- 1Intelligent Polymer Research Institute, University of Wollongong, North Wollongong, NSW, Australia
- 2Shaanxi Key Laboratory of Chemical Additives for Industry, Shaanxi University of Science and Technology, Xi’an, China
High-performance electrolyte is still a roadblock for the development of rechargeable magnesium (Mg) batteries. Grignard-type electrolytes were once the only choice in the early stage of rechargeable Mg batteries research. However, due to their nucleophilic nature and high reactivity, Grignard-type electrolytes have inherent safety issues and low oxidation stability, which restrict the development of rechargeable Mg batteries in terms of practical application. Recently, emerging novel Mg battery systems such as Mg-S, Mg-O2/air batteries also require non‐nucleophilic electrolytes with high oxidation stability. This short review summarizes recent advances in non‐nucleophilic Mg electrolytes and aims to provide insights into electrochemical properties and active Mg ion structure of such electrolytes.
Introduction
After years of extensive research and evolution, rechargeable lithium-ion batteries (LIBs) have become the dominant secondary energy storage system. However, it is still a big challenge to meet the increasing demands for portable electronics and electric vehicles because of limited lithium resources. Rechargeable magnesium (Mg) batteries have been regarded as a promising candidate for novel LIBs technologies, owing to several considerable advantages (Liu et al., 2020) (Kim et al., 2020). Mg anode possesses a theoretical volumetric capacity of 3,833 mAh cm−2 that is five times higher than graphite anode–based LIB (Zhang et al., 2018). Mg has a much higher abundance than Li, ranking 8th among the most 40 abundant elements in the Earth’s crust. The nondendritic feature of Mg enables easy manufacturing of Mg metal batteries compared to Li-based battery systems. Additional clear advantages over Li-based batteries include lower cost and better safety. However, the superiority of rechargeable Mg battery has not been fully illustrated due to several inherent problems. Specifically, the actual energy density is still much lower than expected because of low discharge voltage and capacity; also, the cycle performance of the battery is extremely poor. An important reason for unsatisfying working voltage and poor cyclability of Mg-based battery is the passivation effect on Mg anode in electrolytes. Surface passivation film consists of ionic Mg compounds is formed during stripping/plating, even in nonaqueous electrolytes (Lu et al., 1999; Gofer et al., 2003). Unlike the situation in Li or Na battery, such passivation film hinders Mg2+ migration, thus resulting in high overpotential and irreversible stripping/plating (Aurbach, 1999; Attias et al., 2019).
In the early stage of rechargeable Mg batteries research, the electrolytes that allow high efficient stripping/plating were only limited to Grignard reagents and their analogs (Muldoon et al., 2012). Gregory et al. first developed Mg electrolyte with reversible deposition properties, which is based on a Grignard reagent activated by AlCl3 (Gregory et al., 1990). Since then, many researchers have focused on Mg organohaloaluminate salts generated by the complexation of Grignard reagents RMgX with AlCl3 in the ether solutions (Aurbach et al., 2002, 2003). The development of a novel rechargeable Mg-S, Mg-O2/air battery system promotes the demand for non‐nucleophilic Mg electrolytes. Sulfur and oxygen are prone to be attacked by nucleophiles, making conventional nucleophilic electrolyte incompatible for Mg-S, Mg-O2/air batteries. Even for Mg-ion battery based on intercalation chemistry, nucleophilic electrolyte has its shortcomings. Due to the use of organomagnesium precursors, most of the nucleophilic Mg electrolytes have limited oxidative stability, which restricts the use of high-voltage cathode materials. The highly sensitive and corrosive feature of Grignard-based electrolytes creates difficulties in manufacturing.
The development of Grignard reagent free, non‐nucleophilic Mg electrolyte can not only meet the requirements of rechargeable Mg-S and Mg-O2/air battery but also stepwise fulfill the requirements of practical rechargeable Mg ion battery manufacturing. In this minireview, we briefly summarized the development and recent advances of non‐nucleophilic electrolytes (Table 1). Their electrochemical performance and ionic coordination structure were discussed to provide new insights for the future development of high-performance Mg-based electrolytes.
Electrochemical Performance of Non‐nucleophilic Mg Electrolytes
Hexamethyldisilazide-Based Electrolytes
The first efficient non‐nucleophilic Mg electrolyte was prepared by adding Lewis acid AlCl3 to hexamethyldisilazide magnesium chloride (HMDSMgCl) in THF, demonstrating Coulombic efficiency (CE) of >95% with an increased Mg deposition current density by almost a factor of seven compared to HMDSMgCl-only electrolyte (Kim et al., 2011). Mg-S coin cell using HMDSMgCl-AlCl3 electrolyte displayed discharge capacity of 1,200 mAh g−1 initially; however, it decreased to 394 mAh g−1 in the following cycle. Later on, stable, commercially available (HMDS)2Mg was used to replace HMDSMgCl, which exhibited very high oxidative stability (3.5 V vs. Mg) and reversible Mg deposition up to 40 cycles with an overpotential of <150 mV (Zhao-Karger et al., 2013). To overcome the drawback of byproduct HMDSAlCl2 formation in (HMDS)2Mg-AlCl3 electrolyte, MgCl2 was introduced to convert HMDSAlCl2 into active species (Zhao-Karger et al., 2015). Such novel electrolyte displayed a discharge potential of ∼1.65 V in Mg-S battery, which was close to the theoretical thermodynamic value. Liao et al. further developed the strategy by preparing [(HMDS)MgCl2-Mg2Cl3] via reverse Schlenk equilibrium from the reaction between (HMDS)2Mg and MgCl2. The substitution of MgCl2 for Lewis acid AlCl3 successfully avoided the possible Al deposition commonly observed in AlCl3-containing electrolytes. It displayed comparable performance to HMDSMgCl-AlCl3 in terms of ionic conductivity (0.5 mS cm−1), oxidative stability (2.8 V vs. Mg), and Mg stripping/plating CE (99%) (Liao et al., 2015).
MACC Electrolytes
Using non‐nucleophilic and cheap Mg2+ sources of MgCl2, all-inorganic MgCl2-AlCl3 complex (MACC) electrolytes are of particular interest due to their simplicity and low cost. Early research on MACC electrolyte was prepared in 2005; however, it showed poor electrochemical properties with 0.9 V deposition overpotential and 37% cycling efficiency (Viestfrid et al., 2005). Several years later, R. Doe et al. demonstrated a series of MACC electrolytes in dimethoxyethane (DME) with reversible Mg deposition capability by simply increasing the MgCl2 concentration (Doe et al., 2014). One of the best among them can reach CE of at least 99%, overpotential of <200 mV, and anodic stability up to 3.1 V during 50 cycles. The MACC complex is formed based on acid-based reaction, generating solvated Mg2Cl3+ active cations, which is similar to that of the transmetalation process in Grignard-type complexes (Vestfried et al., 2007). Gewirth et al. (Barile et al., 2014) and Li et al. (Cheng et al., 2015) found that the overpotential of MACC electrolytes can be lowered by repeated CV scans or charge-discharge cycles, called the electrochemical conditioning process. Other inorganic chlorides, such as InCl3, SnCl2, SbCl3, and BiCl3, based binary chloride complex, were also investigated, which presented moderate Mg cycling performance (Barile et al., 2015). According to the retrosynthetic analysis of the Mg2+/Cl−/THF coordination cation formation process, Liu et al. developed a simple “mono-Cl abstraction” strategy to produce highly active MACC-derived electrolytes, which used MgCl2 and Al-based Lewis acid (AlCl3, AlPh3, and AlEtCl2) in THF solvent (Liu et al., 2014). The resulting electrolyte showed CE up to 100%, oxidation stability of 3.4 V, and improved electrophilic stability. A rechargeable Mg battery using Mo6S8 cathode and 0.3 M MgCl2-AlEtCl2 electrolyte delivered first discharge capacity of 104 mAh g−1 and can remain at 95.1% of the initial value after 100 cycles. By adding 0.03 M magnesium bis(trifluoromethane sulfonyl)imide [Mg(TFSI)2] to the MACC electrolyte, the electrochemical performance and water-tolerant property can be significantly improved (He et al., 2019). The CE of Mg(TFSI)2-MACC electrolyte was increased to 97%, with Mg deposition potential of ∼350 mV even without the conditioning process. A fascinating feature of the electrolyte is its moisture and impurity durability. A Mo6S8-Mg battery with such electrolyte can deliver a capacity of 92 mAh g−1 at 0.2 C after 100 cycles.
Mg(TFSI)2 and Mg(CF3SO3)2 Based Electrolytes
Mg(TFSI)2 possesses high solubility in various solvents, good ionic conductivity, and high oxidative stability. However, electrolytes using single Mg(TFSI)2 displayed large overpotential (>2.0 V) and low CE (<50%) even at high temperatures (Ha et al., 2014). Mg(TFSI)2 electrolytes were very sensitive to the impurity species, thus strongly affecting their Mg stripping/plating properties (Connell et al., 2016). Cl− can protect Mg surface from passivation by a trace amount of moisture via complex and dynamic interaction between Cl− and H2O (Connell et al., 2016). Therefore, the introduction of MgCl2 into Mg(TFSI)2 electrolytes was an effective strategy to achieve reversible stripping/plating and reduced overpotential. Li et al. first reported Mg(TFSI)2-MgCl2 combination in DME and presented improved performance of 80% CE, 400 mV overpotential, and 3.5 V anodic stability limit (Cheng et al., 2015). In addition to the consumption of water impurities, MgCl2 can also help to form [MgxCly]n+ electroactive species. Sa et al. confirmed that the active cation in Mg(TFSI)2-MgCl2/THF was dominated by the Cl− component in electrolyte (Sa et al., 2016). When the Cl−/TFSI− ratio increased from 1:5 to 1:2, CE was greatly improved from 12% to 93%; meanwhile, the average capacity in a Mo6S8-Mg rechargeable battery increased by 15%. Of note, the performance of Mg(TFSI)2 based electrolytes is highly dependent on the purity of the Mg salt and can be improved by introducing scavenging reagent other than MgCl2 (Shterenberg et al., 2015). By adding a trace amount of Mg(BH4)2 as moisture scavenger, reversible Mg stripping/plating cycling can be achieved in an Mg(TFSI)2/tetraglyme (G4) electrolyte at onset potentials around -0.35 V (plating) and 0 V (stripping), with CE up to 84% over the initial cycles and ∼75% for 500 cycles overall (Ma et al., 2017). Dimethylamine (DMA) was introduced as cosolvent to prepare a Cl-free, noncorrosive Mg(TFSI)2 based electrolyte, exhibited promising Mg stripping/plating. It showed polarization of 210 mV over 1,500 min in symmetric Mg cell cycling, which was equivalent to the performance in APC electrolyte. When using DMA-THF-G4 as mixed solvent, the CE was increased to 75% compared to 38% from an Mg(TFSI)2-G4 electrolyte (Fan et al., 2020).
Although MgCl2 containing Mg(TFSI)2 electrolytes have already been extensively studied and demonstrated good stripping/plating properties, Mg(TFSI)2 for electrolyte still requires relatively high purity and low moisture level, which caused increased cost (Gao et al., 2017). As a relative “simple” Mg salt, low-cost trifluoromethanesulfonate [Mg(CF3SO3)2, Mg(OTf)2] is commercially available with high purity and ultralow moisture, thus providing an alternative choice other than Mg(TFSI)2. A high-performance electrolyte was prepared by Mg(OTf)2-AlCl3-MgCl2 dissolved in THF and G4 mixed solvents with anthracene as a stabilizing agent, which exhibited low overpotential of 200 mV and high CE up to 98.5% without any conditioning process (Yang et al., 2019). Mg-S battery based on such electrolyte presented the highest capacity of 1,193.8 mAh g−1 and maintained a capacity of 420 mAh g−1 without fluctuations for 50 cycles. Later on, an improved Mg(OTf)2 based electrolyte was reported, by simply dissolving Mg(OTf)2, MgCl2, and AlCl3 in DME (Huang et al., 2020). It showed high efficiency of 99.1% and good anodic stability up to 3.5 V, and also demonstrated excellent performance in Mg-S battery, with a high capacity of 866 mAh g−1 at 200 mA g−1 and power density of 550 W kg−1.
Other MgCl2-Containing Electrolytes
High-performance Mg electrolytes can be achieved using simple MgCl2 salt only, with the help of proper sulfone as cosolvent. MgCl2-sulfone-THF electrolyte enabled highly reversible Mg stripping/plating, without the introduction of AlCl3, Mg(TFSI)2, or Mg(CF3SO3)2. Dipropyl sulfone (DPSO) with a melting point of 29–33°C was selected, combined with THF cosolvent and solute, successfully lowered the melting point of the resulting electrolyte below 10°C. MgCl2/DPSO-THF electrolyte also presented a typical conditioning behavior during consecutive 200 CV cycles and then underwent significant performance improvement with CE value raising from 76.2% to 92.3% (Kang et al., 2017). In the galvanostatic charge-discharge test, the CE value rapidly increased from 68.5% initially to >90% in the following 150 cycles. A Mo6S8-Mg cell using the above-mentioned electrolyte displayed excellent cycle performance up to the 300th cycle with a constant specific capacity of 70–75 mAh g−1 and high CE value (98–99%).
Boron-Centered Mg Electrolytes
Chloride-based non‐nucleophilic electrolytes are still corrosive to all cell parts to some extent. Boron- (B-) based anions are compatible with Mg anode and cell components; thus, they could be promising candidates for non‐nucleophilic electrolytes (Zhang et al., 2017). The first B-based chloride-free electrolyte developed by Mohtadi et al. was Mg(BH4)2 in THF or DME (Mohtadi et al., 2012). The Mg(BH4)2/DME showed better electrochemical performance with stripping/plating onset potential at 0.03 V/0.34 V and CE of 67%. Rechargeable Mg battery using Mg(BH4)2/DME and Mo6S8 cathode delivered reversible cycling for 40 times at 128 mA g−1. However, the reductivity of Mg(BH4)2 resulted in narrow electrochemical windows (1.7 V vs. Mg). A method for widening the electrochemical window of Mg electrolyte is to use weakly coordinating anions because the weakened coordination of anions to Mg2+ can mitigate the key step in the Mg+-mediated reductive decomposition (Lau et al., 2019). Hydroborates with B clusters with weak coordination ability, such as B12H122-, were predicted to possess improved anodic stability of 3.2 V compared to BH42-, thus suitable for potential B-based electrolyte anion (Carter et al., 2014). Unfortunately, the weakly coordinating property of B12H122- renders MgB12H12 insoluble in low-polarity, commonly used ether solvents for Mg batteries. Modified clusters of hydroborates CB11H122--based halogen-free, simple type electrolyte were prepared and exhibited high oxidative stability and improved solubility (Tutusaus et al., 2015). Due to charge reduction, CB11H122- is more lipophilic than B12H122, thus more soluble in low-polarity ethers such as triglyme (G3) and G4 (Keyzer et al., 2016). Magnesium monocarborane (MMC) salt/G4 electrolytes have a high CE of >94.4% and anodic stability up to 3.8 V (Tutusaus et al., 2015). A series of Mg salts containing weakly coordinating fluorinated alkoxyborate anions was prepared, which exhibited good solubility in aprotic solvents due to the high electronegativity of fluorine atoms. Among them, the Mg[B(hfip)4]2/DME electrolyte presented a high CE of >98% of Mg stripping/plating for over 100 cycles (Zhao-Karger et al., 2017). The anodic current density was as high as 45 mA cm−2 and oxidative voltage stability was 4.3 V. J. Luo et al. reported that magnesium fluorinated pinacolatoborate, Mg[B(O2C2(CF3)4)2]2 (Mg-FPB) based electrolyte, is noncorrosive, highly electrochemically active, and more chemically stable (Luo et al., 2019). The electrolyte delivered excellent electrochemical performance, with CE >95%, overpotential of <197 mV, and anodic stability up to 4.0 V. Mg|Mg-FPB|Mg symmetric cell can cycle at 0.1 mA cm−2 for more than 500 h, with stable polarization.
Active Mg Cations for Reversible Deposition
It is still a big challenge to use simple Mg salts to obtain a high-performance Mg electrolyte. Ultrahigh purity is required because even residual moisture can cause the formation of a passivation layer that will inhibit reversible Mg stripping/plating. Of note, Cl-based electrolytes and most of the B-centered electrolytes exhibited good electrochemical performance without rigorous purification. It is believed that Mg-coordinated ions play a vital role in determining the performance of electrolytes (Shao et al., 2013).
An early study on the mechanism of Grignard-type electrolytes suggested that the active cation was [MgCl(THF)5]+ based on an electrochemical quartz crystal microbalance (EQCM) study (Aurbach et al., 2001). In the first reported HMDSCl-based non‐nucleophilic electrolyte, [Mg2(μ-Cl)3(THF)6][HMDSAlCl3] crystal was isolated and determined by single-crystal X-ray diffraction. It is reasonable to presume that [Mg2(μ-Cl)3(THF)6]+ was the active species because a similar cation has already been identified in previously reported Grignard-type electrolytes (Aurbach et al., 2003). The cation has two Mg octahedral coordination centers, three bridging Cl atoms (μ-Cl), and six coordination O atoms from THF. Since then, [Mg2(μ-Cl)3(THF)6]+ became the most commonly accepted active cation in the Cl-THF electrolyte system (Liao et al., 2015)(Barile et al., 2014). See et al. (2016) compared the ion structure in as-prepared and conditioned MACC electrolyte to identify the active species (See et al., 2016). Surface-enhanced Raman spectroscopy (SERS), 27Al and 35Cl NMR, and X-ray pair distribution function (PDF) were applied, indicating that the active specie in MACC electrolyte both before and after conditioning was [Mg2(μ-Cl)3(THF)6]+ (Esbenshade et al., 2015). [Mg2(μ-Cl)3(THF)6]+ also existed in Mg(TFSI)2-MgCl2 electrolyte as confirmed by single-crystal XRD (Sa et al., 2016) and is predicted to be present in Mg(CF3SO3)2-MgCl2 electrolyte (Yang et al., 2019). One possible Mg deposition pathway via [Mg2(μ-Cl)3(THF)6]+, as proposed based on electrochemical and in situ X-ray absorption spectroscopy measurement, was shown in Figure 1A (Benmayza et al., 2013). Solvent molecule plays a vital role in forming the active Mg coordination ions. A [Mg2(µ-Cl)2(DME)4]2+ complex cation was present in MACC/DME and Mg(TFSI)2-MgCl2/DME, confirmed by single-crystal XRD (Cheng et al., 2015). The cation complex consists of two octahedrally coordinated Mg centers, each of which is bridged by two chlorine atoms and coordinated by four oxygen atoms from DME. The formation of solvated Mg2Cl3+ is not thermodynamically favored in DME because of the difficulties in obtaining coordination number of six (Mizrahi et al., 2008). ESI-MS revealed that the coordination ion structure changed during the conditioning process in a Mg(CF3SO3)2-MgCl2-AlCl3 electrolyte. Most of the [Mg2(μ-Cl)2(DME)4]2+ cations were converted to [Mg3(μ3-Cl)(μ2-Cl)2(DME)7]3+ due to increase of Mg/Al ratio caused by Mg/Al codeposition (Huang et al., 2020).
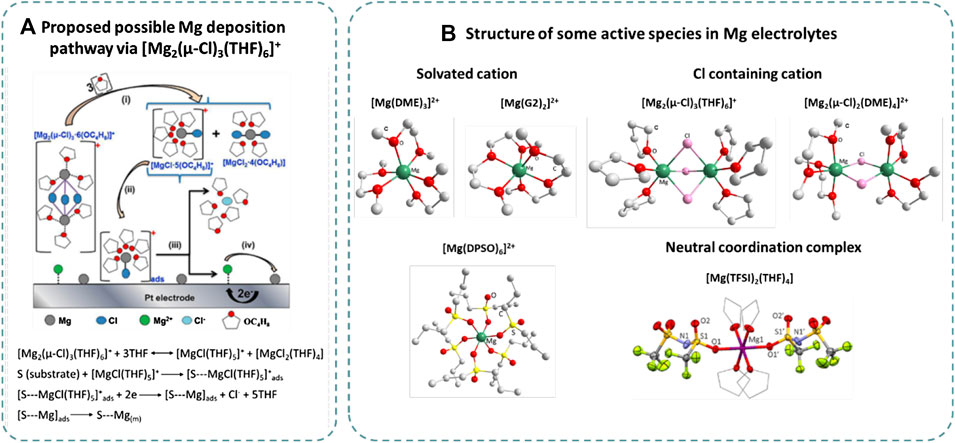
FIGURE 1. (A) Proposed Mg deposition mechanism via [Mg2(μ-Cl)3(THF)6]+ (Benmayza et al., 2013) and (B) structure of active species in non‐nucleophilic Mg electrolyte (Cheng et al., 2015) (Liu et al., 2014) (Fan et al., 2020) (Kang et al., 2017) (Tutusaus et al., 2015), reproduced with permission.
Chloride-free B-centered electrolytes have very different electroactive cation structures, which are mainly solvated Mg cation paired with B-based anion. In the first reported Mg(BH4)2-DME electrolyte, it was proposed that Mg(BH4)2 was present in the form of Mg[(μ-H)2BH2]2, which could partially convert into [Mg(μ-H)2BH2]+ and BH4- (Mohtadi et al., 2012). Then, further dissociation occurs to generate Mg2+ and BH4-. The electrochemical performance of Mg(BH4)2 electrolyte can be further enhanced by the addition of LiBH4, owing to weakened association within the ion pair as evidenced by IR spectrum. The CE was improved with increasing molar ratio of LiBH4/Mg(BH4)2. The ion species in Mg(CB11H12)2 electrolytes were more complicated. [Mg(THF)6](CB11H12)2, [Mg(DME)3](CB11H12)2, and [Mg(diglyme)2] (CB11H12)2 can be collected as precipitates and were unfortunately unable to be redissolved (Tutusaus et al., 2015). The above solids could only be redissolved in larger glymes such as G3 and G4. The successful isolation of [Mg(G4)2(H2O)](CB11H12)2 characterized by single-crystal XRD indicates that [Mg(Gx)2]2+ (x = 3, 4) is highly possible to be the active Mg ion. Similarly, [Mg(DME)3]2+ paired by counterion [B(hfip)4]2- was isolated and verified in Mg[B(hfip)4]/DME electrolyte (Zhao-Karger et al., 2017). It can be redissolved in DME to allow highly efficient Mg stripping/plating. [Mg(G2)2]2+ was determined by single-crystal XRD in a noncorrosive magnesium fluorinated pinacolatoborate Mg[B(O2C2(CF3)4)2]2-G2 electrolyte (Luo et al., 2019).
Employing cosolvents can tune the Mg coordination ion formation, thus affecting the electrolyte properties. In a MgCl2/DPSO-THF electrolyte, [Mg(DPSO)6]2+ cation complex was identified as active Mg ion, coupled by two [MgCl3(THF)]- anions (Kang et al., 2017). The cosolvent THF can help form more stable anion [MgCl3(THF)]- with weakened coordinating ability, thus allowing Mg2+ to be solvated solely by DPSO. The unique structures of such novel active ion enabled highly efficient reversible Mg deposition/dissolution. Mg(HMDS)2-MgCl2 based on certain sulfone/THF mixed solvent exhibited comparable Mg deposition reversibility and much higher thermal stability than that of pure THF-based counterparts (Merrill and Schaefer, 2018). The solvated Mg2Cl3+/MgCl+ ratio, which is affected by cosolvent type, was the key factor in anode reversibility. Butyl sulfone-/THF-based electrolyte with higher Mg2Cl3+/MgCl+ presented facilitated reversible Mg deposition than that of sulfolane/THF electrolyte. When dimethylamine (DMA) was introduced into a Mg(TFSI)2/THF-G4 electrolyte, the CE can be significantly improved. A neutral coordination compound Mg(TFSI)2(THF)4 was identified, which successfully reduced the effective charge and accelerated the kinetics for reversible Mg deposition (Fan et al., 2020). It was suggested that the cosolvent DMA participated in the formation of the ion pair in the solvation shell by Mg···N interaction.
Conclusion Remarks
Non‐nucleophilic electrolytes have become new trends in Mg electrolyte research, especially considering the rapid development of Mg-S and Mg-O2/air battery in recent years. Although many of the drawbacks in conventional Grignard-based electrolytes have been overcome by emerging non‐nucleophilic electrolytes, it is still very challenging to prepare Mg electrolytes for practical application. Cl-based electrolytes including MACC, Mg(TFSI)2-MgCl2, and (HMDS)2Mg-MgCl2 have good processability and excellent performance but are still corrosive to many current collectors and cell components, especially when strong Lewis acid AlCl3 was involved. In Cl-free B-centered electrolytes, Mg(BH4)2 suffered from low anodic stability and limited solubility. Magnesium carbaborate and alkoxyborate originating from Mg(BH4)2 precursor have a wide electrochemical window, albeit with a complicated synthetic procedure. There are some common disadvantages of most Mg electrolytes, such as high raw materials price, moisture sensitivity, and long-term preconditioning behavior. Another key issue in the Mg electrolyte research is understanding the mechanism of Mg stripping/plating, including anode-electrolyte interfacial chemistry, Mg coordination chemistry, and Mg cation electrochemistry. Although there have already been rational hypotheses presented with support from theoretical simulation and experimental results, researchers still need to explore the chemical and electrochemical process during reversible Mg deposition in-depth.
In summary, developing non‐nucleophilic, noncorrosive, less air-/moisture-sensitive electrolyte with wide electrochemical window and high Mg deposition reversibility is preferred to promote the development of practical rechargeable Mg batteries. It is highly recommended to prepare Mg electrolyte from low-cost, commercially available materials via a facile procedure. Further investigation is also needed to better understand the ion structure of electrolyte, electrode-electrolyte interface, and their effect on the electrochemical performance.
Author Contributions
All authors have made a substantial, direct, and intellectual contribution to the work and approved it for publication.
Conflict of Interest
The authors declare that the research was conducted in the absence of any commercial or financial relationships that could be construed as a potential conflict of interest.
Acknowledgments
The authors sincerely acknowledge the National Natural Science Foundation of China (Nos. 52003147, 21978164, 21808249, and 21806097); Key Research and Development Program of Shaanxi Province (No. 2020GY-243); Innovation Supporting Plan of Shaanxi Province-Innovation Research Team (No. 2018TD-015); National High-End Foreign Expert Project (No. GDW20186100428); Industrialization Project of Shaanxi Education Department (No. 19JC010).
References
Attias, R., Salama, M., Hirsch, B., Goffer, Y., and Aurbach, D. (2019). Anode-electrolyte interfaces in secondary magnesium batteries. Joule 3, 27–52. doi:10.1016/j.joule.2018.10.028
Aurbach, D. (1999). “Chapter 6: the electrochemical behavior of active metal electrodes in nonaqueous solutions,” in Nonaqueous Electrochemistry. Hoboken, NJ: Wiley. 296–301. doi:10.1002/3527600655
Aurbach, D., Gizbar, H., Schechter, A., Chusid, O., Gottlieb, H. E., Gofer, Y., et al. (2002). Electrolyte solutions for rechargeable magnesium batteries based on organomagnesium chloroaluminate complexes. J. Electrochem. Soc. 149, A115. doi:10.1149/1.1429925
Aurbach, D., Schechter, A., Moshkovich, M., and Cohen, Y. (2001). On the mechanisms of reversible magnesium deposition processes. J. Electrochem. Soc. 148, A1004. doi:10.1149/1.1387980
Aurbach, D., Weissman, I., Gofer, Y., and Levi, E. (2003). Nonaqueous magnesium electrochemistry and its application in secondary batteries. Chem. Rec. 3, 61–73. doi:10.1002/tcr.10051
Barile, C. J., Barile, E. C., Zavadil, K. R., Nuzzo, R. G., and Gewirth, A. A. (2014). Electrolytic conditioning of a magnesium aluminum chloride complex for reversible magnesium deposition. J. Phys. Chem. C. 118, 27623–27630. doi:10.1021/jp506951b
Barile, C. J., Nuzzo, R. G., and Gewirth, A. A. (2015). Exploring salt and solvent effects in chloride-based electrolytes for magnesium electrodeposition and dissolution. J. Phys. Chem. C. 119, 13524–13534. doi:10.1021/acs.jpcc.5b03508
Benmayza, A., Ramanathan, M., Arthur, T. S., Matsui, M., Mizuno, F., Guo, J., et al. (2013). Effect of electrolytic properties of a magnesium organohaloaluminate electrolyte on magnesium deposition. J. Phys. Chem. C. 117, 26881–26888. doi:10.1021/jp4077068
Carter, T. J., Mohtadi, R., Arthur, T. S., Mizuno, F., Zhang, R., Shirai, S., et al. (2014). Boron clusters as highly stable magnesium-battery electrolytes. Angew. Chemie Int. Ed. 53, 3173–3177. doi:10.1002/anie.201310317
Cheng, Y., Stolley, R. M., Han, K. S., Shao, Y., Arey, B. W., Washton, N. M., et al. (2015). Highly active electrolytes for rechargeable Mg batteries based on a [Mg2(μ-Cl)2]2+ cation complex in dimethoxyethane. Phys. Chem. Chem. Phys. 17, 13307–13314. doi:10.1039/C5CP00859J
Connell, J. G., Genorio, B., Lopes, P. P., Strmcnik, D., Stamenkovic, V. R., and Markovic, N. M. (2016). Tuning the reversibility of Mg anodes via controlled surface passivation by H2O/Cl– in organic electrolytes. Chem. Mater. 28, 8268–8277. doi:10.1021/acs.chemmater.6b03227
Doe, R. E., Han, R., Hwang, J., Gmitter, A. J., Shterenberg, I., Yoo, H. D., et al. (2014). Novel, electrolyte solutions comprising fully inorganic salts with high anodic stability for rechargeable magnesium batteries. Chem. Commun. 50, 243–245. doi:10.1039/C3CC47896C
Esbenshade, J. L., Barile, C. J., Fister, T. T., Bassett, K. L., Fenter, P., Nuzzo, R. G., et al. (2015). Improving electrodeposition of Mg through an open circuit potential hold. J. Phys. Chem. C. 119, 23366–23372. doi:10.1021/acs.jpcc.5b07825
Fan, S., Asselin, G. M., Pan, B., Wang, H., Ren, Y., Vaughey, J. T., et al. (2020). A simple halogen-free magnesium electrolyte for reversible magnesium deposition through cosolvent assistance. ACS Appl. Mater. Interfaces 12, 10252–10260. doi:10.1021/acsami.9b18833
Gao, T., Hou, S., Wang, F., Ma, Z., Li, X., Xu, K., et al. (2017). Reversible S0/MgSx redox chemistry in a MgTFSI2/MgCl2/DME electrolyte for rechargeable Mg/S batteries. Angew. Chemie Int. Ed. 56, 13526–13530. doi:10.1002/anie.201708241
Gofer, Y., Turgeman, R., Cohen, H., and Aurbach, D. (2003). XPS investigation of surface chemistry of magnesium electrodes in contact with organic solutions of organochloroaluminate complex salts. Langmuir 19, 2344–2348. doi:10.1021/la026642c
Gregory, T. D., Hoffman, R. J., and Winterton, R. C. (1990). Nonaqueous electrochemistry of magnesium: applications to energy storage. J. Electrochem. Soc. 137, 775–780. doi:10.1149/1.2086553
Ha, S.-Y., Lee, Y.-W., Woo, S. W., Koo, B., Kim, J.-S., Cho, J., et al. (2014). Magnesium(II) bis(trifluoromethane sulfonyl) imide-based electrolytes with wide electrochemical windows for rechargeable magnesium batteries. ACS Appl. Mater. Interfaces 6, 4063–4073. doi:10.1021/am405619v
He, Y., Li, Q., Yang, L., Yang, C., and Xu, D. (2019). Electrochemical-conditioning-free and water-resistant hybrid AlCl3/MgCl2/Mg(TFSI)2 electrolytes for rechargeable magnesium batteries. Angew. Chemie Int. Ed. 58, 7615–7619. doi:10.1002/anie.201812824
Huang, D., Tan, S., Li, M., Wang, D., Han, C., An, Q., et al. (2020). Highly efficient non-nucleophilic Mg(CF3SO3)2-based electrolyte for high-power Mg/S battery. ACS Appl. Mater. Interfaces 12, 17474–17480. doi:10.1021/acsami.0c00196
Kang, S.-J., Lim, S.-C., Kim, H., Heo, J. W., Hwang, S., Jang, M., et al. (2017). Non-grignard and Lewis acid-free sulfone electrolytes for rechargeable magnesium batteries. Chem. Mater. 29, 3174–3180. doi:10.1021/acs.chemmater.7b00248
Keyzer, E. N., Glass, H. F. J., Liu, Z., Bayley, P. M., Dutton, S. E., Grey, C. P., et al. (2016). Mg(PF6)2-Based electrolyte systems: understanding electrolyte–electrode interactions for the development of Mg-ion batteries. J. Am. Chem. Soc. 138, 8682–8685. doi:10.1021/jacs.6b04319
Kim, H. S., Arthur, T. S., Allred, G. D., Zajicek, J., Newman, J. G., Rodnyansky, A. E., et al. (2011). Structure and compatibility of a magnesium electrolyte with a sulphur cathode. Nat. Commun. 2, 427. doi:10.1038/ncomms1435
Kim, K., Guo, Q., Tang, L., Zhu, L., Pan, C., Chang, C., et al. (2020). Reversible insertion of Mg-Cl superhalides in graphite as a cathode for aqueous dual-ion batteries. Angew. Chemie Int. Ed. 59, 19924–19928. doi:10.1002/anie.202009172
Lau, K.-C., Seguin, T. J., Carino, E. V, Hahn, N. T., Connell, J. G., Ingram, B. J., et al. (2019). Widening electrochemical window of Mg salt by weakly coordinating perfluoroalkoxyaluminate anion for Mg battery electrolyte. J. Electrochem. Soc. 166, A1510–A1519. doi:10.1149/2.0751908jes
Liao, C., Sa, N., Key, B., Burrell, A. K., Cheng, L., Curtiss, L. A., et al. (2015). The unexpected discovery of the Mg(HMDS)2/MgCl2 complex as a magnesium electrolyte for rechargeable magnesium batteries. J. Mater. Chem. 3, 6082–6087. doi:10.1039/C5TA00118H
Liu, F., Wang, T., Liu, X., and Fan, L.-Z. (2020). Challenges and recent progress on key materials for rechargeable magnesium batteries. Adv. Energy Mater. 2000787. doi:10.1002/aenm.202000787
Liu, T., Shao, Y., Li, G., Gu, M., Hu, J., Xu, S., et al. (2014). A facile approach using MgCl2 to formulate high performance Mg2+ electrolytes for rechargeable Mg batteries. J. Mater. Chem. 2, 3430–3438. doi:10.1039/C3TA14825D
Lu, Z., Schechter, A., Moshkovich, M., and Aurbach, D. (1999). On the electrochemical behavior of magnesium electrodes in polar aprotic electrolyte solutions. J. Electroanal. Chem. 466, 203–217. doi:10.1016/S0022-0728(99)00146-1
Luo, J., Bi, Y., Zhang, L., Zhang, X., and Liu, T. L. (2019). A stable, non-corrosive perfluorinated pinacolatoborate Mg electrolyte for rechargeable Mg batteries. Angew. Chemie Int. Ed. 58, 6967–6971. doi:10.1002/anie.201902009
Ma, Z., Kar, M., Xiao, C., Forsyth, M., and MacFarlane, D. R. (2017). Electrochemical cycling of Mg in Mg[TFSI]2/tetraglyme electrolytes. Electrochem. commun. 78, 29–32. doi:10.1016/j.elecom.2017.03.018
Merrill, L. C., and Schaefer, J. L. (2018). Conditioning-free electrolytes for magnesium batteries using sufone–ether mixtures with increased thermal stability. Chem. Mater. 30, 3971–3974. doi:10.1021/acs.chemmater.8b00483
Mizrahi, O., Amir, N., Pollak, E., Chusid, O., Marks, V., Gottlieb, H., et al. (2008). Electrolyte solutions with a wide electrochemical window for rechargeable magnesium batteries. J. Electrochem. Soc. 155, A103. doi:10.1149/1.2806175
Mohtadi, R., Matsui, M., Arthur, T. S., and Hwang, S.-J. (2012). Magnesium borohydride: from hydrogen storage to magnesium battery. Angew. Chemie Int. Ed. 51, 9780–9783. doi:10.1002/anie.201204913
Muldoon, J., Bucur, C. B., Oliver, A. G., Sugimoto, T., Matsui, M., Kim, H. S., et al. (2012). Electrolyte roadblocks to a magnesium rechargeable battery. Energy Environ. Sci. 5, 5941–5950. doi:10.1039/C2EE03029B
Sa, N., Pan, B., Saha-Shah, A., Hubaud, A. A., Vaughey, J. T., Baker, L. A., et al. (2016). Role of chloride for a simple, non-grignard Mg electrolyte in ether-based solvents. ACS Appl. Mater. Interfaces 8, 16002–16008. doi:10.1021/acsami.6b03193
See, K. A., Chapman, K. W., Zhu, L., Wiaderek, K. M., Borkiewicz, O. J., Barile, C. J., et al. (2016). The interplay of Al and Mg speciation in advanced Mg battery electrolyte solutions. J. Am. Chem. Soc. 138, 328–337. doi:10.1021/jacs.5b10987
Shao, Y., Liu, T., Li, G., Gu, M., Nie, Z., Engelhard, M., et al. (2013). Coordination Chemistry in magnesium battery electrolytes: how ligands affect their performance. Sci. Rep. 3, 3130. doi:10.1038/srep03130
Shterenberg, I., Salama, M., Yoo, H. D., Gofer, Y., Park, J.-B., Sun, Y.-K., et al. (2015). Evaluation of (CF3SO2)2N−(TFSI) based electrolyte solutions for Mg batteries. J. Electrochem. Soc. 162, A7118–A7128. doi:10.1149/2.0161513jes
Tutusaus, O., Mohtadi, R., Arthur, T. S., Mizuno, F., Nelson, E. G., and Sevryugina, Y. V. (2015). An efficient halogen-free electrolyte for use in rechargeable magnesium batteries. Angew. Chemie Int. Ed. 54, 7900–7904. doi:10.1002/anie.201412202
Vestfried, Y., Chusid, O., Goffer, Y., Aped, P., and Aurbach, D. (2007). Structural analysis of electrolyte solutions comprising Magnesium−Aluminate Chloro−Organic complexes by Raman spectroscopy. Organometallics 26, 3130–3137. doi:10.1021/om061076s
Viestfrid, Y., Levi, M. D., Gofer, Y., and Aurbach, D. (2005). Microelectrode studies of reversible Mg deposition in THF solutions containing complexes of alkylaluminum chlorides and dialkylmagnesium. J. Electroanal. Chem. 576, 183–195. doi:10.1016/j.jelechem.2004.09.034
Yang, Y., Wang, W., Nuli, Y., Yang, J., and Wang, J. (2019). High active magnesium trifluoromethanesulfonate-based electrolytes for magnesium–sulfur batteries. ACS Appl. Mater. Interfaces 11, 9062–9072. doi:10.1021/acsami.8b20180
Zhang, Z., Cui, Z., Qiao, L., Guan, J., Xu, H., Wang, X., et al. (2017). Novel design concepts of efficient Mg-ion electrolytes toward high-performance magnesium–selenium and magnesium–sulfur batteries. Adv. Energy Mater. 7, 1602055. doi:10.1002/aenm.201602055
Zhang, Z., Dong, S., Cui, Z., Du, A., Li, G., and Cui, G. (2018). Rechargeable magnesium batteries using conversion-type cathodes: a perspective and minireview. Small Methods 2, 1800020. doi:10.1002/smtd.201800020
Zhao-Karger, Z., Gil Bardaji, M. E., Fuhr, O., and Fichtner, M. (2017). A new class of non-corrosive, highly efficient electrolytes for rechargeable magnesium batteries. J. Mater. Chem. 5, 10815–10820. doi:10.1039/C7TA02237A
Zhao-Karger, Z., Zhao, X., Fuhr, O., and Fichtner, M. (2013). Bisamide based non-nucleophilic electrolytes for rechargeable magnesium batteries. RSC Adv. 3, 16330–16335. doi:10.1039/C3RA43206H
Keywords: Mg electrolyte, rechargeable Mg battery, non‐nucleophilic, coordination Mg complex, energy storage
Citation: Wu Q, Shu K, Sun L and Wang H (2021) Recent Advances in Non‐nucleophilic Mg Electrolytes. Front. Mater. 7:612134. doi: 10.3389/fmats.2020.612134
Received: 30 September 2020; Accepted: 17 November 2020;
Published: 14 January 2021.
Edited by:
Anastasiia O. Krushynska, University of Groningen, NetherlandsReviewed by:
Guosheng Li, Pacific Northwest National Laboratory (DOE), United StatesXing-Long Wu, Northeast Normal University, China
Copyright © 2021 Wu, Shu, Sun and Wang. This is an open-access article distributed under the terms of the Creative Commons Attribution License (CC BY). The use, distribution or reproduction in other forums is permitted, provided the original author(s) and the copyright owner(s) are credited and that the original publication in this journal is cited, in accordance with accepted academic practice. No use, distribution or reproduction is permitted which does not comply with these terms.
*Correspondence: Kewei Shu, shukw@sust.edu.cn; Haihua Wang, whh@sust.edu.cn