- 1Hoffmann Institute of Advanced Materials, Shenzhen Polytechnic, Shenzhen, China
- 2Department of Materials Science and Engineering, Southern University of Science and Technology, Shenzhen, China
- 3New York University Abu Dhabi, Abu Dhabi, United Arab Emirates
The organic-inorganic hybrid perovskite solar cells with its great advances in the cost-efficient fabrication process and high-power conversion efficiency have outperformed a range of traditional photovoltaic technologies such as multi-crystal Si and CIGS. Meanwhile, the undesirable operational stability of perovskite solar cell lags its commercialization where perovskite solar cells suffer a lattice degradation and lost the capability of energy harvesting when encountering the crucial environmental factors such as high moisture and strong irradiation. Accordingly, improving the operational stability becomes one of the decisive factors to govern the next wave advancement of the perovskite solar cells. Among a plethora of reported strategies to improve the stability, building a multidimensional (2D/3D) heterojunction perovskite as the light-harvesting layer has recently become one of the most credible approaches to stabilize the PSCs without sacrificing of photovoltaic performance. In this mini-review, the recent progress in 2D/3D multidimensional PSCs has been elaborately reviewed. Detailed information including the long-chain cation materials, development of fabrication process, charge carrier dynamics, optoelectronic properties, and their impact on the photovoltaic performances has been systematically discussed. Finally, some of the further challenges are highlighted while outlining the perspectives of multidimensional 2D/3D perovskites for stable and high-performance PSCs.
Introduction
Organic-inorganic hybrid metal halide perovskite materials have shown lots of promising optoelectronic properties including high absorption coefficient, low exciton binding energy and high defect tolerance (Yan et al., 2018; Kim et al., 2020). Currently, the highest power conversion efficiency (PCE) of three-dimensional (3D) PSCs surpassed 25% (Li and Zhang, 2020), which is comparable with that of commercial silicon solar cells. However, poor stability is the main limitation hindering the commercialization of 3D PSCs. Researchers have devoted tremendous effort to enhance the stability of 3D PSCs and obtained inspiring progress through various approaches such as compositional engineering, interfacial regulation, defects passivation, device encapsulation, and so on (Zheng et al., 2017; Li et al., 2018a; Li and Zhang, 2020). Among them, the introduction of long-chain hydrophobic organic cations based two-dimensional (2D) perovskites is one potential strategy for the stability improvement specified as 2D/3D multidimensional heterojunction perovskites, which secures the long-term stability and high performance simultaneously (Zhang et al., 2018; Krishna et al., 2019). According to the recent studies, the long-chain hydrophobic and bulky organic spacer in 2D perovskites can effectively prevent the moisture adsorption and invasion at the perovskite surface, thereby retard the decomposition at the initial step (Ortiz-Cervantes et al., 2019; Zhang et al., 2020). Whereas, perovskites with pure 2D crystal structures are generally not desirable for high-performance PSCs, own to their wide band gap and non-preferred crystallographic orientation where the former reduces the overall high harvesting and letter retards the vertical charge transfers (that is, the dissociated charge carriers injects into charge transport layers) (Zhang et al., 2020). Therefore, it is very essential to optimize the composition and orientation of the 2D/3D multidimensional perovskites and to combine the 2D perovskite with great moisture tolerances and 3D perovskite with distinct charge carrier dynamics to achieve the facile manipulation in the advanced PSCs. In this mini-review, we will mainly concentrate on the application of 2D/3D multidimensional perovskites in solar cells. First, we will give a introduction to the structural and optoelectronic properties of 2D perovskites. Then, the recent advances of 2D/3D multidimensional PSCs are systematically discussed in two different aspects based on the configuration of perovskite materials in the device. Finally, we will address some current challenges and give the perspectives for 2D/3D perovskites for PSCs with high efficiency and good stability.
Structure and Properties of 2D Perovskites
In 1957, Ruddlesden and Popper firstly reported A2BO4 type compounds where the perovskites exhibit a layered crystal structure. In the metal-halide perovskites, a layered perovskite also adopts the same definition, namely Ruddlesden–Popper perovskites (Yan et al., 2018). The formula of such Ruddlesden–Popper layered perovskites is (RNH3)An-1BnX3n+1 (n = 1, 2, 3, to ∞), where An-1BnX3n+1 is the conductor layer of common 3D perovskite, including methylammonium (MA) lead iodide (MAPbI3), formamidinium (FA) lead iodide (FAPbI3), and cesium (Cs) lead iodide (CsPbI3). The 3D perovskites are separated from one another by the introduction of R–NH3, a long-chain aliphatic or aromatic alkylammonium cation, including phenyl-ethyl ammonium (PEA) and butylammonium (BA) (Zhang et al., 2018; Zhang et al., 2020). The n value in the formula represents the thickness or the number of layers of 3D perovskites which is tunable via controlling the stoichiometry of the precursor solutions. Compared with the 3D perovskites, the 2D layered perovskites possess some unique properties (Yan et al., 2018; Krishna et al., 2019; Ortiz-Cervantes et al., 2019). First, the hydrophobic nature of 2D R cations and the highly oriented structure enables the superior moisture resistance of 2D layered perovskites. Second, 2D perovskites have narrower absorption owing to their larger bandgaps, which varies with the number of 2D layers. Third, the insulation nature of the bulky organic cations with low conductivity of the 2D perovskites induces a multiple-pseudo quantum-well structure where the exciton dissociation is retarded caused by quantum confinement effect associated high exciton binding energy (Grancini and Nazeeruddin, 2019). Moreover, the charge transport in 2D layered perovskites is anisotropic and highly dependent on the orientation of the 2D layered structure (Wang et al., 2019; Zhang et al., 2020). The charge mobility is much better along the in-plane inorganic perovskites sheets than out of plane organic sheets. The difficulty of out-of-plane charge transfer comes from the high interfacial resistance and thus perturbed charge carrier transport between 3D and 2D perovskite contacts (Wang et al., 2019). Furthermore, the material engineering of 2D perovskites can be easily implemented not only by alternating the composition of perovskite layers but also by the molecular design of the spacer cations, such as ammonium dications alkyl chain length, and introduction of the p-conjugated segment (Jagielski et al., 2017; Lan et al., 2019). Compared to 3D perovskites, there is a broad possibility of 2D perovskites in photo-physical investigation and practical devices applications. Figure 1 shown the chemical structure of the reported long organic-chain cations employed for the construction of 2D/3D multidimensional perovskites.
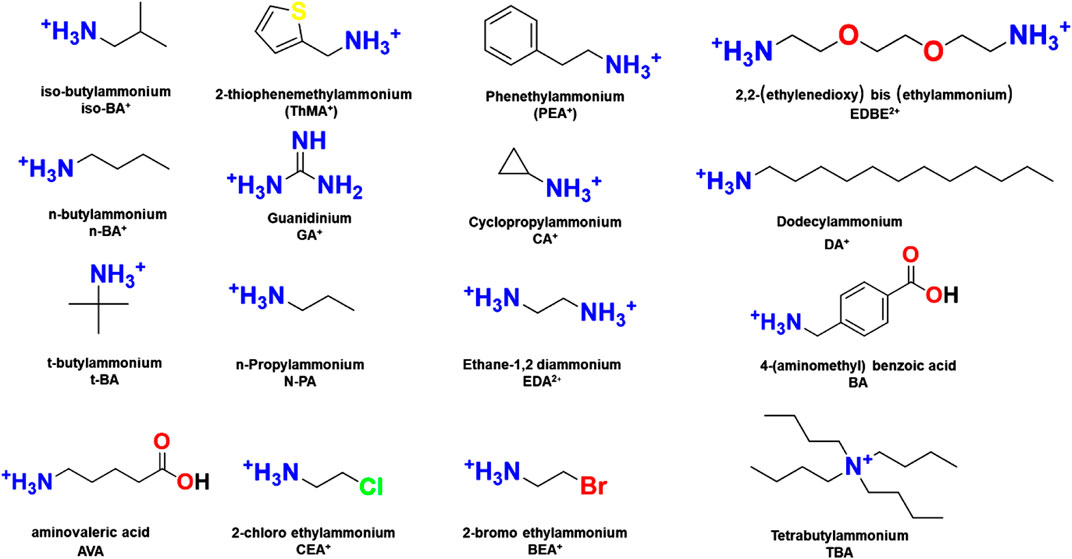
FIGURE 1. Chemical structure of the reported organic cations employed for the construction of 2D/3D multidimensional perovskites.
Mixed 2D/3D Multidimensional PSCs
The concept of mixed 2D/3D multidimensional perovskites based PSCs was first proposed by Karunadasa et al. in 2014, in which a large PEA was mixed MA cations to form a Ruddlesden–Popper structure with the composition of (PEA)2(MA)2[Pb3I10] (n = 3) (Smith et al., 2014). The bandgap of the corresponding 2D and 3D perovskite was 2.10, 1.63 eV, respectively. The (PEA)2(MA)2[Pb3I10] based device displayed a high Voc of 1.18 V but a low PCE of 4.73%. Moreover, this 2D/3D mixed perovskite film exhibited good long-term stability against moisture over 46 days of air exposure with relative humidity (RH) of 52%, while MAPbI3 was completely decomposed under the same condition. Inspired by this research, Guo and his co-workers applied a low-pressure vapor-assisted solution deposition approach (LP-VASP) to prepare a series of 2D/3D hybrid perovskite films by the chemical reaction between MAI vapor and the as-prepared PEAI-doped PbI2 film and in which the ratio of PEAI/PbI2 was tuned from 2 to 0 (Li et al., 2018b). The champion device (PEAI/PbI2 = 0.05) achieves a PCE of 19.10% with a Jsc of 21.91 mA cm−2, a Voc of 1.08 V, and a remarkable fill factor (FF) of 80.36%. Similarly, Hu et al. incorporated PEA in the 2D/3D Pb-Sn alloyed perovskite solar cells through the anti-solvent engineering method and obtained a maximum PCE of 15.93% for the 2D/3D PEAxMA1-xPb0.5Sn0.5I3 based device (Zhang and Hu, 2020). According to their study, the enhanced photovoltaic performance can mainly attribute to the following reasons: the improved spin-orbit coupling (SOC) proved by photoexcitation-polarization dependent photocurrent studies benefits the charge dissociation; the enhanced out-of-plane photoinduced bulk polarization with reduced traps, evidenced by photoinduced impedance measurements and polarization-direction dependent photoluminescence (PL) characteristics, will align the optical transition dipoles and decrease nonradiative recombination loss. Meanwhile, based on the theoretical calculation by Jen’s group, the transition energy form the black phase to the yellow phase of FAPbI3 was raised due to the introduction of FEA+, indicating the improved phase stability of FAPbI3 (Li et al., 2017). Moreover, the hydrophobic PEA+ and I− can passivate the defects at lattice surface and grain boundaries, improving both performance and ambient stability. Nazeeruddin and co-workers reported 1-year stable PSCs with an exceptional gradually organized multidimensional interface at their 2D/3D perovskite junction, yielding the best PCE of 11.2% and stability for >10,000 h without any loss (Grancini et al., 2017). Unlike the work mentioned above, the 2D perovskite formed by self-assemble on the network of metal oxide layer after spin coating the precursor solution containing a small amount (3% molar ratio) of aminovaleric acid iodide (AVAI). The carboxylic acid group of the aminovaleric acid iodide facilitated the self-assembly of the 2D perovskite phase onto the scaffold of TiO2. Moreover, the oriented growth of bulk 3D perovskite grain also can be promoted by this interface. As a result, this 2D/3D interface brings together the excellent stability of 2D perovskites and good charge transport of the 3D ones. Gao et al. presented a simple drop-casting approach to develop hybrid quasi-2D/3D perovskite films by employing PEA and iso-butylammonium (iso-BA) as spacer cations (Zuo et al., 2020). The crystal orientation, film morphology, and phase purity of the hybrid quasi-2D/3D perovskite films can be improved significantly via a simple N2 blow-drying process, involving methylammonium chloride (MACl) as an additive. Furthermore, an enhanced PCE of 16.0% is obtained and retains 91% of the initial value after 500 h with continuous one-sun illumination for an encapsulated device, indicating good durability. For the most case, researchers mainly focus on how the incorporated 2D perovskite materials affect the light and moisture stability rather than the thermal stability of the mixed 2D/3D multidimensional perovskites. Mora-Seró et al. introduced dipropylammonium iodide (DipI) as cation to form 2D/3D perovskite materials with the general formula Dip2MAn−1PbnI3n+1, where n = 3, 5, 10, 50, and 90. They evaluated the thermal stability by IR spectroscopy and the mixed perovskite material (n = 10) exhibited over 30% of the perovskite phase while 3D perovskite decomposed completely at 120°C for 240 min (Rodríguez-Romero et al., 2020). The Apart from PEAI and BAI, 2-thiophenemethylammonium (ThMA) (Zhou et al., 2019), n-propylammonium iodide (Yao et al., 2019), 1-(ammonium acetyl) pyrene (PEY) (Yang et al., 2018), ethane-1,2-diammonium (EDA) (Lu et al., 2017), dodecyl ammonium-chloride (DACl) (Ali et al., 2019), 2-choloro-ethylamine (CEA) and 2-bromo-ethylamine (BEA) (Liu et al., 2019), 4-(aminomethyl) benzoic acid hydroiodide (AB) (Hu et al., 2018b) have also been successfully applied to assemble 2D/3D heterojunctions to modify the PSCs, which has been proved to improve photovoltaic performance, moisture, light and heat resistance in a certain duration.
Concerning the 2D perovskites formed over the grain boundaries, the defects in the grain boundaries are passivated. In general, passivated structures exhibit outstanding optical properties, and homogeneous morphology, fewer defect traps, resulting in a reduced hole-electron recombination. Various passivating agents have been investigated, including thiols, phosphines and phosphine oxides; amines and ammonium salts have also shown the capacity as grain boundary passivating agents. Zhang et al. fabricated 2D/3D heterostructured PSCs combining the advantages of the high-performance of 3D MAPbI3 and the air-stable bismuth-based 2D quasi-perovskite MA3Bi2I9 (Hu et al., 2018a). Herein, the hydrophobic MA3Bi2I9 platelets were vertically inserted among the MAPbI3 grains, forming a lattice-like structure to encompass 3D MAPbI3 perovskite grains. The optimal 2D/3D (9.2%) heterostructured device achieves a high efficiency of 18.97%, with less hysteresis and dramatically enhanced stability. Similarly, Song and his co-workers incorporated a long-chain cation EDBEPbI4 (EDBE = 2,2-(ethylenedioxy)bis(ethylammonium)) into 3D perovskites to construct a phase-segregated vertical heterojunction (PVHH) based 2D/3D multidimensional perovskite (Li et al., 2018c). The grain boundaries (GBs) of 3D perovskite are vertically passivated by phase pure 2D perovskite, which could weaken the photo-induced localization of charge-carrier in low-dimensional perovskites. The vertical passivation would not affect the extraction of charge carriers between 3D perovskite and the charge transfer layers. Efficient (21.06%) and highly stable (maintain 90% of the initial PCE after 3,000 h in the air) planar PSCs are demonstrated using these 2D/3D mixed multidimensional perovskite-based PSCs. This kind of passivation on the grain boundary has mostly been observed by post-treatment of the 3D perovskite with few amounts of A’ to form a really thin layer of 2D perovskite. It is difficult to identify that this modification might be responsible for the improvements in the PCE and long-term stability of 2D/3D mixed multidimensional perovskite-based solar cells. In consequence, significant effort should be devoted to elucidating the mechanisms for a different set of conditions and systems. The photovoltaic performance and stability data of some representative layered 2D/3D multidimensional perovskite solar cells are summarized in Table 1.
Layered 2D/3D Multidimensional PSCS
The schematic illustration of mixed 2D/3D and layered 2D/3D has been shown in Figure 2. Compared to 2D/3D mixed perovskites, the 2D perovskites can be inserted in a controllable way by tuning the stoichiometric composition and concentration of precursor solutions. Moreover, the 2D perovskite mainly existed on the surface or the grain boundary of the 3D active layer, which could take all the advantages of both 2D and 3D perovskites. Generally, thin 2D perovskite films can be introduced as a capping layer on top of 3D perovskites by a two-step method: first, a 3D perovskite layer with excess PbI2 was spin-coated. Then, a solution of 2D organic cations (e.g., PEAI, BAI, OAI, 5-AVA) was coated on top of the 3D perovskites films. The as-prepared mixed 2D/3D perovskite layer usually shows longer photoluminescence lifetime and lower trap-state. In the work of Zhang and his co-workers, a thin layer of 2D (BA)2PbI4 was prepared via the chemical reaction between the post-treated n-butylamine iodide (BAI) and the residual PbI2 on a one-step deposited MAPbI3 film (Zou et al., 2019). The ultra-thin layer of 2D perovskite at the grain boundaries and on the surface of pristine 3D perovskite improves the stability and decreases the crystal defects of 3D perovskite. The obtained 2D/3D PSCs shows a PCE exceeds 18% and retains 80% of its initial value after over 2000 h of storage without encapsulation. Similarly, Lin et al. displayed an in situ growth approach to form a 2D perovskite capping layer by adding a small amount of dimethyl sulfoxide (DMSO) into the BAI solution (Tai et al., 2019). The champion device showed a PCE of 14.5% with significantly enhanced stability, maintaining over 80% PCE after exposure in ambient air (10% RH for 25 days and 25% RH for 25 days) for 50 days under dark conditions. This work developed a novel and effective strategy to prepare 2D/3D CsPbI3 photoabsorber with enhanced moisture stability and without sacrificing device performance. Grätzel and his co-workers proposed an approach for the stabilization of α phase of FAPbI3 with the protection of two-dimensional (2D) IBA2FAPb2I7 (IBA = iso-butylammonium and it showed excellent performance, specially, the stability maintain ∼85% of its initial efficiency with full illustration at 80°C for more than 500 h (Liu et al., 2020).
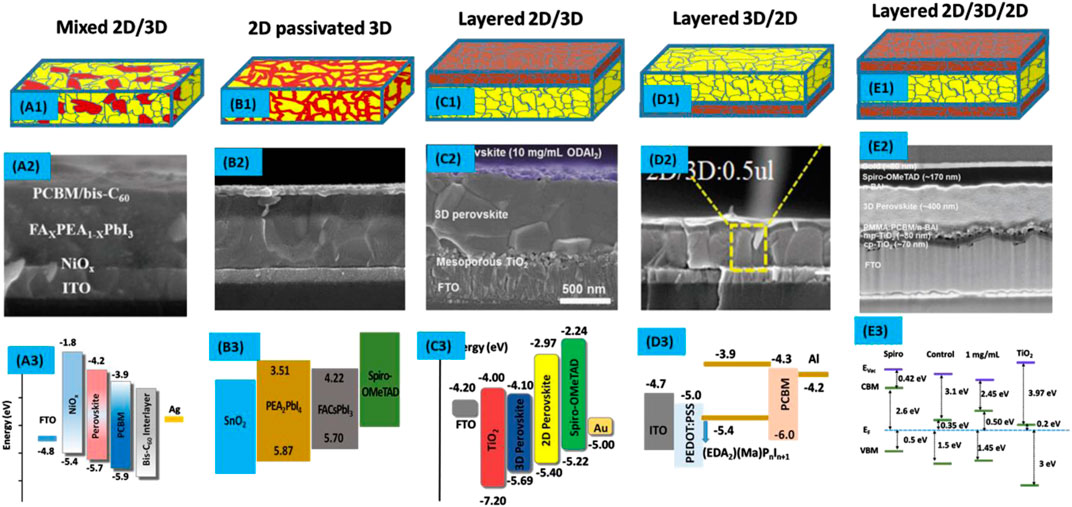
FIGURE 2. Device structure schematics for 2D/3D multidimensional perovskites serving as an (A) 2D perovskite mixed with 3D perovskite; (B) 2D perovskite passivated 3D perovskite at the grain boundary; (C) 2D perovskite interfacial layer on top of 3D perovskite layer; (D) 2D perovskite interfacial layer at the bottom of 3D perovskite layer; (E) 2D perovskite interfacial layer at both the top and bottom of the 3D perovskite layer. Wherein, (1), (2), and (3) in each category displayed the schematic image, cross-sectional SEM image and related energy diagram, respcetively.
On the other hand, the 2D perovskite films also can be deposited at the bottom of 3D perovskites utilizing the self-assembly and/or in situ formation method. Li et al. introduced branched polyethylenimine hydriodide (PEI•HI) and invented an in situ formed 2D perovskite (PEI)2PbI4 on top of PEDOT:PSS as an interfacial layer for the development of stable and efficient MAPbX3 based PSCs (Yao et al., 2015). Herein, the role of (PEI)2PbI4 interfacial layer can be summarized as follows: 1) control over the grain growth and morphology of upper 3D perovskite films; 2) facilitate hole extraction from MAPbX3 into PEDOT:PSS by the alignment of the energy level; 3) enhance the stability by preventing moisture at the interface. Finally, the devices displayed the PCEs over 16% and 13.8% on rigid and flexible substrates, respectively, as well as enhanced moisture stability. Taking the advantages of this design, Loi has demonstrated all-tin-based hybrid PSCs based on 3D/2D (FASnI3/PEA2SnI4) with efficiencies of 9% (Shao et al., 2018). The addition of 2D tin perovskite facilitates the uniform growth of large crystalline and oriented FASnI3 grains at low temperatures. The merits of the stacked 3D/2D structure are: 1) reduced amount of grain boundaries; 2) suppression of the formation of tin vacancies or Sn4+; 3) prolonged lifetime of the charge carriers. This work provides a potential way for further improvement of tin-based PSCs. Park et al. prepared a thin layer of 2D perovskite (EDA2)(CH3NH3)P=I3n+1) beneath the 3D perovskite which serves as a seeding layer for the growth of larger grains compared to that of the pure 3D perovskite films (Mahmud et al., 2020).
Since a layer of the thin 2D interfacial film works either at the bottom or the top of the 3D perovskite layer, a design of 2D/3D/2D is proposed as a double-side (DS) surface modification. In the work of White et al., a novel design of a double-sided passivation approach is presented where thin surface layers of the bulky organic cation (n-butylammonium iodide) based halide compound forming 2D layered perovskite at both the top and bottom of the 3D perovskite films (Mahmud et al., 2020). Highly efficient (22.77%) 2D/3D/2D perovskite-based devices with a remarkable Voc of 1.2 V is reported for a perovskite film possessing an optical bandgap of approximately 1.6 eV. Both 2D layers effectively passivate interfacial defects and suppresses the interfacial carrier recombination at both the interfaces of perovskites/HTL and perovskites/ETL. The discontinuous passivation layers provide conductive pathways for the efficient carrier extraction between the 3D perovskite and the charge transport layers, leading to the improvement of both Voc and FF in DS passivated devices, compared to that of the unpassivated or single-side passivated device. The photovoltaic performance and stability data of some representative layered 2D/3D multidimensional perovskite solar cells are summarized in Table 2.
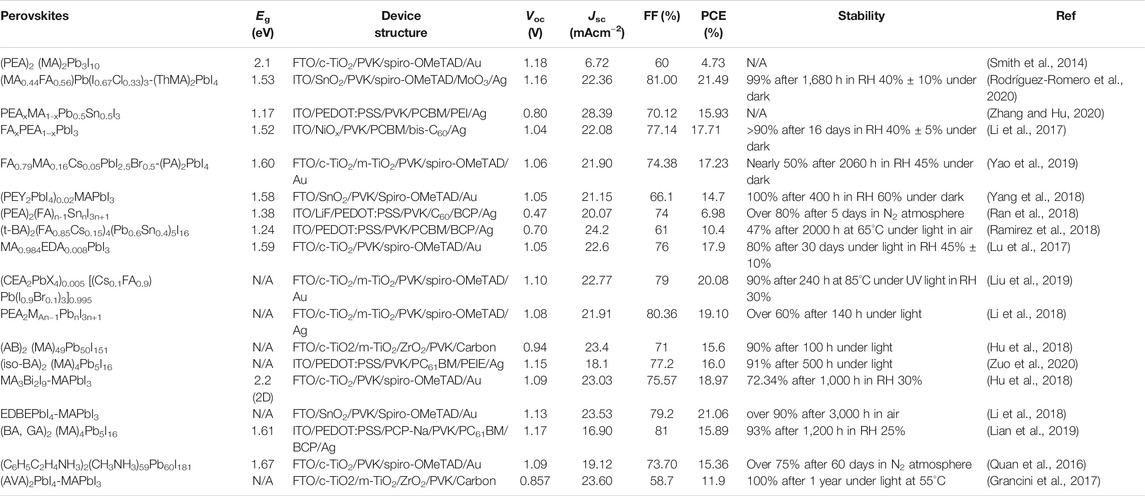
TABLE 1. Photovoltaic performance and stability summary of representative mixed 2D/3D multidimensional perovskite solar cells
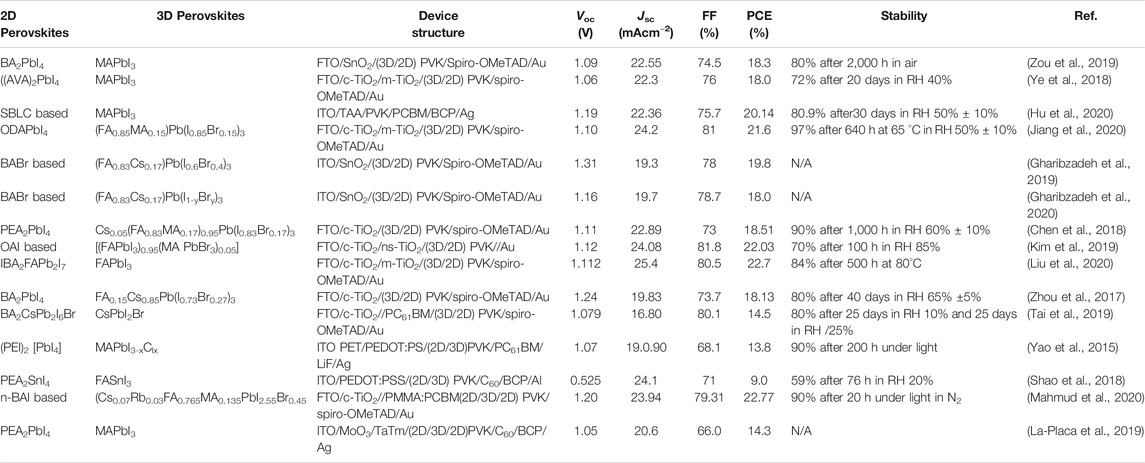
TABLE 2. Photovoltaic performance and stability summary of representative layered 2D/3D multidimensional perovskite solar cells
Challenges and Prospective
In the past few years, many impressing achievements on the development of efficient and stable 2D/3D multidimensional PSC have been demonstrated, which helps to acquire in-depth understanding of the driving forces regarding lattice degradation under moisture/heat/light exposure and design coherent engineering methodologies to cope with these deficiencies. Although the introduction of 2D perovskites into 3D perovskite structures to form the 2D/3D multidimensional perovskite structures have been verified as a promising approach to improve the photovoltaic performance and device stability, there are several challenges have to be addressed.
Challenges
The significant difference of charge mobility along the out-of-plane and in-plane directions for 2D perovskites will remain a hot research topic for diverse optoelectronic applications. Besides, the bulk mixture of 2D/3D perovskite should be studied intensively regarding their capability in the enhancement of device performance. Meanwhile, accurate analysis of the crystal structure of 2D/3D perovskites should not be bypassed since it is essential for revealing the mechanism of 2D/3D perovskite formation and charge transport. Besides, a molecular library should be established by investigating the effect of different molecular lengths, conjugated groups, functional units, and the substituent groups on the charge-transfer capacity and other properties. In terms of the 2D/3D perovskite-based devices, they have presented an encouraging improvement in the matter of long-term stability, especially comparing with their corresponding 3D counterparts. Further technologic tailoring should direct on the efficiency enhancement about push the efficiency close the theoretical Shockley-queisser limit. In particular, while the introduction of 2D perovskites as interfacial layers has obtained some success, a full understanding of the roles of such layers and/or treatments still remain opaque. Additionally, in the Sn-based perovskites, reducing their dimensionality seems to be an effective approach to hinder their oxidation, but the photovoltaic performance still needs to be improved dramatically.
Prospective
2D/3D multidimensional hybrid perovskites have been proved as one of the most promising approaches to improve both PCE and stability compared to that of the pure 3D PSCs. In terms of material engineering, numerous kinds of novel 2D perovskites can be synthesized and the coherent unknown properties can be investigated. Besides, the newly raised theoretical computational study would speed up the process of 2D materials screening and application. In specific, the long alkyl chain cations seem to be suitable to stabilize the 2D Pb-Sn or Sn-based perovskites materials, the performance can be improved through the compositional or/and structural engineering. In addition, it is still vital to investigate the formation mechanism of 2D layered perovskites, which will enable us to prepare the 2D perovskites with high phase purity and out-of-plane orientation and to grow 2D/3D perovskite materials in a more controllable approach. Also, an in-depth understanding of the chemical or physical interplay between 2D and 3D perovskite materials, 2D/3D perovskite materials, and the adjacent charge transportation layers will help to obtain efficient and stable PSCs. With the increase of the bandgap, 2D perovskite might be ideal for tandem solar cells in the further advance of energy harvesting. In summary, the strategy of developing multidimensional 2D/3D perovskites provides an opportunity for efficient and stable PSCs, which will assist an ingenious modification of PSCs and to shed light on its future commercialization.
Author Contributions
HH and BT conceived the idea. CG wrote the manuscirpt. YX and LL were involved the manuscript discussion and correction.
Funding
The financial support from National Natural Science Foundation of China (62004129) is gratefully acknowledged and this work was also supported by Shenzhen Polytechnic.
Conflict of Interest
The authors declare that the research was conducted in the absence of any commercial or financial relationships that could be construed as a potential conflict of interest.
References
Ali, N., Wang, X., Rauf, S., Attique, S., Khesro, A., Ali, S., et al. (2019). Enhanced stability in cesium assisted hybrid 2D/3D-perovskite thin films and solar cells prepared in ambient humidity. Sol. Energy 189, 325–332. doi:10.1016/j.solener.2019.07.081
Chen, P., Bai, Y., Wang, S., Lyu, M., Yun, J.-H., and Wang, L. (2018). In Situ growth of 2D perovskite capping layer for stable and efficient perovskite solar cells. Adv. Funct. Mater. 28 (17), 1706923. doi:10.1002/adfm.201706923
Gharibzadeh, S., Abdollahi Nejand, B., Jakoby, M., Abzieher, T., Hauschild, D., Moghadamzadeh, S., et al. (2019). Record open-circuit voltage wide-bandgap perovskite solar cells utilizing 2D/3D perovskite heterostructure. Adv. Energy Mater. 9 (21), 1803699. doi:10.1002/aenm.201803699
Gharibzadeh, S., Hossain, I. M., Fassl, P., Nejand, B. A., Abzieher, T., Schultes, M., et al. (2020). 2D/3D heterostructure for semitransparent perovskite solar cells with engineered bandgap enables efficiencies exceeding 25% in four-terminal tandems with silicon and CIGS. Adv. Funct. Mater. 30 (19), 1909919. doi:10.1002/adfm.201909919
Grancini, G., and Nazeeruddin, M. K. (2019). Dimensional tailoring of hybrid perovskites for photovoltaics. Nat. Rev. Mater 4 (1), 4–22. doi:10.1038/s41578-018-0065-0
Grancini, G., Roldán-Carmona, C., Zimmermann, I., Mosconi, E., Lee, X., Martineau, D., et al. (2017). One-Year stable perovskite solar cells by 2D/3D interface engineering. Nat. Commun. 8 (1), 15684. doi:10.1038/ncomms15684
Hu, J., Wang, C., Qiu, S., Zhao, Y., Gu, E., Zeng, L., et al. (2020). Spontaneously self-assembly of a 2D/3D heterostructure enhances the efficiency and stability in printed perovskite solar cells. Adv. Energy Mater. 10 (17), 2000173. doi:10.1002/aenm.202000173
Hu, Y., Qiu, T., Bai, F., Ruan, W., and Zhang, S. (2018a). Highly efficient and stable solar cells with 2D MA3Bi2I9/3D MAPbI3 heterostructured perovskites. Adv. Energy Mater. 8 (19), 1703620. doi:10.1002/aenm.201703620
Hu, Y., Zhang, Z., Mei, A., Jiang, Y., Hou, X., Wang, Q., et al. (2018b). Improved performance of printable perovskite solar cells with bifunctional conjugated organic molecule. Adv. Mater. 30 (11), 1705786. doi:10.1002/adma.201705786
Jagielski, J., Kumar, S., Yu, W.-Y., and Shih, C.-J. (2017). Layer-controlled two-dimensional perovskites: synthesis and optoelectronics. J. Mater. Chem. C 5 (23), 5610–5627. doi:10.1039/c7tc00538e
Jiang, X., Zhang, J., Ahmad, S., Tu, D., Liu, X., Jia, G., et al. (2020). Dion-Jacobson 2D-3D perovskite solar cells with improved efficiency and stability. Nano Energy 75, 104892. doi:10.1016/j.nanoen.2020.104892
Kim, H., Lee, S.-U., Lee, D. Y., Paik, M. J., Na, H., Lee, J., et al. (2019). Optimal interfacial engineering with different length of alkylammonium halide for efficient and stable perovskite solar cells. Adv. Energy Mater. 9 (47), 1902740. doi:10.1002/aenm.201902740
Kim, J. Y., Lee, J.-W., Jung, H. S., Shin, H., and Park, N.-G. (2020). High-efficiency perovskite solar cells. Chem. Rev., 120 (15), 7867–7918. doi:10.1021/acs.chemrev.0c00107
Krishna, A., Gottis, S., Nazeeruddin, M. K., and Sauvage, F. (2019). Mixed dimensional 2D/3D hybrid perovskite absorbers: the future of perovskite solar cells? Adv. Funct. Mater. 29 (8), 1806482. doi:10.1002/adfm.201806482
Lan, C., Zhou, Z., Wei, R., and Ho, J. C. (2019). Two-dimensional perovskite materials: from synthesis to energy-related applications. Mater. Today Energy 11, 61–82. doi:10.1016/j.mtener.2018.10.008
La-Placa, M.-G., Gil-Escrig, L., Guo, D., Palazon, F., Savenije, T. J., Sessolo, M., et al. (2019). Vacuum-deposited 2D/3D perovskite heterojunctions. ACS Energy Lett. 4 (12), 2893–2901. doi:10.1021/acsenergylett.9b02224
Li, H., Tong, G., Chen, T., Zhu, H., Li, G., Chang, Y., et al. (2018a). Interface engineering using a perovskite derivative phase for efficient and stable CsPbBr3 solar cells. J. Mater. Chem. A 6 (29), 14255–14261. doi:10.1039/c8ta03811b
Li, M.-H., Yeh, H.-H., Chiang, Y.-H., Jeng, U. S., Su, C.-J., Shiu, H.-W., et al. (2018b). Highly efficient 2D/3D hybrid perovskite solar cells via low-pressure vapor-assisted solution process. Adv. Mater. 30 (30), 1801401. doi:10.1002/adma.201801401
Li, P., Zhang, Y., Liang, C., Xing, G., Liu, X., Li, F., et al. (2018c). Phase pure 2D perovskite for high-performance 2D–3D heterostructured perovskite solar cells. Adv. Mater. 30 (52), 1805323. doi:10.1002/adma.201805323
Li, H., and Zhang, W. (2020). Perovskite tandem solar cells: from fundamentals to commercial deployment. Chem. Rev. 120 (18), 9835–9950. doi:10.1021/acs.chemrev.9b00780
Li, N., Zhu, Z., Chueh, C.-C., Liu, H., Peng, B., Petrone, A., et al. (2017). Mixed cation FAxPEA1–xPbI3 with enhanced phase and ambient stability toward high-performance perovskite solar cells. Adv. Energy Mater. 7 (1), 1601307. doi:10.1002/aenm.201601307
Lian, X., Chen, J., Zhang, Y., Tian, S., Qin, M., Li, J., et al. (2019). Two-dimensional inverted planar perovskite solar cells with efficiency over 15% via solvent and interface engineering. J. Mater. Chem. A 7 (32), 18980–18986. doi:10.1039/C9TA04658E
Liu, G., Zheng, H., Xu, X., Xu, S., Zhang, X., Pan, X., et al. (2019). Introduction of hydrophobic ammonium salts with halogen functional groups for high-efficiency and stable 2D/3D perovskite solar cells. Adv. Funct. Mater. 29 (47), 1807565. doi:10.1002/adfm.201807565
Liu, Y., Akin, S., Hinderhofer, A., Eickemeyer, F. T., Zhu, H., Seo, J.-Y., et al. (2020). Stabilization of highly efficient and stable phase-pure FAPbI3 perovskite solar cells by molecularly tailored 2D-overlayers. Angew. Chem. Int. Ed. 59 (36), 15688–15694. doi:10.1002/anie.202005211
Lu, J., Jiang, L., Li, W., Li, F., Pai, N. K., Scully, A. D., et al. (2017). Diammonium and monoammonium mixed-organic-cation perovskites for high performance solar cells with improved stability. Adv. Energy Mater. 7 (18), 1700444. doi:10.1002/aenm.201700444
Mahmud, M. A., Duong, T., Yin, Y., Pham, H. T., Walter, D., Peng, J., et al. (2020). Double-sided surface passivation of 3D perovskite film for high-efficiency mixed-dimensional perovskite solar cells. Adv. Funct. Mater. 30 (7), 1907962. doi:10.1002/adfm.201907962
Ortiz-Cervantes, C., Carmona-Monroy, P., and Solis-Ibarra, D. (2019). Two-dimensional halide perovskites in solar cells: 2D or not 2D? ChemSusChem 12 (8), 1560–1575. doi:10.1002/cssc.201802992
Quan, L. N., Yuan, M., Comin, R., Voznyy, O., Beauregard, E. M., Hoogland, S., et al. (2016). Ligand-stabilized reduced-dimensionality perovskites. J. Am. Chem. Soc. 138 (8), 2649–2655. doi:10.1021/jacs.5b11740
Ramirez, D., Schutt, K., Wang, Z., Pearson, A. J., Ruggeri, E., Snaith, H. J., et al. (2018). Layered mixed tin–lead hybrid perovskite solar cells with high stability. ACS Energy Lett. 3 (9), 2246–2251. doi:10.1021/acsenergylett.8b01411
Ran, C., Xi, J., Gao, W., Yuan, F., Lei, T., Jiao, B., et al. (2018). Bilateral interface engineering toward efficient 2D–3D bulk heterojunction tin halide lead-free perovskite solar cells. ACS Energy Lett. 3 (3), 713–721. doi:10.1021/acsenergylett.8b00085
Rodríguez-Romero, J., Sanchez-Diaz, J, Echeverría-Arrondo, C., Sofia, M., Esparza, D., Barea, E. M., et al. (2020). widening the 2D/3D perovskite family for efficient and thermal-resistant solar cells by the use of secondary ammonium cations. ACS Energy Lett. 5 (4), 1013–1021. doi:10.1021/acsenergylett.9b02755
Shao, S., Liu, J., Portale, G., Fang, H.-H., Blake, G. R., ten Brink, G. H., et al. (2018). Highly reproducible Sn-based hybrid perovskite solar cells with 9% efficiency. Adv. Energy Mater. 8 (4), 1702019. doi:10.1002/aenm.201702019
Smith, I. C., Hoke, E. T., Solis-Ibarra, D., McGehee, M. D., and Karunadasa, H. I. (2014). A layered hybrid perovskite solar-cell absorber with enhanced moisture stability. Angew. Chem. Int. Ed. 53 (42), 11232–11235. doi:10.1002/anie.201406466
Tai, M., Zhou, Y., Yin, X., Han, J., Zhang, Q., Zhou, Y., et al. (2019). In situ formation of a 2D/3D heterostructure for efficient and stable CsPbI2Br solar cells. J. Mater. Chem. A 7 (39), 22675–22682. doi:10.1039/c9ta08564e
Wang, B., Iocozzia, J., Zhang, M., Ye, M., Yan, S., Jin, H., et al. (2019). The charge carrier dynamics, efficiency and stability of two-dimensional material-based perovskite solar cells. Chem. Soc. Rev. 48 (18), 4854–4891. doi:10.1039/c9cs00254e
Yan, J., Qiu, W., Wu, G., Heremans, P., and Chen, H. (2018). Recent progress in 2D/quasi-2D layered metal halide perovskites for solar cells. J. Mater. Chem. A 6 (24), 11063–11077. doi:10.1039/c8ta02288g
Yang, F., Zhang, P., Kamarudin, M. A., Kapil, G., Ma, T., and Hayase, S. (2018). Addition effect of pyreneammonium iodide to methylammonium lead halide perovskite-2D/3D heterostructured perovskite with enhanced stability. Adv. Funct. Mater. 28 (46), 1804856. doi:10.1002/adfm.201804856
Yao, D., Zhang, C., Zhang, S., Yang, Y., Du, A., Waclawik, E., et al. (2019). 2D–3D mixed organic–inorganic perovskite layers for solar cells with enhanced efficiency and stability induced by n-propylammonium iodide additives. ACS Appl. Mater. Interfaces 11 (33), 29753–29764. doi:10.1021/acsami.9b06305
Yao, K., Wang, X., Xu, Y.-x., and Li, F. (2015). A general fabrication procedure for efficient and stable planar perovskite solar cells: morphological and interfacial control by in-situ-generated layered perovskite. Nano Energy 18, 165–175. doi:10.1016/j.nanoen.2015.10.010
Ye, T., Bruno, A., Han, G., Koh, T. M., Li, J., Jamaludin, N. F., et al. (2018). Efficient and ambient-air-stable solar cell with highly oriented 2D@3D perovskites. Adv. Funct. Mater. 28 (30), 1801654. doi:10.1002/adfm.201801654
Zhang, F., Kim, D. H., and Zhu, K. (2018). 3D/2D multidimensional perovskites: balance of high performance and stability for perovskite solar cells. Curr. Opin. Electrochem. 11, 105–113. doi:10.1016/j.coelec.2018.10.001
Zhang, F., Lu, H., Tong, J., Berry, J. J., Beard, M. C., and Zhu, K. (2020). Advances in two-dimensional organic–inorganic hybrid perovskites. Energy Environ. Sci. 13 (4), 1154–1186. doi:10.1039/c9ee03757h
Zhang, J., and Hu, B. (2020). Revealing photoinduced bulk polarization and spin-orbit coupling effects in high-efficiency 2D/3D Pb–Sn alloyed perovskite solar cells. Nano Energy 76, 104999. doi:10.1016/j.nanoen.2020.104999
Zheng, X., Chen, B., Dai, J., Fang, Y., Bai, Y., Lin, Y., et al. (2017). Defect passivation in hybrid perovskite solar cells using quaternary ammonium halide anions and cations. Nature Energy 2 (7), 17102. doi:10.1038/nenergy.2017.102
Zhou, T., Lai, H., Liu, T., Lu, D., Wan, X., Zhang, X., et al. (2019). Highly efficient and stable solar cells based on crystalline oriented 2D/3D hybrid perovskite. Adv. Mater. 31 (32), 1901242. doi:10.1002/adma.201901242
Zhou, Y., Wang, F., Cao, Y., Wang, J.-P., Fang, H.-H., Loi, M. A., et al. (2017). Benzylamine-treated wide-bandgap perovskite with high thermal-photostability and photovoltaic performance. Adv. Energy Mater. 7 (22), 1701048. doi:10.1002/aenm.201701048
Zou, Y., Cui, Y., Wang, H.-Y., Cai, Q., Mu, C., and Zhang, J.-P. (2019). Highly efficient and stable 2D–3D perovskite solar cells fabricated by interfacial modification. Nanotechnology 30 (27), 275202. doi:10.1088/1361-6528/ab10f3
Keywords: multidimension, 2D/3D, perovksite, stability, solar cells
Citation: Ge C, Xue Y, Li L, Tang B and Hu H (2020) Recent Progress in 2D/3D Multidimensional Metal Halide Perovskites Solar Cells. Front. Mater. 7:601179. doi: 10.3389/fmats.2020.601179
Received: 31 August 2020; Accepted: 09 October 2020;
Published: 29 October 2020.
Edited by:
Annie Ng, Nazarbayev University, KazakhstanReviewed by:
Mannix Balanay, Nazarbayev University, KazakhstanMingjian Yuan, Nankai University, China
Copyright © 2020 Ge, XUE, Li, Tang and Hu. This is an open-access article distributed under the terms of the . The use, distribution or reproduction in other forums is permitted, provided the original author(s) and the copyright owner(s) are credited and that the original publication in this journal is cited, in accordance with accepted academic practice. No use, distribution or reproduction is permitted which does not comply with these terms.
*Correspondence: Hanlin Hu, hanlin.hu@foxmail.com Bin Tang tangbinsci@gmail.com