- Wits Advanced Drug Delivery Platform Research Unit, Department of Pharmacy and Pharmacology, School of Therapeutic Sciences, Faculty of Health Sciences, University of the Witwatersrand, Johannesburg, South Africa
Wound healing is a complicated yet necessary event that takes place in both animals and human beings for the body to repair itself due to injury. Wound healing involves various stages that ensure the restoration of the injured tissue at the end of the process. Wound dressing material acts as a protective extracellular barrier against potential damage to the injury and microbial invasion. Naturally, polysaccharides (chitosan and alginate) have inherent properties that have made them attractive for their usage in wound healing therapy. Alginate and chitosan have been used to develop novel wound healing and smart biomaterials due to various functionalities such as reducing swelling, non-toxic nature, biocompatibility, antimicrobial potential, and maintenance moist environment, ability to absorb wound fluid, and skin regeneration promotion. Functionalization of polysaccharides is one of the many approaches that have been used to modify and enhance the wound healing properties of these biomaterials. Many studies have been done to modify the polysaccharide hydrogels. Some of these are highlighted in this paper. The designing and development of smart hydrogels that react to their environment have recently sparked a significant scientific and pharmaceutical interest. Smart hydrogel development has been the primary focal area for developing highly advanced and sophisticated wound healing therapeutic technologies. This paper seeks to comprehensively shed light on the advancements of functionalized chitosan and alginate-based hydrogels and their applicability in wound healing therapeutics. In addition to this, thus identifying critical drawbacks faced in existing hydrogel systems and how prospective technologies enable digitally controlled bio-platforms coupled with biomaterials to improve wound care. This review hopes to stimulate and encourage researchers to identify future avenues worth investigation.
Introduction
Today wound management remains a serious problem regardless of the recent advancements made regarding wound healing materials development. Wounds are classified based on their depth, appearance, location, etiology, and the nature of the injury (Baranoski and Ayello, 2008). In addition to this, wounds can be further categorized as partial, full, and superficial thickness (Boateng et al., 2008). Worldwide, a wide variety of wounds are generated annually for various reasons such as surgical procedures, non-healing ulcers, gunshots car accidents and burns (Aderibigbe and Buyana, 2018). The cost to manage wounds increases as the population size increases. The urban lifestyle is an indicator for the greater need to develop novel hydrogel-based wound dressings materials that are relevant, effective, and affordable (Cullum et al., 2016). Wound dressings are produced from various biopolymers and synthetic polymers. Alginate has been extensively researched to develop pharmaceutically relevant biomaterials. Alginate is toxic-free, antimicrobial, abundant in nature, offers excellent biodegradability, hydrophilic, biocompatible, and does not elicit immune responses (Noh et al., 2006). Wound dressings developed as hydrogels have become very important for successful wound healing and wound management over the years, more especially when modified chemically, physically or combined with technological devices. Different polymers have been used to produce wound dressings which are available in retail markets (Wild et al., 2009). Hydrogels are defined as water-soluble cross-linked polymeric networks developed by either physical or chemical cross-linking of soluble polymers.
Their unique properties, such as hydrophilic nature, soft tissue-like water content, high-sensitivity to physiological environments, and adequate flexibility, have made them the preferred and excellent choice for wound healing-related therapeutics. Polysaccharide-based hydrogels can swell in water, showing specific stimuli-responsiveness to their environment due to changes in environmental conditions such as temperature, light, and pH. Thus, hydrogels’ intelligence to respond to changes in physiological variables change suggests their meaningful use in several biomedical applications, one of them being wound healing (Sood et al., 2014). Therefore, the importance of this manuscript is to review the recent work done by researchers in the field of wound healing and to look at the main achievements as result of functionalising polysaccharides (alginate and chitosan).
The application of alginate and chitosan for wound healing is due to these polymers having favourable and distinctive attributes. These include having a high absorption capacity, biocompatible, non-toxic, non-immunogenicity, and low cost (Sudarsan et al., 2015). However, these natural polymers can usually be limited by their unfavourable mechanical attributes. As a result, they are combined with other synthetic platforms or are derivatized to enhance their mechanical characteristics (Noh et al., 2006).
This manuscript seeks shed light on the progress made regarding wound healing therapy using functionalised alginate/chitosan as well as the drawbacks which still exist in the development of sophisticated and smart wound healing systems. The importance of developing a wound dressing material which has ideal wound healing properties that create an environment that allows the wound to heal quickly under pathological conditions while achieving an acceptable skin appearance at an affordable price (Sweeney et al., 2012) is also covered to assist researchers in understanding the importance of designing or developing wound healing systems based on the factors that promote successful wound healing therapy and improve patient compliance. A major development in wound healing therapy is the emergence of smart hydrogels which have been developed by combining technological platforms or devices such as chips, wearable bands, and sensors with polymers. These have shown significant advancement in the control of drug release, swelling, or absorption in response to pH, temperature, oxygen levels, or light as developed by Mostafalu et al. [95]. Microneedles are also another type of platform advancing over the years. The development of the first solid microneedle (MNs) was developed in 1971 [97]. More promising technologies have been produced since 2012, resulting in the first hydrogel-based microchip microneedles, which were developed by Donnelly et al. [102]. Therefore, incorporating bioactive components in wound dressings has been found to improve these novel systems’ overall effectiveness.
Therefore, novel and technologically advanced polysaccharide-based-hydrogel dressings may encourage more efficient wound healing over a short period, provide pain relief and reduce scarring, thus improving overall patient compliance. Polysaccharides have diverse structures, which make them differ in their chemical composition and attributes and this should always be taken into consideration when designing and producing modified biomaterials. Different design strategies by functionalization have been highlighted in this manuscript to provide information on the properties and benefits of functionalising different molecules such as peptides (RDG), acyl containing compounds, amine containing compounds and methyl containing compounds These properties provide a range of structural properties and chemical attributes that can be manipulated for the synthesis and combined with various technological systems for the development of novel wound dressing hydrogels specific to the wound etiology, thus providing endless avenues for investigation. This review aims to provide recent information and data regarding the advancement and progress of modified chitosan and alginate wound healing systems and the benefits of combining such systems with technological devices to produce smart and intelligent wound healing products.
Wound Healing Process
Several growth factors like cytokines, chemokines, and other biomolecules facilitate the wound healing process (Thomas, 1990). Wound healing occurs in 4 phases, as seen in Figure 1. The first stage is the exudative phase, 2. Inflammatory phase, 3. Proliferative phase and 4. Regenerative phase (Yadav et al., 2015). Several growth factors are required for wound healing to take place. These mainly include chemokines and cytokines (Coger et al., 2019; Gupta et al., 2019; Stern and Cui, 2019).
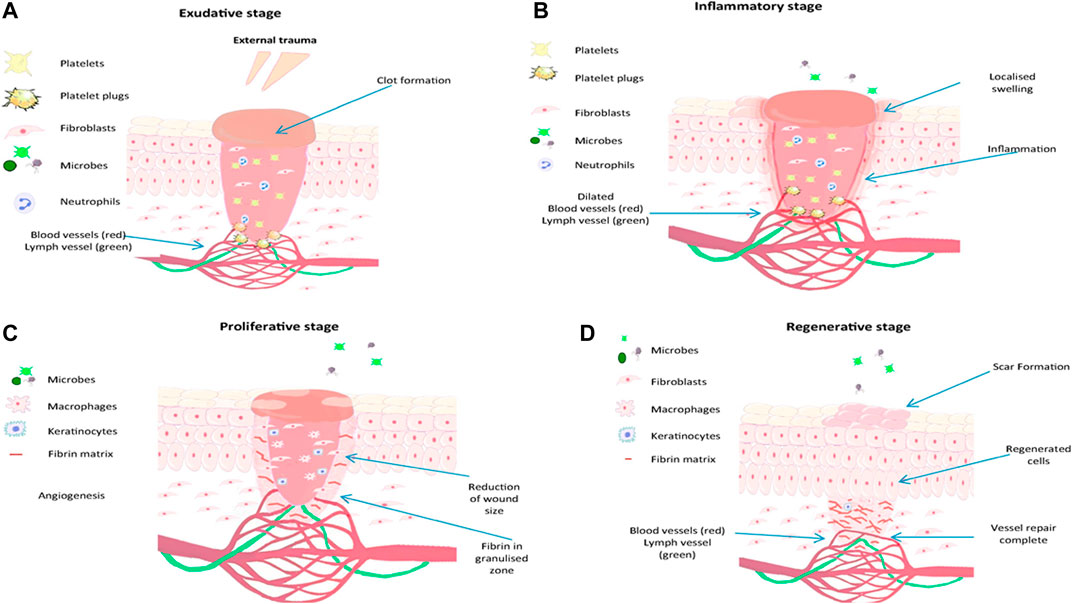
FIGURE 1. Representation of steps involved in wound the healing process, reproduced with permission from Gupta et al. (2019), (A) exudative stage with the formation of blood clot, (B) inflammatory stage, marked with oedema, pain and inflammation, (C) proliferation stage with granulation tissue formation and (D) regenerative stage, characterised by scar tissue formation.
Exudative Stage
The exudative stage also referred to as coagulation and hemostasis, involves preventing blood loss. It is the act of stopping excessive bleeding by hemostatic reflex vasoconstriction and forming an insoluble plug fibrin, and excessive blood loss is prevented. Blood flows and interacts with collagen exposed together with other extracellular matrix components. This phase is followed by platelet activation to release clotting factors before their aggregation forms a plug mash network, thus regulating and preventing blood loss (Ding et al., 2018).
Inflammation Stage
For wound healing to continue effectively, neutrophils must be cleared from the wound through phagocytosis by macrophages, followed by removing the wound utilizing shredding, autolytic debridement, and apoptosis. Mononucleated macrophages make their way to the wound site about 48–72 h post-injury and become changed into reparative macrophages (Yang et al., 2017) that enable and mediate inflammation together with the removal of dead cells by phagocytosis (Vishwakarma et al., 2014; Kuivaniemi and Tromp, 2019).
Proliferation Stage
Upon successful autolytic debridement and the immune response has concluded. The wound healing process then continues to the proliferation stage; at this stage, tissue formation occurs due to the tissue’s repairing and closure of the being started. The area which is damaged is then covered by granulation tissue; this is then followed by the damaged tissue’s epithelialization occurring. The epithelial cells around the wound parameters grow by mitosis and move resulting in a long and wide layer of cells forms. The protein known as collagen is produced by fibroblasts, mimics a temporary matrix, and supports the newly developed granulation tissue, which is crucial in determining scars’ appearance (Wild et al., 2009; Vishwakarma et al., 2014). To distribute nutrients and oxygen to freshly deposited tissue, it is at this phase when angiogenesis can occur. Therefore, allowing a network of microvascular blood capillaries to be formed from existing normal blood vessels (Coger et al., 2019; Kuivaniemi and Tromp, 2019).
Regeneration Stage
The final phase of wound healing is the regeneration phase. During this final step, the typical dermal structure is reproduced, and the mechanical strength of the tissue is improved. All cells responsible for inflammation are cleared from the fixed area while remodeling collagen occurs to improve the tissue’s tensile strength (Simões et al., 2018).
Types of Wound Healing Materials
Materials used as wound healing material can be categorized into traditional, artificial, biomaterial-based, and bioactive dressings (Yu et al., 2018). Passive wound dressings protect wounds from the external environment and stop bleeding (Harding et al., 2000). Classic wound dressing examples include gauze-cotton and gauze composites that typically can absorb high amounts of wound fluid. However, classic dressings can cause the wound to bleed, display low vapor transmission properties, and destroy recently formed tissue (epithelium) when removed. Exudates leaking from such dressings can lead to bacterial invasion (Moura et al., 2013). Wound dressings that are biomaterial-based are further characterized as allografts, tissue derivatives, or xenografts. Skin fragments, also known as allografts, are available as either freeze-dried or newly received tissue from donors. Their usage is often restricted by immunological responses leading to the rejection recipient’s body. In addition to this, the transmission of diseases and infection risk is great (Line et al., 1995). Allografts costs are high and have a low shelf life (Greenwood and Dearman, 2012). Artificial dressings are categorized as composites, films, gels, films, foams, and sprays (Morgan, 2002), [110]. These are synthesized from natural and synthetic polymers. Commonly used biopolymers include alginate, chitosan, cellulose, and xanthan due to their intrinsic and beneficial properties. Such wound dressings are further grouped and characterized as bioactive wound dressing. Alginates, chitosan, xanthan, collagens, hydrofibres, and hydrocolloids are examples of bioactive wound dressings. An improved wound healing process can be achieved by adding growth factors, nanoparticles, functional groups, and antimicrobials (Parenteau et al., 1996). Wound dressing classifications are summarised in Table 1.
Application of Alginate and Chiotsan as Wound Dressings
Sheet Hydrogel Dressings and Amorphous Hydrogels
Hydrogel dressings can exist in two main forms, amorphous dressing, and sheet dressing (Qu et al., 2018). Amorphous hydrogel dressings exhibit low exudate absorptive capabilities because of their high-water content. However, they provide a moist environment for dry wounds; functionalizing these hydrogels improves the absorbent capabilities, swelling profile, moisture retention ability, stretchability, and hydrogels’ mechanical strength (Bradford et al., 2009). Hydrogel dressings classified as sheet dressings are mainly applied during the final phases during the recovery of wounds. Such steps include the epithelial phase and phlebitis. Clear and transparent sheet hydrogels can also be used to monitor wound healing progress in real-time, as seen in Figure 2. Hydrogel dressings are classified as amorphous hydrogels’ primary use is to support autolysis debridement and scab softening. Such amorphous biomaterial is frequently used by doctors and nurses in clinics (Figure 3) (Liang et al., 2019).
Development Approaches of Novel Chitosan and Alginate-Based Hydrogels
The treatment of chronic wounds and acute wounds is a serious challenge. Wound dressings derived from natural polymers offer numerous advantages in comparison to traditional wound dressings (Almeida et al., 2011). Alginate and chitosan-based wound dressings can be made to take up several forms, such as sponges, foams, nanofibers, films, and hydrogels (Huang and Fu, 2010; Mndlovu et al., 2019). Alginate application as a wound-healing material is because of its inherent features (non-toxicity, biocompatible nature, non-immunogenic, cheap to buy, and high absorption profile) (Huang and Fu, 2010). However, natural polymers are usually limited by their unfavorable mechanical attributes. The combination of natural and synthetic polymers is done to enhance their physical properties, degradation patterns, and overall antimicrobial properties (Almeida et al., 2011).
Alginate Based Formulations
Thiol Functionalized Alginate Hydrogels
Alginate, derived from algae, has widely been researched for its suitability as a wound care therapeutic product, and the delivery of drugs to wounds because of its non-toxic and biocompatible attributes (Mansoor et al., 2019) by derivatization led to several favourable properties being imparted onto Alginate. Meidong et al. (Lee and Mooney, 2012) investigated an in situ formed thiolated alginate hydrogel, which was synthesized using disulfide bonds being formed due to oxygen, a water-containing solution with available thiol groups. Therefore, to illustrate the hydrogel’s cytocompatibility properties, human hepatocytes were fabricated in the hydrogel, resulting in 85% cell viability after five days. This meant that the interaction of the thiol groups with hepatocytes cellular environment resulted in the reduction of Reactive Oxygen Species (ROS) resulting in improved cellular viability. In terms of the potential of the functionalized hydrogel to lower the homeostatic time, animal-based experiments were carried out using a rat’s tail. The duration of hemostasis decreased from 8.26 to 3.24 min in comparison with a calcium alginate hydrogel The reason for this is because disulfide bonds have been found to play a role as redox switches. Disulfide bonds are present in the β subunit of the platelet integrin αIIbβ3 and in other integrins involved in thrombus formation. Redox agents such as gluthione, nitric oxide and enzymatic thiol isomerase are responsible for the regulation of the integrins because of thiol or disulfide exchange or disulfide bond reduction thus controlling the rate of thrombus formation. Therefore, thiolated platform could absorb a significant amount of blood compared to calcium alginate hydrogel (Xu et al., 2016). Yin and co-worker’s (Li et al., 2017) investigated another cross-linked disulfide hydrogel of thiolated alginate as a biomaterial for tissue engineering (Lynch et al., 2020). The platform was stable for 14 days in an aqueous solution, and stability was influenced by the degree of thiolation and the degree of cross-linking. This hydrogel further showed homeostatic potential and significant biocompatibility. This was made possible due to the abundant free thiol groups as these groups are easily oxidized when they are in aqueous solutions. The degree of crosslinking on the other hand further provides reinforcement by improving the mechanical stability to the biomaterial. Hydrogel-based wound dressings have been intensively studied as promising materials for wound healing and care. Popescu et al. (Lynch et al., 2020) reported the mixed-mode thiol-acrylate photopolymerization is used in their work for alginate/poloxamer hydrogels formation. First, the alginate was modified with thiol groups using the esterification reaction with cysteamine, and second, the terminal hydroxyl groups of poloxamer were esterified with acryloyl chloride to introduce polymerizable acrylate groups. Finally, the cross-linking reaction between the two macromers was performed to produce degradable alginate/poloxamer hydrogels. The biological assays show that the alginate/poloxamer hydrogels induce proliferation of human keratinocyte and have an anti-inflammatory effect on lipopolysaccharides (LPS)-activated keratinocytes by inhibiting the extracellular signal-regulated kinases (ERK)/nuclear factor (NF)-kB/tumor necrosis factor (TNF)-α signalling pathway. Their results showed that the chemical cross-linked alginate/poloxamer hydrogels may function as a dressing/patch applied directly on the skin lesion to heal the wound by reducing the exacerbated inflammation which causes wounds not to heal or increases their healing time.
Alginate Functionalization With Furan Compounds
Since alginate can be modified by both its carboxyl groups and hydroxyl present in its backbone structure, it enables several interesting features to be achieved which will enhance the wound healing capabilities of the polysaccharide. Oxidation or light-curable cross-linking strategies have been used to prepare hydrogels in drug delivery. Li et al. (2017) synthesized chemically cross-linked alginate hydrogels by reacting alginate modified using furfuryl amine and stimuli-responsive crosslinkers. The ensuing hydrogels’ investigation toward pH stimuli revealed an apparent pH-dependent swelling. The system can be designed to absorb wound fluid and release drugs at low pH. It is at a pH of 5 whereby fibroblasts are activated and proliferate the most in contrast to neutral or alkali pH. This design strategy would result in a faster wound healing process and ensure that the wound is kept moist and does not contain excess wound fluid as opposed to ionic cross-linked alginate hydrogels. The synthesized cross-linked alginate hydrogels had stable polymeric networks, and their properties can be adjusted according to the cross-linking degree. However, this can be limiting as certain properties are not imparted onto the pristine polymer when crosslinking is done. The introduction of functional groups to the pristine biopolymer will result new physical, chemical and biomedical properties being obtained which are beneficial to the success and efficiency of the wound healing process. Heo and coworkers (Heo et al., 2020) developed a light curable Furfuryl alginate hydrogel responsive to light stimulus. The release studies demonstrated that the prepared furan alginate hydrogels showed different gelation, swelling, and degradation abilities in response to light exposure. Therefore, they appeared to be good candidates for a dermal drug delivery system (Heo et al., 2020). In addition to this the introduction of bioactive drugs and nanocarriers into the furan alginate-based biomaterials could accelerate and enhance the wound healing process due to the adjustable release, gelling, and degradation properties of bioinspired smart hydrogels. This type of hydrogel could be designed in the form of microneedles perhaps this would improve the mechanical strength of the biomaterial, release kinetics of the system, drug bioavailability, interaction between the modified biomaterial and the wound tissue due to the large surface area.
Aldehyde Functionalized Alginate Hydrogels
Lu et al. (Deng et al., 2020) synthesized a multifunctional alginate hydrogel for healing complex skin injuries. Their study developed a sodium alginate aldehyde hydrogel and cross-linked it with divalent Strontium ions (Sr 2+). The rationale behind their study incorporating an aldehyde group to their alginate was to achieve excellent biocompatibility, biodegradability, non-toxic, and improved swelling properties and could adhere closely to tissues. They also observed self-healing abilities due to the slow release of strontium ions. In addition to this, the hydrogel promoted immediate and fast angiogenesis of the skin tissue. This occurred due to the slow release of the strontium ions which have been found to play an important role in promoting angiogenesis of skin tissue. The repair of the defects was accelerated at the same time. Such properties imparted to alginate are suitable for allowing rapid skin defects in complex wound injuries (Mondal et al., 2020).
This type of study was significant in shedding light on other focal areas in which alginate can be modified to advance wound healing. Such areas of study should be further evaluated as most studies only focus on the healing of wounds using composite biomaterials or synthetic polymers with biopolymers. In contrast, few studies have considered evaluating the skin tone restoration and architecture using functionalized polysaccharides. In addition to this it would be interesting to conduct research on the hemostatic potentials of these aldehyde-based alginate hydrogels.
Arginine-Glycine-Aspartic Acid Derivatized Alginate Hydrogel
Even though alginate has excellent biocompatibility properties, it is made of inert monomers that naturally lack the bioactive ligands necessary for cell anchoring. This cell-crosslinking method introduces ligands, such as the arginine-glycine-aspartic acid (RGD) sequence, onto alginate for cells to adhere to the Alginate-RDG modified hydrogel by chemically coupling, which uses water-soluble carbodiimide synthesis has been extensively used (Ansari et al., 2021). Upon the addition of mammalian cells to the RGD-modified alginate, a uniform dispersion is formed within the solution, and receptors follow this on the cell surface, binding to the RDG-Alginate ligands. The RGD-modified alginate solution is subsequently cross-linked to form network structures through specific receptor-ligand interactions between the cells and RGD sequences. The combination of RGD-alginate-with stem cells can further accelerate and improve soft tissue regeneration. Ansari et al. (2021) reported the use of Gingival mesenchymal stem cells (GMSCs) in RGD modified alginate hydrogels. The regenerative potential of mesenchymal stem cells (MSCs) encapsulated in hydrogels is well documented, however for polysaccharide-based polymers little work has been reported for wound healing therapy. Here, an RGD-alginate–gelatin-methacrylate (GelMA) hydrogel formulation was developed encapsulating GMSCs within the developed hydrogel. From their results it was observed that the encapsulated MSCs remain viable within the hydrogel with enhanced collagen deposition. An excisional wound model in mice was utilized in their study to evaluate the in vivo functionality of the RGD-Alginate GMSC–hydrogel construct for wound healing and soft tissue regeneration. The histology and immunofluorescence analyses confirmed the effectiveness of the GMSC–hydrogel in expediting wound healing was through enhancing angiogenesis and suppressing local proinflammatory cytokines. Based on these findings, it can be seen that GMSCs encapsulated in an functionalized polysaccharide (alginate) based sheet hydrogel can be used to expedite wound healing and soft tissue regeneration, with potential applications in not just wound healing therapy but also plastic and reconstructive surgery as well as dentistry (Ansari et al., 2021).
Krause et al. (Krause et al., 2012) concluded that RGD-pentapeptide functionalized hydrogel exhibit a biomimetic microenvironment on wounds. Their study further displayed enhanced biocompatibility, cell proliferation, cell adhesion, and good biodegradation (Yu et al., 2010). Although the cell-crosslinked hydrogel shows excellent bioactivities, the major drawbacks that the network exhibited were low mechanical strength and toughness, limiting the practical applications of their advanced biomaterial. Such types of hydrogels could be made as a 3D printed scaffold in combination with an additional polymer such as chitosan, to maximize the systems interaction with the wound. This approach could further enhance the mechanical strength of the scaffold and improve the absorptive (due to a controllable pore size), cell adhesion (presence of RDG) cell proliferative capabilities (chitosan) and hemostatic (chitosan properties) properties of the system. Studies should not look at the functionalization of one specific polymer but rather a combination of polymers which can be combined to produce or develop a product with novel properties. Very few of these types of studies involving RDG and two types of polysaccharide-based polymers have been reported in literature.
Chitosan-Based Formulations
Heparin Functionalized Chitosan Hydrogels
Peptide-based polysaccharide formations have attracted significant attention over the years. This an attractive strategy for wound healing biomaterials, except for using the delivery of blood factors as an approach, is to derivatize the natural polysaccharide materials with amino acid sequences that would interact with surface receptors of dermal cells. Such receptors are those of skin cells, thus enabling cell differentiation, proliferation, and cell migration, thus enabling a more rapid and complete healing process. Heparin functionalized with chitosan has favorable outcomes in the last phases of wound healing stages. Kratz et al. (Kampf et al., 2018) formulated heparin complexed chitosan bio-platform. Their study discovered heparin-chitosan platforms to have wound healing potential, which was depended on the concentration of heparin. Furthermore, 1.9% (w/w) heparin could not induce re-epithelialization, but 7.7% of heparin (w/w) generated almost complete re-epithelialization. From this, it can be concluded that the time-controlled delivery of heparin at the correct dose is required to reach therapeutic efficacy. It was further observed that heparin-chitosan hydrogels exhibited no tissue ingrowth, resulting in the formation of a highly vascularized granulation layer (Krajewska, 2004). Thus, implying that heparin functionalized chitosan’s ability to initiate an angiogenic response. Jin et al. (Mi et al., 2002) compared chitosan’s effect when used as a powder, without and with the presence of heparin on deep partial burns. The wounds were created on the dorsum of rats. Following 72 h, their study’s histology results revealed that chitosan in its powdered form stopped an early extension of burns, while the peptide under study, whether by itself or embedded inside the polysaccharide, was not effective (Kampf et al., 2018). Based on most of literature the contrary, heparin functionalized with chitosan exhibited positive effects on the last stages of healing.
Arginine-Glycine-Aspartic Acid (RGD) Functionalized Chitosan Hydrogels
Arginine-glycine-aspartic acid (RGD) sequence has been found to induce cell adhesion by binding and activating integrin, allowing cell to cell and cell to extracellular matrix adhesion (Kratz et al., 1997). Arginine-glycine-aspartic acid (RGD) functionalized with chitosan were tested for cell adhesive properties to the extracellular matrix (ECM) (Chai et al., 2017). It was shown that RGD functionalization onto chitosan biomaterials imparts improved properties. Such properties are cell adhesive and biocompatibility properties. This process occurs because the adhesion cell receptors recognize RGD. Therefore, the functionalized chitosan takes up an artificial ECM role, allowing cellular adhesion to occur (Jin et al., 2007), these finding further show the potential which chitosan has in wound healing therapy. Furthermore, the combination of peptides with natural polymers yields numerous properties in the product which is much more suitable for wound care application as compared to the pristine polymers. Karakecili et al. (Chai et al., 2017) studied cell interactions with peptides using chitosan membranes functionalized with RGD sequences. Results from the in vitro study suggested that L929 mouse fibroblasts exhibited an increased cell attachment with time. They concluded that RGD was crucial for cell attachment, cell migration, and cell proliferation. Their findings further show the importance of attaching key peptides with known wound healing properties to natural biomaterials like alginate to impart favorable properties to the biomaterials. The relevance of their study further highlights the role cell attachment, cell migration and cell proliferation in the wound healing therapy.
Ammonium Functionalized Chitosan Hydrogels
One of the many factors that limit the application of native chitosan is its non-solubility in neutral and alkaline aqueous solutions (Jia et al., 2001). N-trimethyl chitosan chloride and found that it interacts with negatively charged cell membranes thus, inhibiting the growth of microorganisms (Abueva et al., 2021). They also observed that their synthesized N-trimethyl chitosan was water-soluble, and therefore an important new property was obtained compared to the pristine polymer. They further observed that the chitosan’s heterogeneous chemical modification could tune the hydrogel’s surface properties. Their observations noted that when a stearoyl group was attached to N-Trimethyl chitosan hydrogels, the hydrogels became more hydrophobic and promoted protein adsorption. Antimicrobial resistance has been a problem for public health professionals. Therefore, wound healing patches with broad spectrum bioactivity against Hospital-Associated Multi-Drug resistance (MDR) strains of bacterial and fungal pathogens is important. Abueva et al. (Jia et al., 2001) N,N,N-trimethyl chitosan chloride (TMC) with inherent bactericidal property was synthesized and complexed with povidone-iodine (PVP-I) to create a potentially more potent antibacterial properties that could also significantly enhance the wound healing process. TMC, a positively charged, water-soluble derivative of chitosan, formed stable solutions with PVP-I at 5% w/v TMC concentration. TMC5/PVP-I was significantly effective against multidrug-resistant bacteria S. aureus compared with PVP-I alone. TMC/PVP-I solutions also showed fungicidal property against C. albicans. This further illustrates that although derivatization may impart new and useful properties to alginate, further modification or analogs of quaternary ammonium alginates can introduce novel properties beneficial for wound healing application. Chitosan’s insolubility in water makes it difficult for the biomaterial to dissolve into wound fluid over time as it degrades. However, by functionalizing the biomaterial with a trimethyl group chitosan then become more wound friendly. This is a major achievement as one of the ideal properties of any wound healing system is its ability to solubilize in aqueous solutions.
N-Succinyl Chitosan
Succinyl chitosan (NSC) is synthesized through acyl group introduction. These groups’ introduction imparts chitosan with excellent biological, chemical, and physical attributes (Nam et al., 2004). NSC has excellent water retention attributes, which can be exploited for the development of wound healing matrices. Straccia et al. (Zhai et al., 2002) developed a hydrogel consisting of NSC with alginate, and this wound healing material exhibited antimicrobial activity, showed good stability, a significant degree of swelling, and a regulated rate at which water vapor is transmitted, most modified polysaccharide biomaterials exhibit poor absorption especially pristine chitosan.
Furthermore, chitosan-based hydrogel was effective in maintaining a moist wound environment resulting in the enhancement of tissue regeneration and epithelialization (Kamoun and Menzel, 2012). Also, NSC bio-platforms were evaluated in vivo, and their result suggested that the NCS system successfully prevented microbial infection and improved wound healing. In a different approach Qing et al. (2021) reported that polyvinyl alcohol (PVA)/N–succinyl chitosan (NSCS)/lincomycin hydrogels were effective in their wound healing properties. From their results it can be observed that the introduction of NSCS remarkably enhanced the swelling capacity, leading to the maximum swelling ratio of 19.68 g/g in deionized water. The optimal compression strength of 0.75 MPa was achieved with 30% NSCS. Additionally, the incorporation of lincomycin brought a remarkable antibacterial activity against both Escherichia coli and Staphylococcus aureus. Their hydrogel showed not toxicity properties. Therefore,/NSCS/lincomycin hydrogel shows promising potential for wound dressing due to its non-toxic, excellent absorption and significant compression strength which makes this functionalized composite suitable for wound healing. Such findings further show that the combination of functuionalized polymers with other polymers can further improve the properties of the pristine and functionalized polymer. Modified types of Chitosan hydrogels are shown in Table 2. These findings show that NSC or NSC-Alginate possess most of the properties required for an accelerated and successful wound healing process. The combination of NSC with alginate further shows the importance of combining a modified polymer with a pristine polymer to achieve a variety of new properties which will be beneficial for wound healing.
Drawbacks of Alginate and Chitosan-Based Hydrogels
Natural polysaccharides have generally demonstrated favourable properties which allow them to be used as a therapeutic option for chronic wounds. Despite this, a natural polysaccharide may elicit the immune system to react adversely and cause irritation. Hence, the natural polysaccharide’s molecular weight control may be used to overcome such limitations. In addition to this, in dry wounds, these properties could affect the wound healing process (Saarai et al., 2012). This may result in dehydration, thus reducing blood flow and the ability of epithelial cell migration within the wound site, interrupting new tissue creation. As evidence, re-epithelialization of the wound site is more rapid under moist conditions than under dry ones with natural polysaccharide wound dressing treatment (Kamoun et al., 2017), with a controllable molecular weight, probably, the capabilities of natural polysaccharides in speeding up the wound healing process can be exploited to treat various types of wound injury. The advantages and disadvantages of hydrogel wound dressing materials are shown in Table 3, as reported by Kamoun et al. (Price et al., 2007).
Natural polysaccharides are the human dermal Extracellular matrix ECM (Suzuki et al., 1998). Polysaccharides are abundant in nature, and they have been shown to have great potential for pharmaceutical, medical and biomedical applications. Wound healing dressings used for tissue regeneration have been developed from polysaccharides because of their economic, low toxic nature, and favourable compatibility profile. However, possessing a lack of protein structure, natural polysaccharide exhibits a very poor bio-stability and difficulty assembling a “matrix” to cover the damaged tissue during the wound healing, thus facilitating contraction of the wound resulting in scar formation (Chansiripornchai et al., 2003). In addition to this, the synthesis of temperature or pH-responsive and functionalized biomaterials requires toxic chemicals that are expensive and hazardous.
Properties of an Ideal Polysaccharide-Based Hydrogel for Wound Healing
Polysaccharide hydrogels are considered effective candidates for wound healing therapy. This because of their high water-retaining capacity, non-toxicity, biodegradability, and biocompatibility (Winter, 1962; Silva et al., 2018; Zhu et al., 2019). Over the years, different approaches in designing or developing efficient and effective wound dressing materials have been considered; it has been demonstrated that healing of wounds with wet dressings is faster than dried dressings. This is ultimately ascribed to the healing and formation of renewed skin without eschars or inflammation, taking place only in a wet environment (Lee and Mooney, 2012).
Thus, wet or moisten dressings were the suitable dressing candidate for skin repair, hence the successful applicability of hydrogel dressings in wound care therapy due to their high-water content and inherent permeability (Becker et al., 2016). An ideal wound dressing material should fulfil the following characteristics, as shown in Table 4 (Winter, 1962). If most of these properties can be contained in a developed hydrogel system, this would result in wound healing taking place efficiently and faster in a wet environment, as provided by hydrogel in contrast to the dried environment. Given the growing demand, developments and manufactures of novel wound dressings with high performance have become a research focus in the field of medical materials, among which hydrogel is found to satisfy most of the criteria for treating and managing wounds. A hydrogel is a 3D network composed of hydrophilic polymers, which can absorb and swell in water because of the highly mimic natural extracellular matrix (ECM) properties, hydrogel has been extensively utilized in pharmaceutical and biomedical applications. In terms of wound dressings, hydrogel do not only form a physical barrier and remove excess exudate but also encapsulate bioactive molecules and provide a moisture environment that promotes the wound healing process (Silva et al., 2018).
In addition, the injectable hydrogel would also be able to completely fulfill the irregular shaped wound and deal with deep bleeding wound more efficiently. Due to the numerous merits of hydrogels, a series of commercial hydrogels as wound dressings have been emerged, such as Algisite M, Tegaderm TM hydrocolloid dressing, Evicel VR, and Coseal VR. Despite their good wound healing performance, there exist some deficiencies, including high cost, harsh storage conditions, inability to provide adequate mechanical protection, and poor permeability of gases. Since the demand for higher performance dressings, novel hydrogel dressings with multifunctional properties (antibacterial ability, biodegradability, responsiveness, and injectability) received increasing attention in the field of wound dressings in recent years (Lee and Mooney, 2012; Zhu et al., 2019). Interestingly, hydrogels can control scab formation and allow a swift and well-coordinated cell proliferation and epithelialization process to take place. Furthermore, smart hydrogels’ development using modern technology and software has used some of these ideal properties to develop the most sophisticated biomaterial dressings that are beneficial for wound healing therapeutics.
Smart and Inteligent Hydrogels Used in Wound Healing Therapy
Smart drug delivery devices were designed to control the level of drug, cytokines, pH, and temperature in patients at desirable levels (Stewart, 2002). Existing medical instruments can either monitor or deliver bioactive agents. Such individuals must interpret information and recommend the appropriate therapy course. Electronic devices’ advancements have led to the development of devices with fast data interpreting abilities in wearable and portable forms, which have been attached to the biopolymer (Figures 4–6). Other devices include the development of microneedles, which penetrate through the wound to all direct delivery of active drugs or growth factors and provide direct interaction of the biomaterial with the wound. Such devices also have a feature that allows them to be paired to wireless devices through Bluetooth communication, Infrared, or internet connectivity (Kasagana and Karumuri, 2011).
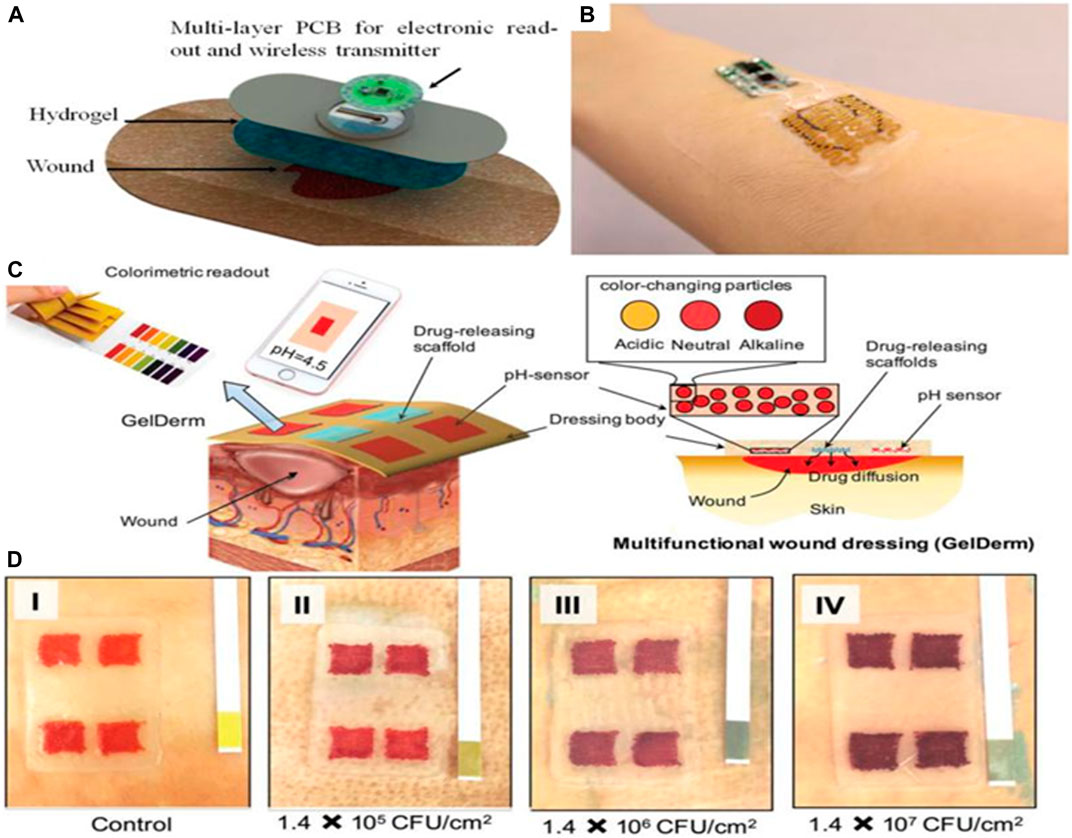
FIGURE 4. Smart and automated bandages for treatment of chronic wounds. (A) Schematic of multi-layer dressing with both sensing and drug delivery. The onboard electronics can process the data and trigger the drug delivery if needed. (B) A photograph of the wearable bandage with both sensing and drug delivery capabilities. (C) Schematic of a 3D printed bandage with colorimetric pH sensor and drug delivery capability. (D) Effect of bacterial culture on the color of the engineered bandage.
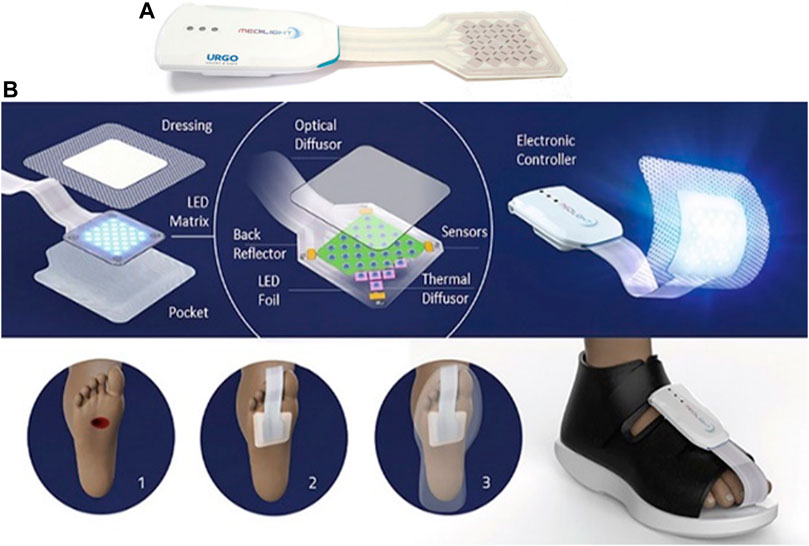
FIGURE 6. (A) Developed smart foot patch used in the treatment of chronic wounds, with the permission from MEDILIGHT © Group AMIRES-EU Projects, (B1) Illustration of the components and application of the MEDILIGHT © foot patch used in wound healing therapy. Depiction of bare chronic wound, (B2) depiction of the MEDILIGHT © foot patch being applied on a patient’s foot, and (B3) MEDILIGHT © foot patch appearance when the shoes are fitted on.
Thus, allowing the data to be quickly processed onboard or online, and the decisions can be made automatically for proper treatment of the wounds. Chronic wounds affect many populations and the continuous need for hospital visit by patients to be assessed on their wound healing progress place substantial pressure on medical professionals and patients. The development of such technologies will assist in the simplification of wound management and assist in the reduction or eradication of the transmission of wound related infections. These intelligent systems or devices also provide medical stuff and patients with confidence and certainty of the wound healing progress of the injury(s) being treated in real-time.
Smart Bandages
Two separate studies looked at the development of automated bandages equipped with either pH sensors, drug delivery modules, or temperature sensors (Figure 4) (Botsis and Hartvigsen, 2008; Mirani et al., 2017). Critical pH values can be set on the controller, and once the pH reaches outside the acceptable range, the heater is triggered to release antibiotics. Overall, this area is still developing, and it is expected that more advanced automated devices are to be engineered. A pain-free and easy-to-use automated system would revolutionize the way hydrogels are used and how they respond to different types of wounds. Such systems’ effectiveness can be enhanced by incorporating nanosystems that can offer dual insulin delivery (De la Harpe et al., 2020), a drug containing nanoparticles (Chivere et al., 2020), to chronic wounds. Mostafalu et al. (2015) were the first to design and develop an integrated multilayer bandage. An array of electrochemical pH sensors was embedded within a hydrogel layer carrying thermo-responsive drug carriers cast on a flexible heater (Mirani et al., 2017). The sensors and heater were connected to a microcontroller that could communicate with smartphones. The nanosystem proposed by Essa et al. (De la Harpe et al., 2020) makes use of a hydrophobic core, a cationic component, and surface density of Polyethylene glycol (PEG) chains, which created favorable-sized particles and drug entrapment efficiencies suitable for drug delivery. Their hydrophobic poly (lactide-co-glycolide) (PLGA) core further encouraged a high degree of carrier-drug interactions. Simultaneously, chitosan and PEG coatings provided cationic and hydrophilic properties to enable enhanced biocompatibility and solubility.
Microneedle Based-Smart Wound Patches
In 2012, the first hydrogel-forming microneedle (HFMs) were the newest form of MNs (Figure 5) (Cheung and Das, 2016). They were made of polymers with swelling properties (cross-linked); these HFMs exhibited different operation mechanisms compared to other microneedles. Hollow microneedles are solid needles and have a hollow center that enables the delivery of desired drugs upon applying pressure or response to the pH or temperature of the wound. HFMs swell when inserted into the skin and release drugs, and uptake wound fluid (Cheung and Das, 2016; Chai et al., 2017). The development of Injectable smart thermo-responsive hydrogel systems for biomedical applications has sparked great attention over the years (Essa et al., 2020; Popescu et al., 2021). Injectable smart hydrogel can be introduced into solid biodegradable microneedles. Since microneedles interact directly with damaged tissue, they can be directly applied in the same way injectable hydrogel would have been directly injected into the wound. This method would be less painful and long-lasting. A casted microneedle casing could be made using RGD peptide-functionalized chitosan or alginate hydrogel as the starting polymer. The smart thermoresponsive hydrogel would then be pumped into the microneedle. Therefore, the biopolymer acts as the second layer within the microneedle upon degradation of the functionalized starting polymer. The second hydrogel, which may contain growth factors, drugs, stem cells, or nanoparticles, is released. These agents would interact with the wound in a sustained manner following the starting polymers degradation as its role would be to attach the microneedles to cells following the end of the first stages of wound healing. After this, the release of growth factors and drugs will encourage sustainable wound healing. Thus, allowing complete tissue regeneration.
LED-Based System for Treating Foot-Based Chronic Wounds
Based on what is reported in the literature, light therapy could be used for various medical conditions, especially skin-related abnormalities or even tissue regeneration. Non-healing of acute or chronic wounds occurs because of impaired cell function, unregulated and frequent inflammatory processes, and molecular deficiencies inside the wound (Chi et al., 2020). The antimicrobial and anti-proliferative effects of blue light are well known and explicitly described in the literature (Frykberg and Banks, 2015; Mamalis et al., 2015). MEDILIGHT suggested that blue light could be used in the early wound healing stages to enhance wound healing and inhibit bacterial growth. It is also essential to prevent excess epimerization by keratinocytes at the wound surface as this can lead to premature closure of the trauma. Contrary, red light is supposed to stimulate cell proliferation, migration, and differentiation (Dai et al., 2012), which is vital in later stages of healing, leading to a wound’s closure. This process would start right from the wound bed. Figures 6A,B shows the smart patch prototype, which can be controlled via Bluetooth and modern mobile software.
Future Prospects
It is therefore essential to gain insight into the benefit of using other derivatization approaches together with the combination of sophisticated electronic devices as this will provide new information and data as to how existing systems can be further enhanced and controlled electronically to best suit the intended biomedical application. The new properties gained can therefore be practically understood before being translated to human wound healing application(s). It is for this reason why there are a few smart hydrogels are being commercialized, in addition to this the rate at which these technologies are being commercialized is very slow. New and promising applications of polysaccharide-based hydrogels like stable or semisolid-state microneedle hydrogels, bioinspired smart, flexible LED foot patches for robust electrolyte and flexible electronic devices, controlled growth factors release, and medications, pH, and temperature response should be further studied as these advanced technological bio-platforms are the future of biomedical therapeutics.
Although these technologies have been developing for some time now, only a few of these smart devices have reached the commercialization stage; secondly, these smart devices have been studied using the combination of biopolymers and synthetic polymers. None of them looked at the applicability of functionalized hydrogels with smart systems for example RGD-Alginate microneedle system which is linked to a electrical smart biosensor device. More defined biomarkers and wearable sensors should be identified for rapid diagnosis and treatment as this allows accurate diagnostics and provide advanced wound care therapy using a combination of multi-drug delivery systems. These include the measurement of oxygen levels, moisture content of the wound and pH of the wound, collagen and hydroxylysine levels, reactive oxygen species (ROS) concentrations. The advantage of smart bandages is that they are automated and can efficiently respond to the recorded data.
Thus, initiating a therapeutic response usually does not require a single drug or initiator molecule to induce healing or alleviate patients’ symptoms. Continuous research is necessary to develop cheaper, unique, versatile, and smart wound healing hydrogels that will significantly improve patient compliance, accessibility, and a greener environment. Challenges such as controllable drug release, hydrogel interaction with human wound fluid and tissue, and the biodegradation of polysaccharide-based hydrogels in vivo is another a big problem which still needs to be solved in the future, this requires more extensive to be done. Lastly since it is very difficult to translate in vitro based studies to in vivo studies as the two study approaches offer varying environmental conditions and these parameters influence the overall applicability of the developed biomaterial system novel testing approaches need to be developed to ensure that the data obtained at levels of production is accurate and ensures that the developed biomaterial can work on human beings.
Conclusion
Alginate and chitosan-modified hydrogels possess unique properties such as excellent water retention abilities, biocompatibility with human beings and animals, biodegradable properties, and non-toxic nature. Such properties can be very useful in the research and development of smart wound healing biomaterials. In addition to this, such polysaccharide-based platforms are advantageous in specific medical applications due to their structure, which can be manipulated to have desired physical or chemical properties.
Such gels would help facilitate several functions such as controlling the rate of diffusion, response to environmental stimulus, growth factor, drug release, and gas exchange between the wound and external environment. Even though polysaccharide-based hydrogels have become popular and possess numerous superiorities.
Significant obstacles that need to be circumvented for polysaccharide hydrogels’ to be used as commercial products still exist even today. The major issues include introducing hazardous solvents or reagents during the synthesis process, modification, and cross-linking of the hydrogels. Therefore, there is an urgent need to explore advanced ways of chemically developing smart polysaccharide platforms with broad biomedical application properties not just for general wound healing but also skin cancer, pressure sores, and ulcer-related wounds. The use of green chemistry and advanced technological devices can be used as a smart approach in achieving this. In addition to this, it remains a significant challenge not just for wound healing but also for drug management and patient compliance as products are not environmentally friendly and affordable for purchase by people, especially the poor. Research should also be streamlined towards achieving biopolymers with unique properties, such as excellent stability, ability to maintain suitable pH required for wound healing, wound fluid enzyme tolerance, sound absorption, improve bioavailability of loaded drug, self-healing properties, provide mechanical support and protection to the wound, superior elasticity and can be integrated to electronic devices with ease.
Author Contributions
The manuscript was completed through contributions of all authors. CK, PPDK, PK and YEC conceived, designed the framework and main content of the manuscript, as well as wrote and revised the manuscript. All authors finally approved the submission.
Funding
This work was financially supported by the National Research Foundation (NRF) of South Africa.
Conflict of Interest
The authors declare that the research was conducted in the absence of any commercial or financial relationships that could be construed as a potential conflict of interest.
Publisher’s Note
All claims expressed in this article are solely those of the authors and do not necessarily represent those of their affiliated organizations, or those of the publisher, the editors and the reviewers. Any product that may be evaluated in this article, or claim that may be made by its manufacturer, is not guaranteed or endorsed by the publisher.
Acknowledgments
Professor Viness Pillay, who passed away on July 24, 2020, is hereby kindly acknowledged and remembered for his contributions to the conceptualization of this work.
References
Abueva, C., Ryu, H. S., Min, J. W., Chung, P. S., Sook, Y. H., Suk, Y. M., et al. (2021). Quaternary Ammonium N, N, N-Trimethyl Chitosan Derivative and Povidone-Iodine Complex as a Potent Antiseptic with Enhanced Wound Healing Property. Int. J. Biol. Macromol 182, 1713. doi:10.1016/j.ijbiomac.2021.05.153
Aderibigbe, B. A., and Buyana, B. (2018). Alginate in Wound Dressings. Pharmaceutics 10 (2), 42. doi:10.3390/pharmaceutics10020042
Almeida, J. F., Ferreira, P., Lopes, A., and Gil, M. H. (2011). Photocrosslinkable Biodegradable Responsive Hydrogels as Drug Delivery Systems. Int. J. Biol. macromolecules 49 (5), 948–954. doi:10.1016/j.ijbiomac.2011.08.010
Ansari, S., Pouraghaei Sevari, S., Chen, C., Sarrion, P., and Moshaverinia, A. (2021). RGD-modified Alginate–GelMA Hydrogel Sheet Containing Gingival Mesenchymal Stem Cells: A Unique Platform for Wound Healing and Soft Tissue Regeneration. ACS Biomater. Sci. Eng. doi:10.1021/acsbiomaterials.0c01571
A. Vishwakarma, P. Sharpe, S. Shi, and M. Ramalingam (Editors) (2014). Stem Cell Biology and Tissue Engineering in Dental Sciences (Cambridge: Academic Press).
Baranoski, S., and Ayello, E. A. (2008). Wound Care Essentials: Practice Principles. Philadelphia: Lippincott Williams & Wilkins.
Becker, A., Klapczynski, A., Kuch, N., Arpino, F., Simon-Keller, K., De la Torre, C., et al. (2016). Gene Expression Profiling Reveals Aryl Hydrocarbon Receptor as a Possible Target for Photobiomodulation when Using Blue Light. Sci. Rep. 6 (1), 1–11. doi:10.1038/srep33847
Boateng, J. S., Matthews, K. H., Stevens, H. N. E., and Eccleston, G. M. (2008). Wound Healing Dressings and Drug Delivery Systems: a Review. J. Pharm. Sci. 97 (8), 2892–2923. doi:10.1002/jps.21210
Botsis, T., and Hartvigsen, G. (2008). Current Status and Future Perspectives in Telecare for Elderly People Suffering from Chronic Diseases. J. Telemed. Telecare 14 (4), 195–203. doi:10.1258/jtt.2008.070905
Bradford, C., Freeman, R., and Percival, S. L. (2009). In Vitro study of Sustained Antimicrobial Activity of a New Silver Alginate Dressing. The J. Am. Coll. Certified Wound Specialists 1 (4), 117–120. doi:10.1016/j.jcws.2009.09.001
Chai, Q., Jiao, Y., and Yu, X. (2017). Hydrogels for Biomedical Applications: Their Characteristics and the Mechanisms behind Them. Gels 3 (1), 6. doi:10.3390/gels3010006
Chansiripornchai, P., Pramatwinai, C., Rungsipipat, A., Ponsamart, S., and Nakchat, O. (2003). “February. The Efficiency of Polysaccharide Gel Extracted from Fruit-Hulls of Durian (Durio Zibethinus L.) for Wound Healing in Pigskin,” in III WOCMAP Congress on Medicinal and Aromatic Plants, Chiang Mai, February 1, 2005, 37–43.
Cheung, K., and Das, D. B. (2016). Microneedles for Drug Delivery: Trends and Progress. Drug Deliv. 23 (7), 2338–2354. doi:10.3109/10717544.2014.986309
Chi, J., Zhang, X., Chen, C., Shao, C., Zhao, Y., and Wang, Y. (2020). Antibacterial and Angiogenic Chitosan Microneedle Array Patch for Promoting Wound Healing. Bioactive Mater. 5 (2), 253–259. doi:10.1016/j.bioactmat.2020.02.004
Chivere, V. T., Kondiah, P. P. D., Choonara, Y. E., and Pillay, V. (2020). Nanotechnology-based Biopolymeric Oral Delivery Platforms for Advanced Cancer Treatment. Cancers 12 (2), 522. doi:10.3390/cancers12020522
Coger, V., Million, N., Rehbock, C., Sures, B., Nachev, M., Barcikowski, S., et al. (2019). Tissue Concentrations of Zinc, Iron, Copper, and Magnesium during the Phases of Full Thickness Wound Healing in a Rodent Model. Biol. Trace Elem. Res. 191 (1), 167–176. doi:10.1007/s12011-018-1600-y
Cullum, N., Buckley, H., Dumville, J., Hall, J., Lamb, K., Madden, M., et al. (2016). “Data Extraction Tables: Silver Dressings for Venous Leg Ulcers,” in Wounds Research for Patient Benefit: A 5-year Program of Research (Southampton United Kingdom: The National Institute for Health Research (NIHR)).
Dai, T., Gupta, A., Murray, C. K., Vrahas, M. S., Tegos, G. P., and Hamblin, M. R. (2012). Blue Light for Infectious Diseases: Propionibacterium Acnes, Helicobacter pylori, and beyond?. Drug Resist. Updates 15 (4), 223–236. doi:10.1016/j.drup.2012.07.001
De la Harpe, K. M., Kondiah, P. P., Marimuthu, T., Du Toit, L. C., Kumar, P., Choonara, Y. E., et al. (2020). An Advanced 3D Monofilament Biosuture. South Afr. Pharm. J. 87 (1), 45–48.
Deng, Z., Wang, H., Ma, P. X., and Guo, B. (2020). Self-healing Conductive Hydrogels: Preparation, Properties and Applications. Nanoscale 12 (3), 1224–1246. doi:10.1039/c9nr09283h
Ding, X., Duan, S., Ding, X., Liu, R., and Xu, F.-J. (2018). Versatile Antibacterial Materials: an Emerging Arsenal for Combatting Bacterial Pathogens. Adv. Funct. Mater. 28 (40), 1802140. doi:10.1002/adfm.201802140
Essa, D., Choonara, Y. E., Kondiah, P. P. D., and Pillay, V. (2020). Comparative Nanofabrication of PLGA-Chitosan-PEG Systems Employing Microfluidics and Emulsification Solvent Evaporation Techniques. Polymers 12 (9), 1882. doi:10.3390/polym12091882
Frykberg, R. G., and Banks, J. (2015). Challenges in the Treatment of Chronic Wounds. Adv. Wound Care 4 (9), 560–582. doi:10.1089/wound.2015.0635
Greenwood, J. E., and Dearman, B. L. (2012). Split Skin Graft Application over an Integrating, Biodegradable Temporizing Polymer Matrix. J. Burn Care Res. 33 (1), 7–19. doi:10.1097/bcr.0b013e3182372be9
Gupta, A., Kowalczuk, M., Heaselgrave, W., Britland, S. T., Martin, C., and Radecka, I. (2019). The Production and Application of Hydrogels for Wound Management: A Review. Eur. Polym. J. 111, 134–151. doi:10.1016/j.eurpolymj.2018.12.019
Harding, K. G., Jones, V., and Price, P. (2000). Topical Treatment: Which Dressing to Choose. Diabetes Metab. Res. Rev. 16 (S1), S47–S50. doi:10.1002/1520-7560(200009/10)16:1+<::aid-dmrr133>3.0.co;2-q
Heo, Y., Akimoto, J., Kobatake, E., and Ito, Y. (2020). Gelation and Release Behavior of Visible Light-Curable Alginate. Polym. J. 52 (3), 323–332. doi:10.1038/s41428-019-0280-6
Huang, S., and Fu, X. (2010). Naturally Derived Materials-Based Cell and Drug Delivery Systems in Skin Regeneration. J. Controlled Release 142 (2), 149–159. doi:10.1016/j.jconrel.2009.10.018
Jia, Z., shen, D., and Xu, W. (2001). Synthesis and Antibacterial Activities of Quaternary Ammonium Salt of Chitosan. Carbohydr. Res. 333 (1), 1–6. doi:10.1016/s0008-6215(01)00112-4
Jin, Y., Ling, P.-X., He, Y.-L., and Zhang, T.-M. (2007). Effects of Chitosan and Heparin on Early Extension of burns. Burns 33 (8), 1027–1031. doi:10.1016/j.burns.2006.12.002
Kamoun, E. A., Kenawy, E.-R. S., and Chen, X. (2017). A Review on Polymeric Hydrogel Membranes for Wound Dressing Applications: PVA-Based Hydrogel Dressings. J. Adv. Res. 8 (3), 217–233. doi:10.1016/j.jare.2017.01.005
Kamoun, E. A., and Menzel, H. (2012). HES-HEMA Nanocomposite Polymer Hydrogels: Swelling Behavior and Characterization. J. Polym. Res. 19 (4), 9851. doi:10.1007/s10965-012-9851-3
Kampf, N., Wachtel, E. J., Zilman, A., Ben-Shalom, N., and Klein, J. (2018). Anomalous Viscosity-Time Behavior of Polysaccharide Dispersions. J. Chem. Phys. 149 (16), 163320. doi:10.1063/1.5027720
Kasagana, V., and Karumuri, S. S. (2011). Recent Advances in Smart Drug Delivery Systems. Int. J. Novel Drug Deliv. 1 (3), 201–207.
Krajewska, B. (2004). Application of Chitin-And Chitosan-Based Materials for Enzyme Immobilizations: a Review. Enzyme Microb. Technol. 35 (2-3), 126–139. doi:10.1016/j.enzmictec.2003.12.013
Kratz, G., Arnander, C., Swedenborg, J., Back, M., Falk, C., Gouda, I., et al. (1997). Heparin-chitosan Complexes Stimulate Wound Healing in Human Skin. Scand. J. Plast. Reconstr. Surg. Hand Surg. 31 (2), 119–123. doi:10.3109/02844319709085478
Krause, A., Kirschning, A., and Dräger, G. (2012). Bioorthogonal Metal-free Click-Ligation of cRGD-Pentapeptide to Alginate. Org. Biomol. Chem. 10 (29), 5547–5553. doi:10.1039/c2ob25604e
Kuivaniemi, H., and Tromp, G. (2019). Type III Collagen (COL3A1): Gene and Protein Structure, Tissue Distribution, and Associated Diseases. Gene 707, 151. doi:10.1016/j.gene.2019.05.003
Lee, K. Y., and Mooney, D. J. (2012). Alginate: Properties and Biomedical Applications. Prog. Polym. Sci. 37 (1), 106–126. doi:10.1016/j.progpolymsci.2011.06.003
Lee, K. Y., and Mooney, D. J. (2012). Alginate: Properties and Biomedical Applications. Prog. Polym. Sci. 37 (1), 106–126. doi:10.1016/j.progpolymsci.2011.06.003
Li, M., Li, H., Li, X., Zhu, H., Xu, Z., Liu, L., et al. (2017). A Bioinspired Alginate-Gum Arabic Hydrogel with Micro-/nanoscale Structures for Controlled Drug Release in Chronic Wound Healing. ACS Appl. Mater. Inter. 9 (27), 22160–22175. doi:10.1021/acsami.7b04428
Liang, M., Chen, Z., Wang, F., Liu, L., Wei, R., and Zhang, M. (2019). Preparation of Self‐regulating/anti‐adhesive Hydrogels and Their Ability to Promote Healing in Burn Wounds. J. Biomed. Mater. Res. 107 (5), 1471–1482. doi:10.1002/jbm.b.34239
Line, A. S., Morykwas, M. J., and Line, S. W. (1995). Use of Cultured Human Epidermal Xenografts for Wound Treatment in Nonhuman Primates. J. Zoo Wildl. Med., 517–524.
Lynch, C. R., Kondiah, P. P. D., Choonara, Y. E., Du Toit, L. C., Ally, N., and Pillay, V. (2020). Hydrogel Biomaterials for Application in Ocular Drug Delivery. Front. Bioeng. Biotechnol. 8, 228. doi:10.3389/fbioe.2020.00228
Mamalis, A., Garcha, M., and Jagdeo, J. (2015). Light Emitting Diode-Generated Blue Light Modulates Fibrosis Characteristics: Fibroblast Proliferation, Migration Speed, and Reactive Oxygen Species Generation. Lasers Surg. Med. 47 (2), 210–215. doi:10.1002/lsm.22293
Mansoor, S., Kondiah, P. P. D., Choonara, Y. E., and Pillay, V. (2019). Polymer-based Nanoparticle Strategies for Insulin Delivery. Polymers 11 (9), 1380. doi:10.3390/polym11091380
Mi, F.-L., Wu, Y.-B., Shyu, S.-S., Schoung, J.-Y., Huang, Y.-B., Tsai, Y.-H., et al. (2002). Control of Wound Infections Using a Bilayer Chitosan Wound Dressing with Sustainable Antibiotic Delivery. J. Biomed. Mater. Res. 59 (3), 438–449. doi:10.1002/jbm.1260
Mirani, B., Pagan, E., Currie, B., Siddiqui, M. A., Hosseinzadeh, R., Mostafalu, P., et al. (2017). An Advanced Multifunctional Hydrogel-Based Dressing for Wound Monitoring and Drug Delivery. Adv. Healthc. Mater. 6 (19), 1700718. doi:10.1002/adhm.201700718
Mndlovu, H., du Toit, L. C., Kumar, P., Marimuthu, T., Kondiah, P. P. D., Choonara, Y. E., et al. (2019). Development of a Fluid-Absorptive Alginate-Chitosan Bioplatform for Potential Application as a Wound Dressing. Carbohydr. Polym. 222, 114988. doi:10.1016/j.carbpol.2019.114988
Mondal, S., Das, S., and Nandi, A. K. (2020). A Review on Recent Advances in Polymer and Peptide Hydrogels. Soft Matter 16 (6), 1404–1454. doi:10.1039/c9sm02127b
Mostafalu, P., Amugothu, S., Tamayol, A., Bagherifard, S., Akbari, M., Dokmeci, M. R., Khademhosseini, A., and Sonkusale, S. (2015). “October. Smart Flexible Wound Dressing with Wireless Drug Delivery,” in 2015 IEEE biomedical circuits and systems conference (BioCAS) (Piscataway: IEEE), 1–4.
Moura, L. I. F., Dias, A. M. A., Carvalho, E., and de Sousa, H. C. (2013). Recent Advances on the Development of Wound Dressings for Diabetic Foot Ulcer Treatment-A Review. Acta Biomater. 9 (7), 7093–7114. doi:10.1016/j.actbio.2013.03.033
Nam, S. Y., Nho, Y. C., Hong, S. H., Chae, G. T., Jang, H. S., Suh, T. S., et al. (2004). Evaluations of Poly(vinyl Alcohol)/alginate Hydrogels Cross-Linked by γ-ray Irradiation Technique. Macromol. Res. 12 (2), 219–224. doi:10.1007/bf03218391
Noh, H. K., Lee, S. W., Kim, J.-M., Oh, J.-E., Kim, K.-H., Chung, C.-P., et al. (2006). Electrospinning of Chitin Nanofibers: Degradation Behavior and Cellular Response to normal Human Keratinocytes and Fibroblasts. Biomaterials 27 (21), 3934–3944. doi:10.1016/j.biomaterials.2006.03.016
Parenteau, N., Sabolinski, M., Prosky, S., Nolte, C., Oleson, M., Kriwet, K., et al. (1996). Biological and Physical Factors Influencing the Successful Engraftment of a Cultured Human Skin Substitute. Biotechnol. Bioeng. 52 (1), 3–14. doi:10.1002/(sici)1097-0290(19961005)52:1<3::aid-bit1>3.0.co;2-p
Popescu, I., Turtoi, M., Suflet, D. M., Dinu, M. V., Darie-Nita, R. N., Anghelache, M., et al. (2021). Alginate/poloxamer Hydrogel Obtained by Thiol-Acrylate Photopolymerization for the Alleviation of the Inflammatory Response of Human Keratinocytes. Int. J. Biol. Macromolecules 180, 418–431. doi:10.1016/j.ijbiomac.2021.03.082
Price, R. D., Berry, M. G., and Navsaria, H. A. (2007). Hyaluronic Acid: the Scientific and Clinical Evidence. J. Plast. Reconstr. Aesthet. Surg. 60 (10), 1110–1119. doi:10.1016/j.bjps.2007.03.005
Qing, X., He, G., Liu, Z., Yin, Y., Cai, W., Fan, L., et al. (2021). Preparation and Properties of Polyvinyl alcohol/N-Succinyl Chitosan/lincomycin Composite Antibacterial Hydrogels for Wound Dressing. Carbohydr. Polym. 261, 117875. doi:10.1016/j.carbpol.2021.117875
Qu, J., Zhao, X., Liang, Y., Zhang, T., Ma, P. X., and Guo, B. (2018). Antibacterial Adhesive Injectable Hydrogels with Rapid Self-Healing, Extensibility and Compressibility as Wound Dressing for Joints Skin Wound Healing. Biomaterials 183, 185–199. doi:10.1016/j.biomaterials.2018.08.044
Saarai, A., Sedlacek, T., Kasparkova, V., Kitano, T., and Saha, P. (2012). On the Characterization of Sodium Alginate/gelatine-Based Hydrogels for Wound Dressing. J. Appl. Polym. Sci. 126 (S1), E79–E88. doi:10.1002/app.36590
Silva, R., Singh, R., Sarker, B., Papageorgiou, D. G., Juhasz-Bortuzzo, J. A., Roether, J. A., et al. (2018). Hydrogel Matrices Based on Elastin and Alginate for Tissue Engineering Applications. Int. J. Biol. macromolecules 114, 614–625. doi:10.1016/j.ijbiomac.2018.03.091
Simões, D., Miguel, S. P., and Correia, I. J. (2018). Biofunctionalization of Electrospun Poly(caprolactone) Fibers with Maillard Reaction Products for Wound Dressing Applications. Reactive Funct. Polym. 131, 191–202. doi:10.1016/j.reactfunctpolym.2018.07.021
Sood, A., Granick, M. S., and Tomaselli, N. L. (2014). Wound Dressings and Comparative Effectiveness Data. Adv. Wound Care 3 (8), 511–529. doi:10.1089/wound.2012.0401
Stern, D., and Cui, H. (2019). Crafting Polymeric and Peptidic Hydrogels for Improved Wound Healing. Adv. Healthc. Mater. 8 (9), 1900104. doi:10.1002/adhm.201900104
Stewart, J. (2002). Next-generation Products for Wound Management. Surgical Materials Testing Laboratory. Wales, UK: Worldwide wounds.
Sudarsan, S., Franklin, D. S., and Guhanathan, S. (2015). Imbibed Salts and pH-Responsive Behaviors of Sodium-Alginate-Based Eco-Friendly Biopolymeric Hydrogels-A Solventless Approach. MMAIJ 11, 24–29.
Suzuki, Y., Nishimura, Y., Tanihara, M., Suzuki, K., Nakamura, T., Shimizu, Y., et al. (1998). Evaluation of a Novel Alginate Gel Dressing: Cytotoxicity to Fibroblastsin Vitro and Foreign-Body Reaction in Pig Skinin Vivo. J. Biomed. Mater. Res. 39 (2), 317–322. doi:10.1002/(sici)1097-4636(199802)39:2<317::aid-jbm20>3.0.co;2-8
Sweeney, I. R., Miraftab, M., and Collyer, G. (2012). A Critical Review of Modern and Emerging Absorbent Dressings Used to Treat Exuding Wounds. Int. Wound J. 9 (6), 601–612. doi:10.1111/j.1742-481x.2011.00923.x
Turner, J. G., White, L. R., Estrela, P., and Leese, H. S. (2020). Hydrogel‐Forming Microneedles: Current Advancements and Future Trends. Macromol. Biosci. 21, 2000307. doi:10.1002/mabi.202000307
Wild, D., Eremenco, S., Mear, I., Martin, M., Houchin, C., Gawlicki, M., et al. (2009). Multinational Trials-Recommendations on the Translations Required, Approaches to Using the Same Language in Different Countries, and the Approaches to Support Pooling the Data: The ISPOR Patient-Reported Outcomes Translation and Linguistic Validation Good Research Practices Task Force Report. Value in health 12 (4), 430–440. doi:10.1111/j.1524-4733.2008.00471.x
Winter, G. D. (1962). Formation of the Scab and the Rate of Epithelization of Superficial Wounds in the Skin of the Young Domestic Pig. Nature 193 (4812), 293–294. doi:10.1038/193293a0
Winter, G. D. (1962). Formation of the Scab and the Rate of Epithelization of Superficial Wounds in the Skin of the Young Domestic Pig. Nature 193 (4812), 293–294. doi:10.1038/193293a0
Xu, G., Cheng, L., Zhang, Q., Sun, Y., Chen, C., Xu, H., et al. (2016). In Situ thiolated Alginate Hydrogel: Instant Formation and its Application in Hemostasis. J. Biomater. Appl. 31 (5), 721–729. doi:10.1177/0885328216661557
Yadav, P., Yadav, H., Shah, V. G., Shah, G., and Dhaka, G. (2015). Biomedical Biopolymers, Their Origin and Evolution in Biomedical Sciences: A Systematic Review. J. Clin. Diagn. Res. 9 (9), ZE21–5. doi:10.7860/JCDR/2015/13907.6565
Yang, X., Liu, W., Li, N., Wang, M., Liang, B., Ullah, I., et al. (2017). Design and Development of Polysaccharide Hemostatic Materials and Their Hemostatic Mechanism. Biomater. Sci. 5 (12), 2357–2368. doi:10.1039/c7bm00554g
Yu, H. C., Zhang, H., Ren, K., Ying, Z., Zhu, F., Qian, J., et al. (2018). Ultrathin κ-Carrageenan/Chitosan Hydrogel Films with High Toughness and Antiadhesion Property. ACS Appl. Mater. Inter. 10 (10), 9002–9009. doi:10.1021/acsami.7b18343
Yu, J., Du, K. T., Fang, Q., Gu, Y., Mihardja, S. S., Sievers, R. E., et al. (2010). The Use of Human Mesenchymal Stem Cells Encapsulated in RGD Modified Alginate Microspheres in the Repair of Myocardial Infarction in the Rat. Biomaterials 31 (27), 7012–7020. doi:10.1016/j.biomaterials.2010.05.078
Zhai, M., Yoshii, F., Kume, T., and Hashim, K. (2002). Syntheses of PVA/starch Grafted Hydrogels by Irradiation. Carbohydr. Polym. 50 (3), 295–303. doi:10.1016/s0144-8617(02)00031-0
Keywords: smart, wound management, wound healing, functionalization, polysaccharide, biomaterial
Citation: Kibungu C, Kondiah PPD, Kumar P and Choonara YE (2021) This Review Recent Advances in Chitosan and Alginate‐Based Hydrogels for Wound Healing Application. Front. Mater. 8:681960. doi: 10.3389/fmats.2021.681960
Received: 17 March 2021; Accepted: 15 July 2021;
Published: 28 July 2021.
Edited by:
Yanju Liu, Harbin Institute of Technology, ChinaReviewed by:
Haibao Lu, Harbin Institute of Technology, ChinaYilong Cheng, Xi’an Jiaotong University, China
Copyright © 2021 Kibungu, Kondiah, Kumar and Choonara. This is an open-access article distributed under the terms of the Creative Commons Attribution License (CC BY). The use, distribution or reproduction in other forums is permitted, provided the original author(s) and the copyright owner(s) are credited and that the original publication in this journal is cited, in accordance with accepted academic practice. No use, distribution or reproduction is permitted which does not comply with these terms.
*Correspondence: Yahya E. Choonara, WWFoeWEuQ2hvb25hcmFAd2l0cy5hYy56YQ==