the experiment; LP, BL, SL, and CD performed the experiments and recorded data, and they were responsible for data acquisition; JZ performed the data analysis and statistical analysis; LP and BL wrote the paper and contributed equally to this work, they were co-first authors; JZ, NX and ZY contributed to the theoretical analysis. QL and HL revised the manuscript critically for important intellectual content, they were co-corresponding authors. All authors contributed to the general discussion and gave approval to the final manuscript.
This study was funded by the National Natural Science Foundation of China (Grant No.: 81470861 to Qinghua Li) and supported bythe Top-Level Clinical Discipline Project of Shanghai Pudong (PWYgf2018-04 to Meidong Xu).
The authors declare that the research was conducted in the absence of any commercial or financial relationships that could be construed as a potential conflict of interest.
The Supplementary Material for this article can be found online at: https://www.frontiersin.org/articles/10.3389/fmats.2021.682844/full#supplementary-material
ANOVA, analysis of variance; aHSCs, activated hepatic stellate cells; CCK-8, cell counting kit-8; DMEM, Dulbecco’s Modified Eagle’s Medium; DR4, death receptor 4; DR4+, DR4-positive; DR5, death receptor 5; ECM, extracellular matrix; FBS, fetal bovine serum; GAPDH, glyceraldehyde-3-phosphate dehydrogenase; IF, immunofluorescence; NK, natural killer; PAI-1, plasminogen activator inhibitor type-1; PBS, phosphate buffer saline; PEG, polyethylene glycol; SD, standard deviations; TNF, tumor necrosis factor; TRAIL, tumor necrosis factor–related apoptosis-inducing ligand; α-SMA, α-smooth muscle actin; TUNEL, terminal deoxynucleotidyl transferase dUTP [2'-deoxyuridine 5'-triphosphate] nick end labeling; tBid, truncated BH3 interacting domain death agonist; uPA, urokinase plasminogen activator
AlQahtani, A. D., O’Connor, D., Domling, A., and Goda, S. K. (2019). Strategies for the Production of Long-Acting Therapeutics and Efficient Drug Delivery for Cancer Treatment. Biomed. Pharmacother. 113, 108750. doi:10.1016/j.biopha.2019.108750
Arabpour, M. (2016). Addressing Liver Fibrosis by TRAIL Targetied to Hepatic Stellate Cells. Groningen: University of Groningen.
Bansal, R., Prakash, J., Post, E., Beljaars, L., Schuppan, D., and Poelstra, K. (2011). Novel Engineered Targeted Interferon-Gamma Blocks Hepatic Fibrogenesis in Mice. Hepatol. 54 (2), 586–596. doi:10.1002/hep.24395
Beaussier, Wendum, D., Schiffer, E., Dumont, S., Rey, C., Lienhart, A., Bataller, R., et al. (2005). Liver Fibrosis. J. Clin. Invest. 114 (2), 209–218. doi:10.1172/JCI24282
Beljaars, L., Molema, G., Weert, B., Bonnema, H., Olinga, P., Groothuis, G. M., et al. (1999). Albumin Modified with Mannose 6-phosphate: A Potential Carrier for Selective Delivery of Antifibrotic Drugs to Rat and Human Hepatic Stellate Cells. Hepatol. 29 (5), 1486–1493. doi:10.1002/hep.510290526
Beltrán-Gracia, E., López-Camacho, A., Higuera-Ciapara, I., Velázquez-Fernández, J. B., and Vallejo-Cardona, A. A. (2019). Nanomedicine Review: Clinical Developments in Liposomal Applications. Cancer Nanotechnol. 10 (1), 11. doi:10.1186/s12645-019-0055-y
Bychkov, M. L., Gasparian, M. E., Dolgikh, D. A., and Kirpichnikov, M. P. (2014). Combination of TRAIL with Bortezomib Shifted Apoptotic Signaling from DR4 to DR5 Death Receptor by Selective Internalization and Degradation of DR4. PLoS One 9 (10), e109756. doi:10.1371/journal.pone.0109756
Chae, S. Y., Kim, T. H., Park, K., Jin, C.-H., Son, S., Lee, S., et al. (2010). Improved Antitumor Activity and Tumor Targeting of NH(2)-Terminal-Specific PEGylated Tumor Necrosis Factor-Related Apoptosis-Inducing Ligand. Mol. Cancer Ther. 9 (6), 1719–1729. doi:10.1158/1535-7163.mct-09-1076
Chu, C., Lyu, X., Wang, Z., Jin, H., Lu, S., Xing, D., et al. (2020). Cocktail Polyprodrug Nanoparticles Concurrently Release Cisplatin and Peroxynitrite-Generating Nitric Oxide in Cisplatin-Resistant Cancers. Chem. Eng. J. 402, 126125. doi:10.1016/j.cej.2020.126125
Di Gregorio, J., Sferra, R., Speca, S., Vetuschi, A., Dubuquoy, C., Desreumaux, P., et al. (2017). Role of Glycogen Synthase Kinase-3β and PPAR-γ on Epithelial-To-Mesenchymal Transition in DSS-Induced Colorectal Fibrosis. PLoS One 12 (2), e0171093. doi:10.1371/journal.pone.0171093
Friedman, S. L. (2003). Liver Fibrosis - from Bench to Bedside. J. Hepatol. 38 (1), 38–53. doi:10.1016/s0168-8278(02)00429-4
Glässner, A., Eisenhardt, M., Krämer, B., Körner, C., Coenen, M., Sauerbruch, T., et al. (2012). NK Cells from HCV-Infected Patients Effectively Induce Apoptosis of Activated Primary Human Hepatic Stellate Cells in a TRAIL-, FasL- and NKG2D-dependent Manner. Lab. Invest. 92 (7), 967–977. doi:10.1038/labinvest.2012.54
Harris, J. M., and Chess, R. B. (2003). Effect of Pegylation on Pharmaceuticals. Nat. Rev. Drug Discov. 2 (3), 214–221. doi:10.1038/nrd1033
Harris, J. M., Martin, N. E., and Modi, M. (2001). Pegylation. Clin. Pharmacokinet. 40 (7), 539–551. doi:10.2165/00003088-200140070-00005
Hasenfuss, S. C., Bakiri, L., Thomsen, M. K., Hamacher, R., and Wagner, E. F. (2014). Activator Protein 1 Transcription Factor Fos-Related Antigen 1 (Fra-1) is Dispensable for Murine Liver Fibrosis, But Modulates Xenobiotic Metabolism. Hepatol. 59 (1), 261–273. doi:10.1002/hep.26518
Hellwig, C. T., and Rehm, M. (2012). TRAIL Signaling and Synergy Mechanisms Used in TRAIL-Based Combination Therapies. Mol. Cancer Ther. 11 (1), 3–13. doi:10.1158/1535-7163.mct-11-0434
Higashi, T., Friedman, S. L., and Hoshida, Y. (2017). Hepatic Stellate Cells as Key Target in Liver Fibrosis. Adv. Drug Deliv. Rev. 121, 27–42. doi:10.1016/j.addr.2017.05.007
Holland, P. M. (2014). Death Receptor Agonist Therapies for Cancer, Which Is the Right TRAIL? Cytokine Growth Factor. Rev. 25 (2), 185–193. doi:10.1016/j.cytogfr.2013.12.009
Holoch, P. A., and Griffith, T. S. (2009). TNF-related Apoptosis-Inducing Ligand (TRAIL): A New Path to Anti-cancer Therapies. Eur. J. Pharmacol. 625 (1-3), 63–72. doi:10.1016/j.ejphar.2009.06.066
Hu, X., Liu, G., Li, Y., Wang, X., and Liu, S. (2015). Cell-Penetrating Hyperbranched Polyprodrug Amphiphiles for Synergistic Reductive Milieu-Triggered Drug Release and Enhanced Magnetic Resonance Signals. J. Am. Chem. Soc. 137 (1), 362–368. doi:10.1021/ja5105848
Iimuro, Y., Nishio, T., Morimoto, T., Nitta, T., Stefanovic, B., Choi, S. K., et al. (2003). Delivery of Matrix Metalloproteinase-1 Attenuates Established Liver Fibrosis in the Rat. Gastroenterol. 124 (2), 445–458. doi:10.1053/gast.2003.50063
Inagaki, Y., Nemoto, T., Kushida, M., Sheng, Y., Higashi, K., Ikeda, K., et al. (2003). Interferon Alfa Down-Regulates Collagen Gene Transcription and Suppresses Experimental Hepatic Fibrosis in Mice. Hepatol. 38 (4), 890–899. doi:10.1002/hep.1840380415
Jean-Charles, S., Zsuzsanna, M., Petr, Z., Barna, S., István, A., Erzsébet, J., et al. (2011). Randomized Phase II Study of Dulanermin in Combination with Paclitaxel, Carboplatin, and Bevacizumab in Advanced Non-small-cell Lung Cancer. J. Clin. Oncol. Official J. Am. Soc. Clin. Oncol. 29 (33), 4442
Kelley, S. K., Harris, L. A., Xie, D., Deforge, L., Totpal, K., Bussiere, J., et al. (2001). Preclinical Studies to Predict the Disposition of Apo2L/Tumor Necrosis Factor-Related Apoptosis-Inducing Ligand in Humans: Characterization of In Vivo Efficacy, Pharmacokinetics, and Safety. J. Pharmacol. Exp. Ther. 299 (1), 31–38.
Kim, T. H., Jiang, H. H., Park, C. W., Youn, Y. S., Lee, S., Chen, X., et al. (2011a). PEGylated TNF-Related Apoptosis-Inducing Ligand (TRAIL)-loaded Sustained Release PLGA Microspheres for Enhanced Stability and Antitumor Activity. J. Control Release 150 (1), 63–69. doi:10.1016/j.jconrel.2010.10.037
Kim, T. H., Jiang, H.-H., Youn, Y. S., Park, C. W., Lim, S. M., Jin, C.-H., et al. (2011b). Preparation and Characterization of Apo2L/TNF-Related Apoptosis-Inducing Ligand-Loaded Human Serum Albumin Nanoparticles with Improved Stability and Tumor Distribution. J. Pharm. Sci. 100 (2), 482–491. doi:10.1002/jps.22298
Kim, T. H., Youn, Y. S., Jiang, H. H., Lee, S., Chen, X., and Lee, K. C. (2011c). PEGylated TNF-Related Apoptosis-Inducing Ligand (TRAIL) Analogues: Pharmacokinetics and Antitumor Effects. Bioconjug. Chem. 22 (8), 1631–1637. doi:10.1021/bc200187k
Lemke, J., von Karstedt, S., Zinngrebe, J., and Walczak, H. (2014). Getting TRAIL Back on Track for Cancer Therapy. Cell Death Differ. 21 (9), 1350–1364. doi:10.1038/cdd.2014.81
Li, F., Li, Q.-h., Wang, J.-y., Zhan, C.-y., Xie, C., and Lu, W.-y. (2012). Effects of Interferon-Gamma Liposomes Targeted to Platelet-Derived Growth Factor Receptor-Beta on Hepatic Fibrosis in Rats. J. Control Release 159 (2), 261–270. doi:10.1016/j.jconrel.2011.12.023
Li, Q., Yu, Q., Ju, J., You, T., Yan, Z., Nan, X., et al. (2017). Long-Circulating Liposomal Delivery System Targeting at PDGFR-β Enhances the Therapeutic Effect of IFN-α on Hepatic Fibrosis. Curr. Pharm. Des. 23 (20), 3034–3046. doi:10.2174/1381612822666161208144953
Li, X., Su, Y., Hua, X., Xie, C., Liu, J., Huang, Y., et al. (2017). Levels of Hepatic Th17 Cells and Regulatory T Cells Upregulated by Hepatic Stellate Cells in Advanced HBV-Related Liver Fibrosis. J. Transl. Med. 15 (1), 75. doi:10.1186/s12967-017-1167-y
Lim, S. M., Kim, T. H., Jiang, H. H., Park, C. W., Lee, S., Chen, X., et al. (2011). Improved Biological Half-Life and Anti-tumor Activity of TNF-Related Apoptosis-Inducing Ligand (TRAIL) Using PEG-Exposed Nanoparticles. Biomater. 32 (13), 3538–3546. doi:10.1016/j.biomaterials.2011.01.054
Macfarlane, M., Kohlhaas, S. L., Sutcliffe, M. J., Dyer, M. J. S., and Cohen, G. M. (2005). TRAIL Receptor-Selective Mutants Signal to Apoptosis via TRAIL-R1 in Primary Lymphoid Malignancies. Cancer Res. 65 (24), 11265–11270. doi:10.1158/0008-5472.can-05-2801
Mahmud, A., Mcnulty, M., Mcgovern, E., Young, V., Tolan, M., and Feely, J. (2007). 10.01 Arterial Stiffness Is Associated with Elastin Defragmentation and Medial Collagen Content in the Human Aorta. Artery Res. 1 (2), 51–52. doi:10.1016/j.artres.2007.07.054
Marconi, M., Ascione, B., Ciarlo, L., Vona, R., Garofalo, T., Sorice, M., et al. (2013). Constitutive Localization of DR4 in Lipid Rafts Is Mandatory for TRAIL-Induced Apoptosis in B-Cell Hematologic Malignancies. Cell Death Dis. 4 (10), e863. doi:10.1038/cddis.2013.389
Marcuzzi, A., Secchiero, P., Crovella, S., and Zauli, G. (2012). TRAIL Administration Down-Modulated the Acute Systemic Inflammatory Response Induced in a Mouse Model by Muramyldipeptide or Lipopolysaccharide. Cytokine. 60 (1), 43–46. doi:10.1016/j.cyto.2012.06.001
Martinez-Lostao, L., Garcí´a-Alvarez, F. c., Basáñez, G., Alegre-Aguarón, E., Desportes, P., Larrad, L., et al. (2010). Liposome-bound APO2L/TRAIL Is an Effective Treatment in a Rabbit Model of Rheumatoid Arthritis. Arthritis Rheum. 62 (8), 2272–2282. doi:10.1002/art.27501
Milosavljevic, N., Gazdic, M., Simovic Markovic, B., Arsenijevic, A., Nurkovic, J., Dolicanin, Z., et al. (2018). Mesenchymal Stem Cells Attenuate Liver Fibrosis by Suppressing Th17 Cells - an Experimental Study. Transpl. Int. 31 (1), 102–115. doi:10.1111/tri.13023
Oh, Y., Park, O., Swierczewska, M., Hamilton, J. P., Park, J.-S., Kim, T. H., et al. (2016). Systemic PEGylated TRAIL Treatment Ameliorates Liver Cirrhosis in Rats by Eliminating Activated Hepatic Stellate Cells. Hepatol. 64 (1), 209–223. doi:10.1002/hep.28432
Park, J.-S., Oh, Y., Park, O., Foss, C. A., Lim, S. M., Jo, D.-G., et al. (2017). PEGylated TRAIL Ameliorates Experimental Inflammatory Arthritis by Regulation of Th17 Cells and Regulatory T Cells. J. Control Release 267, 163–171. doi:10.1016/j.jconrel.2017.10.004
Poynard, T., Mchutchison, J., Davis, G. L., Esteban-Mur, R., Goodman, Z., Bedossa, P., et al. (2000). Impact of Interferon Alfa-2b and Ribavirin on Progression of Liver Fibrosis in Patients with Chronic Hepatitis C. Hepatol. 32 (5), 1131–1137. doi:10.1053/jhep.2000.19347
Sato, M., Suzuki, S., and Senoo, H. (2003). Hepatic Stellate Cells: Unique Characteristics in Cell Biology and Phenotype. Cell Struct. Funct. 28 (2), 105–112. doi:10.1247/csf.28.105
Sato, Y., Murase, K., Kato, J., Kobune, M., Sato, T., Kawano, Y., et al. (2008). Resolution of Liver Cirrhosis Using Vitamin A-Coupled Liposomes to Deliver siRNA against a Collagen-specific Chaperone. Nat. Biotechnol. 26 (4), 431–442. doi:10.1038/nbt1396
Shen, Z., Fisher, A., Liu, W. K., and Li, Y. (2018). “PEGylated “Stealth” Nanoparticles and Liposomes,” in Engineering of Biomaterials for Drug Delivery Systems: Beyond Polyethylene Glycol (Cambridge: Elsevier), 1–26. doi:10.1016/b978-0-08-101750-0.00001-5
Shiraishi, K., and Yokoyama, M. (2019). Toxicity and Immunogenicity Concerns Related to PEGylated-Micelle Carrier Systems: a Review. Sci. Technol. Adv. Mater. 20 (1), 324–336. doi:10.1080/14686996.2019.1590126
Swierczewska, M., Lee, K. C., and Lee, S. (2015). What Is the Future of PEGylated Therapies? Expert Opin. Emerg Drugs. 20 (4), 531–536. doi:10.1517/14728214.2015.1113254
Tacke, F., and Zimmermann, H. W. (2014). Macrophage Heterogeneity in Liver Injury and Fibrosis. J. Hepatol. 60 (5), 1090–1096. doi:10.1016/j.jhep.2013.12.025
Veronese, F. M., and Pasut, G. (2005). PEGylation, Successful Approach to Drug Delivery. Drug Discov. Today 10 (21), 1451–1458. doi:10.1016/s1359-6446(05)03575-0
Wang, W., Liang, G., Zhang, W., Xing, D., and Hu, X. (2018). Cascade-promoted Photo-Chemotherapy against Resistant Cancers by Enzyme-Responsive Polyprodrug Nanoplatforms. Chem. Mater. 30 (10), 3486–3498. doi:10.1021/acs.chemmater.8b01149
Wang, Z., Zhan, M., Li, W., Chu, C., Xing, D., Lu, S., et al. (2021). Photoacoustic Cavitation‐Ignited Reactive Oxygen Species to Amplify Peroxynitrite Burst by Photosensitization‐Free Polymeric Nanocapsules. Angew. Chem. Int. Ed. 60 (9), 4720–4731. doi:10.1002/anie.202013301
Webster, R., Elliott, V., Park, B. K., Walker, D., Hankin, M., and Taupin, P. (2009). “PEG and PEG Conjugates Toxicity: towards an Understanding of the Toxicity of PEG and its Relevance to PEGylated Biologicals,” in PEGylated Protein Drugs: Basic Science and Clinical Applications (Basel: Springer), 127–146. doi:10.1007/978-3-7643-8679-5_8
Xiang, H., Nguyen, C. B., Kelley, S. K., Dybdal, N., and Escandón, E. (2004). Tissue Distribution, Stability, and Pharmacokinetics of Apo2 Ligand/tumor Necrosis Factor-Related Apoptosis-Inducing Ligand in Human colon Carcinoma COLO205 Tumor-Bearing Nude Mice. Drug Metab. Dispos. 32 (11), 1230–1238. doi:10.1124/dmd.104.000323
Xu, F., Zhou, D., Meng, X., Wang, X., Liu, C., Huang, C., et al. (2016). Smad2 Increases the Apoptosis of Activated Human Hepatic Stellate Cells Induced by TRAIL. Int. Immunopharmacol. 32, 76–86. doi:10.1016/j.intimp.2016.01.013
Yamaoka, T., Tabata, Y., and Ikada, Y. (1994). Distribution and Tissue Uptake of Poly(ethylene Glycol) with Different Molecular Weights after Intravenous Administration to Mice. J. Pharm. Sci. 83 (4), 601–606. doi:10.1002/jps.2600830432
Yoon, J.-H., and Gores, G. J. (2002). Death Receptor-Mediated Apoptosis and the Liver. J. Hepatol. 37 (3), 400–410. doi:10.1016/s0168-8278(02)00209-x
Keywords: PEG, TRAIL, long circulation, hepatic fibrosis, hepatic stellate cells (HSCs), LX-2
Citation: Lu B, Peng L, Luo S, Zhou J, Xu N, Dong C, Yan Z, Li H and Li Q (2021) Long Circulation of PEG-TRAIL Improves Anti-Hepatic Fibrosis Effect of TRAIL Via Targeting Activated Hepatic Stellate Cells. Front. Mater. 8:682844. doi: 10.3389/fmats.2021.682844
Received: 19 March 2021; Accepted: 11 May 2021;
Published: 17 June 2021.
Edited by:
Larry D Unsworth, University of Alberta, CanadaReviewed by:
Yong Wang, Nanjing Medical University, ChinaCopyright © 2021 Lu, Peng, Luo, Zhou, Xu, Dong, Yan, Li and Li. This is an open-access article distributed under the terms of the Creative Commons Attribution License (CC BY). The use, distribution or reproduction in other forums is permitted, provided the original author(s) and the copyright owner(s) are credited and that the original publication in this journal is cited, in accordance with accepted academic practice. No use, distribution or reproduction is permitted which does not comply with these terms.
*Correspondence: Qinghua Li, TFFIYXJ0aWNsZUBhbGl5dW4uY29t; Huiyi Li, bGh5MTc3Mjc5QDE2My5jb20=
†These authors have contributed equally to this work
Disclaimer: All claims expressed in this article are solely those of the authors and do not necessarily represent those of their affiliated organizations, or those of the publisher, the editors and the reviewers. Any product that may be evaluated in this article or claim that may be made by its manufacturer is not guaranteed or endorsed by the publisher.
Research integrity at Frontiers
Learn more about the work of our research integrity team to safeguard the quality of each article we publish.