- Hoffmann Institute of Advanced Materials, Shenzhen Polytechnic, Shenzhen, China
Perovskite-based light-emitting diodes (PLEDs) have emerged as a promising alternative owning to the excellent optoelectronic properties including narrow emission linewidths, high photoluminescence quantum yield (PLQY), tunable emission wavelength, and high color purity. Over the past several years, significant progress has been obtained for green, red, and near-infrared PLEDs with a high external quantum efficiency (EQE) of over 20%. However, the development of blue PLEDs has been limited by several technical issues including poor film quality, inefficient device structure, higher trap density, and so on. To overcome these drawbacks with improved PLQY and EQE, we summarize and analyze the recent advances towards the development of three dimensional (3D), two dimensional (2D) and quasi-2D perovskites film employing composition and/or dimensional engineering. Moreover, the utilization of perovskite nanocrystals (PNCs) is also considered as a feasible strategy for the realization of high-performance blue PLEDs. We systematically evaluate the performance of sky-blue, pure-blue and deep blue PLEDs based on different perovskite materials. Finally, future prospective are proposed to promote the development of efficient blue PLEDs.
Introduction
Currently, metal halide perovskites has a general formula of ABX3 (A+ = monovalent organic or inorganic cation, e.g., CH3NH3+ (MA+), CH(NH2)2+ (FA+), Cs+; B2+ = divalent metal cation such as Pb2+, Sn2+, Zn2+: X− = halide anion, e.g., Cl−, Br−, I−, and SCN−) display outstanding optoelectronic properties including high charge carrier mobility, long excitation diffusion length, high absorption coefficient, narrow emission linewidth, tunable emission wavelengths from ultra-violet to near-infrared (Kumawat et al., 2019; Hu et al., 2020; Lu and Wei, 2020). Particularly, the solution-processable perovskite materials make them suitable for large-scale, cost-effective, optoelectronic applications such as solar cells (Ge et al., 2020), photodetectors (Ramasamy et al., 2016), X-ray detector (Chen P. et al., 2018), amplified lasing (Wang et al., 2015), and light-emitting diodes (LEDs) (Chiba et al., 2018; Xing et al., 2018). In 2014, the first PLEDs operated at room temperature was developed by Tan et al. with EQE of 0.76%, 0.1%, and 0.018% for near-infrared, red, and green emission, respectively (Tan et al., 2014).
Thereafter, many strategies have been employed to improve the PLQY and EQE of PLEDs through composition engineering (Leng et al., 2018; Tan et al., 2018), dimensional engineering (Chen Q. et al., 2018; Xing et al., 2018), and the application of perovskite NCs (Braly et al., 2017; Guria et al., 2017). Recently, high-performance PLEDs have been reported for the green, red and near-infrared emission with EQE of 20.3% (Lin et al., 2018), 21.3% (Chiba et al., 2018), and 20.7% (Cao et al., 2018), respectively. The outstanding progress indicates a bright future for PLEDs in the applications of displays and solid-state lighting. However, blue PLEDs, an important component for displays and lighting, show limited advancement. In this review, we categorize the recent advances of blue PLEDs into three sections based on kinds of the perovskite materials, including 3D perovskites, 2D or quasi-2D perovskites, and perovskite nanocrystals (NCs). In each category, the development of PLEDs with sky-blue, pure-blue and deep-blue emission (Figure 1A,B) are discussed by using compositional engineering (Figure 2A) in which A-, B- and X-site doping, dimensional engineering (Figure 2B) by forming low-dimensional (2D and quasi-2D) perovskite, and the utilization of perovskite NCs. Possible strategies are provided to stabilize the color of blue PLEDs and improve the PLQY of blue emissive perovskites. Finally, the future prospective is proposed for preparing high-performance blue PLEDs.
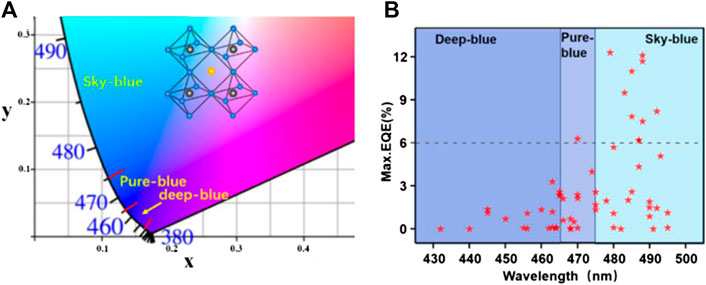
FIGURE 1. (A) Corresponding CIE coordinates for the EL spectrum; (B) Development of blue perovskite LEDs.
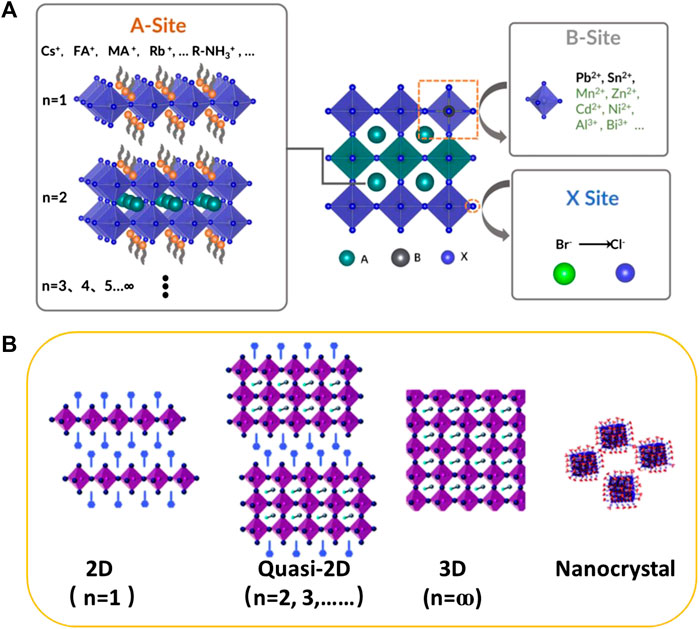
FIGURE 2. (A) Schematic illustration describing the regulation of A-, B- and X-site atoms of the perovskite to achieve blue luminescence. (B) Structure diagrams of 2D, quasi-2D, 3D perovskite and perovskite nanocrystal for dimensional engineering of PLEDs.
Recent Advances of PLEDs
3D Perovskite-Based Device
Cl/Br doping is one of the most important approaches to fabricate 3D blue PLEDs, and it was firstly proposed by Kumawat et al., in 2015 via the utilization of MAPb(Br1-XClX) (0 ≤ x ≤ 1), showing a significant blue shift when the content of Cl was increased from 0 to 1 (Kumawat et al., 2015). They successfully fabricated PLEDs with a brightness of 2 cd/m2 and a low EQE of 0.0001% when x was 0.64 at room temperature. The poor performance can be attributed to a low quality of the perovskite film and the emission quenching by [6,6]-Phenyl C61 butyric acid methyl ester (PCBM) in the device. Many efforts have been made to enhance the EQE by improving the quality of perovskite films. A triple cations strategy coupled with anti-solvent treatment was applied to obtain uniform and pinhole-free film for blue PLEDs (Kim et al., 2017). A sky-blue PLED with the device structure (ITO/ZnO/Cs10(MA0.17FA0.83)100−xPbBr1.5Cl1.5/NPD(4,4-bis [N-(1-naphthyl)-N-phenylamino]biphenyl)/MoO3/Ag was assembled by Kim et al., exhibiting a high brightness of 3,567 cd/m2 and an EQE of 1.7%. The device exhibits stable EL spectra at different bias voltages. Yuan and his co-workers systematically investigated the effect of five different A-site cations on the film morphology, crystal orientation, and photovoltaic performance (Yuan et al., 2020). They developed high-quality (Cs/Rb/FA/PEA/K)Pb(Cl/Br)3 (PEA = phenylethylammonium) based blue PLEDs and obtained an EQEmax of 2.01% with a maximum brightness of 4,015 cd/m2. Therefore, it is an effective approach to tune the emission wavelength and which suggests that multiple cations can diminish the phase segregation in mixed halide perovskites.
To realize a pure-blue emission in the range of 465–475 nm, it is necessary to introduce more amount of Cl than that for shy-blue emission, which will bring more defects in the perovskite film, leading to a lower PLQY. Meanwhile, it might cause phase separation of the mixed halides and a worse morphology of perovskite film. Wang and his co-workers introduced RbX (X is Cl/Br) as a dopant in CsPbBr3 perovskite film (Li X.-L. et al., 2019). Due to the smaller ion radius of Rb+, the bond between lead and halide is increased, leading to a shrink to the lattice. Due to the synergistic effect of partial Cl doping and Rb+ substitution, the bandgap was expanded from 2.36 to 2.52 eV (RbBr-) and 2.65 eV (RbCl−) for the doped films. The incorporated RbX could not only dramatically improve the quality and surface coverage of the film but also increase the bandgap of the doped perovskite film to achieve blue photoluminescence (PL) emission with an EQE of 0.062%. Additionally, these blue PLEDs display good color stability.
In terms of the deep-blue emission PLEDs, it becomes more difficult by using the system of MAPb(Br1−XClX), even Sadhanala et al. reported a deep emission PLEDs with two emission peaks at 425 and 450 nm without the detailed parameters of the device (Sadhanala et al., 2015). Rare works have been reported based on the application of 3D perovskite materials for the following reasons: Firstly, there are a lot of defects in MACl-based perovskite because it is relatively easy to sublime and absorb moisture, these defects become carrier recombination centers, resulting in a decrease of efficiency of radiative recombination and a reduced PLQY. Secondly, replacing MACl with inorganic chloride salt (such as CsCl), the low dissolution makes it impossible to significantly increase the chlorine content in the perovskite, deep-blue light emission cannot be realized.
Two Dimensional (2D)/Quasi Two-Dimensional (Quasi-2D) Perovskites Based PLEDs
Mixing halide perovskites is not a perfect choice because of the color instability and phase separation caused by an electric field. Generally, the PLQY is low for the chloride-containing perovskites due to the existence of a large number of halide vacancies. To enhance the luminous efficiency, a quasi-2D strategy involving long-chain ammonium ligands has been developed. The molecular formula of quasi-two-dimensional perovskite is (RNH3)2An−1BnX3n+1, where R is an aryl or alkyl long chain group and n is the number of stacked perovskite octahedron. Liang et al. developed on 2D perovskites-based blue PLEDs for the first time by employing (PEA)2PbBr4 as the emissive material, which displayed a light emission peak at 410 nm at room temperature with an EQE of 0.04% (Liang et al., 2016). Cheng et al. proposed a sky-blue PLEDs of ITO/ZnO/PEIE/(4-PBA)2PbBr4/TFB/MoO3/Al, (PEIE = polyethylenimine ethoxylated, TFB = poly (9,9-dioctyl-fluorene-co-N-(4-butylphenyl) diphenylamine), and 4-PBA = 4-phenylbutylamine), showing a brightness of 186 cd/m2 and an EQE of 0.015% (Cheng et al., 2017). Various long-chain ligands such as 2-phenyl ethyl ammonium (PEA), n-butylammonium (BA), ethyl ammonium (EA) and oleylammonium (OLA) can serve as potential barriers in the structure leading to a size reduction of the perovskite crystals. Meanwhile, increased migration of blue light and enhanced micelle binding energy are generated because of the quantum confinement effect. It’s worth noting that the quenching and enrichment of the long-chain ligand amine will retard the carrier transport of the blue PLEDs, leading to a very low EQE. A significant charge/energy transfer effect always exist in most of the quasi-two-dimensional perovskite-based researches. Yang et al. prepared PEA2(FAPbBr3)n−1PbBr4 quasi-two-dimensional perovskite film which displayed the many luminescence peaks of 2D perovskite with different n values in the PL spectrum. However, only one pure green luminescence peak at 532 nm was observed, indicating a very the presence of efficient charge/energy transfer in the quasi-2D perovskite, making it difficult to achieve blue emission (Yang et al., 2018). Xing et al. introduced dual organic small molecule phenethylamine bromide (PEABr) and isopropylammonium bromide (IPABr) based (IPA:PEA)2 (MA:Cs)n−1PbnBr3n+1 quasi 2D perovskite to reduce the charge/energy transfer (Xing et al., 2018). They found that a certain amount of short-chain IPABr can reduce the Van der Waals interaction among 2D perovskites to suppress the formation of green-emitting 2D perovskites with n = 1 or large n value, which facilitated the formation of quasi 2D perovskite with n = 2, 3, 4, leading to a blue shift. Li et al. applied an X-position component engineering to solve the charge/energy transfer problem (Li Z. et al., 2019). They increased the content of chloride to make the spectrum blue-shifted from about 523 nm (green light) to 485 nm (sky blue). Then, PEABr was introduced to form a quasi-2D perovskite, which is beneficial for reducing the defect density and improving PLQY. Based on these engineering, a high EQE up to 5.7% and a maximum brightness of 3,780 cd m−2 was obtained with further adjustment of the carriers recombination area. Recently, Chu et al. introduced ethylamine cations into the quasi-2D PEA2(CsPbBr3)2PbBr4 perovskite system and found that ethylamine ions can partially replace Cs+ in the lattice of perovskite and thereby changing the Pb-Br orbital coupling and increasing the bandgap, realizing the color regulation in the emission range of 466–508 nm (Chu et al., 2020). The maximum EQE of the sky- blue devices is 12.1%, which is currently the best performance in this field. Ren et al. proposed an effective approach to enhance the energy transfer between quasi-2D perovskite layers of the perovskite film for achieving high-performance PLEDs (Ren et al., 2020). They employed a bi-functional ligand of 4-(2-aminoethyl)benzoic acid (ABA) cation into the perovskite film to reduce the weak van der Waals gap between each perovskite layer and to promote the coupling of quasi-2D perovskite layers. Therefore, efficient energy transfer in the perovskite films generated due to the enhanced interaction between coupled quasi-2D perovskite layers. The introduced ABA can also simultaneously passivate the perovskite defects by reducing metallic Pb for a decreasing loss of non-radiative recombination. Take the advantage of the incorporated ABA, highly efficient blue PLEDs with EQE of 10.11% and long operational stability of 81.3 min were achieved. Wang and his co-workers reported a dual emission (473 and 485 nm) PLEDs based on (EA)2MAn–1PbnBr3n+1 and obtained an EQEmax of 2.6% (Wang et al., 2017). However, they found that multiple n values exist in the quasi-2D perovskite crystals and it is challenging to control the n values accurately. Chen’s group studied the energy-transfer and charge-transfer among crystals with different n values in quasi-2D PLEDs (Chen et al., 2018). Four distinct emission peaks were observed at 425, 452, 452, and 505 nm, which corresponded to n = 1, 2, 3, and 4 of PA2(CsPbBr3)n–1PbBr4 (PA = propylammonium), and the main emission peak of 505 nm was attributed to the charge-transfer. Finally, a cyan PLED emitting at 505 nm with an EQEmax of 3.6% was obtained.
Controlling the value of n is the main approach to realize the pure blue emission which can be done by the pathways as below:
1.Adjust the small-sized A-site cations to control the growth kinetics of the quasi-2D perovskite. Yantara et al. compared the UV absorption and PL spectra of the quasi-2D perovskite based on three A-site cations with different solubility: MA+, FA+ and Cs+, when forming a quasi-two-dimensional perovskite together with PBA+, and found that as the solubility of A-site ion decreasing, the absorption and PL distribution of quasi-2D perovskite became narrow, corresponding to the formation of 2D perovskites with medium n-values (Yantara et al., 2020). Considering that the formation of small n-value components requires a large amount of organic small molecule additives (PBA+), they adjusted the ratio between PBA+, Cs+ and Pb2+, and applied anti-solvent processing methods to further accelerate the nucleation process which further narrows the n value distribution. Finally, they achieved a pure blue emission with the middle n-value component (n = 3, 4), showing the maximum EQE of 2.34% for the pure blue.
2.Introduce a variety of large-size organic cations to regulate the distribution of n-value for the quasi-2D perovskite. Yuan et al. employed a small diamine (1,4-bis(aminomethyl)phenyl bromide) (P-PDABr2)) to the CsPbBr3 to obtain a higher PLQY of quasi-2D perovskite with n = 2 and n = 4. However, the final light-emitting peak located at 477 nm, which was not in the pure blue light range (Yuan et al., 2019). Then they introduced PEABr into the previous quasi-2D system, and the distribution of n-value in the new system with two different large-size organic cations was regulated again. The quasi-2D perovskite with n = 2 and n = 3 contribute mainly to the luminescence with improved PLQY. They got an EQE of 2.6% and 211 cd m−2 for the pure-blue PLED when n = 3.
3.The introduction of inorganic additives to control the distribution of n value. Pang et al. found that a certain amount of NaBr in PEA2Csn−1Pbn(ClxBr1−x)3n+1 can suppress the formation of 2D perovskites with low luminescence properties with n = 1 and promote those with n = 2, 3, 4 to realize the redistribution of quasi-2D perovskite (Pang et al., 2020). The performance of pure-blue LED based device has been greatly improved but the related mechanism needs more study in the future.
Although there are several reports about the application of quasi-2D perovskite to achieve deep-blue light emission, but the efficiency are very low. Congreve et al. introduced a small butylamine molecule into MAPbr3 to prepare quasi-2D Perovskite based PLED with an EQE of 0.0054% (Congreve et al., 2017). By applying POEA into MAPbr3, Chen et al. achieved a single EL emission peak at 462 nm, but the EQE was only 0.06% (Chen et al., 2017). Therefore, blue emission from quasi-two-dimensional Perovskite is not ideal at present. The main reasons can be summarized as below: Firstly, for the pure bromine system with a single halogen, the quasi 2D perovskite with n = 1 and n = 2 contribute mainly to the luminescence, these perovskites often have thermally induced exciton quenching, resulting in a lower PLQY. Secondly, a large number of insulating organic ligands are often introduced to obtain quasi 2D perovskite with n = 1 and n = 2, this will greatly affect the charge injection and transfer. Thirdly, the efficient charge/energy transfer in quasi-2D perovskite leads to the photon emission from the component with the minimum bandgap, which is not conducive to the deep blue emission; Fourthly, the low solubility of the Cl salts in dimethyl sulfoxide (DMSO) and N,N-Dimethylformamide (DMF) limits the possibility of regulating the composition (Zou et al., 2021).
Colloidal Perovskite Nanocrystals (PNCs) Based PLEDs
Schmidt et al. reported the first PNCs-based PLED by using MAPbBr3 PNCs with good stability and high PLQY, a low EQE was obtained due to the poor morphology of the thin perovskite film (Schmidt et al., 2014). The first attempt on blue PNC-based PLEDs was developed by Song et al., who used blue CsPb(Br1−xClx)3 (0 < x < 1) prepared by the hot-injection method to assemble a blue PLEDs with a turn-on voltage of 5.1 V (at 1 cd/m2), an EQE of 0.07%, and the highest brightness of 742 cd/m2 (Song et al., 2015). To enhance the performance of blue PNC-LEDs, surface defects and ligand density of PNCs also need to be optimized for better charge injection. Pan and co-workers conducted surface engineering for optimization of the ligand density to achieve better charge injection, which was confirmed by treating the as-prepared PNCs with oleylamine and oleic acid using DDABC (didodecy dimethyl ammonium bromide chloride) through a ligand exchange strategy (Pan et al., 2016). Ultimately, a sky-blue PLEDs) was achieved with a turn-on voltage of 3.0 V, an EQE of 1.9%, and a luminance of 35 cd/m2. The low turn-on voltage indicates that the improved charge injection after ligand density optimization. Dong et al. re-treated the surface of CsPbBr3 quantum dots by adding isopropylammonium bromide (IPABr) to the solution of CsPbBr3 quantum dots to modify the Br vacancy on the surface of quantum dots, forming an “anionic inner shell” (Dong et al., 2020). Then, adding NaBr solution to replace IPA+ on the surface by Na+, another “shell” composed of Na+ and polar solvent molecules is formed on the outer surface. This “bipolar Shell” makes the ξ potential of quantum dots (QDs) present a higher positive potential and there are no long-chain organic ligands around the QDs, therefore, the charge injection characteristics of QDs are greatly improved. This strategy enables higher carrier mobility of over 0.01 cm2 V−1 s−1 with low defect state density and high PLQY (>90%). The maximum EQE of this sky-blue PLEDs is 12.3%, which is the highest efficiency currently.
Perovskite nanocrystal also can be used as pure blue emission devices with high performance. Usually, only the perovskite quantum dots (QDs) based on the mixed ABBbxCl3-x can reach the pure-blue range. Thus, improving the quality of QDs becomes the key to fabricate high-performance pure blue LED devices. It is found that the cation doping of ABBbxCl3-x QDs is an effective method to improve its overall quality. Meng et al. prepared perovskite QDs based on (Rb0.33Cs0.67)0.42FA0.58PbBr1.75Cl1.25 system were by hot injection of multiple A-site cations (Meng et al., 2019). They found that adding a certain amount of FABr to the precursor solution could enhance the quantum confinement effect and improve the film quality, while a small amount of Rb+-doping could distort the lattice of perovskite and widen its bandgap, achieved a high PLQY of 49.8%, and an EQE of 0.61%. Additionally, Hou et al. improved the quality of perovskite QDs by the doping of B site cations with trace metal ions of Mn2+ (Hou et al., 2018). They found that the doping of Mn2+ could increase PLQY from 9% to 28% and improve the stability of the QDs. However, the rapid energy transfer between Mn2+ and perovskite QDs results in the luminescence of Mn2+ in the long-wavelength region. Therefore, in the subsequent process of synthesis, they carefully tuned the amount of Mn2+ by two-step ion exchange method to suppress self-luminescence, achieving a high-performance pure-blue perovskite LED with a EL peak at 466 nm and an EQEmax of 2.12%.
Currently, using perovskite QDs is still the most important means for deep blue perovskite LEDs. The synthesis methods of perovskite QDs could introduce a sufficient amount of Cl ions without considering the solubility of inorganic salts in the solvent of DMF or DMSO. Moreover, the quantum confinement effect brought by nanometer size is also beneficial to the shifting of the emission spectra of perovskite QDs to the deep blue zone. Song et al. developed a deep blue LED device based on perovskite QDs for the first time in 2015 by hot injection of cesium stearate (CsSt) into the PbBr2 solution, obtained pure inorganic perovskite QDs based on CsPbBr3 (Song et al., 2015). Then, the X site was doped by a certain amount of chloride ions, showing a deep-blue emission at 455 nm with an EQEmax of 0.07% and a maximum brightness of 742 cd m−2. High density of defect state of perovskite QDs is the main barrier to enhance the PLQY and device performance. Wang et al. designed a core-shell structure based CsPbBr3@CsPbBrx to reduce the loss of the radiative recombination and increase the PLQY up to 84% (Wang et al., 2018). Zhang et al. believed that the traditional ligand exchange strategy might generate a large number of point defects at the surface of QDs, resulting in a non-radiative recombination central which will decrease the efficiency (Zhang et al., 2019). They chose thionyl halide to replace the starting organic ligand and fill the vacancies of the halogen ions for better coordination with Pb2+. A deep Blue perovskite LED device was assembled successfully with an EL peak at 460 nm and the EQEmax can reach 1.35%. Yao and his co-workers applied a short-chain calcium tributylphosphine oxide (Ca-TBPO) ligand to replace the traditional long Chain organic ligands, which can effectively passivate the surface defects of CsPbCl3 and increase its PLQY from 48% to 75% (Yao et al., 2020). Next, adding a certain amount of CaBr2 into the system to adjust its emission peak to 462 nm. Finally, an EQE of 3.3% was realized and which was the highest efficiency for the current deep blue PLEDs. Migration of halogen ions is a common phenomenon in mixed-Cl/Br perovskites system for blue PLEDs, which cause the formation of Br-rich and Cl-rich phases with voltage bias, leading to a green emission (Fang et al., 2019). As an effective approach, doping has been employed to improve the EQE and stability of PLEDs. Generally, another energy level forms between the conduction band and the valence band of the perovskite by doping with various elements, therefore decreasing its color purity. However, Hou introduced a small amount of Mn to increase the brightness and life time of the PL without showing the Mn-emission peak (Hou et al., 2018). For Mn-doped device, the EQE increased from 0.50% to 2.12% with a higher PLQY and brightness and a narrower emission band. The B-site doping perovskites mentioned above possess several advantages, including the spectra adjustability by mixed halides and the stability improvement by doping, to assure higher performance in blue PLEDs. The representative research results of PLEDs based on 3D, Quasi-2D perovskites and perovskite nanocrystals are summarized in Table 1.
Prospective
We summarized the current advance of blue PLEDs and discussed the issues in terms of color and long-term instability, poor PLQY, and device structure. We also provide strategies to stabilize the improved stability and the PLQE of blue PLEDs. Decreasing the dimensionality and compositional engineering of mixed Br/Cl perovskites can be a very effective approach to realize efficient and colour-stable blue emissive perovskites, which can be conducted together with the defects passivation of perovskites employing additives. In addition, emission quenching at the interfaces between ETL/HTL and the perovskite layer needs to be minimized. It is critical to improve the charge injection ability and control the injection balance of electrons and holes by optimizing the structure of PLEDs. Other than the efficiency, device operational lifetime and spectra stability is also the main concern for blue PLEDs. It will emerge as a promising candidate for f displays and lighting applications in future to develop PLEDs with high efficiency and excellent stability.
Author Contributions
HH conceived the idea and reviewed the manuscript. CG wrote the manuscirpt. QF and HL were involved the manuscript discussion and correction.
Conflict of Interest
The authors declare that the research was conducted in the absence of any commercial or financial relationships that could be construed as a potential conflict of interest.
Acknowledgments
The financial support from National Natural Science Foundation of China (62004129) is gratefully acknowledged and this work was also supported by Shenzhen Polytechnic.
References
Braly, I. L., Stoddard, R. J., Rajagopal, A., Uhl, A. R., Katahara, J. K., Jen, A. K.-Y., et al. (2017). Current-induced phase segregation in mixed halide hybrid perovskites and its impact on two-terminal tandem solar cell design. ACS Energ. Lett. 2 (8), 1841–1847. doi:10.1021/acsenergylett.7b00525
Cao, Y., Wang, N., Tian, H., Guo, J., Wei, Y., Chen, H., et al. (2018). Perovskite light-emitting diodes based on spontaneously formed submicrometre-scale structures. Nature 562 (7726), 249–253. doi:10.1038/s41586-018-0576-2
Chen, P., Meng, Y., Ahmadi, M., Peng, Q., Gao, C., Xu, L., et al. (2018). Charge-transfer versus energy-transfer in quasi-2D perovskite light-emitting diodes. Nano Energy 50, 615–622. doi:10.1016/j.nanoen.2018.06.008
Chen, Q., Wu, J., Ou, X., Huang, B., Almutlaq, J., Zhumekenov, A. A., et al. (2018). All-inorganic perovskite nanocrystal scintillators. Nature 561 (7721), 88–93. doi:10.1038/s41586-018-0451-1
Chen, Z., Zhang, C., Jiang, X. F., Liu, M., Xia, R., Shi, T., et al. (2017). High-performance color-tunable perovskite light emitting devices through structural modulation from bulk to layered film. Adv. Mater. Weinheim 29 (8), 1603157. doi:10.1002/adma.201603157
Cheng, L., Cao, Y., Ge, R., Wei, Y.-Q., Wang, N.-N., Wang, J.-P., et al. (2017). Sky-blue perovskite light-emitting diodes based on quasi-two-dimensional layered perovskites. Chin. Chem. Lett. 28 (1), 29–31. doi:10.1016/j.cclet.2016.07.001
Chiba, T., Hayashi, Y., Ebe, H., Hoshi, K., Sato, J., Sato, S., et al. (2018). Anion-exchange red perovskite quantum dots with ammonium iodine salts for highly efficient light-emitting devices. Nat. Photon 12 (11), 681–687. doi:10.1038/s41566-018-0260-y
Chu, Z., Zhao, Y., Ma, F., Zhang, C. X., Deng, H., Gao, F., et al. (2020). Large cation ethylammonium incorporated perovskite for efficient and spectra stable blue light-emitting diodes. Nat. Commun. 11 (1), 4165. doi:10.1038/s41467-020-17943-6
Congreve, D. N., Weidman, M. C., Seitz, M., Paritmongkol, W., Dahod, N. S., and Tisdale, W. A. (2017). Tunable light-emitting diodes utilizing quantum-confined layered perovskite emitters. ACS Photon. 4 (3), 476–481. doi:10.1021/acsphotonics.6b00963
Dong, Y., Wang, Y. K., Yuan, F., Johnston, A., Liu, Y., Ma, D., et al. (2020). Bipolar-shell resurfacing for blue LEDs based on strongly confined perovskite quantum dots. Nat. Nanotechnol. 15 (8), 668–674. doi:10.1038/s41565-020-0714-5
Fang, T., Zhang, F., Yuan, S., Zeng, H., and Song, J. (2019). Recent advances and prospects toward blue perovskite materials and light-emitting diodes. InfoMat 1, 211–233. doi:10.1002/inf2.12019
Ge, C., Xue, Y. Z. B., Li, L., Tang, B., and Hu, H. (2020). Recent progress in 2D/3D multidimensional metal halide perovskites solar cells. Front. Mater. 7, 380. doi:10.3389/fmats.2020.601179
Guria, A. K., Dutta, S. K., Adhikari, S. D., and Pradhan, N. (2017). Doping Mn2+ in lead halide perovskite nanocrystals: successes and challenges. ACS Energ. Lett. 2 (5), 1014–1021. doi:10.1021/acsenergylett.7b00177
Hou, S., Gangishetty, M. K., Quan, Q., and Congreve, D. N. (2018). Efficient blue and white perovskite light-emitting diodes via manganese doping. Joule 2 (11), 2421–2433. doi:10.1016/j.joule.2018.08.005
Hu, H., Qin, Mi., Fong, P. W. K., Ren, Z., Wan, X., Singh, M., et al. (2020). Perovskite quantum wells formation mechanism for stable efficient perovskite photovoltaics-A real‐time phase‐transition study. Advanced Materials 33 (7). doi:10.1002/adma.202006238
Kim, H. P., Kim, J., Kim, B. S., Kim, H.-M., Kim, J., Yusoff, A. R. b. M., et al. (2017). High-efficiency, blue, green, and near-infrared light-emitting diodes based on triple cation perovskite. Adv. Opt. Mater. 5 (7), 1600920. doi:10.1002/adom.201600920
Kumawat, N. K., Liu, X. K., Kabra, D., and Gao, F. (2019). Blue perovskite light-emitting diodes: progress, challenges and future directions. Nanoscale 11 (5), 2109–2120. doi:10.1039/c8nr09885a
Kumawat, N. K., Dey, A., Kumar, A., Gopinathan, S. P., Narasimhan, K. L., and Kabra, D. (2015). Band gap tuning of CH3NH3Pb(Br1-xClx)3 hybrid perovskite for blue electroluminescence. ACS Appl. Mater. Inter. 7 (24), 13119–13124. doi:10.1021/acsami.5b02159
Leng, M., Yang, Y., Chen, Z., Gao, W., Zhang, J., Niu, G., et al. (2018). Surface passivation of bismuth-based perovskite variant quantum dots to achieve efficient blue emission. Nano Lett. 18 (9), 6076–6083. doi:10.1021/acs.nanolett.8b03090
Li, X.-L., Cai, X., Ali, M. U., Su, S.-J., and Meng, H. (2019). Highly efficient thermally activated delayed fluorescence yellow organic light-emitting diodes with a low efficiency roll-off. J. Mater. Chem. C 7 (26), 8063–8069. doi:10.1039/c9tc02383f
Li, Z., Chen, Z., Yang, Y., Xue, Q., Yip, H. L., and Cao, Y. (2019). Modulation of recombination zone position for quasi-two-dimensional blue perovskite light-emitting diodes with efficiency exceeding 5. Nat. Commun. 10 (1), 1027. doi:10.1038/s41467-019-09011-5
Liang, D., Peng, Y., Fu, Y., Shearer, M. J., Zhang, J., Zhai, J., et al. (2016). Color-pure violet-light-emitting diodes based on layered lead halide perovskite nanoplates. ACS Nano 10 (7), 6897–6904. doi:10.1021/acsnano.6b02683
Lin, K., Xing, J., Quan, L. N., de Arquer, F. P. G., Gong, X., Lu, J., et al. (2018). Perovskite light-emitting diodes with external quantum efficiency exceeding 20 per cent. Nature 562 (7726), 245–248. doi:10.1038/s41586-018-0575-3
Lu, J., and Wei, Z. (2020). The strategies for preparing blue perovskite light-emitting diodes. J. Semiconductors 41 (5), 051203. doi:10.1088/1674-4926/41/5/051203
Meng, F., Liu, X., Cai, X., Gong, Z., Li, B., Xie, W., et al. (2019). Incorporation of rubidium cations into blue perovskite quantum dot light-emitting diodes via FABr-modified multi-cation hot-injection method. Nanoscale 11 (3), 1295–1303. doi:10.1039/c8nr07907b
Pan, J., Quan, L. N., Zhao, Y., Peng, W., Murali, B., Sarmah, S. P., et al. (2016). Highly efficient perovskite-quantum-dot light-emitting diodes by surface engineering. Adv. Mater. 28 (39), 8718–8725. doi:10.1002/adma.201600784
Pang, P., Jin, G., Liang, C., Wang, B., Xiang, W., Zhang, D., et al. (2020). Rearranging low-dimensional phase distribution of quasi-2D perovskites for efficient sky-blue perovskite light-emitting diodes. ACS Nano 14 (9), 11420–11430. doi:10.1021/acsnano.0c03765
Ramasamy, P., Lim, D. H., Kim, B., Lee, S. H., Lee, M. S., and Lee, J. S. (2016). All-inorganic cesium lead halide perovskite nanocrystals for photodetector applications. Chem. Commun. 52 (10), 2067–2070. doi:10.1039/c5cc08643d
Ren, Z., Yu, J., Qin, Z., Wang, J., Sun, J., Chan, C. C. S., et al. (2020). High-performance blue perovskite light-emitting diodes enabled by efficient energy transfer between coupled quasi-2D perovskite layers. Adv. Mater. 33 (1), e2005570. doi:10.1002/adma.202005570
Sadhanala, A., Ahmad, S., Zhao, B., Giesbrecht, N., Pearce, P. M., Deschler, F., et al. (2015). Blue-green color tunable solution processable organolead chloride-bromide mixed halide perovskites for optoelectronic applications. Nano Lett. 15 (9), 6095–6101. doi:10.1021/acs.nanolett.5b02369
Schmidt, L. C., Pertegás, A., González-Carrero, S., Malinkiewicz, O., Agouram, S., Mínguez Espallargas, G., et al. (2014). Nontemplate synthesis of CH3NH3PbBr3 perovskite nanoparticles. J. Am. Chem. Soc. 136 (3), 850–853. doi:10.1021/ja4109209
Song, J., Li, J., Li, X., Xu, L., Dong, Y., and Zeng, H. (2015). Quantum dot light-emitting diodes based on inorganic perovskite cesium lead halides (CsPbX3). Adv. Mater. Weinheim 27 (44), 7162–7167. doi:10.1002/adma.201502567
Tan, Z. K., Moghaddam, R. S., Lai, M. L., Docampo, P., Higler, R., Deschler, F., et al. (2014). Bright light-emitting diodes based on organometal halide perovskite. Nat. Nanotechnol. 9 (9), 687–692. doi:10.1038/nnano.2014.149
Tan, Z., Li, J., Zhang, C., Li, Z., Hu, Q., Xiao, Z., et al. (2018). Highly efficient blue-emitting Bi-doped Cs2 SnCl6 perovskite variant: photoluminescence induced by impurity doping. Adv. Funct. Mater. 28 (29), 1801131. doi:10.1002/adfm.201801131
Wang, Q., Ren, J., Peng, X. F., Ji, X. X., and Yang, X. H. (2017). Efficient sky-blue perovskite light-emitting devices based on ethylammonium bromide induced layered perovskites. ACS Appl. Mater. Inter. 9 (35), 29901–29906. doi:10.1021/acsami.7b07458
Wang, S., Bi, C., Yuan, J., Zhang, L., and Tian, J. (2018). Original core–shell structure of cubic CsPbBr3@Amorphous CsPbBrx perovskite quantum dots with a high blue photoluminescence quantum yield of over 80%. ACS Energ. Lett. 3 (1), 245–251. doi:10.1021/acsenergylett.7b01243
Wang, Y., Li, X., Song, J., Xiao, L., Zeng, H., and Sun, H. (2015). All-inorganic colloidal perovskite quantum dots: a new class of lasing materials with favorable characteristics. Adv. Mater. 27 (44), 7101–7108. doi:10.1002/adma.201503573
Xing, J., Zhao, Y., Askerka, M., Quan, L. N., Gong, X., Zhao, W., et al. (2018). Color-stable highly luminescent sky-blue perovskite light-emitting diodes. Nat. Commun. 9 (1), 3541. doi:10.1038/s41467-018-05909-8
Yang, X., Zhang, X., Deng, J., Chu, Z., Jiang, Q., Meng, J., et al. (2018). Efficient green light-emitting diodes based on quasi-two-dimensional composition and phase engineered perovskite with surface passivation. Nat. Commun. 9 (1), 570. doi:10.1038/s41467-018-02978-7
Yantara, N., Jamaludin, N. F., Febriansyah, B., Giovanni, D., Bruno, A., Soci, C., et al. (2020). Designing the perovskite structural landscape for efficient blue emission. ACS Energ. Lett. 5 (5), 1593–1600. doi:10.1021/acsenergylett.0c00559
Yao, J., Wang, L., Wang, K., Yin, Y., Yang, J., Zhang, Q., et al. (2020). Calcium-tributylphosphine oxide passivation enables the efficiency of pure-blue perovskite light-emitting diode up to 3.3%. Sci. Bull. 65 (14), 1150–1153. doi:10.1016/j.scib.2020.03.036
Yuan, F., Ran, C., Zhang, L., Dong, H., Jiao, B., Hou, X., et al. (2020). A cocktail of multiple cations in inorganic halide perovskite toward efficient and highly stable blue light-emitting diodes. ACS Energ. Lett. 5 (4), 1062–1069. doi:10.1021/acsenergylett.9b02562
Yuan, S., Wang, Z. K., Xiao, L. X., Zhang, C. F., Yang, S. Y., Chen, B. B., et al. (2019). Optimization of low-dimensional components of quasi-2D perovskite films for deep-blue light-emitting diodes. Adv. Mater. 31 (44), e1904319. doi:10.1002/adma.201904319
Zhang, B. B., Yuan, S., Ma, J. P., Zhou, Y., Hou, J., Chen, X., et al. (2019). General mild reaction creates highxly luminescent organic-ligand-lacking halide perovskite nanocrystals for efficient light-emitting diodes. J. Am. Chem. Soc. 141 (38), 15423–15432. doi:10.1021/jacs.9b08140
Keywords: blue light-emitting diodes (LEDs), metal halide perovskite, external quantum efficiencies, stability, perovskite nanocrystal
Citation: Ge C, Fang Q, Lin H and Hu H (2021) Review on Blue Perovskite Light-Emitting Diodes: Recent Advances and Future Prospects. Front. Mater. 8:635025. doi: 10.3389/fmats.2021.635025
Received: 29 November 2020; Accepted: 11 January 2021;
Published: 15 March 2021.
Edited by:
Annie Ng, Nazarbayev University, KazakhstanReviewed by:
Sajid Ali Ansari, King Saud University, Saudi ArabiaAhmed Mourtada Elseman, Central Metallurgical Research and Development Institute, Egypt
Copyright © 2021 Ge, Fang, Lin and Hu. This is an open-access article distributed under the terms of the Creative Commons Attribution License (CC BY). The use, distribution or reproduction in other forums is permitted, provided the original author(s) and the copyright owner(s) are credited and that the original publication in this journal is cited, in accordance with accepted academic practice. No use, distribution or reproduction is permitted which does not comply with these terms.
*Correspondence: Hanlin Hu, hanlin.hu@szu.edu.cn