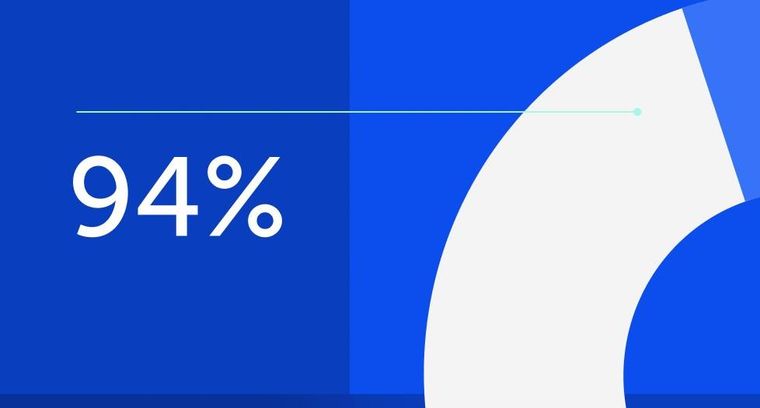
94% of researchers rate our articles as excellent or good
Learn more about the work of our research integrity team to safeguard the quality of each article we publish.
Find out more
REVIEW article
Front. Mater., 11 June 2021
Sec. Mechanics of Materials
Volume 8 - 2021 | https://doi.org/10.3389/fmats.2021.649456
This article is part of the Research Topic2021 Retrospective: Mechanics of MaterialsView all 9 articles
Biomaterials are evolving quite rapidly over the last decade. Many applications have been considered toward their involvement in saving lives in the line of duty for law enforcement agencies and military operations. This article discusses recent work on the role of biomaterials that can be considered as a competitive alternative to composites, being used against ballistic impacts. The fish-scaled biomaterials are focused on in this paper, highlighting their excellent mechanical properties and structural configurations. In its natural environment, the scale provides fishes with an armor plating, which is significantly effective in their survival against attacks of predator and the impact inflicted from sharp teeth. These bioinspired materials, if engineered properly, can provide an excellent alternative to current Kevlar® type armors, which are significantly heavier and can cause fatigue to the human body over long-term usage. The investigated materials can provide effective alternatives to heavier and expensive materials currently used in different industrial applications. Additionally, some recent development in the usage of fish scales as a biomaterial and its applications in rapid prototyping techniques are presented. Finally, this review provides useful information to researchers in developing and processing cost-effective biomaterials.
Since the beginning of time, humans have encountered many conflicts and wars, which could be at a domestic level or even at a national level. These kinds of confrontations have led to many fatalities in past and therefore put forwarded an idea to protect ourselves from various types of weapons that have been used in such instances. For example, the invention and usage of body armor protection for the chest and the head are vital as they being the necessary vital organs. Interestingly, as the armor technology has evolved over time, so is the firepower and penetration power of ammunitions that have been developed to pierce through them. Formerly, these armors used to be a single solid thick metallic stiff plate though quite heavy but effective in deflecting any kind of impact. However, as the material technologies advanced, armor plating became flexible and much lighter so that the personnel can be relieved of some bodily fatigue and blunt force trauma.
In the twenty-first century, Kevlar® (DuPont, Wilmington, DE, United States1) emerged as a specially designed composite material for armor plating with very good strength and impact resistance against small firearms. Moreover, it offers considerably lower weight than the traditional armor plating primarily used for the same scenarios. However, it is also understood that the properties of Kevlar are not sufficient enough to tackle and counter bullets of large caliber. Although Kevlar® offers excellent bulletproof properties, nevertheless it leaves behind trauma marks on the body due to the forces dispersed from the momentum of the bullet upon impact, which can be very uncomfortable to experience (Stopforth and Adali, 2018).
Bioinspired materials are under constant research to produce new and innovative protective body armors by carefully looking into scale configurations for effective protection and modes of energy dissipation from projectiles. Nevertheless, there always is a risk of energy leakage, which is dominantly presented at the joints or boundaries of the scales, for example when considering fishes. Studies are being done in this area so that the armors can absorb most of the energy from the bullet or projectile instead of just crushing the bullet and leaking that energy toward the human body, which can cause some traumatic injuries leading to an uncomfortable and painful situation. In this quest, Lin (2017) proposed simple protecto-flexible gloves designed from detailed research and simulation of Carp scale. A more recent study has presented the idea of using ultra high molecular weight polyethylene (UHMWPE) and Ceramic layered round overlapping scales with a supporting base of Kevlar® to disperse energy from a bullet by undergoing a fracture of ceramic layer and allowed for a dampening effect upon impact to drastically diminish the kinetic energy of the bullet through UHMWPE and Kevlar® in coordination. Additionally, according to the National Institute of Justice standard level III (NIJ-III), the new body armor design is proposed to have a ballistic limit of around 1,100 m/s, which is more than NIJ-III ballistic rating of 838 m/s (Zhang et al., 2020). Correspondingly, ceramic plates mimicked from fish scales have been proposed to have 10 times the puncture resistance than soft elasmoid type scales (Ha and Lu, 2020).
At present, the armor development is still undergoing extensive research to favor penetration resistance as the flexibility and mobility of the wearer are taken into consideration for such applications (White and Vernerey, 2018). It should also be noted that soft body armors have no match for a high caliber round for which only hard plate armors are used, known as level III and level IV armors. In addition, glass–rubber application against sharp high-speed projectiles has proven to be very effective provided that the upper layer is segmented in a hexagonal glass formation with a lower soft rubber substrate facilitating an effective reduction in flexural stresses and providing a multihit capability with 70% increase in failure resistance as compared to a thick slab of glass with a similar rubber substrate (Chintapalli et al., 2014). Nevertheless, not all types of fish possess armor like properties, and it solely depends on factors like their size, mobility, and agility of the fish species itself and the natural configuration of each scale, which could lead to an experimental design where we can observe the varying density of mineralization, strain–stiffening effect, and good bending response (Vernerey and Barthelat, 2010).
This review paper provides a compilation of fish scales and their respective mechanical properties for biomimicry applications, specifically to provide protection as body armor. The history of the design of fish-scaled armor provides us the principles of their unique design (Ehrlich, 2015). Although the field of research is quite new, there has been a couple of breakthroughs with the respective problem in making the armors lighter and thinner while still retaining and relying on the bulletproof attributes of a Kevlar® vest.
The presence of naturally occurring armors dates back to the Dinosaur age as paleontological studies have presented data about the type of epidermis the fossils used to have. After the evolutionary processes of living organisms, there are still traits present in some mammals and fishes that possess a naturally occurring body armor, which can demonstrate excellent mechanical properties like Young’s modulus. Arapaima, for instance, a bioinspired material, has gained a lot of attention for its numerous applications in the medical industry, where different sorts of problems have been rectified using inspiration and imagination from the living species around. Chen et al. (2012) performed and validated the scale structure of Arapaima gigas (Figure 1) and alligator gar fishes to better understand the mechanical properties through nanoindentation tests, which showed great resistive properties against predators like piranhas. Nonetheless, moving toward the armor applications from biomaterials, fish scales, and shrimp bodies have been a major focal point in developing an impact-resistant material.
Figure 1. Dermal armor for Arapaima gigas (Chen et al., 2012).
There are a handful number of research articles available on fish scales primarily focusing on the key parameters like the scales, the structure, placement, and symmetry of each scale to the next, performance under impact loading, etc. It is a known fact that fish scales are quite hard in reality and serve as naturally occurring armor to prevent attacks from a predator whose teeth would deliver a significant amount of impact as it strikes and plunges onto its prey for appetite (Yao et al., 2015). A. gigas, for instance, shows an overall Young’s modulus of their dermal region to be about 1.2 GPa, given they have a two-region dermal layer in which has a bony layer on top for protection and a soft collagen thick layer in the bottom for flexibility (Yang et al., 2013) as shown in Figure 2. It is also claimed that low-speed projectiles with a point load can provide promising results in favor of penetration resistance, but it would be quite uncertain to claim the same for ballistics (Yao et al., 2015).
Figure 2. Bioinspired bulletproof vest inspired from scales of fish (Yang et al., 2013).
Vernerey and Barthelat (2014) presented another species of fish called teleost, which can demonstrate good mechanical performance in terms of different kinds of loadings. However, since their scales are not as strong as A. gigas, it has been given an insight into the development of thin flexible armor that can provide protection against knives and sharp objects (including shrapnel) to be considered a fair application for the scales found on the body of teleost fish, which claims to offer a decent level of results despite the fact that individual scales can fracture under extreme impact loadings. However, to further improve the defensive mechanisms in armor applications, strategies like sacrificial deformation could be adapted in the microstructure to dissipate energy without allowing penetration of the projectile (Song et al., 2011).
There are many other species of fish in the ocean, which possess credible mechanical properties that are required for body armor applications. For example, boxfish offers a hexagonal structure as shown in Figure 3. Through visual inspection, it can be noticed that they are arranged in a symmetry, and from this symmetry, the borders and joining points of each scale are considered to provide an energy dissipation method, which actually hinders the load propagation, consequently reducing chances of catastrophic damage.
Figure 3. Boxfish (Lactoria cornuta) scale structures (Miranda et al., 2019).
The Cyprinus carpio has attracted an extensive scale mechanical properties study. However, results demonstrated a fairly weaker scale structure and stress–strain endurance whether in hydrated or dehydrated conditions and can reach almost as half of what can be achieved and observed from a scale of A. gigas (Garrano et al., 2012).
Elasmoid fishes are largely known to have great toughness of their scales that present naturally occurring composite having three layers. These layers consist of an outer limiting layer (hard inorganic and highly mineralized), a middle layer consisting of a softer organic yet tough layer of collagen fibers, and finally the last layer of thin and orthogonal collagen fiber (Murcia et al., 2017). Considering a scale from a grass carp, the structure of the scale is found to have a double-twisted plywood-like structure with each layer alternating between rich and poor collagen fibers longitudinally. Through observation of a sample under SEM, the eight layers of lamellae (Figure 4) show the orientation of each fiber in each of the eight regions in red color (Feng et al., 2020).
Figure 4. Orientation of collagen fibers (in red) in eight-layered lamellae of grass carp under SEM (Feng et al., 2020).
Scales harvested from elasmoid fish like C. carpio and Megalops atlanticus commonly knowns as freshwater carp and tarpon, respectively, show an extensive yet prominent three layers of a bony limiting layer and two orthogonal elasmodine layers (external and internal) as an SEM imaging (Figure 5) at 100 μm depicts (Arola et al., 2018).
Figure 5. SEM cross-section image of an elasmoid fish scale (tarpon) (Arola et al., 2018).
Moreover, Grass Carp scales were also investigated in terms of their structure and mechanical properties to be used for probable biomimetic protection applications. Those scales were found to have a two-layer structure, which displayed better mechanical properties at dry conditions rather than in wet conditions. This was found primarily after conducting several tensile tests on whole scale samples containing both the layers and analyzing results in uniaxial and biaxial loading scenarios (Liu et al., 2017).
Considering the structural and mechanical properties of the elasmoid fish scale altogether from common carp species like A. gigas displays an exceptional result when subjected to longitudinal and transverse loadings, and due to a single-twisted Bouligand structure with each layer having a unique angular orientation gives us the following properties as shown in Table 1 (Quan et al., 2020).
Table 1. Uniaxial tensile test results of a scale from Arapaima gigas (Quan et al., 2020).
The scales from A. gigas displays the reason behind a protective characteristic against tooth bites of a piranha because of the lamellar structure of collagen fibers within the scales. These fibers of collagen demonstrate a sliding behavior as the tooth penetrates, which also provides lamellar sliding resulting in tension of the soft fibers and compression of the hard-bony shells (limiting layer), resulting in tooth fracture of hampering (Yang et al., 2014). Similarly, the puncture resistant property in scales of carp and arapaima are found to be advantageous against physical threats, which can resist failure at even strain rate of puncture loadings, while being close to the head region in the fish (Ghods et al., 2018). Dimensionally, arapaima scale thickness range approximately 1,600 μm, constituting 600 μm of its limiting layer and the remaining 1,000 μm of elasmoid region containing an orthogonal arrangement of fibers in stacks ranging between 30 and 60 μm in ply thickness with each fiber of lamella of 1 μm of diameter and each small fibril of approximately 100 nm in diameter or even smaller (Estrada et al., 2020). The phenomenon when these collagen fibers undergo tensile loading has previously been described to slide and reorient in a different direction, and this reorientation mechanism was studied using the X-ray diffraction method where the fibers tend to straighten themselves in the axis of tensile stress because of each individual fibril slides and stretches in the direction of tension (Zimmerman et al., 2013) and enabling them to undergo excessive strains without failure (Sherman et al., 2017). Upon further investigations, it was found that the upper limiting layer of the arapaima scales consisted of high mineralization of calcium content, which are present in voids (Bezerra et al., 2019) and hydroxyapatite (HA), which makes the limiting layer strong, hard, and tough, determined using energy-dispersive X-ray spectroscopy (Bezerra et al., 2020). Nevertheless, Bouligand structures can also undergo fracture, but it is resisted due to being in a twisted manner that propagates crack along the twisted path but greatly resists fractures as found numerically by Suksangpanya et al. (2017).
In addition, dermal armor of boxfish, which is also known to have a protective property against predators. Their structural configuration found to be hollow honeycomb-like pattern as shown in Figure 6. These are also composed of collagen fibers but not as strong as the structural arrangement found in other fish scales (Garner et al., 2020). The very reason for its low-strength armor capabilities as compared to other fish scales lies within the fact that these scales do not overlap and are only in a single-layer configuration, but due to high mineralization within the scales, they tend to exhibit some protection and flexibility of some consideration (Yang et al., 2015).
Figure 6. Boxfish scale geometry and structure in high magnification (Garner et al., 2020).
Teleost fish scales, similar to those in A. gigas, also display a great toughness due to being highly rich with collagen fibers. Though having a cross-ply layer of stacked collagen fibers reinforced with HA (Dastjerdi and Barthelat, 2015) rather than in a twisted plywood configuration as found in A. gigas. the presence of collagen is quite prominent in every type of fish scale whether it provides excellent protecto-flexible property or adequate enough for some optimization and consideration. Interestingly, the reason collagen fibers display such an exquisite mechanical property is due to the fact of their own structure, which at a molecular level, is constructed in a triple helix manner for each fibril (Sherman et al., 2015), and this is what provides different favorable properties that vary on mineralization and hydration property in each fish scale as Table 2 displays a summary for each type of fish scale.
As far as the simulative study of biomaterials are concerned, their rapid prototyping (RP) can ensure a promising application in the industrial sector and in military applications. However, the availability of materials for rapid prototyping by modern means can pose additional challenges.
The most recent type of work being performed in order to manufacture biomaterials proved to be a very ingenious method, utilizing the ultrasound direct-write printing technique (Ultrasound-DW) to tailor the microstructures relevant to desired mechanical and material properties. It was noticed that the factors in the Ultrasound-DW setup like frequency of operation and printing speed determined the overall manufacturing properties (Wadsworth et al., 2019). Similarly, in terms of the medical field, the RP techniques being used recently are at a whole new level, especially taking into consideration of 3D printing technologies, which mainly include PolyJet multimaterial printing2 and two-photon polymerization (Meyers et al., 2018).
Additionally, there are other manufacturing perspectives of bioinspired materials, which can ensure very authentic outcomes upon the implementation of modified RP techniques that can benefit greatly if usage of liquid-based, solid-based, or powder-based feed materials are considered by which there is a very high probability of achieving exceptional quality macrostructures in the case of bioprinters (Touri et al., 2019). Moreover, fused deposition modeling (FDM) should be practiced to print composite like bioinspired materials as that is the quickest form of 3D printing, with proper printing parameters like nozzle size and nozzle temperature taken into account (Ko et al., 2019). To add more, there exists a wide gap between the industry and the research in order to develop a fine-tune method and biomaterial optimization if we talk about 3D printing as the best possible technique to provide benefit to the different industrial sectors like energy, medicine, automotive, environment, and military (Poomathi et al., 2020). However, during recent times, it has been observed that the fabrication of biomaterials is also possible by utilizing all the rapid prototyping techniques like 3D printing, mineralization, freeze-casting, laser engraving, and coating-assembly. For instance, collagen material, which is an abundant fundamental material in fish scales, can be printed using HA inks easily, but their availability is a concern in some third world countries (Gu et al., 2016). Upon the fabrication and manufacturing of potential biomaterials, the ones to be used in the desired industry have shown the need to focus on mechanical properties at micro and nano scales to improve the toughness and the deformation mechanics for a better quality of synthesis (Mirkhalaf and Zreiqat, 2020). In further applications of these biomimetic techniques, ceramic materials of excellent mechanical properties can be incorporated into a very flexible base to be formed for gloves, which when undergoing stretch, the overlapped optimized and synthetic scales made of ceramics will be providing adequate protection and flexure of finger and palm (Martini and Barthelat, 2016a).
As the RP methods in the development and evolution of biomaterials are being carried at a whole new level with exceptional quality of technological advancements, it has been observed that their usage in the present time is widely dispersed over the medical field. Since, grafts of bones and skin are being flawlessly made using biomimicry and materials that represent a better phase of mechanical properties when subjected to a specific loading and fixation point. Although the military use in the sector of defense against ballistics is also being studied at great depth by the application of RP; nevertheless, there is still a need for specialty material in order to stand alongside the materials that are light in weight and have immense toughness. Consequently, the possibilities also emerge to look into RP of different types of scale geometry, which can be mimicked to mechanical destructive testing while inspirationally adding modifications not possible in nature (Porter et al., 2017). On a different perspective, given that arapaima scales can be further strengthened at a molecular bonding level, providing new techniques in the development of flexible armors, an organic solvent like ethanol proved to be very effective in strength increment and puncture resistance for low mineralized scales, which are usually present and obtained from the lower middle to tail region of these fishes (Arola et al., 2019). These strengthening mechanism works on the collagen fibrils as hydrogen bonding begins to form among the solvents that could be methanol, ethanol, and acetone, providing good and enhanced mechanical properties (Jiang et al., 2020). To add, fiber-reinforced composites (FRCs) can also be considered as a very effective way to construct and mimic the collagenous formation in a material, as FRCs provide favorable mechanical properties and also are quite popular (Yaraghi and Kisailus, 2018).
Finite element modeling and analyses performed for different types of fish scales have provided interesting data concerning their failure mechanism and crack propagation. Lightweight fish like P. senegalus of considerably small size with thin outer layer for being and big heavy fish with a thick outer layer like A. spatula and A. gigas depicted contrasting results when a quasi-static indentation tests were numerically realized (Chandler et al., 2014). This presents a considerable potential in designing a new bulletproof vest by using fish scales. However, before commercially undertaking the project, to use them on a vast scale in the bulletproof vest technology, a vast experimental campaign needs to be executed to validate these results. It would otherwise be unsafe to claim the effectiveness of the design approach.
Investigations on marine laminates of five different materials show the damage penetration in the respective thickness of the materials. If the laminates were very thick, the face would undergo bending, which will delaminate the soft substrate layer and eventually giving up to penetration (Sutherland and Soares, 2006). Therefore, a resistance to impact forces can be accompanied with a laminate of Kevlar® along with another marine type composite to be applicable for ballistic purposes and used only for small caliber ammunition.
Miranda et al. (2019) performed a numerical simulation using Abaqus® (Dassault Systemes, France3) as the finite element modeling software to carry out different impact testing scenarios with changing scale dimensions as compared to the dimensions of the projectile.
Inspired by boxfish scale structure (Figure 3), a numerical simulation was performed (Miranda et al., 2019). Miranda et al. used a projectile of a diameter that would be as big as a single scale. However, these experiments were conducted on varying scale sizes, shapes, and configurations to optimize the design for the best protection, reduction in kinetic energy, energy absorption. and impact resistance. Figure 7 illustrates a variety of configurations toward impact loading using a 20-mm diameter projectile. For the tested case scenario, both the front and back surfaces of load distribution were investigated to figure out the extent of force and energy transferred from the front (impact side) of the sample to its backside if different configurations like square, hexagonal, rhombic, and circular arrangements. The projectile was shot at the center and off-center for each configuration to observe how and what reaction will each configuration and impact positioning offer. Consequently, the findings were that it would be better to use a projectile as big as the scale because it implied that the forces would be transferred to the scales adjacent to the targeted ones, especially in a hexagonal configuration. Also, if scales were smaller than the projectile itself, the boundaries could be a potential risk to leak some of the kinetic and impact energy toward the body. However, in the boundaries, there was a risk of some of the kinetic energy being leaked through the sample, which posed quite a concern that it would be the weak point of the whole structure, and it will not be able to hold after a couple of impacts at the boundary points.
Figure 7. Impact of projectile on segmented composite armor (A) square, (B) hexagonal, (C) rhombic, and (D) circular geometries to observe force distribution when the impact is on center of scale (on left column) and when off-center (on the right column) (Miranda et al., 2019).
As scales are housed upon a soft substrate, it provides flexibility to the body and allows some dampening effect. In this perspective, mechanical tests were performed on scales mounted on a soft elastic substrate. The scales were covered with four different types of soft-layered covers, and in some conditions, grit was also used in the soft layer of cover on top of the scales to examine the probability of impact force deflection if a blunt projectile or object is targeted on it (White et al., 2019). It makes a lot of sense to add soft layers of covers with grit particles suspended inside, since it will provide a significant amount of dampening if subject to a blunt projectile. As the purpose of bulletproof vests is to reduce the number of forces and energy travel toward the human torso that can cause mild injuries, which is very disturbing, considering the scale of momentum being transferred from a small bullet at a very high speed toward the human body, which will first be experienced by the bulletproof vest and subsequently be transferred to the human body after whatever remains of the energy. Additionally, using bio-based epoxy laminates could be a potential source of additive manufacturing to obtain excellent flexure properties and a good amount of impact energy absorption (Scarponi et al., 2016). Since the joints like shoulders, elbows, and knees require constant and undisturbed movement, protection with thick armor in these areas could be problematic as it can interfere with movement in a combat scenario. Hence, incorporating these bio-based flexible epoxy laminates with scales embedded in them could be a very good feature and improvement to develop and produce armor for the joints by providing ease of mobility.
According to Yang and Quan (2019), fish species known as Arapaima have scales, which possess excellent mechanical properties as they are known to be found in the Amazon River, these fishes protect themselves from piranha bites, which has a brutal force to even tear off human flesh into pieces. Therefore, for close combat situations where combat knives and small caliber firearms are suspected, these materials could be considered as one of the choice materials for the bulletproof vest technology, and they can be processed through various techniques while keeping in focus the principle of elasmoid scales, which acts strong and flexible due to a soft layer beneath the hard scales to provide ease of mobility. Torres et al. (2015) tested A. gigas scales through different types of impact loadings to determine their true values of elastic modulus as it is known to have a deviation when hydrated and dry. It was found that in conditions where impact tests were conducted at room temperature and cryogenic conditions clearly indicated a full fracture at the latter condition and did not undergo full fracture in room temperature, i.e., these scales were dry. Moreover, in case of hydrated conditions, the Young’s modulus was lower in the scales than in dry conditions, i.e., 1.2 GPa instead of 0.1 GPa after performing a series of tensile tests (Lin et al., 2011).
Bruet et al. (2008) have cited historical aspect of fish and their scales in their research about fish scales and associated mechanical properties. They found that a species, which goes by the biological name of Polypterus senegalus bear a very tough and outer scale on its body. Owing to extensive finite element analysis (FEA) and experimentations, it was concluded that they possess some outstanding mechanical properties that are maximum at the outermost hard scale and decrease gradually as we move further in toward the body, all the way to the bones showing a very good amount of stress values that it can endure. These findings show their potential candidacy for body armor applications.
Synthesis and manufacturing of these scales for experimental testing are very essential to gather authentic experimental data. Thanks to the technological advancement in manufacturing, like additive manufacturing techniques that are capable of producing parts using composites and hybrid materials that can possess similar properties as metals but can be lower in weight and material handling. Fish scale materials and specimenfor impact tests to study their resistance against ballistic projectiles can be manufactured. This is how biomimicry could help validate all the numerical data being recorded via finite element modeling and simulations (Le et al., 2019). Martini et al. (2017) have looked into this very technology of 3D printing bioinspired scales from alligators, fishes, and armadillos to understand and study the effectiveness of each type of scale, scale orientation, and geometry. They conducted the experiments using 3D printed ABS photopolymer on a polyurethane substrate in isolated and arrayed scale arrangements to observe the behavior individually, and the scales were of rectangular and slanted geometry, and upon experimentation, it depicted that slanted orientation of the scales was much effective in providing scale-to-scale interaction and stability to the overall network of scales, which also deflects the projectile away from the target, as shown in Figure 8. For further experimentations on flexibility and puncture resistivity aspects, it was extremely important to preserve these properties in an array of scale for which topologically interlocked scale geometry and configuration was preferred as it provided 10 times the puncture resistance with very minor sacrifice in flexibility, which is still considered to be 18 times beneficial than a simple rectangular array of scales. Additionally, upon modeling different scales at different slant angles and aspect ratio, the gap between each scale is also a crucial parameter, as too much of a slant angle can potentially increase contact points under concentrated loading. Consequently, the scale would raise the puncture resistance but at the cost of impaired flexibility (Shafiei et al., 2020) as illustrated in Figure 9.
Figure 8. (A) Isolated rectangular scale, (B) simple array of rectangular scales, and (C) simple array of slanted scales (Martini et al., 2017).
Figure 9. A detailed illustration of scale slant angle, aspect ratio, and scale thickness for optimized flexibility and puncture resistance (Shafiei et al., 2020).
Moreover, in the last decade, there has been a breakthrough toward the application of body armor inspired by fish scales in a research funded by The United States Army where an extremely flexible and light-weight body armor was given preference for the development (Rudykh and Boyce, 2014). These armor applications were applied after considering the laminations of the scales and their arrangement to obtain super flexible structure without compromising protective properties of the elasmoid composite (Rudykh and Boyce, 2014). For such an inspiration, fish scales were used and 3D printed using a very complex and unique sort of hybrid polymer that persisted an elasmoid type attributes and also provided exceptional ballistic protection from firearms (Rudykh et al., 2015). The scale orientation in these experiments was also considered. In a three-point bending test, the scales demonstrated exceptional resistance against a 5-mm radius pointed cylinder. For the variations in inclination angles of the scales with respect to the volume fractions, interesting results were witnessed as illustrated in Figure 10. Moreover, upon examining the substrates with overlapping scale configurations, there is always a limit toward the non-idealistic geometry. This happens when the scales are present on only one side of the substrate, and the scale engagement can cause a huge difference in the displacement of the whole sample when subjected to bending in contrasting directions (Ghosh et al., 2017).
Figure 10. Deformation illustration on the laminates of scales at increasing inclination angles (top to bottom) with decreased volume fraction and increased indentation depth (left to right) (c: volume fraction) (Rudykh et al., 2015).
These results being gathered by Rudykh and his team present the different aspect of inclination came to a verdict about 30° of angle being an optimal configuration of the setup to be used to have a greater penetration resistance and flexibility that was very impressive considering the protecto-flexibility required by the new kind of light-weight and extremely effective vests for the future. Moreover, a similar design pattern was studied numerically by Liu et al. (2016). It was a more focused study toward the shape and geometry of a scale intended for the ballistic impact testing. Initially, a hexagonal shape of scales was considered, but they were then finally considered to be circularly overlapped with each other of some specific thickness. The results produced from the impact tests showed that the scales would puncture or fail if a projectile traveled at 878 m/s. Silicon carbide circular scales were prepared with an aluminum substrate held together by Kevlar® showed quite impressive results. Due to the increased hardness of the scale and an overlapped configuration, it was determined that the projectile will just transfer the kinetic energy to the adjacent scales and may just deform the scale plastically instead of puncturing through it. Similarly, upon further investigations, it was found that elasmoid fishes possess some really intriguing properties, especially the species like teleost, which have an overlapping scale configuration, naturally making it a very credible candidate for armor applications as the numerical results can demonstrate the capability of withstanding larger values of puncture loads of teleost fish scales (Vernerey et al., 2014). Furthermore, the protection and flexibility of armor were determined by the scale overlapping and the volume of material used which should be effective enough to provide resistance against ballistic impacts as results from numerical simulations shown in Figure 11 (Browning et al., 2012).
Figure 11. Contours of stress and strain taken at 5 N/mm force over three configurations of scale overlapping and scale volume (Browning et al., 2012).
It could be very illustrative and clear that the biomimicry being adapted in body armor applications has a lot to offer but with specially designed materials for additive manufacturing. Since, these materials are not available readily and need additional development techniques to incorporate properties to sustain ballistic impacts lighter in weight than conventional Kevlar® type vests.
Upon further examining the structure and mechanical performance of the modern fish scale of striped bass, the scales were studied further for penetration of a sharp projectile if subjected to impact on their hard-outer bony layer supported by a softer cross-ply of collagen fibrils (Zhu et al., 2012). The results generated from these experiments illustrated a puncture and penetration of the projectile in two stages, i.e., the first stage consists of bony layer cracking in fourfolds which into the second layer of collagen layer is resisted very effectively due to the bilateral tension produced in the collagen layer, which requires the projectile to have 50% more penetration force to completely penetrate through all the scales.
Zhu et al. (2013) conducted further real-life experimentations with improved scale overlapping in different configurations namely stacked, staggered, and rotated. They summarized that if the scales are stacked upon each other, it would present a much greater penetration resistance, and with the added mineralized collagen fibrils, it would be even tougher than the previous tests conducted as shown in Figure 12. Since indenters/projectiles are designed for considerable damage and penetration, therefore, if they could be coated with a material to prevent the catastrophic fracture failure, these biomaterials can be ensured to withstand much higher penetration indenters (Yao et al., 2015). Comparably, a sample of teleost fish was investigated for a similar purpose of its flexure as a whole, and it proves that having an external tendon on its skin provides locomotion and flexibility that subsequently has also no major effect on stiffness reduction when descaled (Szewciw et al., 2017).
Figure 12. (A) Scale arrangements used for tests: stacked, staggered (natural) overlap, and rotated, (B) force displacement curves for the three scale arrangements, and (C) “sink-in” mechanism of scale and substrate deformation (Zhu et al., 2013).
From the illustrated SEM imaging in part (C) of Figure 13, it can be observed that the outer bony layer was completely punctured, whereas the softer layer of collagen holding the bony structure together experienced only a slight penetration and resisted from puncturing throughout the whole protective layer. Where part (A) and part (B) are illustrates a graphical and visual perspective, respectively, of the projectile penetration in three stages of deformation. Part (B) provides a side view of the scale and the projectile and the depth with of deformation in each gradual succession. Similarly, when discussing arapaima fish being attacked by piranhas, their fierce and forceful bites fail to penetrate completely toward the body of fish and the teeth suffer from fracture before achieving complete penetration (Meyers et al., 2012). Vernerey and Barthelat (2010) also support the fact about fish scales to be of greater penetration resistance when the scale density is increased, i.e., the strain–stiffening property is greatly enhanced. This could imply the fact that it would be quite challenging for a bullet or a sharp projectile carrying speed and energy, if it were to collide with a specially designed scale armor to completely penetrate. The reason also lies within the microstructures of these scales as they contain the collagen fibrils in a laminated form, and each of the fibers is co-aligned in each layer, which is stacked at about a 90° rotation in each layer. These scales are then further reinforced with HA nanocrystals, which explains their excellent mechanical properties (Torres et al., 2008). However, when considering a soft substrate with a hard plate or scale on the outer layer, concerns arise for the stability of scale under impact loading. This can be tackled successfully by designing the scale of a considerable size while having greater friction between the substrate and the scale, which could potentially provide a much sturdy condition to resist puncture and sustain flexibility (Martini and Barthelat, 2016b).
Figure 13. Detailed illustration of puncture from the sharp projectile into the scale with a soft substrate (Zhu et al., 2012).
Considering other options to deflect energy and resist penetration, there are some other structural designs to incorporate into the vest as a dampening mechanism and penetration-resistant mechanism for the ballistic projectiles. A honeycomb-like sandwich structure, as shown in Figure 14, containing scales on either surface of the honeycomb structure could provide excellent energy absorption and penetration resistance on highly exposed flat areas, like the chest, which would be light weight and flexible as a similar technology is being considered for military vehicle and bunker purposes for protection against improvised explosive devices (IEDs) and bombs (Qi et al., 2017). However, considering armor-piercing incendiary 12.7 mm rounds, extreme cases of heavy armor can be studied. These could still be lighter than traditional level-II heavy armor if incorporated into hexagonal thick scale-like protective coating with epoxy is considered. Latter is often used against concrete and has displayed favorable results in reduced damage and crack propagation (Sun et al., 2015). However, this condition could be applicable only if many staggered layers of protection are used which would only make the armor bulkier yet still lighter.
Figure 14. Honeycomb-like structural sandwich for impact absorption (Qi et al., 2017).
Furthermore, looking into great depths of Bouligand-like structure of a scale from an arapaima scale, numerical simulations provide an evidence in preferring a discontinuity in fibers through the layers of collagen ply to successfully increase fracture resistance and crack insensitivity which is demonstrated in Figure 15 (Wu et al., 2020).
Figure 15. (A) Discontinuous Bouligand fibers and (B) the numerical result of energy absorption (Wu et al., 2020).
This paper presents a clear and up-to-date picture of the various types of scales that can be considered as potential candidates to develop new and improved body armors. A. gigas fish possesses remarkable mechanical properties and is found effective to counter ballistic projectile from small firearms and small caliber bullets. Since these fish contain collagen fibrils beneath their scales and are covered with an extremely dense mineralized material over the top, the scales act very effectively in providing the protection required and the flexibility needed in a combat situation. The effective armor-like properties in these scales arise due to the helicoidal or twisted cross plywood-like orientation of each lamellae of collagen fibers with the convincing distribution of forces and energy upon impact loading (Liu et al., 2020). However, teleost fish is also considered to demonstrate great mechanical properties in similar conditions where penetration resistance is favored, but they possess an anomaly in both dry and hydrated conditions of the scale while from different regions on the body of fish (Rawat et al., 2021).
Numerical simulations and experimental campaigns have been very helpful in determining the type and configuration to use for the scales of the manufacturing of a real-life armored material by use of lightweight yet tough impact resistant materials in the form of a composite. The point of interest also includes the amount of energy and momentum transferred through the bullet, which will be quite significant if the overall weight and thickness of the vest would be reduced. However, to deal with the energy absorption and dissipation, the microstructures found naturally in these scales could be a potential choice given their excellent mechanical characteristics. It is concluded that ballistic performances are not as favorable for cross-ply laminates, which mimic the Bouligand structure. Since the failure mechanism occurs in front which forms a domino of delamination letting the projectile slip through the fibers. On the other hand, when fibers are tough and brittle, they interfere with the performance of projectile and saving an object from the impact on the other side.
Similarly, considering the manufacturing and synthesis of biomaterials will be utilized later on in their respective fields of applications. The methods exist but on a wide variety of microstructure, building blocks are available and each configuration at a micro-scale level poses a challenge in obtaining important mechanical properties like toughness and deformation. Therefore, the availability of feed material for 3D printing or stereolithography is crucial. Furthermore, biomaterial processing for the medical field, energy industry, defense sector, or environmental sector is also challenging. Rapid prototyping of these materials does display a huge benefit, especially with the cost and efficiency of designing and manufacturing of the components. It is expected that the application of rapid prototyping will rise and grow at a much greater level if the raw material for 3D printers and PolyJet printers can be designed and utilized with desired mechanical properties and building block microstructures.
Each author was responsible in a certain percentage of work toward the successful completion of this research. TW was involved in gathering necessary references related to the applications of fish scales and how they were considered a potential option as an alternative biomaterial. MA and FD on the other hand, studied deeply about fish scales’ microstructures and similarly conducted studies carried out by professionals in this field where they tested the integrity and durability of their numerical and experimental models by conducting various mechanical tests. All authors contributed to the article and approved the submitted version.
The authors declare that the research was conducted in the absence of any commercial or financial relationships that could be construed as a potential conflict of interest.
Arola, D., Ghods, S., Son, C., Murcia, S., and Ossa, E. A. (2019). Interfibril hydrogen bonding improves the strain rate response of natural armour. J. R. Soc. Interface 16:20180775. doi: 10.1098/rsif.2018.0775
Arola, D., Murcia, S., Stossel, M., Pahuja, R., Linley, T., and Devraj, A. (2018). The limiting layer of fish scales: structure and properties. Acta Biomaterialia 67, 319–330. doi: 10.1016/j.actbio.2017.12.011
Bezerra, W. B., Oliveira, M. S., Filho, F. D., Demosthenes, L. C., Silva, L. C., and Monteiro, S. (2019). Characterization of arapaima fish scales and related reinforced epoxy matrix composites by XRD, EDS, and SEM. Med. Radiol. 117–124.
Bezerra, W. B. A., Monteiro, S. N., Oliveira, M. S., Demosthenes, L. C. D. C., and Costa, U. O. (2020). Processing and characterization of arapaima gigas scales and their reinforced epoxy composites. J. Materials Res. Technol. 9, 3005–3012. doi: 10.1016/j.jmrt.2020.01.051
Browning, A., Ortiz, C., and Boyce, M. C. (2012). Mechanics of composite elasmoid fish scale assemblies and their bioinspired analogues. J. Mech. Behav. Biomed. Materials 19, 75–86. doi: 10.1016/j.jmbbm.2012.11.003
Bruet, B. J. F., Song, J., Boyce, M. C., and Ortiz, C. (2008). Materials design principles of ancient fish armour. Nat. Mater 7, 748–756. doi: 10.1038/nmat2231
Chandler, M. Q., Allison, P. G., Rodriguez, R. I., Moser, R. D., and Kennedy, A. J. (2014). Finite element modelling of multi-layered structures of fish scales. J. Mech. Behav. Biomed. Materials 04, 375–389.
Chen, P. Y., Lin, Y. S., Yang, W., Lopez, M. I., Li, J., Olevsky, E. A., et al. (2012). Predation versus Protection: fish teeth and scales evaluated by nanoindentation. J. Materials Res. 27, 100–112. doi: 10.1557/jmr.2011.332
Chintapalli, R. K., Mirkhalaf, M., Dastjerdi, A. K., and Barthelat, F. (2014). Fabrication, testing and modelling of a new flexible armour inspired from natural fish scales and osteoderms. Bioinspir. Biomim. 9:036005. doi: 10.1088/1748-3182/9/3/036005
Dastjerdi, A. K., and Barthelat, F. (2015). Teleost fish scales amongst the toughest collagenous materials. J. Mech. Behav. Biomed. Materials 52, 95–107. doi: 10.1016/j.jmbbm.2014.09.025
Ehrlich, H. (2015). “Materials Design Principles of Fish Scales and Armor,” in Biological Materials of Marine Origin, 237–262. doi: 10.1007/978-94-007-5730-1_5
Estrada, S., Munera, J. C., Hernandez, J., Arroyave, M., Arola, D., and Ossa, A. (2020). Bioinspired hierarchical impact tolerant materials. Bioinspir. Biomim. 15: 046009. doi: 10.1088/1748-3190/ab8e9a
Feng, H., Li, X., Deng, X., Li, X., Guo, J., Ma, K., et al. (2020). The lamellar structure and biomimetic properties of a fish scale matrix. RSC Adv. 10, 875–885. doi: 10.1039/c9ra08189e
Garner, S. N., Naleway, S. E., Hosseini, M. S., Acevedo, C., Gludovatz, B., Schaible, E., et al. (2020). The role of collagen in the dermal armour of the boxfish. J. Materials Res. Technol. 9, 13825–13841. doi: 10.1016/j.jmrt.2020.09.090
Garrano, A. M. C., Rosa, G. L., Zhang, D., Niu, L. N., Tay, F. R., Majd, H., et al. (2012). On the mechanical behavior of scales from Cyprinus carpio. J. Mech. Behav. Biomed. Materials 7, 17–29. doi: 10.1016/j.jmbbm.2011.07.017
Ghods, S., Murcia, S., Ossa, E. A., and Arola, D. (2018). Design for resistance to puncture: the dynamic response of fish scales. J. Mech. Behav. Biomed. Materials 90, 451–459. doi: 10.1016/j.jmbbm.2018.10.037
Ghosh, R., Ebrahimi, H., and Vaziri, A. (2017). Non-Ideal effects in bending response of soft substrates covered with biomimetic scales. J. Mech. Behav. Biomed. Materials 72, 1–5. doi: 10.1016/j.jmbbm.2017.04.019
Gu, G. X., Su, I., Sharma, S., Voros, J. L., Qin, Z., and Buehler, M. J. (2016). 3D-printing of bio-inspired composites. J. Biomech. Eng. 138:021006.
Ha, N. S., and Lu, G. (2020). A review of recent research on bio-inspired structures and materials for energy absorption applications. Composites Part B: Eng. 181:101496.
Jiang, H., Ghods, S., Weller, E., Waddell, S., Ossa, E. A., Yang, F., et al. (2020). Contributions of intermolecular bonding and lubrication to the mechanical behaviour of a natural armour. Acta Biomaterialia. 106, 242–255. doi: 10.1016/j.actbio.2020.02.014
Ko, K., Jin, S., Lee, S. E., Lee, I., and Hong, J. W. (2019). Bio-inspired biomaterial composite patterned using three-dimensional printing. Composites Part B: Eng. 165, 594–603. doi: 10.1016/j.compositesb.2019.02.008
Le, T. V., Gazlan, A., Ngo, T., Nguyen, T., and Remennikov, A. (2019). A comprehensive review of selected biological armour systems-From structure-function to bio-mimetic techniques. Composite Structures 225:111172. doi: 10.1016/j.compstruct.2019.111172
Lin, A. (2017). Design of Flexible Puncture Resistant Gloves Inspired by Natural Dermal Armours. Master of Science thesis, Washington, WA: University of Washington.
Lin, Y. S., Wei, C. T., Olevsky, E. A., and Meyers, M. A. (2011). Mechanical properties and the laminate structure of Arapaima gigas scales. J. Mech. Behav. Biomed. Materials 4, 1145–1156. doi: 10.1016/j.jmbbm.2011.03.024
Liu, J. L., Singh, A. K., Lee, H. P., Tay, T. E., and Tan, V. B. C. (2020). The response of bio-inspired helicoidal laminates to small projectile impact. Int. J. Impact Eng. 142:103608. doi: 10.1016/j.ijimpeng.2020.103608
Liu, P., Zhu, D., Wang, J., and Bui, T. Q. (2017). Structure, mechanical behavior and puncture resistance of grass carp scales. J. Bionic Eng. 14, 356–368. doi: 10.1016/s1672-6529(16)60404-3
Liu, P., Zhu, D., Yao, Y., Wang, J., and Bui, T. Q. (2016). Numerical simulation of ballistic impact behavior of bio-inspired scale-like protection system. Materials Design 99, 201–210. doi: 10.1016/j.matdes.2016.03.040
Martini, R., Balit, Y., and Barthelat, F. (2017). A comparative study of bio-inspired protective scales using 3D printing and mechanical testing. Acta Biomaterialia 55, 360–372. doi: 10.1016/j.actbio.2017.03.025
Martini, R., and Barthelat, R. (2016a). Stability of hard plates on soft substrate and application to the design of bioinspired segmented armour. J. Mech. Phys. Solids 92, 195–209. doi: 10.1016/j.jmps.2016.04.009
Martini, R., and Barthelat, R. (2016b). Stretch-and-release fabrication, testing and optimization of a flexible ceramic armour inspired from fish scales. Bioinspir. Biomim. 11:066001. doi: 10.1088/1748-3190/11/6/066001
Meyers, M. A., Lin, Y. S., Olevsky, E. A., and Chen, P. Y. (2012). Battle in the Amazon: arapaima versus piranha. Adv. Materials 14, 279–288.
Meyers, M. A., Xu, J., and Velasco-Hogan, A. (2018). Additive manufacturing as a method to design and optimize bioinspired structures. Adv. Materials 30:1800940. doi: 10.1002/adma.201800940
Miranda, P., Pajares, A., and Meyers, M. A. (2019). Bioinspired composite segmented armour: numerical simulations. J. Materials Res. Technol. 8, 1274–1287. doi: 10.1016/j.jmrt.2018.09.007
Mirkhalaf, M., and Zreiqat, H. (2020). Fabrication and mechanics of bioinspired materials with dense architectures: current status and future perspectives. J. Minerals Metals Materials Soc. 72, 1458–1476. doi: 10.1007/s11837-019-03986-8
Murcia, S., Lavoie, E., Linley, T., Devaraj, A., Ossa, E. A., and Arola, D. (2017). The natural armors of fish A comparison of the lamination patter and structure of scales. J. Mech. Behav. Biomed. Materials 73, 17–27. doi: 10.1016/j.jmbbm.2016.09.025
Poomathi, N., Singh, S., Prakash, C., Subramanian, A., Sahay, R., Cinappan, A., et al. (2020). 3D printing in tissue engineering: a state-of-the-art review of technologies and biomaterials. Rapid Prototyp. J. 26, 1355–2546.
Porter, M. M., Ravikumar, N., Barthelat, F., and Martini, R. (2017). 3D-printing and mechanics of bio-inspired articulated and multi-material structures. J. Mech. Behav. Biomed. Materials 73, 114–126. doi: 10.1016/j.jmbbm.2016.12.016
Qi, C., Remennikov, A., Pei, L. Z., Yang, S., Yo, Z. H., and Ngo, T. D. (2017). Impact and close-in blast response of auxetic honeycomb-cored sandwich panels: experimental tests and numerical simulations. Composite Structures 180, 161–178. doi: 10.1016/j.compstruct.2017.08.020
Quan, H., Yang, W., Lapeyriere, M., Schaible, E., Ritchie, R. O., and Meyers, M. A. (2020). Structure and mechanical adaptability of a modern elasmoid fish scale from the common carp. Matter 3, 842–863. doi: 10.1016/j.matt.2020.05.011
Rawat, P., Zhu, D., Rahman, M. Z., and Barthelat, F. (2021). Structural and mechanical properties of fish scales for the bio-inspired design of flexible body armours: a review. Act Biomaterialia 121, 41–67. doi: 10.1016/j.actbio.2020.12.003
Rudykh, S., and Boyce, M. C. (2014). Analysis of elasmoid fish imbricated layered scale-tissue systems and their bio-inspired analogues at finite strains and bending. IMA J. Appl. Math. 79, 830–847. doi: 10.1093/imamat/hxu005
Rudykh, S., Ortiz, C., and Boyce, M. C. (2015). Flexibility and protection by design: imbricated hybrid microstructures of bio-inspired armour. Soft Matter 11, 25–27.
Scarponi, C., Sarasini, F., Tirillo, J., Lampani, L., Valente, T., and Guadenzi, P. (2016). Low-Velocity impact behavior of hemp fibre reinforced bio-inspired epoxy laminates. Composites Part B 91, 162–168. doi: 10.1016/j.compositesb.2016.01.048
Shafiei, A., Pro, J. W., Martini, R., and Barthelat, F. (2020). The very hard and they very soft: modelling bio-inspired skins using discrete element method. J. Mech. Phys. Solids 104176. doi: 10.1016/j.jmps.2020.104176
Sherman, V. R., Quan, H., Yang, W., Ritchie, R., and Meyers, M. (2017). A comparative study of piscine defence: the scales of Arapaima gigas, Latimeria chalumnae and Atractosteus spatula. J. Mech. Behav. Biomed. Materials 73, 1–16. doi: 10.1016/j.jmbbm.2016.10.001
Sherman, V. R., Yang, W., and Meyers, M. A. (2015). The materials science of collagen. J. Mech. Behav. Biomed. Materials 52, 22–50.
Song, J., Ortiz, C., and Boyce, M. C. (2011). Threat-protection mechanics of an armoured fish scale. J. Mech. Behav. Biomed. Materials 4, 699–712. doi: 10.1016/j.jmbbm.2010.11.011
Stopforth, R., and Adali, S. (2018). “Full metal jacket projectile penetration analysis of kevlar only bulletproof vest,” in Proceeding of the Conference: 3rd International Conference on Composites, Biocomposites and Nanocomposites 2018, (Gqeberha).
Suksangpanya, N., Yaraghi, N. A., Kisailus, D., and Zavattieri, P. (2017). Twisting cracks in bouligand structures. J. Mech. Behav. Biomed. Materials 76, 38–57. doi: 10.1016/j.jmbbm.2017.06.010
Sun, Y., Yu, Z., Wang, Z., and Liu, X. (2015). Novel protective covering to enhance concrete resistance against projectile impact. Construct. Build. Materials 96, 484–490. doi: 10.1016/j.conbuildmat.2015.08.054
Sutherland, L. S., and Soares, C. G. (2006). Impact behavior of typical marine composite laminates. Composites: Part B 37, 89–100. doi: 10.1016/j.compositesb.2005.09.001
Szewciw, L., Zhu, D., and Barthelat, R. (2017). The nonlinear flexural response of a whole teleost fish: contribution of scales and skin. J. Mech. Behav. Biomed. Materials 76, 97–103. doi: 10.1016/j.jmbbm.2017.06.014
Torres, F. G., Malasquez, M., and Troncoso, O. P. (2015). Impact and fracture analysis of fish scales from Arapaima gigas. Materials Sci. Eng. 51, 153–157. doi: 10.1016/j.msec.2015.02.034
Torres, F. G., Troncoso, O. P., Nakamatsu, J., Grande, C. J., and Gomez, C. M. (2008). Characterization of the nanocomposite laminate structure occurring in fish scales from Arapaima Gigas. Materials Sci. Eng. C 28, 1276–1283. doi: 10.1016/j.msec.2007.12.001
Touri, M., Kabirian, F., Saadati, M., Ramakrishna, S., and Mozafari, M. (2019). Additive manufacturing of biomaterials-the evolution of rapid prototyping. Adv. Eng. Materials 21:2.
Vernerey, F. J., and Barthelat, F. (2010). On the mechanics of fish scale structures. Int. J. Solids Structures 47, 2268–2275.
Vernerey, F. J., and Barthelat, F. (2014). Skin and scales of teleost fish: simple structure but high performance and multiple functions. J. Mech. Phys. Solids 68, 66–76. doi: 10.1016/j.jmps.2014.01.005
Vernerey, F. J., Musiket, K., and Barthelat, F. (2014). Mechanics of fish skin: a computational approach for bio-inspired flexible composites. Int. J. Solids Structures 51, 274–283. doi: 10.1016/j.ijsolstr.2013.10.001
Wadsworth, P., Nelson, I., Porter, D. L., Raeymaekers, B., and Naleway, S. E. (2019). Manufacturing bioinspired flexible materials using ultrasound directed self-assembly and 3D printing. Materials Design 185:108243. doi: 10.1016/j.matdes.2019.108243
White, Z., Shen, T., Volk, E. M., and Vernerey, F. J. (2019). The role of surface properties of penetration resistance of scaled skins. Mech. Res. Commun. 98, 1–8. doi: 10.1016/j.mechrescom.2019.05.001
White, Z. W., and Vernerey, F. J. (2018). Armour for soft bodies: how far can bioinspiration take us? Bioinspir. Biomim. 13:041004. doi: 10.1088/1748-3190/aababa
Wu, K., Song, Z., Zhang, S., Ni, Y., Cai, S., Gong, X., et al. (2020). Discontinuous fibrous Bouligand architecture enabling formidable fracture resistance with crack orientation insensitivity. Proc. Natl. Acad. Sci. U.S.A. 117, 15465–15472. doi: 10.1073/pnas.2000639117
Yang, W., Chen, I. H., Gludovatz, B., Zimmermann, E. A., Ritchie, R. O., and Meyers, M. A. (2013). Natural flexible dermal armor. Adv. Materials 25, 31–48. doi: 10.1002/adma.201202713
Yang, W., Naleway, S. E., Morter, M., Meyers, M. A., and McKittrick, J. (2015). The armoured carapace of the boxfish. Acta Biomaterialia 23, 1–10. doi: 10.1016/j.actbio.2015.05.024
Yang, W., and Quan, H. (2019). Arapaima fish scale: once of the toughest biological materials. Matter 1, 1557–1566. doi: 10.1016/j.matt.2019.09.014
Yang, W., Sherman, V. R., Gludovatz, B., Mackey, M., Zimmerman, E. A., Chang, E. H., et al. (2014). Protective role of arapaima gigas fish scales: structure and mechanical behaviour. Acta Biomaterialia 10, 3599–3614. doi: 10.1016/j.actbio.2014.04.009
Yao, H., Xie, Z., He, C., and Dao, M. (2015). Fracture mode control: a bio-inspired strategy to combat catastrophic damage. Sci. Rep. 5:8011.
Yaraghi, N. A., and Kisailus, D. (2018). Biomimetic structural materials: inspiration from design and assembly. Annu. Rev. Phys. Chem. 69, 23–57. doi: 10.1146/annurev-physchem-040215-112621
Zhang, C., Rawat, P., Liu, P., and Zhu, D. (2020). A new design and performance optimization of bio-inspired flexible protective equipment. Bioinspir. Biomim. [Online ahead of print] doi: 10.1088/1748-3190/aba414
Zhu, D., Ortega, C. F., Motamedi, R., Szewci, L., Vernerey, F., and Barthelat, F. (2012). Structure and mechanical performance of a “Modern”, Fish Scale. Adv. Eng. Materials 14, 185–194.
Zhu, D., Szewciv, L., Vernerey, F., and Barthelat, F. (2013). Puncture resistance of the scaled skin from striped bass: collective mechanisms and inspiration for new flexible armour designs. J. Mech. Behav. Biomed. Materials 24, 30–40. doi: 10.1016/j.jmbbm.2013.04.011
Keywords: fish scale, mechanical properties, military applications, rapid prototype, biomaterials, medical application
Citation: Yaseen AA, Waqar T, Khan MAA, Asad M and Djavanroodi F (2021) Fish Scales and Their Biomimetic Applications. Front. Mater. 8:649456. doi: 10.3389/fmats.2021.649456
Received: 05 January 2021; Accepted: 24 March 2021;
Published: 11 June 2021.
Edited by:
Federico Bosia, Politecnico di Torino, ItalyReviewed by:
Dongchan Jang, Korea Advanced Institute of Science and Technology, South KoreaCopyright © 2021 Yaseen, Waqar, Khan, Asad and Djavanroodi. This is an open-access article distributed under the terms of the Creative Commons Attribution License (CC BY). The use, distribution or reproduction in other forums is permitted, provided the original author(s) and the copyright owner(s) are credited and that the original publication in this journal is cited, in accordance with accepted academic practice. No use, distribution or reproduction is permitted which does not comply with these terms.
*Correspondence: Asim Asghar Yaseen, YXNpbWFzZ2hhcjk2QGdtYWlsLmNvbQ==
Disclaimer: All claims expressed in this article are solely those of the authors and do not necessarily represent those of their affiliated organizations, or those of the publisher, the editors and the reviewers. Any product that may be evaluated in this article or claim that may be made by its manufacturer is not guaranteed or endorsed by the publisher.
Research integrity at Frontiers
Learn more about the work of our research integrity team to safeguard the quality of each article we publish.