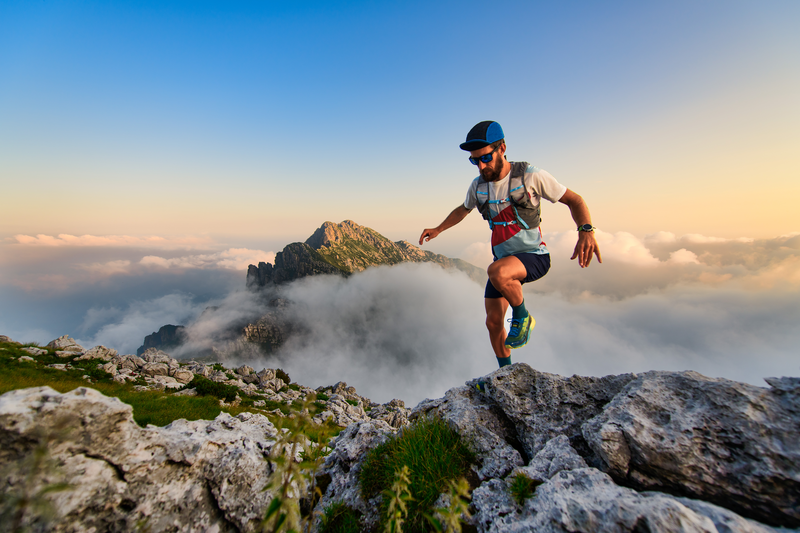
95% of researchers rate our articles as excellent or good
Learn more about the work of our research integrity team to safeguard the quality of each article we publish.
Find out more
MINI REVIEW article
Front. Mater. , 16 April 2021
Sec. Colloidal Materials and Interfaces
Volume 8 - 2021 | https://doi.org/10.3389/fmats.2021.630182
This article is part of the Research Topic 2021 Retrospective: Colloidal Materials and Interfaces View all 9 articles
Recently, there has been significant advancement in efforts toward achieving zero-energy buildings. It is quite evident that the HVAC systems consume a considerable percentage of the total energy consumption of a building; therefore, making them sustainable is of the utmost importance. Nanofluid serves as a simple, yet innovative, approach in decreasing the power consumption of the HVAC systems by improving the thermophysical properties of the coolants and enhancing heat transfer. As a result, this has attracted the attention of many researchers across the globe. This manuscript reviews the different preparation methods of nanofluid, surfactants used in stabilizing the nanofluid, and the different areas in which it can be used.
Due to global warming, the increase in demand for alternate sources of energy and sustainable technologies has increased by leaps and bounds. Consequently, in the year 2011, the American Society of Heating, Refrigerating and Air-Conditioning Engineers (ASHRAE) (Ashare and Ce, 2011) highlighted the need for energy-efficient buildings as the majority of the energy consumption is in the commercial sector. The publication also issued a design guide for small-to-medium-sized office buildings to cut down their electricity costs as well as the running cost of the HVAC systems. Since then, there has been tremendous progress in achieving net-zero buildings either by making the existing HVAC systems sustainable or by developing disruptive technologies, which can solve the purpose of reducing energy consumption. Out of these, the development of the nanofluid has been a very interesting milestone. In simple terms, nanofluid can be better understood by taking the example of the research work of Bialik et al. (2008), where the authors demonstrated how adding salt to distilled water can improve the thermophysical properties of the resultant fluid mixture such as the boiling point. The research study inferred that adding salt not only shortened the intermolecular distance but also strengthened the intermolecular bond. It was evident that, by adding salt, the ion–dipole attraction increase led to a much stronger bond than the hydrogen bonding of the water molecules. As a result, a greater amount of thermal energy was required to break the bonds, which led to the increase in the boiling point of the resultant solution. Fabuss and Korosi (1966) and Meranda and Furter (1977) also presented a comprehensive study on the effect of the boiling point of water at different salt chemical compositions and concentrations. Frederick et al. (1980) and Bujanovic and Cameron (2001) executed similar experimentations on black liquor and slash pine black liquor. Similarly, nanofluids are also a new branch of thermally optimized fluids, which are developed by mixing nanoparticles at different volume fractions into the solvent, as described in the study of Trisaksri and Wongwises (2007). The nanoparticles are generally in the form of nanotubes comprising ground micro-sized metallic powders and organic powders. The nanoparticles being in the solid form when mixed with the solvent, which is in a liquid form, together produce a two-phase (solid–liquid) mixture. As reported in various research studies discussed further, these mixtures generally have higher thermal conductivity, viscosity, coefficient of thermal convection, specific heat, and Nusselt numbers compared to other existing coolants. The article has, therefore, been divided into three parts which are discussed in detail: first, the preparation methods of the nanofluid; second, the various surfactants used in stabilizing the nanofluid mixture; and lastly, the different existing and possible applications of this fluid. Although there are a number of case studies in the domain of inorganic nanofluids, such as the ones by Wang and Mujumdar (2007, 2008), Li et al. (2009); Kakaç and Pramuanjaroenkij (2009), Özerinç et al. (2010); Yu and Xie (2012), and Devendiran and Amirtham (2016), any review work related to organic nanofluids is missing to date. As a result, the scope of this study has been limited only to organic nanofluids.
Researchers around the world are trying to synthesize environment-friendly organic nanofluids that are not only lower in cost but also have lower emissions due to rapid climate change (Mehrali et al., 2016; Zareh-Desari and Davoodi, 2016; Amani et al., 2017; Sinha et al., 2017). In inorganic nanofluids, metal ions such as Cu, Fe, Ag, Mn are used along with some solvents to synthesize the nanofluid (Wang and Mujumdar, 2007; Wang and Mujumdar, 2008; Kakaç and Pramuanjaroenkij, 2009; Li et al., 2009; Özerinç et al., 2010; Yu and Xie, 2012; Devendiran and Amirtham, 2016). On the other hand, organic nanofluids generally have alkyl groups attached with the base liquid, such as in the research work of Shanbedi et al. (2013), where the authors studied the effect of multi-walled carbon nanotubes on the efficiency of two-phase closed thermosyphon. It is seen that, along with an increase in the volume fraction of the nanoparticle in the nanofluid (organic/inorganic), the thermal conductivity and other properties such as viscosity, specific heat, and coefficient of thermal convection also increase. Consequently, the improvement of the thermophysical properties of the nanofluid using carbon nanotubes has been further explored in the research works of Mehta and Khandekar (2007), Afshar et al. (2009), Garg et al. (2009) and Mahian et al. (2013). In the research work of Majumder and Das (2020), the authors used the same technique to harness novel sodium methoxide-based nanofluid. The study revealed an increase of heat transfer by 10–12% and a decrease in power consumption of chillers by 5–10%.
It was found that, with an increase in the nanoparticle concentration in the nanofluid, the Nusselt number has also simultaneously increased (as shown in Figures 1C,D). It is known that, by improving the thermophysical properties, such as thermal conductivity and coefficient of thermal convection, of the nanofluid, the need for higher compression by the compressors decreased significantly due to the reduction on temperature difference to achieve a required refrigerating effect. As compressors are among the major power consumption devices, a reduction in their operating cost will be a huge boon for centralized air-conditioned buildings or offices as it will lead to an increase in the coefficient of performance (COP) of the system. Another eco-friendly way to reduce carbon impact is by adopting solar thermal collectors. The solar thermal collector is basically a heat exchanger that converts solar irradiation into thermal energy by trapping the heat inside its chamber and then passing the heat into a fluid medium through tubes having high thermal conductivity. Solar collectors come in different shapes depending on the extent of heating required for the fluid, such as in the review work of Tian and Zhao (2013), where the authors provided the temperature ranges in which different types of collectors work. The maximum temperature that can be reached by the flat plate collectors, parabolic troughs, and dish collectors is 373, 873, and 1473 K, respectively. The temperature at which the fluid can be heated is also dependent on the concentration ratio (CR). The CR of flat plate collectors, parabolic troughs, and dish collectors is around 1, 100, and 10,000, respectively. Due to their performance, solar tube collectors are now used widely in space heating applications and even in industrial processes, such as heat solar desalination systems, solar power systems, solar furnaces, and solar chemistry applications. Said et al. (2021) and Tiwar et al. (2021) provided a detailed review exploring the use of nanofluids in enhancing the performance of solar collectors and reducing the energy consumption of conventional air conditioning and space heating systems.
Figure 1. Development of organic nanofluid by Majumder and Das (2020) - (A) Processes involved in developing and checking the stability of the nanofluid, (B) Visual of the developed nanofluid. (C) Variation of specific heat and thermal conductivity with an increase in the volume fraction of the nanoparticle, and (D) Variation of Nusselt number with an increase in the volume fraction of the nanoparticle.
The various preparation methods adopted in different research studies for developing organic nanofluids have been discussed in detail in this section. In general, the preparation techniques have been classified based on the number of steps: (1) one-step method and (2) two-step method. First, the one-step preparation methods have been discussed in detail followed by the two-step method.
Harnessing nanosolid particles from a fluid mixture was a bit difficult, initially due to agglomeration. To eradicate this problem, Akoh et al. (1978) invented a technique known as the direct evaporation method, which was later named the Vacuum Evaporation into a Running Oil Substrate (VEROS), as shown in Figure 2A. This method uses high-pressure magnetron sputtering to synthesize solid nanoparticles from a fluid mixture. This method was further improvised by Wagener et al. (1997) and Eastman et al. (1997) for the synthesis of fluids having dispersions of Cu, Fe, and Ag particles, respectively. Another popular single-step method is the chemical vapor condensation (CVC). This process was developed by Wang et al. (2003), where the final product was achieved using evaporation and sputtering of the nanoparticles inside a vacuum chamber filled with an inert gas such as helium. The temperature inside the chamber is also fairly low due to very low pressure. As a result, when the nanoparticles collide with each other, condensation occurs and they get collected in a collection chamber at the bottom of the device, as shown in Figure 2B. Hong et al. (2005) used this process to harness Fe-based nanofluids, whereas Ramzan et al. (2020) used a numerical simulation to illustrate this process for nanotube-based organic nanofluids. Such numerical-based studies were also conducted by other researchers (Khanafer et al., 2003; Khan and Pop, 2010; Hassani et al., 2011; Sheikholeslami et al., 2013b; Zeeshan et al., 2016; Saleh et al., 2017; Srinivasacharya and Shafeeurrahman, 2017). Simultaneously, the chemical precipitation method, as shown in Figure 2C, used by Mondal et al. (2016), also serves as a single-step approach for the synthesis of nanofluids. In this study, the nanoparticles are left to be filtered away or settle at the bottom of the beaker or collecting flask. The settling mainly occurs due to the flocculation of the nanoparticles in the medium over a given period of time. Paul et al. (2010) explored the use of this method for determining the thermal properties of gold nanoparticle-based nanofluid for the first time. However, literature related to the use of this method for the synthesis of organic nanofluids is unavailable to date and, therefore, offers excellent future scope for further experimental research. Despite the advance mechanisms of synthesis, single-step approaches are often detrimental as it leads to chemical contamination and deposition, which are often difficult to treat and dispose of into the environment (Cerpa et al., 2009).
Figure 2. Different preparation methods of organic nanofluid. (A) Vacuum evaporation into a running oil substrate process by Akoh et al. (1978), (B) chemical vapor condensation process by Wang et al. (2003), (C) chemical precipitation process by Mondal et al. (2016), (D) condensation/dispersion method by Ali et al. (2018), and (E) bio-based process by Sadri et al. (2017).
Unlike the single-step approach, the nanofluid synthesis here is done in two steps. The most commonly used two-step approach for the synthesis of nanofluid is the gas condensation/dispersion method, as shown in Figure 2D. In this method, first, the nano-sized powders/particles are being synthesized using chemical methods, such as steam distillation (Fahlbusch et al., 2000; Yu and Xie, 2012), or other forms of vapor condensation and physical methods, such as grinding. Once the particles are obtained, they are then dispersed into a solvent at certain volume fractions. Following this, using stabilizers, such as centrifuge agitation, ultrasonic agitation, and magnetic sputtering, the mixture is being homogenized and is left stable for a given interval of time. This method is known for lack of complications, consumes less time and monetary expenses, and, at the same time, produces almost zero contamination (Esfe et al., 2014a). Therefore, this method found its use in the synthesis of almost all organic nanofluids for the past two decades (Baghalha and Kamal-Ahmadi, 2011; Yousefi et al., 2012; Esfe et al., 2014b; Ghozatloo et al., 2014; Sarafraz et al., 2016; Soltanimehr and Afrand, 2016; Sabiha et al., 2016; Alirezaie et al., 2017; Sadri et al., 2017; Raju et al., 2017; Choi et al., 2018; Karami et al., 2019; Zhai et al., 2019; Pourrajab et al., 2020; Almanassra et al., 2020; Dovjuu et al., 2020). Figure 1A shows a typical layout of the processes involved during the research work of Majumder and Das (2020) and an illustration of their synthesized nanofluid has been shown in Figure 1B. With this process, the fluid has also achieved stability of around –36.2, revealing no instances of agglomeration and coagulation of the nanoparticles. However, as all these methods often lead to unwanted emissions of greenhouse gases, there was a need for an eco-friendly approach. The bio-based process developed by Sadri et al. (2017) and later modified by many other authors (Sadri et al., 2017) provides a solution to this problem. In this study, we proposed a novel non-hazardous approach for synthesizing carbon nanotube-based nanofluids using a free-radical grafting reaction. Figure 2E shows the schematic diagram of the experimental setup of this process. Similar to other synthesis processes, the solubility of the nanofluid is a crucial factor while determining the thermophysical properties as finer the mixture, the better will be the heat transfer. Often, heat transfer takes place properly as the number of solids required for the energy transfer decreases due to agglomeration, thereby resulting in an overall decrease in the thermal efficiency of the system. Peng et al. (2003) and Tasis et al. (2007) focused their works on improving the solubility of the organic nanofluids in their research works and found that free-radical grafting can indeed have a positive influence on the physical characteristics of the nanofluid. Finally, it was concluded that this method was beneficial than using other chemical alternatives, such as sulfuric acid, nitric acid, or a mixture of both the chemicals, as mentioned in the research works of Yang et al. (2010); Kolacyak et al. (2011), and Chen et al. (2012).
As discussed in previous research works (Tasis et al., 2007; Yang et al., 2010), stabilizing the mixture is a crucial step toward enabling proper heat transfer through the medium. Therefore, surfactants are a commonly used material for serving this purpose (Islam et al., 2003; Murakami and Nakashima, 2006). Surfactants are amphiphile molecules, which mean that they comprise both hydrophilic and hydrophobic ends (Schubert et al., 1995). The purpose of using such a material is to break the intermolecular bonds, leading to a decrease in the surface tension and density. As the surface tension reduces, the ability of particles to float or sink in a medium can also be altered. With a lower density, the mixtures can attain better stability as the surface area over which the forces are acting increases. As a result, when surfactants are added to a given nanofluid, the chances of agglomeration and coagulation of the nanoparticles reduce drastically. This is a very critical parameter as coagulation of nanoparticles might deteriorate the thermal diffusivity of a medium, resulting in poor heat conduction and convection. Therefore, the higher the stability, the better is the thermal performance of the nanofluid. Based on the nature of the surfactant, it can be divided into three groups: (1) anionic, such as carboxylates, sulfates, sulfonates, and phosphates; (2) cationic, such as ammoniums and amines; and (3) uncharged, such as diacylglycerol and oligoethyleneglycols.
This section provides a generalized overview of the different surfactants used in different research studies in the domain of organic nanofluids. Shanbedi et al. (2013) and Sarsam et al. (2016) studied the thermal performance of multi-walled carbon nanotubes (MWCNTs)/H2O nanofluid. In this study, the nanotubes were first dispersed, and then, the mixture was stabilized using an ultrasonic stabilizer along with the addition of surfactants such as sodium dodecyl sulfate (SDS), sodium dodecyl benzene sulfonate (SDBD), cetyltrimethyl ammonium bromide (CTAB), and gum arabic (GA). Then, the effect of the aforementioned surfactants was studied at different volume fractions of the graphene nanoparticle. Both the research studies show a similar trend in their results, where the effectiveness was higher in the case of GA and lower in cases of SDS, SDBD, and CTAB. This claim was further solidified in various other research works (Bandyopadhyaya et al., 2002; Baghalha and Kamal-Ahmadi, 2011; Yousefi et al., 2012; Ghozatloo et al., 2014; Sabiha et al., 2016; Raju et al., 2017; Choi et al., 2018; Zhai et al., 2019; Almanassra et al., 2020; Dovjuu et al., 2020). However, the effectiveness of the surfactants cannot be judged on the basis of just one or two parameters. It also additionally depends on the polarity of the base fluid, such as, in the research work of Al-Waeli et al. (2019), CTAB was found to provide the maximum stability to the nanofluid, which was used by the authors to study the efficiency of the photovoltaic–thermal systems. SDS, on the other hand, has also been used extensively for stabilizing graphene/water-based nanofluid for welding, turning, and grinding processes (Haque et al., 2015; Seong et al., 2018; Zhai et al., 2019) and has been found to be quite effective. Selvam et al. (2017) studied the stability mechanism and performance of sodium deoxycholate on graphene-based nanofluids and concluded that not only the mixture was stable, but the magnitude of the coefficient of thermal convection surpassed 100%. Other surfactants such as diphenyl sulfone, benzethonium chloride (BZC), and benzalkonium chloride (BAC) have been used by Gimeno-Furio et al. (2017) and Timofeeva et al. (2011) in their research studies, where they have shown promising stability results within the standard range of –30 to +30 using the zeta potential analyzer. As the performance of the surfactants varies with temperature (Chen et al., 2008), the most plausible way of finding out the best surfactant would be to analyze the magnitude of the hydrophilic/lipophilic balance (HLB) as available in the review work of Ali et al. (2018). A higher HLB value indicates the need for a water-soluble surfactant while a lower HLB value indicates an oil-soluble surfactant.
Over the last two decades, there has been significant advancement in the development of efficient, environment-friendly, and cost-effective organic nanofluids. These thermally optimized nanofluids generally have better thermophysical properties in terms of thermal conductivity, specific heat, coefficient of thermal convection, and Nusselt number. It is quite evident from various research works cited in this review that organic nanofluids generally perform better than their inorganic counterparts. This is possible due to the long chains of the carbon nanotube-based nanoparticles and the covalent behavior of other organic additives, which provide them with better stability for a longer period of time. Despite the advantages, nanofluid does come with few disadvantages, which can be dealt with in future research works such as: (1) at high temperatures, the bond strength of surfactants reduces; (2) with an increase in the volume fraction of nanoparticle, the viscosity is observed to increase, which often leads to increase in the pumping power requirements; (3) as the experimental results are often not in close agreement with Dittus–Boelter equations, there is, therefore, a need for the development of better mathematical models to accurately predict the thermophysical properties of the nanofluid; and (4) apart from the surfactants and surfactant-free methods for achieving stability of nanofluids, future works should focus more on the shape and geometry of the nanoparticles instead. If accomplished, the need for surfactants will be eradicated. Also, as zero-energy buildings would be a new technological marvel in a few years from now, therefore, making the HVAC systems sustainable from an economical standpoint is of prime importance. As a result, more research studies should now focus on employing nanofluids in the central air conditioning systems of buildings and minimize the use of conventional refrigerants.
Both authors listed have made a substantial, direct and intellectual contribution to the work, and approved it for publication.
The authors declare that the research was conducted in the absence of any commercial or financial relationships that could be construed as a potential conflict of interest.
We would like to thank Dr. Rahul Baidya (Research Secretary, International Society of Waste Management, Air and Water and Assistant Professor, Institute of Engineering and Management, Kolkata, India) for his valuable inputs related to the review work. American Society of Mechanical Engineers (ASME) is acknowledged for their monetary support (Grant number - W-8BEN 1545-1621).
The Supplementary Material for this article can be found online at: https://www.frontiersin.org/articles/10.3389/fmats.2021.630182/full#supplementary-material
Afshar, H., Shams, M., Nainian, S., and Ahmadi, G. (2009). Microchannel heat transfer and dispersion of nanoparticles in slip flow regime with constant heat flux. Int. Commun. Heat Mass Transfer 36, 1060–1066. doi: 10.1016/j.icheatmasstransfer.2009.07.011
Ahmadi, H., Rashidi, A., Nouralishahi, A., and Mohtasebi, S. S. (2013). Preparation and thermal properties of oil-based nanofluid from multi-walled carbon nanotubes and engine oil as nano-lubricant. Int. Commun. Heat and Mass Transfer 46, 142–147. doi: 10.1016/j.icheatmasstransfer.2013.05.003
Akoh, H., Tsukasaki, Y., Yatsuya, S., and Tasaki, A. (1978). Magnetic properties of ferromagnetic ultrafine particles prepared by vacuum evaporation on running oil substrate. J. Cryst. Growth 45, 495–500. doi: 10.1016/0022-0248(78)90482-7
Ali, N., Teixeira, J. A., and Addali, A. (2018). A review on nanofluids: fabrication, stability, and thermophysical properties. J. Nanomater. 2018:6978130. doi: 10.1155/2018/6978130
Alirezaie, A., Saedodin, S., Esfe, M. H., and Rostamian, S. H. (2017). Investigation of rheological behavior of MWCNT (COOH-functionalized)/MgO-engine oil hybrid nanofluids and modelling the results with artificial neural networks. J. Mol. Liquids 241, 173–181. doi: 10.1016/j.molliq.2017.05.121
Almanassra, I. W., Manasrah, A. D., Al-Mubaiyedh, U. A., Al-Ansari, T., Malaibari, Z. O., and Atieh, M. A. (2020). An experimental study on stability and thermal conductivity of water/CNTs nanofluids using different surfactants: a comparison study. J. Mol. Liquids 304:111025. doi: 10.1016/j.molliq.2019.111025
Al-Waeli, A. H., Chaichan, M. T., Kazem, H. A., and Sopian, K. (2019). Evaluation and analysis of nanofluid and surfactant impact on photovoltaic-thermal systems. Case Stud. Therm. Eng. 13:100392. doi: 10.1016/j.csite.2019.100392
Amani, M., Amani, P., Mahian, O., and Estellé, P. (2017). Multi-objective optimization of thermophysical properties of eco-friendly organic nanofluids. J. Clean. Prod. 166, 350–359. doi: 10.1016/j.jclepro.2017.08.014
Ashare, R., and Ce, A. (2011). Advanced Energy Design Guide for Small to Medium Office Buildings: Achieving 50% Energy Savings Toward A Net Zero Energy Building. Atlanta, GA: American Society of Heating, Refrigerating and Air-Conditioning Engineers.
Baghalha, M., and Kamal-Ahmadi, M. (2011). Copper corrosion in sodium dodecyl sulphate solutions and carbon nanotube nanofluids: a modified Koutecky–Levich equation to model the agitation effect. Corrosion Sci. 53, 4241–4247. doi: 10.1016/j.corsci.2011.08.035
Bandyopadhyaya, R., Nativ-Roth, E., Regev, O., and Yerushalmi-Rozen, R. (2002). Stabilization of individual carbon nanotubes in aqueous solutions. Nano Lett. 2, 25–28. doi: 10.1021/nl010065f
Bialik, M., Sedin, P., and Theliander, H. (2008). Boiling point rise calculations in sodium salt solutions. Ind. Engi. Chem. Res. 47, 1283–1287. doi: 10.1021/ie070564c
Bujanovic, B., and Cameron, J. H. (2001). Effect of sodium metaborate on the boiling point rise of slash pine black liquor. Ind. Eng. Chem. Res. 40, 2518–2524. doi: 10.1021/ie000675a
Cerpa, M. G., Mato, R. B., Cocero, M. J., Ceriani, R., Meirelles, A. J. A., Prado, J. M., et al. (2009). Steam Distillation Applied to the Food Industry. Boca Raton, FL: CRC Press.
Chen, J., Chen, Q., and Ma, Q. (2012). Influence of surface functionalization via chemical oxidation on the properties of carbon nanotubes. J. Colloid Interface Sci. 370, 32–38. doi: 10.1016/j.jcis.2011.12.073
Chen, L., Xie, H., Li, Y., and Yu, W. (2008). Nanofluids containing carbon nanotubes treated by mechanochemical reaction. Thermochimica Acta 477, 21–24. doi: 10.1016/j.tca.2008.08.001
Choi, T. J., Jang, S. P., and Kedzierski, M. A. (2018). Effect of surfactants on the stability and solar thermal absorption characteristics of water-based nanofluids with multi-walled carbon nanotubes. Int. J. Heat and Mass Transfer 122, 483–490. doi: 10.1016/j.ijheatmasstransfer.2018.01.141
Chougule, S. S., and Sahu, S. K. (2014). Thermal performance of automobile radiator using carbon nanotube-water nanofluid—experimental study. J. Therm. Sci. Eng. Appl. 6:041009.
Devendiran, D. K., and Amirtham, V. A. (2016). A review on preparation, characterization, properties and applications of nanofluids. Renew. Sustain. Energy Rev. 60, 21–40. doi: 10.1016/j.rser.2016.01.055
Dovjuu, O., Kim, S., Lee, A., Kim, J., Noh, J., Huh, S., et al. (2020). A simple approach for heat transfer enhancement of carbon nanofluids in aqueous media. J. Nanosci. Nanotechnol. 20, 2337–2343. doi: 10.1166/jnn.2020.17375
Eastman, J. A., Choi, S. U., Li, S., Thompson, L. J., and Lee, S. (1997). “Enhanced thermal conductivity through the development of nanofluids,” in Proceedings of the 1996 MRS Fall Symposium, Vol. 457, eds E. P. George, R. Gotthardt, K. Otsuka, S. Trolier-McKinstry, and M. Wun-Fogle (Pittsburgh, PA: Materials Research Society), 3–11.
Esfe, M. H., Saedodin, S., Mahian, O., and Wongwises, S. (2014a). Thermophysical properties, heat transfer and pressure drop of COOH-functionalized multi walled carbon nanotubes/water nanofluids. Int. Commun. Heat Mass Transfer 58, 176–183. doi: 10.1016/j.icheatmasstransfer.2014.08.037
Esfe, M. H., Saedodin, S., Mahian, O., and Wongwises, S. (2014b). Heat transfer characteristics and pressure drop of COOH-functionalized DWCNTs/water nanofluid in turbulent flow at low concentrations. Int. J. Heat Mass Transfer 73, 186–194. doi: 10.1016/j.ijheatmasstransfer.2014.01.069
Fabuss, B. M., and Korosi, A. (1966). Boiling point elevations of sea-water and its concentrates. J. Chem. Eng. Data 11, 606–609. doi: 10.1021/je60031a049
Fahlbusch, K. G., Hammerschmidt, F. J., Panten, J., Pickenhagen, W., Schatkowski, D., Bauer, K., et al. (2000). “Flavors and fragrances,” in Ullmann’s Encyclopedia of Industrial Chemistry, (Weinheim: Wiley-VCH).
Frederick, W. J., Sachs, D. G., Grady, H. J., and Grace, T. M. (1980). Boiling point elevation and solubility limit for black liquors. Tappi J. 63:151.
Garg, P., Alvarado, J. L., Marsh, C., Carlson, T. A., Kessler, D. A., and Annamalai, K. (2009). An experimental study on the effect of ultrasonication on viscosity and heat transfer performance of multi-wall carbon nanotube-based aqueous nanofluids. Int. J. Heat Mass Transf 52, 5090–5101.
Ghozatloo, A., Rashidi, A. M., and Shariaty-Niasar, M. (2014). Effects of surface modification on the dispersion and thermal conductivity of CNT/water nanofluids. Int. Commun. Heat and Mass Transfer 54, 1–7. doi: 10.1016/j.icheatmasstransfer.2014.02.013
Gimeno-Furio, A., Navarrete, N., Mondragon, R., Hernandez, L., Martinez-Cuenca, R., Cabedo, L., et al. (2017). Stabilization and characterization of a nanofluid based on a eutectic mixture of diphenyl and diphenyl oxide and carbon nanoparticles under high temperature conditions. Int. J. Heat. Mass Transf. 113, 908–913. doi: 10.1016/j.ijheatmasstransfer.2017.05.097
Haque, A. M., Kwon, S., Kim, J., Noh, J., Huh, S., Chung, H., et al. (2015). An experimental study on thermal characteristics of nanofluid with graphene and multi-wall carbon nanotubes. J. Central South Univ. 22, 3202–3210. doi: 10.1007/s11771-015-2857-3
Hassani, M., Tabar, M. M., Nemati, H., Domairry, G., and Noori, F. (2011). An analytical solution for boundary layer flow of a nanofluid past a stretching sheet. Int. J. Ther. Sci. 50, 2256–2263. doi: 10.1016/j.ijthermalsci.2011.05.015
He, M., Yang, L., Lin, W., Chen, J., Mao, X., and Ma, Z. (2019). Preparation, thermal characterization and examination of phase change materials (PCMs) enhanced by carbon-based nanoparticles for solar thermal energy storage. J. Energy Storage 25:100874. doi: 10.1016/j.est.2019.100874
Hong, T. K., Yang, H. S., and Choi, C. J. (2005). Study of the enhanced thermal conductivity of Fe nanofluids. J. Appl. Phys. 97:064311. doi: 10.1063/1.1861145
Huang, H., Zhu, J., and Yan, B. (2016). Comparison of the performance of two different Dual-loop organic Rankine cycles (DORC) with nanofluid for engine waste heat recovery. Energy Conv. Manage. 126, 99–109. doi: 10.1016/j.enconman.2016.07.081
Islam, M. F., Rojas, E., Bergey, D. M., Johnson, A. T., and Yodh, A. G. (2003). High weight fraction surfactant solubilization of single-wall carbon nanotubes in water. Nano Lett. 3, 269–273. doi: 10.1021/nl025924u
Kakaç, S., and Pramuanjaroenkij, A. (2009). Review of convective heat transfer enhancement with nanofluids. Int. J. Heat Mass Transfer 52, 3187–3196. doi: 10.1016/j.ijheatmasstransfer.2009.02.006
Karami, H., Papari-Zare, S., Shanbedi, M., Eshghi, H., Dashtbozorg, A., Akbari, A., et al. (2019). The thermophysical properties and the stability of nanofluids containing carboxyl-functionalized graphene nano-platelets and multi-walled carbon nanotubes. Int. Commun. Heat Mass Transfer 108:104302. doi: 10.1016/j.icheatmasstransfer.2019.104302
Khan, W., and Pop, I. (2010). Boundary-layer flow of a nanofluid past a stretching sheet. Int. J. Heat Mass Transfer 53, 2477–2483. doi: 10.1016/j.ijheatmasstransfer.2010.01.032
Khanafer, K., Vafai, K., and Lightstone, M. (2003). Buoyancydriven heat transfer enhancement in a two-dimensional enclosure utilizing nanofluids. Int. J. Heat Mass Transfer 46, 3639–3653. doi: 10.1016/S0017-9310(03)00156-X
Kolacyak, D., Ihde, J., Merten, C., Hartwig, A., and Lommatzsch, U. (2011). Fast functionalization of multi-walled carbon nanotubes by an atmospheric pressure plasma jet. J. Colloid Interface Sci. 359, 311–317. doi: 10.1016/j.jcis.2011.03.069
Kulkarni, D. P., Das, D. K., and Vajjha, R. S. (2009). Application of nanofluids in heating buildings and reducing pollution. Appl. Energy 86, 2566–2573. doi: 10.1016/j.apenergy.2009.03.021
Li, Y., Zhou, J., Tung, S., Schneider, E., and Xi, S. (2009). A review on development of nanofluid preparation and characterization. Powder Technol. 196, 89–101. doi: 10.1016/j.powtec.2009.07.025
Li, Z. X., Khaled, U., Al-Rashed, A. A., Goodarzi, M., Sarafraz, M. M., and Meer, R. (2020). Heat transfer evaluation of a micro heat exchanger cooling with spherical carbon-acetone nanofluid. Int. J. Heat Mass Transfer 149:119124. doi: 10.1016/j.ijheatmasstransfer.2019.119124
Loni, R., Kasaeian, A. B., Mahian, O., and Sahin, A. Z. (2016). Thermodynamic analysis of an organic rankine cycle using a tubular solar cavity receiver. Energy Conv. Manage. 127, 494–503. doi: 10.1016/j.enconman.2016.09.007
Mahian, O., Kianifar, A., Kleinstreuer, C., Al-Nimr, M. A., Pop, I., Sahin, A. Z., et al. (2013). A review of entropy generation in nanofluid flow. Int. J. Heat Mass Transf 65, 514–532. doi: 10.1016/j.ijheatmasstransfer.2013.06.010
Majumder, S. D., and Das, A. (2020). Numerical and experimental investigation of Aq. Sodium methoxide as a nanofluid. Mater. Today: Proc. 43, 93–100.
Marquis, F. D. S., and Chibante, L. P. F. (2005). Improving the heat transfer of nanofluids and nanolubricants with carbon nanotubes. J. Miner. Metals Mater. Soc 57, 32–43. doi: 10.1007/s11837-005-0180-4
Mehrali, M., Sadeghinezhad, E., Akhiani, A. R., Tahan Latibari, S., Talebian, S., DolatshahiPirouz, A., et al. (2016). An ecofriendly graphene-based nanofluid for heat transfer applications. J. Clean. Prod. 137, 555–566. doi: 10.1016/j.jclepro.2016.07.136
Mehta, B., and Khandekar, S. (2007). “Two-phase closed thermosyphon with nanofluids,” in Proceedings of the 14th International Heat Pipe Conference (14th IHPC), (Brazil).
Meranda, D., and Furter, W. F. (1977). Elevation of the boiling point of water by salts at saturation: data and correlation. J. Chem. Eng. Data 22, 315–317.
Mondal, S., Dey, A., and Pal, U. (2016). Low temperature wet-chemical synthesis of spherical hydroxyapatite nanoparticles and their in situ cytotoxicity study. Adv. Nano Res. 4:295.
Murakami, H., and Nakashima, N. (2006). Soluble carbon nanotubes and their applications. J. Nanosci. Nanotechnol. 6, 16–27. doi: 10.1166/jnn.2006.17900
Nelson, C., Banerjee, D., and Ponnappan, R. (2009). Flow loop experiments using polyalphaolefin nanofluids. J. Thermophys. Heat Transfer 23, 752–761. doi: 10.2514/1.31033
Özerinç, S., Kakaç, S., and YazIcIoğlu, A. G. (2010). Enhanced thermal conductivity of nanofluids: a state-of-the-art review. Microfluid. Nanofluidics 8, 145–170. doi: 10.1007/s10404-009-0524-4
Paul, G., Pal, T., and Manna, I. (2010). Thermo-physical property measurement of nano-gold dispersed water based nanofluids prepared by chemical precipitation technique. J. Colloid Interface Sci. 349, 434–437. doi: 10.1016/j.jcis.2010.05.086
Peng, H., Reverdy, P., Khabashesku, V. N., and Margrave, J. L. (2003). Sidewall functionalization of single-walled carbon nanotubes with organic peroxides. Chem. Commun. 3, 362–363. doi: 10.1039/b209456h
Pourrajab, R., Noghrehabadi, A., Behbahani, M., and Hajidavalloo, E. (2020). An efficient enhancement in thermal conductivity of water-based hybrid nanofluid containing MWCNTs-COOH and Ag nanoparticles: experimental study. J. Therm. Anal. Calorim. 143, 3331–3343. doi: 10.1007/s10973-020-09300-y
Rahman, S., Issa, S., Said, Z., Assad, M. E. H., Zadeh, R., and Barani, Y. (2019). Performance enhancement of a solar powered air conditioning system using passive techniques and SWCNT/R-407c nano refrigerant. Case Stud. Therm. Eng. 16:100565. doi: 10.1016/j.csite.2019.100565
Raju, R. A., Andhare, A., and Sahu, N. K. (2017). Performance of multi-walled carbon nanotube-based nanofluid in turning operation. Mater. Manuf. Proc. 32, 1490–1496. doi: 10.1080/10426914.2017.1279291
Ramzan, M., Riasat, S., Kadry, S., Long, C., Nam, Y., and Lu, D. (2020). Numerical simulation of 3D condensation nanofluid film flow with Carbon nanotubes on an inclined rotating disk. Appl. Sci. 10:168. doi: 10.3390/app10010168
Refiei, A., Loni, R., Najafi, G., Sahin, A. Z., and Bellos, E. (2020). Effect of use of MWCNT/oil nanofluid on the performance of solar organic Rankine cycle. Energy Rep. 6, 782–794. doi: 10.1016/j.egyr.2020.03.035
Sabiha, M. A., Mostafizur, R. M., Saidur, R., and Mekhilef, S. (2016). Experimental investigation on thermo physical properties of single walled carbon nanotube nanofluids. Int. J. Heat and Mass Transfer 93, 862–871. doi: 10.1016/j.ijheatmasstransfer.2015.10.071
Sadhik Basha, J., and Anand, R. B. (2009). “Performance and emission characteristics of a DI compression ignition engine using carbon nanotubes blended diesel,” in Proceedings in International Conference on Advances in Mechanical Engineering, (Surat), 312–316.
Sadri, R., Hosseini, M., Kazi, S. N., Bagheri, S., Zubir, N., Solangi, K. H., et al. (2017). A bio-based, facile approach for the preparation of covalently functionalized carbon nanotubes aqueous suspensions and their potential as heat transfer fluids. J. Colloid Interface Sci. 504, 115–123. doi: 10.1016/j.jcis.2017.03.051
Said, Z., Hachicha, A. A., Aberoumand, S., Yousef, B. A., Sayed, E. T., and Bellos, E. (2021). Recent advances on nanofluids for low to medium temperature solar collectors: energy, exergy, economic analysis and environmental impact. Prog. Energy Combust. Sci. 84:100898. doi: 10.1016/j.pecs.2020.100898
Saleh, H., Alali, E., and Ebaid, A. (2017). Medical applications for the flow of carbon-nanotubes suspended nanofluids in the presence of convective condition using Laplace transform. J. Assoc. Arab Univ. Basic Appl. Sci. 24, 206–212. doi: 10.1016/j.jaubas.2016.12.001
Sarafraz, M. M., Hormozi, F., and Nikkhah, V. (2016). Thermal performance of a counter-current double pipe heat exchanger working with COOH-CNT/water nanofluids. Exp. Ther. Fluid Sci. 78, 41–49. doi: 10.1016/j.expthermflusci.2016.05.014
Sarsam, W. S., Amiri, A., Kazi, S. N., and Badarudin, A. (2016). Stability and thermophysical properties of non-covalently functionalized graphene nanoplatelets nanofluids. Energy Conv. Manag. 116, 101–111. doi: 10.1016/j.enconman.2016.02.082
Schubert, D., Behl, C., Lesley, R., Brack, A., Dargusch, R., Sagara, Y., et al. (1995). Amyloid peptides are toxic via a common oxidative mechanism. Proc. Natl. Acad. Sci. U.S.A. 92, 1989–1993. doi: 10.1073/pnas.92.6.1989
Selvam, C., Balaji, T., Lal, D. M., and Harish, S. (2017). Convective heat transfer coefficient and pressure drop of water-ethylene glycol mixture with graphene nanoplate lets. Exp. Therm. Fluid Sci. 80, 67–76. doi: 10.1016/j.expthermflusci.2016.08.013
Seong, H., Kim, G., Jeon, J., Jeong, H., Noh, J., Kim, Y., et al. (2018). Experimental study on characteristics of grinded graphene nanofluids with surfactants. Materials 11:950. doi: 10.3390/ma11060950
Shanbedi, M., Zeinali Heris, S., Baniadam, M., and Amiri, A. (2013). The effect of multi-walled carbon nanotube/water nanofluid on thermal performance of a two-phase closed thermosyphon. Exp. Heat Transfer 26, 26–40. doi: 10.1080/08916152.2011.631078
Sheikholeslami, M., Hatami, M., and Ganji, D. D. (2013b). Analytical investigation of MHD nanofluid flow in a semi-porous channel. Powder Technol. 246, 327–336.36. doi: 10.1016/j.powtec.2013.05.030
Sinha, M. K., Madarkar, R., Ghosh, S., and Rao, P. V. (2017). Application of eco-friendly nanofluids during grinding of Inconel 718 through small quantity lubrication. J. Clean. Prod. 141, 1359–1375. doi: 10.1016/j.jclepro.2016.09.212
Soltanimehr, M., and Afrand, M. (2016). Thermal conductivity enhancement of COOH-functionalized MWCNTs/ethylene glycol–water nanofluid for application in heating and cooling systems. Appl. Ther. Eng. 105, 716–723. doi: 10.1016/j.applthermaleng.2016.03.089
Srinivas, V., Moorthy, C. V., Dedeepya, V., Manikanta, P. V., and Satish, V. (2016). Nanofluids with CNTs for automotive applications. Heat Mass Transfer 52, 701–712.
Srinivasacharya, D., and Shafeeurrahman, M. (2017). Hall and ion slip effects on mixed convection flow of nanofluid between two concentric cylinders. J. Assoc. Arab Univ. Basic Appl. Sci. 24, 223–231. doi: 10.1016/j.jaubas.2017.03.002
Tasis, D., Papagelis, K., Prato, M., Kallitsis, I., and Galiotis, C. (2007). Water-soluble carbon nanotubes by redox radical polymerization. Macromol. Rapid Commun. 28, 1553–1558. doi: 10.1002/marc.200700235
Tian, Y., and Zhao, C. Y. (2013). A review of solar collectors and thermal energy storage in solar thermal applications. Appl. Energy 104, 538–553. doi: 10.1016/j.apenergy.2012.11.051
Timofeeva, E. V., Moravek, M. R., and Singh, D. (2011). Improving the heat transfer efficiency of synthetic oil with silica nanoparticles. J. Colloid Interface Sci. 364, 71–79. doi: 10.1016/j.jcis.2011.08.004
Tiwar, A. K., Kumar, V., Said, Z., and Paliwal, H. K. (2021). A review on the application of hybrid nanofluids for parabolic trough collector: recent progress and outlook. J. Clean. Prod. 292:126031. doi: 10.1016/j.jclepro.2021.126031
Trisaksri, V., and Wongwises, S. (2007). Critical review of heat transfer characteristics of nanofluids. Renew. Sustain. Energy Rev 11, 512–523. doi: 10.1016/j.rser.2005.01.010
Wagener, M., Murty, B. S., and Günther, B. (1997). “Preparation of metal nanosuspensions by high-pressure dc-sputtering on running liquids,” in Proceedings of the 1996 MRS Fall Symposium, eds E. P. George, R. Gotthardt, K. Otsuka, S. Trolier-McKinstry, and M. Wun-Fogle (Pittsburgh, PA: Materials Research Society), 149–154.
Wang, X. Q., and Mujumdar, A. S. (2007). Heat transfer characteristics of nanofluids: a review. Int. J. Ther. Sci. 46, 1–19. doi: 10.1016/j.ijthermalsci.2006.06.010
Wang, X. Q., and Mujumdar, A. S. (2008). A review on nanofluids—part I: theoretical and numerical investigations. Braz. J. Chem. Eng. 25, 613–630. doi: 10.1590/S0104-66322008000400001
Wang, Z. L., Liu, Y., and Zhang, Z. (2003). Chemical Vapor Deposition. Handbook of Nanophase and Nanostructured Materials. Cham: Springer Nature, 102–144.
Yang, Y., Qiu, S., Xie, X., Wang, X., and Li, R. K. Y. (2010). A facile, green, and tunable method to functionalize carbon nanotubes with water-soluble azo initiators by one-step free radical addition. Appl. Surface Sci. 256, 3286–3292. doi: 10.1016/j.apsusc.2009.12.020
Yousefi, T., Veisy, F., Shojaeizadeh, E., and Zinadini, S. (2012). An experimental investigation on the effect of MWCNT-H2O nanofluid on the efficiency of flat-plate solar collectors. Exp. Therm. Fluid Sci. 39, 207–212. doi: 10.1016/j.expthermflusci.2012.01.025
Yu, W., and Xie, H. (2012). A review on nanofluids: preparation, stability mechanisms, and applications. J. Nanomater. 2012:435873. doi: 10.1155/2012/435873
Yu, W., Xie, H., Chen, L., and Li, Y. (2009). Investigation of thermal conductivity and viscosity of ethylene glycol based ZnO nanofluid. Thermochimica Acta 491, 92–96. doi: 10.1016/j.tca.2009.03.007
Zareh-Desari, B., and Davoodi, B. (2016). Assessing the lubrication performance of vegetable oilbased nano-lubricants for environmentally conscious metal forming processes. J. Clean. Prod. 135, 1198–1209. doi: 10.1016/j.jclepro.2016.07.040
Zeeshan, A., Hassan, M., Ellahi, R., and Nawaz, M. (2016). Shape effect of nanosize particles in unsteady mixed convection flow of nanofluid over disk with entropy generation. Proc. Inst. Mech. Eng. E J. Proc. Mech. Eng. 231, 871–879. doi: 10.1177/0954408916646139
Keywords: organic nanofluids, HVAC systems, heat transfer, preparation, thermophysical properties
Citation: Deb Majumder S and Das A (2021) A Short Review of Organic Nanofluids: Preparation, Surfactants, and Applications. Front. Mater. 8:630182. doi: 10.3389/fmats.2021.630182
Received: 16 November 2020; Accepted: 23 March 2021;
Published: 16 April 2021.
Edited by:
Miloslav Pekař, Brno University of Technology, CzechiaReviewed by:
Rajkumar Kaliyamoorthy, SSN College of Engineering, IndiaCopyright © 2021 Deb Majumder and Das. This is an open-access article distributed under the terms of the Creative Commons Attribution License (CC BY). The use, distribution or reproduction in other forums is permitted, provided the original author(s) and the copyright owner(s) are credited and that the original publication in this journal is cited, in accordance with accepted academic practice. No use, distribution or reproduction is permitted which does not comply with these terms.
*Correspondence: Samarpan Deb Majumder, ZGVic2FtYXJwYW5tYWp1bWRlckBnbWFpbC5jb20=
Disclaimer: All claims expressed in this article are solely those of the authors and do not necessarily represent those of their affiliated organizations, or those of the publisher, the editors and the reviewers. Any product that may be evaluated in this article or claim that may be made by its manufacturer is not guaranteed or endorsed by the publisher.
Research integrity at Frontiers
Learn more about the work of our research integrity team to safeguard the quality of each article we publish.