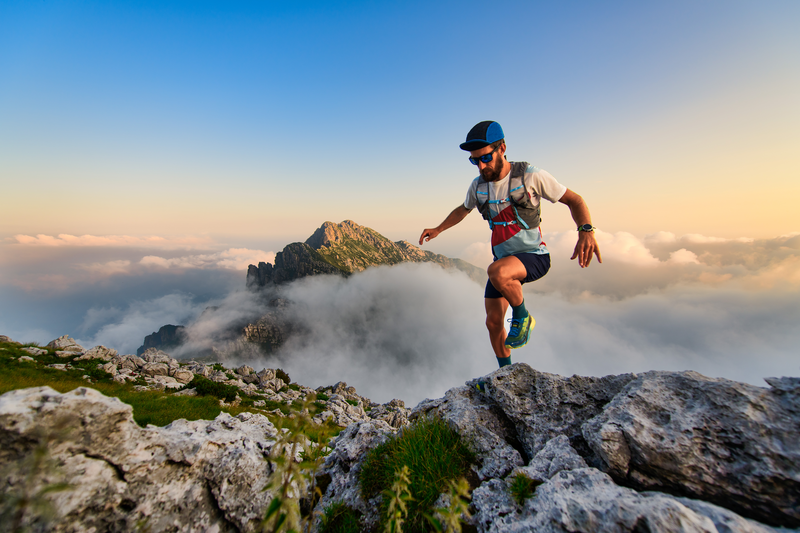
94% of researchers rate our articles as excellent or good
Learn more about the work of our research integrity team to safeguard the quality of each article we publish.
Find out more
ORIGINAL RESEARCH article
Front. Mater. , 29 January 2021
Sec. Energy Materials
Volume 7 - 2020 | https://doi.org/10.3389/fmats.2020.617199
This article is part of the Research Topic Hierarchical Nanostructures for Energy Applications View all 5 articles
The convective and conductive heat transfer between the solar collector and working fluids make photothermal performance limited, and result in a higher rate of heat loss from the surface of the conventional absorber to the surroundings. Direct absorption solar collectors (DASC) are a favorable alternative for their improved photothermal performance. In this study, a simulation based on the performance of a nanostructured solar collector has been carried out using TRNSYS. The connective and conductive heat transfer from direct solar collectors were improved by using nanofluids and three different nanostructured materials, CuO, GO, and ZnO, in this study. The analysis determines the outlet temperature of the working fluids that passed through the direct solar collector. The TRNSYS model consists of a direct solar collector and weather model for Lahore city, the simulations were performed for the whole year for 1,440 h. The stability of these nanostructured materials in the water was investigated by using a UV‐Vis spectrophotometer. Various performance parameters of direct solar collectors were determined, such as variation in outlet collector temperature and heat transfer rates. The numerical model is validated with experimental results. A maximum outlet temperature of 63°C was observed for GO-based nanofluids. The simulation results show that for the whole year, nanofluids improved the performance of direct solar collectors. Significant improvements in the heat transfer rate of 23.52, 21.11, and 15.09% were observed for the nanofluids based on nanostructures of CuO, ZnO, and GO respectively, as compared to water. These nanostructured energy materials are beneficial in solar-driven applications like solar desalination, solar water, and space heating.
Due to the rapid increase in population, energy demands are increasing day by day and fossil fuels are being widely used to meet this demand (Kriegler et al., 2017; Grubler et al., 2018). The burning of fossil fuels causes serious environmental issues (Ağbulut and Sarıdemir, 2019); to overcome these problems, alternative energy resources are being explored. Among alternative energy resources, solar energy has gained the attention of many researchers for differing reasons (Dudin et al., 2016; Oh et al., 2018; Guangul and Chala, 2019). The Sun is a universal renewable energy source (Moner-Girona et al., 2016). Solar energy utilization can be done by converting sun heat energy into a chemical form of energy, an electric form of energy, or a thermal form of energy. Thermal energy is the most commonly used among all of these. Solar collectors have been utilized for the converting of solar radiation into thermal energy (Conrado et al., 2017; Bellos and Tzivanidis, 2019). Solar energy is collected through flat-surface-based solar plates, converting this collected energy into heat and then delivering it to working fluid (Amjad et al., 2018a; Dehaj and Mohiabadi, 2019). However, the major drawback of this conventional surface base flat type is that, as the temperature of the flat plate increases, the radiant loss of heat occurs (Kılkış, 2000; Sint et al., 2017; Shafieian et al., 2019).
Therefore, it is necessary to exchange these typical solar collectors with directly absorbing solar collectors (Amjad et al., 2018b; Goel et al., 2020). Another way to increase photothermal conversion performance is through the use of composite nanofluids, which can be obtained by mixing binary nanoparticles. To abridge the collector and increase its efficiency, direct absorption solar collector (DASC) has been suggested. DASC absorbs large amounts of solar radiation from the Sun, meaning temperature distribution is uniform throughout the surface and results in a small amount of heat loss as compared to surface-based collectors (Arefin, 2019; Poompavai and Kowsalya, 2020). The study of direct absorption solar thermal collectors has been carried out using a composite nanofluids solution (Gorji and Ranjbar, 2017; Wang et al., 2017). The application of nanoparticles in DASC is a relatively new concept (Pathak, 2020). Nanoparticles disseminate in the base fluids and offer the potential to improve the optical characteristics of their nanofluids. Nanofluids consisting of nanoparticles are the best medium for transferring heat in solar collectors; composite nanofluids can be obtained by mixing these absorbing dark nanoparticles into the working fluids, a method which has been broadly studied previously (Chamsa-ard et al., 2017; Olia et al., 2019), (Amjad et al., 2017a; Amjad et al., 2017b). In addition to this, direct absorption solar collectors show greater efficiency as compared to the conventional absorption of Al/H2O nanofluids with that of pure water. Al/H2O nanofluids have a high absorption capacity and are 10% more efficient with an indirect absorption solar collector using nanofluids than that of the typical type of flat plate collector (Salehi et al., 2020). Dark metal has a better absorbance rate (Zook et al., 2011). When the frequency of incident light becomes constant with the fluctuating frequency of electrons of dark nanoparticles, then localized surface Plasmon resonance will start occurring (Coronado et al., 2011; Yu et al., 2019), thus increasing absorption capacity in the spectral range of nanoparticles. Further, a binary combination of metal composite materials and nanostructures not only increases visual absorption (Basavarajappa et al., 2020), but also alters the spectral range properties to increase efficiency. Composite nanoparticles with different size range structures can achieve an effective capacity of absorption of solar energy, and studying the spectral properties revealed that the spectrum of absorption can be adjusted by changing the shape, size, and material of nanoparticles, so as to attain the most suitable solar absorption (Li, 2012). The concentration of particles to some amount improves visual absorption, while changing to a higher rate of concentration will create some issues, such as flow viscosity increasing (Viamajala et al., 2009).
The solar irradiance of typical solar thermal collectors heats up the surface of an absorber by using the method of selective coating and, due to temperature differences, heat is transferred to the working fluid (Madhukeshwara and Prakash, 2012). Nanofluids containing nanoparticles that are efficiently absorbing solar rays promise to remove these difficulties in the conversion of photo thermal solar energy (Wang et al., 2017). Particularly, their higher level of visual and thermophysical properties, and thermal conductivity, play vital roles in increasing the capacity of solar energy absorption and its conversion (Mehrali et al., 2018).
Goudarzi et al. (Goudarzi et al., 2014) used CuO nanofluids in evacuated solar collectors and investigated its thermal performance; it was observed that the performance was enhanced by 30% when using CuO nanofluid as compared to water. Ghaderian et al. (Ghaderian et al., 2017) used nanoparticles for heat transfer; the thermal performance analysis of a solar thermal collector was investigated by deploying the finite difference method in MATLAB, the results of which exhibit in the temperature range of 30–100°C the radiant loss was increased from 26 to 58 W/m. With the addition of multiple wall carbon nanotubes into the base fluid, the convective heat coefficient increased, and a considerable improvement in coefficients of heat transfer was observed with increasing concentrations of nanoparticles. Bianco et al. (Bianco et al., 2018) carried out numerical simulations of Al2O3-based nanofluids in a flat plate photovoltaic cell; a two-dimensional model was developed in COMSOL and nanofluids of different concentrations (0%–6%) were passed through PV/T with laminar flow. The results show excellent improvement in the cooling of PV/T cell, which improves the overall efficiency of the cell.
In this present study, three different nanostructured materials, CuO, GO, and ZnO, were added into water, and these nanofluids were passed through a direct solar collector to absorb the heat of the solar collector. TRNSYS software was used in this study to simulate the heat absorbed by these nanofluids; the simulation was performed for Lahore city weather conditions for the whole year.
The basic schematic diagram of the numerical model has been shown in Figure 1. The “Type 109” weather conditions were used for Lahore city. In TRNSYS there are built-in files saved for weather data of many cities across the globe, and it also has updated weather data in its database for Lahore. The direct solar collector of “Type1b” was used, which absorbed the incoming radiation of the sun for a time span of 12 h and transmitted these radiations directly to the working fluid, named “Type 14 h,” for water mixed with nanoparticles. The remaining components interpolate the information of thermal heat absorbed by nanoparticles and transmit it to the water. The plotter was used to plot every millisecond of information in its database and then, after summarizing the result in the database of TRNSYS, “Type 25a” printer was used to represent the graphical results. The operating conditions of the TRNSYS model have been shown in Table 1.
FIGURE 1. Arrangement of the components in TRNSYS for the simulation process where direct solar collector is of Type 1b, weather condition is Type 109, and printer is Type 25a.
The continuity equation:
Momentum equations:
Energy equations of working fluids:
Energy equations for walls of direct solar collector:
Where, u, v, and w are the velocity components in the x, y, and z directions, respectively.
Nanoparticles have gained the attention of many researchers due to their wide range of applications in the heat transfer process. The selection of nanoparticles depends upon their stability in base fluids; metal oxide and carbon-based nanoparticles, including ZnO, CuO, and GO, were used in this study due to their high stability, higher thermal conductivity, and high absorptivity as compared to others. The stability of these nanoparticles in water was checked by UV-spectrography and the kinematic viscosity of these nanofluids was determined by using a rheometer. The physiochemical characteristics of these nanoparticles were shown in Table 2. These nanoparticles were added to water to make nanofluids and passed from the bottom surface of the direct solar collector. All nanoparticles have different specific heat capacity values, due to which all nanofluids show a different rise in temperature and heat transfer rates. The simulation for these nanofluids was done daily for 4 h from 10:00 AM to 2:00 PM over a one-year time span. The stability of these nanoparticles has been shown in Figure 2. Water shows minimum absorbance as compared to all three nanofluids because water is a poor absorber of visible light. The solar absorbance of water can be increased by adding nanoparticles, due to their better absorptivity into visible light. The stability of these nanoparticles into base water was checked by using a UV-spectrometer. All nanofluids exhibited different absorption peaks at different wavelengths. The maximum absorptivity was shown by GO-based nanofluids at a wavelength of 270 nm. Both metal-based nanofluids exhibited lower absorbance as compared to GO nanofluids; the peaks for ZnO and CuO nanofluids were observed at a wavelength of ranges from 275 to 287 nm.
The nanofluids that were passed through the directly absorbing solar collector were simulated using a component-based software known as TRNSYS, which simulates the performance of several transient systems. This software involves different options for different systems, which are referred to as “Type.” In this present study, Type 1b system was used to model a direct solar collector with an entrenched layer of nanofluids passing from the bottom of this system. These nanofluids absorb the direct radiation that comes from the sun and transfer heat; in this study the overall solar collection has been calculated based on the intensity of sun radiation and cell temperature of the solar collector. Nanofluids configuration with a direct solar collector has been shown in Figure 3. The direct solar collector consists of transparent glass and adiabatic surfaces; the upper transparent glass surface absorbs the solar radiation directly from the sun, while the lower surface is adiabatic. Due to solar radiation, the temperature of the solar collector rises, which distinctly affects the photothermal efficiency of the solar collector. The photothermal efficiency of the direct solar collector can be improved to remove the absorbed heat; the conductive and convective heat generated in the direct solar collector were removed by passing nanofluids through the collector. Suspended nanoparticles in the base water absorb the heat from the upper surface of the solar collector and cool down the solar collector.
FIGURE 3. Schematic diagram of a direct solar collector with nanofluids (adapted from (Karami et al., 2014)).
The location used in TRNSYS for simulation was Lahore city and the coordinates are 31.695o North latitude and 74.244o East longitude at an elevation of 220 m above sea level. Type 109 weather condition model was used for the selected city for the whole year. The solar flux of four months from May to August has been shown in Figure 4; the maximum solar intensity was found in these four months in Pakistan. Figure 5 represents the average change in day and nighttime temperature for Lahore city for the whole year. The peak temperature was observed from May to August 2020. The maximum daytime temperature of 41°C was observed in June, while at nighttime the temperature starts to decrease.
The overall efficiency of direct solar collectors is adversely affected by increasing temperatures. Various techniques have been developed to reduce temperature; a coating of phase change was used at the backside of the PV cell to decrease the temperature, but very few studies have been done on aqueous nanofluids for direct solar collectors to reduce the temperature (Rezvanpour et al., 2020). As metal-oxide-based and carbon-based nanoparticles are better heat absorbers than water, a mixture of nanoparticles with water—termed as nanofluids—decreased the temperature of solar collectors effectively by absorbing the heat released by the collector. The simulation of nanofluids passed through the direct solar collector was performed for 4 h daily from 10:00 AM to 02:00 PM for the whole year. The direct solar collector absorbs the direct radiation from the sun and increases the inside temperature of the collector; four different working fluids were passed through the direct solar collector, including water and nanofluids consisting of three different nanoparticles (CuO, GO, and ZnO). The numerical results obtained from TRNSYS were compared with experimental results. The numerical results were linked with the experimental results observed by Sattar et al. (Sattar et al., 2020) and the variation in collector temperature (Tcol) with simulation hours for the whole year has been shown in Figure 6.
FIGURE 6. Variation in experimental and simulation results for the outlet temperature of the solar collector for various working fluids.
The maximum temperature peaks for all working fluids were observed for summer hours. The maximum temperature was observed for GO-based nanofluids, followed by ZnO and CuO, and the minimum temperature was observed for pure water. Both numerical and experimental results show a similarity in behavior as time increases the temperature rises for all working fluids. For GO-based nanofluids, the difference between numerical and experimental results was observed at 20%. The difference between the experimental and numerical temperature results is associated with instrumental and human error and the heat leakage to surroundings with the increase in temperature may also contribute to this difference. Moreover, the TRNSYS model may also generate relatively smaller deviated results due to the difference in the characteristics defined in the numerical model than the actual physical properties, like specific heat value, thermal conductivity, and density. The maximum temperature shown by GO-based nanofluids was 63°C, followed by ZnO showing 49°C, CuO showing 45°C, and water showing 37°C. The temperature profile for all these working fluids for a specific day of the summer season for a time duration of 10:00 AM to 2:00 PM has been shown in Figure 7. The maximum temperature was observed in Lahore in June; during peak hours the temperature of the direct solar collectors also increased. The temperature profile of working fluids represents the heat absorption of these fluids which raises the temperature of the nanofluids passes through the solar collectors. The maximum temperature was shown for the GO nanofluid, followed by ZnO, CuO, and water. The maximum temperature of 63°C was observed during peak hours of the summer season (June 30, 2019). The temperature profile for all working fluids increased sharply from 10:00 AM to 01:00 PM and then started to decrease. Rezvanpour et al. (Rezvanpour et al., 2020) investigated the enhancement of conversion efficiency photovoltaic cell by using phase change materials and performed experimental and simulation analysis. A significant reduction of 26°C in temperature was observed with the use of phase change materials in a photovoltaic cell.
FIGURE 7. Temperature profile of all working fluids for a specific day of the summer season of June 2019.
The variation in heat transfer for four different working fluids has been shown in Figure 8. As the direct solar collector absorbs all radiation that comes from the sun and then heats up, this continued rise in temperature of the solar collector affects its overall efficiency. To improve the efficiency of the direct solar collector, different techniques are used for cooling purposes. One technique is to use pure water, but the use of metal-oxide-and carbon-based nanoparticles gain the most attention. The maximum heat transfer rate has been observed for GO-based nanofluids as, due to their black color, they absorb most of the heat that comes from the sun directly. Both metal-based nanoparticles, zinc oxide and copper oxide, due to their better thermal conductivities also absorb more heat as compared to basefluid. The heat transfer rate has been improved with the addition of nanoparticles in water; a significant improvement in the heat transfer rate of 11.33, 20, and 28.25% has been observed for GO, CuO, and ZnO nanofluids, respectively, as compared to basefluid (water) at a simulation time of 108 h.
The heat transfer modes in direct solar collectors are convective and radiate heat transfer; both modes of heat transfer have been shown in Figure 9. In convective heat transfer, the flowing fluid from the bottom surface of the solar collector absorbs the dissipating heat from the upper transparent glass and cools it down. The second mode of heat transfer is radiation in which no medium is required for heat transfer; anything that is above the absolute temperature emits radiation and this radiation is absorbed by nanofluids.
In this study, the solar energy captured using nanostructured energy materials in a direct absorption solar collector is simulated using TRNSYS. The performance of the direct solar collector was evaluated at various concentrations of nanostructured materials, including GO, CuO, and ZnO, where water was used as basefluid. The weather model used in TRNSYS was Type 109 model and the location of the selected model was Lahore, Pakistan. Photothermal performance of the dark-colored nanofluid GO was maximum due to its higher thermal conductivity and better absorption characteristics. The maximum outlet temperature of 63°C was observed for GO nanofluid during peak hours of the summer season (June 30, 2019). A significant improvement in the heat transfer rate of 23.52, 21.11, and 15.09% was observed for the nanofluids of GO, ZnO, and CuO, respectively, when compared with water as basefluid. The results showed that GO nanofluid is a potential candidate for solar thermal energy-based applications.
The original contributions presented in the study are included in the article/Supplementary Material, further inquiries can be directed to the corresponding author.
MZ and MA conceived the idea and planned work. MZ executed the work and drafted the manuscript in coordination with MA. MA and MF supervised the work, ZA and RS refined the draft and help explaining the underneath mechanism of enhanced photothermal performance. EF and XD reviewed the manuscript and refined the result interpretations with other authors. All authors contributed equally in the preparation of this manuscript to the submission phase.
Financial assistance for the study was received from the University of Engineering and Technology Lahore (UET) under FRG (Faculty Research Grant) and is highly acknowledged.
The authors declare that the research was conducted in the absence of any commercial or financial relationships that could be construed as a potential conflict of interest.
Ağbulut, Ü., and Sarıdemir, S. (2019). A general view to converting fossil fuels to cleaner energy source by adding nanoparticles. Int. J. Ambient Energy, 1–6. doi:10.1080/01430750.2018.1563822
Akbar, N. S., Raza, M., and Ellahi, R. (2016). Copper oxide nanoparticles analysis with water as base fluid for peristaltic flow in permeable tube with heat transfer. Comput. Methods Progr. Biomed. 130, 22–30. doi:10.1016/j.cmpb.2016.03.003
Amjad, M., Raza, G., Xin, Y., Pervaiz, S., Xu, J., Du, X., et al. (2017a). Volumetric solar heating and steam generation via gold nanofluids. Appl. Energy. 206, 393–400. doi:10.1016/j.apenergy.2017.08.144
Amjad, M., Yang, Y., Raza, G., Gao, H., Zhang, J., Zhou, L., et al. (2017b). Deposition pattern and tracer particle motion of evaporating multi-component sessile droplets. J. Colloid Interface Sci. 506, 83–92. doi:10.1016/j.jcis.2017.07.025
Amjad, M., Gardy, J., Hassanpour, A., and Wen, D. (2018a). Novel draw solution for forward osmosis based solar desalination. Appl. Energy. 230, 220–231. doi:10.1016/j.apenergy.2018.08.021
Amjad, M., Jin, H., Du, X., and Wen, D. (2018b). Experimental photothermal performance of nanofluids under concentrated solar flux. Sol. Energy Mater. Sol. Cells. 182, 255–262. doi:10.1016/j.solmat.2018.03.044
Arefin, M. A. (2019). Analysis of an integrated photovoltaic thermal system by top surface natural circulation of water. Front. Energy Res. 7, 97. doi:10.3389/fenrg.2019.00097
Barreneche, C., Mondragon, R., Ventura-Espinosa, D., Mata, J., Cabeza, L. F., Fernández, A. I., et al. (2018). Influence of nanoparticle morphology and its dispersion ability regarding thermal properties of water used as phase change material. Appl. Therm. Eng. 128, 121–126. doi:10.1016/j.applthermaleng.2017.09.014
Basavarajappa, P. S., Patil, S. B., Ganganagappa, N., Reddy, K. R., V Raghu, A., and Reddy, C. V. (2020). Recent progress in metal-doped TiO2, non-metal doped/codoped TiO2 and TiO2 nanostructured hybrids for enhanced photocatalysis. Int. J. Hydrogen Energy. 45 (13), 7764–7778. doi:10.1016/j.ijhydene.2019.07.241
Bellos, E., and Tzivanidis, C. (2019). Alternative designs of parabolic trough solar collectors. Prog. Energy Combust. Sci. 71, 81–117. doi:10.1016/j.pecs.2018.11.001
Bianco, V., Scarpa, F., and Tagliafico, L. A. (2018). Numerical analysis of the Al2O3-water nanofluid forced laminar convection in an asymmetric heated channel for application in flat plate PV/T collector. Renew. Energy. 116, 9–21. doi:10.1016/j.renene.2017.09.067
Cabaleiro, D., Pastoriza-Gallego, M. J., Piñeiro, M. M., and Lugo, L. (2013). Characterization and measurements of thermal conductivity, density and rheological properties of zinc oxide nanoparticles dispersed in (ethane-1,2-diol + water) mixture. J. Chem. Thermodyn. 58, 405–415. doi:10.1016/j.jct.2012.10.014
Chamsa-ard, W., Brundavanam, S., Fung, C. C., Fawcett, D., and Poinern, G. (2017). Nanofluid types, their synthesis, properties and incorporation in direct solar thermal collectors: a review. Nanomaterials 7 (6), 131. doi:10.3390/nano7060131
Conrado, L. S., Rodriguez-Pulido, A., and Calderón, G. (2017). Thermal performance of parabolic trough solar collectors. Renew. Sustain. Energy Rev. 67, 1345–1359. doi:10.1016/j.rser.2016.09.071
Coronado, E. A., Encina, E. R., and Stefani, F. D. (2011). Optical properties of metallic nanoparticles: manipulating light, heat and forces at the nanoscale. Nanoscale 3 (10), 4042–4059. doi:10.1039/c1nr10788g
Dehaj, M. S., and Mohiabadi, M. Z. (2019). Experimental investigation of heat pipe solar collector using MgO nanofluids. Sol. Energy Mater. Sol. Cells. 191, 91–99. doi:10.1016/j.solmat.2018.10.025
Dudin, M. N., Frolova, Е. Е., Kucherenko, P. A., Vernikov, V. A., and Voykova, N. A. (2016). China in innovative development of alternative energy advanced industrial technologies. Int. J. Energy Econ. Pol. 6 (3), 537–541.
Esfahani, M. R., Languri, E. M., and Nunna, M. R. (2016). Effect of particle size and viscosity on thermal conductivity enhancement of graphene oxide nanofluid. Int. Commun. Heat Mass Tran. 76, 308–315. doi:10.1016/j.icheatmasstransfer.2016.06.006
Ghaderian, J., Sidik, N. A. C., Kasaeian, A., Ghaderian, S., Okhovat, A., Pakzadeh, A., et al. (2017). Performance of copper oxide/distilled water nanofluid in evacuated tube solar collector (ETSC) water heater with internal coil under thermosyphon system circulations. Appl. Therm. Eng. 121, 520–536. doi:10.1016/j.applthermaleng.2017.04.117
Goel, N., Taylor, R. A., and Otanicar, T. (2020). A review of nanofluid-based direct absorption solar collectors: design considerations and experiments with hybrid PV/Thermal and direct steam generation collectors. Renew. Energy. 145, 903–913. doi:10.1016/j.renene.2019.06.097
Gorji, T. B., and Ranjbar, A. A. (2017). A review on optical properties and application of nanofluids in direct absorption solar collectors (DASCs). Renew. Sustain. Energy Rev. 72, 10–32. doi:10.1016/j.rser.2017.01.015
Goudarzi, K., Shojaeizadeh, E., and Nejati, F. (2014). An experimental investigation on the simultaneous effect of CuO–H2O nanofluid and receiver helical pipe on the thermal efficiency of a cylindrical solar collector. Appl. Therm. Eng. 73 (1), 1236–1243. doi:10.1016/j.applthermaleng.2014.07.067
Grubler, A., Wilson, C., Bento, N., Boza-Kiss, B., Krey, V., McCollum, D. L., et al. (2018). A low energy demand scenario for meeting the 1.5 C target and sustainable development goals without negative emission technologies. Nat. energy. 3 (6), 515–527. doi:10.1038/s41560-018-0172-6
Guangul, F. M., and Chala, G. T. (2019). “Solar energy as renewable energy source: SWOT analysis,” 2019 4th MEC international conference on big data and smart city, Muscat, Oman, January 15–16, 2019 (Oman: ICBDSC), 1–5.
Karami, M., Raisee, M., and Delfani, S. (2017). Numerical investigation of nanofluid-based solar collectors, IOP Conf. Ser.: Mater. Sci. Eng. 64, 012044. doi:10.1088/1757-899X/64/1/012044
Kriegler, E., Bauer, N., Popp, A., Humpenöder, F., Leimbach, M., Strefler, J., et al. (2017). Fossil-fueled development (SSP5): an energy and resource intensive scenario for the 21st century. Global Environ. Change. 42, 297–315. doi:10.1016/j.gloenvcha.2016.05.015
Kılkış, B. (2020). Development of a composite PVT panel with PCM embodiment, TEG modules, flat-plate solar collector, and thermally pulsing heat pipes. Sol. Energy. 200, 89–107.
Li, Y. (2012). Molecular design of photovoltaic materials for polymer solar cells: toward suitable electronic energy levels and broad absorption. Acc. Chem. Res. 45 (5), 723–733. doi:10.1021/ar2002446
Madhukeshwara, N., and Prakash, E. S. (2012). An investigation on the performance characteristics of solar flat plate collector with different selective surface coatings. Int. J. Energy Environ. 3 (1), 99–108.
Mehrali, M., Ghatkesar, M. K., and Pecnik, R. (2018). Full-spectrum volumetric solar thermal conversion via graphene/silver hybrid plasmonic nanofluids. Appl. Energy. 224, 103–115. doi:10.1016/j.apenergy.2018.04.065
Moner-Girona, M., Bódis, K., Huld, T., Kougias, I., and Szabó, S. (2016). Universal access to electricity in Burkina faso: scaling-up renewable energy technologies. Environ. Res. Lett. 11 (8), 84010. doi:10.1088/1748-9326/11/8/084010
Oh, T. H., Hasanuzzaman, M., Selvaraj, J., Teo, S. C., and Chua, S. C. (2018). Energy policy and alternative energy in Malaysia: issues and challenges for sustainable growth–an update. Renew. Sustain. Energy Rev. 81, 3021–3031. doi:10.1016/j.rser.2017.06.112
Olia, H., Torabi, M., Bahiraei, M., Ahmadi, M. H., Goodarzi, M., and Safaei, M. R. (2019). Application of nanofluids in thermal performance enhancement of parabolic trough solar collector: state-of-the-art. Appl. Sci. 9 (3), 463. doi:10.3390/app9030463
Pathak, N. K. (2020). Plasmonic nanostructures for energy application. Front. Mech. Eng. 6, 53. doi:10.3389/fmech.2020.00053
Poompavai, T., and Kowsalya, M. (2020). Investigation of standalone solar photovoltaic water pumping system with reduced switch multilevel inverter. Fronti. Energy Res. 8 (9). doi:10.3389/fenrg.2020.00009
Rezvanpour, M., Borooghani, D., Torabi, F., and Pazoki, M. (2020). Using CaCl2·6H2O as a phase change material for thermo-regulation and enhancing photovoltaic panels’ conversion efficiency : experimental study and TRNSYS validation. Renew. Energy. 146, 1907–1921. doi:10.1016/j.renene.2019.07.075
Salehi, N., Mehdipour, R., Lavasani, A. M., and Yazdi, M. E. (2020). Investigating the increased heat performance of direct steam generation of Fresnel power plant using nanoparticles. Environ. Prog. Sustain. Energy, e13480. doi:10.1002/ep.13480
Sattar, A., Farooq, M., Amjad, M., Saeed, M. A., Nawaz, S., Mujtaba, M. A., et al. (2020). Performance evaluation of a direct absorption collector for solar thermal energy conversion. Energies 13 (18), 4956. doi:10.3390/en13184956
Shafieian, A., Khiadani, M., and Nosrati, A. (2019). Strategies to improve the thermal performance of heat pipe solar collectors in solar systems: a review. Energy Convers. Manag. 183, 307–331. doi:10.1016/j.enconman.2018.12.115
Sint, N. K. C., Choudhury, I. A., Masjuki, H. H., and Aoyama, H. (2017). Theoretical analysis to determine the efficiency of a CuO-water nanofluid based-flat plate solar collector for domestic solar water heating system in Myanmar. Sol. Energy. 155, 608–619. doi:10.1016/j.solener.2017.06.055
Viamajala, S., McMillan, J. D., Schell, D. J., and Elander, R. T. (2009). Rheology of corn stover slurries at high solids concentrations–effects of saccharification and particle size. Bioresour. Technol. 100 (2), 925–934. doi:10.1016/j.biortech.2008.06.070
Wang, X., He, Y., Liu, X., Shi, L., and Zhu, J. (2017). Investigation of photothermal heating enabled by plasmonic nanofluids for direct solar steam generation. Sol. Energy. 157, 35–46. doi:10.1016/j.solener.2017.08.015
Yu, J., Dang, Y., Bai, M., Peng, J., Zheng, D., Zhao, J., et al. (2019). Graphene-modified 3D copper foam current collector for dendrite-free lithium deposition. Front. in Chem. 7, 748. doi:10.3389/fchem.2019.00748
Keywords: solar energy, performance investigation, hierarchical nanostructure, TRNSYS, direct solar collector
Citation: Zain M, Amjad M, Farooq M, Anwar Z, Shoukat R, Bandarra Filho EP and Du X (2021) Performance Investigation of a Solar Thermal Collector Based on Nanostructured Energy Materials. Front. Mater. 7:617199. doi: 10.3389/fmats.2020.617199
Received: 14 October 2020; Accepted: 07 December 2020;
Published: 29 January 2021.
Edited by:
Muhammad Aftab Akram, National University of Sciences and Technology (NUST), PakistanReviewed by:
Mohsin Saleem, National University of Sciences and Technology, PakistanCopyright © 2021 Zain, Amjad, Farooq, Anwar, Shoukat, Bandarra Filho and Du. This is an open-access article distributed under the terms of the Creative Commons Attribution License (CC BY). The use, distribution or reproduction in other forums is permitted, provided the original author(s) and the copyright owner(s) are credited and that the original publication in this journal is cited, in accordance with accepted academic practice. No use, distribution or reproduction is permitted which does not comply with these terms.
*Correspondence: Muhammad Amjad, YW1qYWQ5MDAyQHVldC5lZHUucGs=
Disclaimer: All claims expressed in this article are solely those of the authors and do not necessarily represent those of their affiliated organizations, or those of the publisher, the editors and the reviewers. Any product that may be evaluated in this article or claim that may be made by its manufacturer is not guaranteed or endorsed by the publisher.
Research integrity at Frontiers
Learn more about the work of our research integrity team to safeguard the quality of each article we publish.