- 1Department of Dental Materials, School of Dentistry, Federal University of Rio Grande do Sul, Porto Alegre, Brazil
- 2Ph.D. Program in Biomedical Sciences, University of Maryland School of Dentistry, Baltimore, MD, United States
- 3Department of Restorative Dental Sciences, Imam Abdulrahman Bin Faisal University, College of Dentistry, Dammam, Saudi Arabia
- 4Division of Operative Dentistry, Department of General Dentistry, University of Maryland School of Dentistry, Baltimore, MD, United States
- 5Ph.D. Program in Biomedical Sciences, University of Maryland School of Dentistry, Baltimore, MD, United States
The incorporation of metallic oxides in dental adhesives has been a strategy to confer improved radiopacity and physicochemical properties for polymers. Tailoring the structure of these fillers could contribute to their application in therapeutic strategies for dental restorations. The aim of this study was to evaluate the incorporation of niobic acid into experimental dental adhesives, and compare these adhesives to niobium pentoxide containing adhesives. A control group without Nb2O5·n H2O or Nb2O5 was also used for comparison. Niobium-based particles have been used as a feasible approach, mainly because of their bioactivity. In this study, hydrated niobium pentoxide, also called niobic acid (Nb2O5·n H2O), was incorporated into an experimental dental adhesive as a potential catalyst for monomer conversion. A base resin for dental adhesive was formulated with methacrylate monomers and photoinitiators. Two types of oxides were tested as filler for this adhesive: Nb2O5·n H2O or niobium pentoxide (Nb2O5). Both fillers were added separately into the experimental adhesive at 0, 2.5, 5, and 10 wt.%. One group without Nb2O5·n H2O or Nb2O5 (0 wt.% of filler addition) was used as a control group. The formulated materials were analyzed for radiopacity according to the ISO 4049 and used FTIR analysis to assess the degree of conversion (DC) and the maximum polymerization rate (RPmax). Mechanical properties were analyzed by ultimate tensile strength (UTS) in a testing machine. Softening in solvent was conducted by measuring Knoop microhardness before and after immersion of samples in ethanol. Normality of data was assessed with Shapiro-Wilk, and comparisons between factors were conducted with two-way ANOVA and Tukey at 5% of significance. Both fillers, Nb2O5 or Nb2O5·n H2O, increased the radiopacity of dental adhesives in comparison to the unfilled adhesive (p < 0.05). There were no differences among groups for the ultimate tensile strength (p > 0.05), and all groups containing Nb2O5 or Nb2O5·n H2O improved the resistance against softening in solvent (p < 0.05). The groups with 5 and 10 wt% addition of Nb2O5 showed decreased DC compared to the control group (p < 0.05), while the addition of Nb2O5·nH2O up to 10 wt% did not alter the DC (p > 0.05). The polymerization rate did not change among groups (p > 0.05). In conclusion, Nb2O5·n H2O is a promising filler to be incorporated into dental adhesives providing proper mechanical properties, improved resistance against solvents, and increased radiopacity, without changing the DC.
Introduction
Adhesive systems for dental restoration have undergone several modifications so that their physical and chemical properties could be improved, supporting long-lasting restorative treatments. Inorganic fillers have been incorporated into dental adhesives aiming to reduce polymeric degradation via increasing hydrolytic stability (Münchow and Bottino, 2017). Furthermore, these particles have been tuning in adhesives formulation to improve polymers’ radiopacity (Garcia et al., 2020a) and mechanical properties (Lohbauer et al., 2010; Belli et al., 2014). Inorganic fillers have also been proposed to improve the therapeutic activity of resin-based materials. Bioactive particles have been used to assist in tooth remineralization (Balhaddad et al., 2019; Braga and Fronza, 2020), making bioactive resin-based dental materials to be feasible approaches to prevent dental demineralization and to provide the recovery of hard dental tissues affected by caries (Garcia et al., 2017; Ibrahim et al., 2020a; Ibrahim et al., 2020b). Calcium phosphates (Garcia et al., 2017; Braga and Fronza, 2020), calcium silicates (Profeta, 2014), bioactive glasses (Balbinot et al., 2020a; Yao et al., 2020), and zinc-based particles (Toledano et al., 2016) were already tested and showed mineral deposition or remineralization effect on dental hard tissues. Recently, niobium-based particles have been highlighted due to their interesting properties for dentistry, such as high radiopacity and bioactivity (Marins et al., 2018).
Niobium pentoxide (Nb2O5) has been proposed as a stable bioactive inorganic compound that has been used in different biomaterials in the biomedical field (Obata et al., 2012; Balbinot et al., 2018). The ability to promote deposition and growth of hydroxyapatite crystals on its surface when in contact with simulated body fluid (Karlinsey and Yi, 2008), artificial or natural saliva (Karlinsey et al., 2006) was explored in different materials in dentistry (Mazur et al., 2015) (Lopes et al., 2014; Altmann et al., 2017; Balbinot et al., 2018; Balbinot et al., 2019; Marins et al., 2019; Balbinot et al., 2020a; Balbinot et al., 2020b; Marins et al., 2020). Nb2O5 was studied in experimental adhesives (Leitune et al., 2013a; Collares et al., 2014; Marins et al., 2018), endodontic sealer (Leitune et al., 2013b), and glass ionomer cements (Garcia et al., 2016), improving their radiopacity (Leitune et al., 2013a; Leitune et al., 2013b; Garcia et al., 2016; Marins et al., 2018), physical properties (Leitune et al., 2013a; Leitune et al., 2013b) and inducing mineral deposition on adhesives surfaces (Collares et al., 2014). Increasing the Nb2O5 content into the polymeric matrix may lead to modifications to the properties of the material. The higher concentration is related to the reduction in the conversion of carbon-carbon double bonds (C=C) into single carbon-bonds (C-C) of methacrylate groups (Leitune et al., 2013a; Leitune et al., 2013b). This ineffective polymerization results in more residual monomers are available in the resin matrix, which may lead to higher cytotoxic effects (Salehi et al., 2015), greater sorption and solubility (Collares et al., 2011), lower bonding effectiveness to dentin (Hass et al., 2013), and lower mechanical properties (Ferracane, 1985). Balancing the potential bioactivity of Nb2O5 with adequate physicochemical and mechanical properties is essential for the application of these materials in a clinical scenario.
Among the oxides of niobium (Nb), Nb2O5 is the most stable. Nb can present a wide range of oxidation numbers from +5 to −3, with higher oxidation stated being more frequent to be found, mainly +5 states (Nowak and Ziolek, 1999). Due to the coordination numbers variability of Nb, this is a versatile element to develop novel materials, including organometallic structures. Among the different forms of niobium oxides, it is possible to synthesize hydrated niobium pentoxide, also called niobic acid (Nb2O5·nH2O), through the hydrolysis of niobium pentachloride (NbCl5) or niobium ethoxide (Nb(OC2H5)5) (Nakajima et al., 2011) or chemical reaction with acetophenones (Skrodczky et al., 2019). Niobic acid has been used in industry as a heterogeneous catalyst for chemical reactions due to its behavior as Lewis acid (Tanabe, 2003; Nakajima et al., 2011).
Nb2O5·nH2O has a high acid strength (Ho −5.6∼−8.2) and Lewis and Brønsted acid sites are available in the Nb2O5·nH2O surface, making this oxide an efficient catalyst due to the reception of electrons (Lewis acid) or donation of protons (Brønsted acid) (Ziolek and Ziolek, 1999). As a heterogeneous catalyst, niobic acid may confer lower necessary energy to activate the chemical reactions (Nakajima et al., 2011). These differences in chemical structure may result in an optimized response of Nb2O5·nH2O for dental applications. Although Nb2O5 was analyzed in dental adhesives previously (Leitune et al., 2013a; Marins et al., 2018), the potential effect of Nb2O5·nH2O was not investigated so far. The aim of this study was to evaluate the incorporation of niobic acid into experimental dental adhesives and compare these adhesives to niobium pentoxide containing dental adhesives. A control group without Nb2O5·nH2O or Nb2O5 was also used for comparison. The following physicochemical properties were analyzed: radiopacity, ultimate tensile strength, softening in solvent, degree of conversion, and polymerization rate.
Materials and Methods
Dental Adhesives Formulation
The experimental dental adhesives were formulated by mixing 66.66 wt.% of bisphenol A glycerolate dimethacrylate (BisGMA) and 33.33 wt.% 2-hydroxyethyl methacrylate (HEMA). Camphorquinone and ethyl 4-dimethylaminobenzoate at 1 mol% and butylated hydroxytoluene at 0.01 wt.% were added to all groups as photoinitiator system. Niobium pentoxide (Nb2O5) and niobic acid (Nb2O5·nH2O) were incorporated at three different concentrations into this base resin: 2.5; 5 and 10 wt.%. As a control group, this base resin was used without Nb2O5 or Nb2O5·nH2O addition in all tests. Table 1 describes the components used to design the adhesives resin groups according to the addition of fillers by weight. The monomers and the photoinitiator materials were obtained from Aldrich Chemical Co. (Milwaukee, WI, USA) and used without further purification. The fillers were hand-mixed for 5 min, sonicated for 180 s, and hand-mixed again for 5 min. A light-emitting diode (LED) unit (Radii, SDI, Bayswater, VIC, Australia) with 1,200 mW/cm2 was used to perform the photoactivation for all tests.
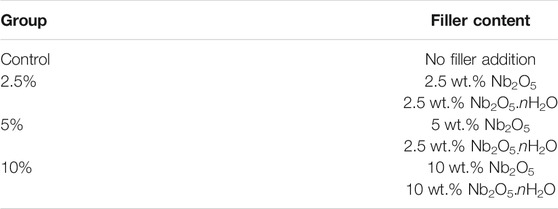
TABLE 1. Description of the components used to design the dental adhesives according to the addition of filles by weight.
Dental Adhesives Evaluation
Radiopacity
The radiopacity of the dental adhesives was evaluated according to ISO 4049 [(ISO), 2009], using five samples per group (10.0 mm diameter and 1.0 mm thick, n = 5). The samples were prepared using a polyvinyl siloxane mold (ADSIL, Vigodent, Rio de Janeiro, RJ, Brazil), 20 s of photoactivation on each side, and stored in distilled water for 24 h at 37°C. An X-ray source (DabiAtlante model Spectro 70X) at 70 kV and 8 mA was used to expose the samples and acquire the X-ray images. Phosphorus plates (Digital System, VistaScan, Dürr Dental GmbH & Co. KG, Bietigheim-Bissingen, Baden-Württemberg, Germany) were used with 0.8 s of exposition and a focus-film distance of 400 mm. Each film was exposed with one sample per group together with an aluminum step-wedge (8 mm thick with 1 mm steps) in all images acquirement. The images were saved in TIFF format, and the mean and standard deviation values of gray for each sample and each aluminum millimeter were obtained (Photoshop software, Adobe Systems Incorporated, San Jose, CA, USA). The values were expressed as the equivalent thickness of aluminum in mm (Leitune et al., 2013a).
Ultimate Tensile Strength
Ten samples per group (n = 10) were prepared using a metallic mold with an hourglass shape (8 mm long, 2 mm wide, 1 mm thick, 1 mm2 cross-sectional area). Each specimen was photoactivated for 20 s on each side, removed from the mold, and stored in distilled water at 37°C for 24 h. After this period, each sample had its constriction area measured with a digital caliper and fixed with a cyanoacrylate resin into metallic jigs. Each jig was positioned in a universal testing machine (EZ-SX Series, Shimadzu, Tokyo, Kantō, Japan). The upper part of the jig was tensile at a crosshead speed of 1 mm/min until the samples fracture. The values were recorded in Newtons (N), divided by the area of each sample (mm), and expressed in megapascals (MPa).
Softening in Solvent
Three samples per group (1 mm thick and 5 mm diameter, n = 3) (Garcia et al., 2019) were prepared with a polyvinyl siloxane (Express™ VPS Impression Material, 3 M ESPE, St. Paul, MN, USA) mold, 20 s of photoactivation on each side, and stored in distilled water for 24 h at 37°C. The samples were embedded in self-curing acrylic resin (Clássico, São Paulo, São Paulo, Brazil) and polished (Model 3v, Arotec, Cotia, SP, BR) with silicon carbide sandpapers (from 600 to 2000-grit, Klingspor, Pinhas, PR, Brazil) and felt disc sutured with alumina suspension (Alumina 1 μm, Fortel, São Paulo, SP, BR). The embedded samples were washed with running water and sonicated for 480 s (L100; Schuster, Santa Maria, RS, Brazil) with distilled water. After 24 h, the specimens were evaluated by three indentations at 10 g/5 s to obtain the initial Knoop hardness (KHN1) using a digital microhardness tester (HMV 2, Shimadzu, Tokyo, Kantō, Japan). The specimens were subjected to softening in ethanol for 2 h, and the hardness test was performed again (KHN2). The percentual hardness reduction was calculated for each sample and each group, according to Eq. 1:
Degree of Conversion
Three samples per group (n = 3) were evaluated by Fourier Transform Infrared Spectroscopy (FTIR) with a spectrometer (Vertex 70, Bruker Optics, Ettlingen, Baden-Württemberg, Germany) equipped with an attenuated total reflectance device (ATR). The uncured adhesives were dispensed onto the crystal using a polyvinyl siloxane mold of 1 mm thick and 5 mm diameter. The spectra from each sample were acquired using Opus 6.5 software (Bruker Optics, Ettlingen, Baden-Württemberg, Germany) with Blackman Haris 3-Term apodization, in the range from 400 to 4,000 cm−1, with 64 scans and 4 cm−1 resolution. Then, the sample was light-cured for 20 s at a standardized distance of 1 mm between the tip of the LED unit and the top of the sample. Immediately after the light-curing process, the spectra of the cured adhesive samples were obtained. The degree of conversion (DC) was calculated according to previous studies using the peaks at 1,610 cm−1 (aromatic carbon-carbon double bonds) and 1,640 cm−1 (aliphatic carbon-carbon double bonds) (Collares et al., 2011; Collares et al., 2013; Garcia et al., 2018; Garcia et al., 2020b).
Polymerization Rate
The polymerization rate of three samples per group of dental adhesives (n = 3) were evaluated by differential scanning calorimetry (DSC, DSC-Q2000, TA Instrument Co., New Castle, DE, USA) with a photocalorimetric accessory (PCA) adjusted to 100 mW/cm2. The uncured adhesives were photoactivated with PCA under a nitrogen flow rate of 50 ml/min. The maximum polymerization rate was determined according to a previous study (Rodrigues et al., 2015).
Statistical Analysis
SigmaPlot software (version 12.0, Systat Software, San Jose, CA, USA) was used for the statistical analyses. Shapiro-Wilk test was used to analyze the data distribution. Radiopacity, DC, polymerization rate, UTS, and softening in solvent were evaluated using two-way ANOVA and Tukey post-hoc test considering the two factors: concentrations (0, 2.5, 5, or 10 wt.%) and filler (Nb2O5 or Nb2O5·nH2O). A significance level of 0.05 was used to analyze the data statistically.
Results
The results of the radiopacity evaluation of the dental adhesives are displayed in Figure 1. Representative x-ray images were shown in Figure 1A with an aluminum scale bar used as a comparison for mm Al calculation. With increasing the content of Nb2O5 or Nb2O5·nH2O, the radiopacity increased in contrast to the control group (p < 0.05). There were no differences between the groups with 2.5 or 5 wt.% of fillers regardless of its type (Nb2O5 or Nb2O5·nH2O) (p > 0.05). The group with 10 wt.% of Nb2O5 showed higher radiopacity in comparison to 10 wt% of Nb2O5·nH2O (p < 0.05). However, within the same filler type, the incorporation of 10 wt% of Nb2O5 or Nb2O5·nH2O showed the highest radiopacity (p < 0.05), with values close to 1 mm of aluminum.
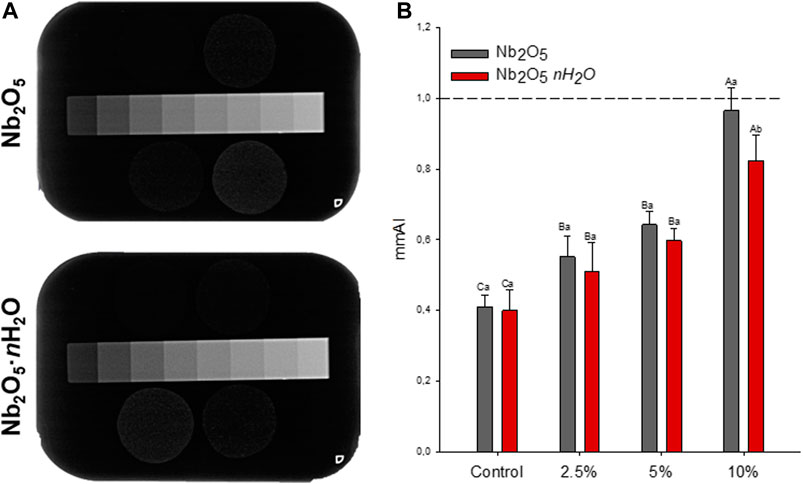
FIGURE 1. Radiopacity of the formulates dental adhesives (A) Representative images of X-rays radiographs from the adhesives with different types of fillers (Nb2O5 or Nb2O5. nH2O) and different concentrations (0, 2.5, 5, and 10 wt%). The aluminum scale bar was positioned close to the specimens to obtain mm Al values (B) Quantification of radiopacity in mm Al. The dotted line represents 1 mm Al, which is the same radiopacity of 1 mm of dentin. Different capital letters indicate a statistically significant difference among the concentrations (0, 2.5, 5, and 10 wt%) for the same type of filler (Nb2O5 or Nb2O5. nH2O) (p < 0.05). Different lowercase letters indicate a statistically significant difference within the same concentration (0, 2.5, 5, or 10 wt%) comparing the different types of filler (Nb2O5 or Nb2O5. nH2O) (p < 0.05).
The results from the mechanical evaluation of the dental adhesives are shown in Figure 2. Among all groups, the values ranged from 55.17 (±9.48) for 5 wt.% of Nb2O5·nH2O to 65.80 (±7.79) for 2.5 wt.% of Nb2O5·nH2O. There were no statistically significant differences among groups regardless of the fillers’ concentration or filler type (p > 0.05).
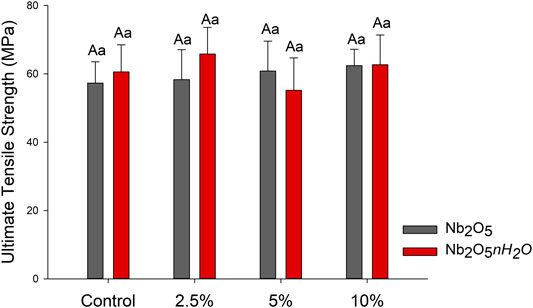
FIGURE 2. Mean and standard deviation values of the ultimate tensile strength (UTS) of the dental adhesives in MPa. Different capital letters indicate a statistically significant difference among the concentrations (0, 2.5, 5, and 10 wt%) for the same type of filler (Nb2O5 or Nb2O5.nH2O) (p < 0.05). Different lowercase letters indicate a statistically significant difference within the same concentration (0, 2.5, 5, or 10 wt%) comparing the different types of filler (Nb2O5 or Nb2O5. nH2O) (p < 0.05).
Figure 3 displays the results of softening in solvent. For Nb2O5 groups, the addition from 2.5 wt.% of this filler decreased the percentage of softening compared to the control group (p < 0.05), without statistically significant differences among 2.5, 5, and 10 wt.% (p > 0.05). The same behavior occurred for Nb2O5·nH2O groups: the incorporation of any tested concentration of Nb2O5·nH2O improved the resistance against softening in solvent in comparison to the unfilled adhesive (p < 0.05), and no changed were observed from 2.5 to 10 wt.% of Nb2O5·nH2O (p > 0.05).
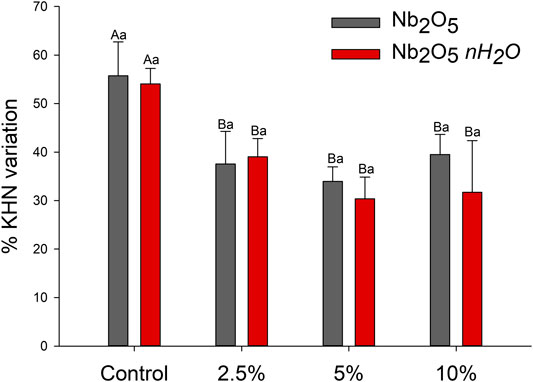
FIGURE 3. Mean and standard deviation of softening in solvent after immersion in ethanolic solution in percentage of microhardness variation. Different capital letters indicate a statistically significant difference among the concentrations (0, 2.5, 5, and 10 wt%) for the same type of filler (Nb2O5 or Nb2O5.nH2O) (p < 0.05). Different lowercase letters indicate a statistically significant difference within the same concentration (0, 2.5, 5, or 10 wt%) comparing the different types of filler (Nb2O5 or Nb2O5. nH2O) (p < 0.05).
The DC and Rpmax are presented in Figure 4. For Nb2O5 groups, the DC ranged from 64.93 (±0.78) for the control to 51.49 (±2.55) for 10 wt.% (p < 0.05). From 5 wt.% of Nb2O5 addition, the DC decreased in comparison to 0 and 2.5 wt.% (p < 0.05), with lower DC result for the 10 wt.% Nb2O5 addition (p < 0.05). For Nb2O5·nH2O groups, the DC ranged from 65.13 (±0.68) for 2.5 wt.% to 62.74 (±1.71) for 5 wt.% (Figure 4A). There were no statistically significant differences among groups with different concentrations of Nb2O5·nH2O (p > 0.05). The addition of five or 10 wt.% of Nb2O5·nH2O induced higher DC in comparison to five or 10 wt% of Nb2O5. The Rpmaxvia DSC showed no difference among groups, neither between the two types of filler, neither among fillers concentration (Figure 4B).
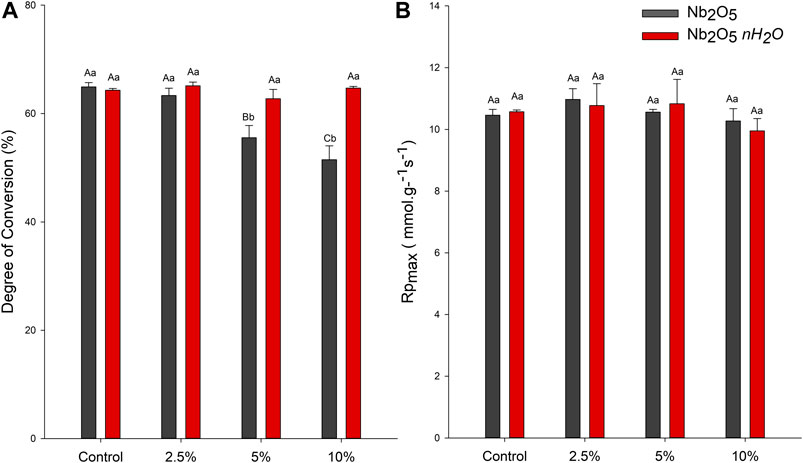
FIGURE 4. Degree of conversion (DC) and maximum polymerization rate (RPmax) of dental adhesives (A) Percentage of converted carbon-carbon double bonds of the dental adhesives after photoactivation via FTIR-ATR (B) RPmax of the dental adhesives via DSC-PCA. Different capital letters indicate a statistically significant difference among the concentrations (0, 2.5, 5, and 10 wt%) for the same type of filler (Nb2O5 or Nb2O5. nH2O) for the DC or RPmax evaluation (p < 0.05). Different lowercase letters indicate a statistically significant difference within the same concentration (0, 2.5, 5, or 10 wt%) comparing the different types of filler (Nb2O5 or Nb2O5. nH2O) for the DC or RPmax evaluation (p < 0.05).
Discussion
Dental adhesives have been modified to present superior therapeutic and physicochemical properties (Profeta, 2014; Balbinot et al., 2020a; Bendary et al., 2020; Garcia et al., 2020b; Yao et al., 2020). In this context, bioactive fillers have been incorporated into dental adhesives (Profeta, 2014; Balbinot et al., 2020a; Bendary et al., 2020; Braga and Fronza, 2020; Yao et al., 2020). However, the effect of these fillers in the physicochemical properties of resin-based materials must be addressed to guarantee the proper behavior for dental adhesives. In the present study, niobic acid was studied as a potential catalyst bioactive inorganic filler into dental adhesives. The radiopacity, the resistance against softening in solvents, and the mechanical properties were maintained for niobic acid-containing adhesives when compared to niobium pentoxide filled materials. The highest concentrations of Nb2O5·nH2O supported a high DC in comparison to the unfilled adhesive, with an increased percentage of conversion when compared to Nb2O5 in the same concentrations.
The life span of resin composite restorations is importantly affected by the recurrence of caries and the identification of marginal gaps, leading to the premature replacement of composites (Brouwer et al., 2016). Adhesives must be distinguishable from the adjacent tissues in the radiographic examination to assist in the proper diagnose of carious affected tissues (Brouwer et al., 2016; Mjor, 2005). The low radiopacity of adhesives hampers their distinguish from adjacent tissues and pathological processes, and as shown in Figure 1A, unfilled adhesives are difficult to be observed on X-ray radiographs. The International Organization for Standardization (ISO) established that polymer-based restorative materials must present radiopacity equal or superior to dentin (International Organisation for Standardization, 2009) and it is known that 1 mm of dentin has a radiopacity similar to 1 mmAl in the aluminum step-wedge (Mjor, 2005). Thus, reaching this value is the goal of the development of radiopaque materials. Neither particle reached a radiopacity average with this value, as shown in Figure 1B. However, both types of filler, Nb2O5, and Nb2O5·nH2O, provided dental adhesives with higher radiopacity than the unfilled material, which has a similar composition to a commercial widely used adhesive (Ogliari et al., 2006). Our results indicated that Nb2O5 or Nb2O5·nH2O at 10 wt.% reached ∼0.8–0.95 mm Al for the groups suggesting that, despite the potential bioactivity, these fillers could provide suitable radiopacity for the developed dental adhesives.
The main responsibility for the increased radiopacity is the higher atomic number of Nb (41) in comparison to the other components of the dental adhesive [carbon (6), hydrogen (1), nitrogen (7), oxygen (8)]. At 10 wt.% concentration, higher values were observed for Nb2O5 when compared to Nb2O5·nH2O (Figure 1; p < 0.05). Differences in chemical composition between both particles may have led to the higher radiopacity of adhesives doped with Nb2O5 when 10 wt.% was added. The particles of Nb2O5·nH2O are commonly derived from the hydrolysis of niobium pentachloride, forming Nb2O5·nH2O. In contrast, the particles of Nb2O5 are mostly composed of Nb, and O. Differences in the particle densities and, consequently, in the volume of filler incorporated, maybe was responsible for the radiopacity variation between the groups of adhesives with 10 wt.% of Nb2O5 or Nb2O5·nH2O.
The formulated dental adhesives were also analyzed for their mechanical properties via UTS and softening in solvent. The UTS shows the intrinsical resistance of materials against fractures when they are tensioned. Inorganic particles may act via toughening behavior such as crack deflection and increased elastic modulus (Lohbauer et al., 2010). In this study, we did not observe differences in the UTS (Figure 2; p > 0.05), while the softening in solvent for all groups containing a filler, regardless of its type, decreased in comparison to the control group (Figure 3). This test evaluates the ability of polymers to resist solvents. Therefore, the hydrophilicity and the crosslinking density of the polymers are involved in this process outcome.
When exposed to solvents, polymers are prone to swell because the forces among the polymer chains are surpassed by the attraction between polymer chains and molecule solvents (Schneider et al., 2008). Then, depending on the polymer network formation, the time of immersion, and the solubility parameter of the solution used, polymer chains maybe degrade via hydrolysis (Ferracane, 2006; Schneider et al., 2008). In this study, after the immersion in solvent, all groups presented lower Knoop hardness, corroborating with previous analyses (Schneider et al., 2008; Garcia et al., 2020b). Resins with inorganic fillers, such as the metallic oxides here tested, are less susceptible to suffer chemical degradation mainly because ionic bonding between atoms in oxides is more stable than covalent bonding within the organic matrix. Therefore, adhesives doped with inorganic fillers can present lower softening in solvent compared to unfilled adhesives (Stürmer et al., 2020). Irrespective of the concentration of Nb2O5 or Nb2O5·nH2O, both materials provided successful decreased softening for the dental adhesives. Therefore, the crosslinking density of the filled materials was superior to the control group, or the fillers were able to increase stability by reducing the amount of organic content.
The properties of Nb2O5 and Nb2O5·nH2O particles, such as density, size, and volume, as well as their chemical composition, may affect the loading of these fillers into the dental adhesives. The ability to convert carbon-carbon double bonds into carbon-carbon single bonds highly depends on the availability of light through the polymer thickness since these materials are photoactivated (Par et al., 2020). By increasing metallic oxides content into adhesives, it is likely to observe a decreased DC (Leitune et al., 2013a; Garcia et al., 2018; Garcia et al., 2020a). This effect may be related to the higher opacity that filled adhesives may present in comparison to unfilled adhesives (Shortall, 2005). Moreover, it was suggested that the presence of oxides into the composition of unsilanized glasses could also jeopardize the degree of conversion, depth of cure, and polymerization rate via “premature termination of free-radical-mediated polymerization” (Par et al., 2020). The results of the present study were in accordance with previous analyses when the addition of Nb2O5 decreased the DC of dental adhesives (Leitune et al., 2013a). While the addition of 5 wt.% of Nb2O5 was enough to reduce the DC in comparison to the control group, the use of Nb2O5·nH2O did not change this property up to 10 wt.% (Figure 2A). The high DC, even with 10 wt.% of Nb2O5·nH2O, could be a result of the activity of this oxide within the resin during the polymerization reaction. The presence of Lewis and Brønsted acid sites in these particles may increase the reaction during the polymerization (Tanabe, 2003; Nakajima et al., 2011). In this way, even with possible lower light penetration through the filled adhesives, the catalyst activity of Nb2O5·nH2O could minimize this effect and support the DC. Interestingly, there were no differences in the Rpmax to indicate the adhesive with Nb2O5·nH2O decreased the energy required for the polymerization reaction (Figure 2B). However, the catalytic effect may also have shifted the chain mobility without altering the Rpmax and maintaining DC.
While the catalysis effect could explain the DC values, during the manipulation and polymerization process, differences in the optical properties between groups were observed. Nb2O5 is known to have a high refractive index (Leitune et al., 2013a), and the adhesives in this group presented an opaque white color, while Nb2O5·nH2O containing materials were more translucent and yellowish. These differences could be observed in both uncured and cured adhesives, and this finding may be endorsed in the precursors of Nb2O5·nH2O synthesis since NbCl5 is a yellowish material. However, other features such as the refractive index difference between Nb2O5·nH2O and Nb2O5, the density of the fillers, and the volume incorporated may lead to these color variations. Although color and translucency were not investigated in this study, with this exploratory tuning of both fillers was already possible to identify some interesting differences between Nb2O5·nH2O and Nb2O5.
The balance between the bioactivity and the physicochemical properties is a demand in the study of bioactive dental materials. Niobium pentoxide was shown to induce mineral deposition by the formation of a phosphate-rich layer on 2.5 wt.% and 5 wt.% loaded dental adhesives (Collares et al., 2014). Increasing the quantity of bioactive fillers into the adhesives could lead to a higher release of niobium compounds that may induce higher mineral formation in the adhesive layer as the availability of ions could cause deposition of mineral as described previously (Obata et al., 2012). Nb2O5·nH2O doped adhesives showed improved outcomes, such as higher radiopacity, maintenance of mechanical strength, and lower softening in solvent. With the incorporation of Nb2O5·nH2O up to 10 wt.%, the DC was sustained, suggesting that the control of particle properties may be an alternative for the development of dental adhesives doped with mettalic oxides. It is a limitation of the present study to not have tested the mineral deposition capacity of the formulated adhesives. Future studies are encouraged to evaluate the possible bioactivity of Nb2O5·nH2O doped adhesives.
Conclusion
In this study, we explored two niobium-based fillers for dental adhesives: niobium pentoxide (Nb2O5) and niobic acid (Nb2O5HY). These fillers were tested at 0, 2.5, 5, and 10 wt.%. Overall, Nb2O5 and Nb2O5HY doped adhesives showed improved outcomes, such as higher radiopacity, maintenance of mechanical strength, and lower softening in solvent. Through of addition of Nb2O5HY up to 10 wt.%, the DC was sustained as presented the unfilled adhesive. In conclusion, Nb2O5HY is a promising filler to be incorporated into dental adhesives providing proper mechanical properties, improved resistance against solvents, and increased radiopacity, without changing the DC.
Data Availability Statement
The original contributions presented in the study are included in the article/Supplementary Material, further inquiries can be directed to the corresponding author.
Author Contributions
IG: Investigation, Formal Analysis, Data Curation, Visualization, Writing—Original Draft. VL: Conceptualization, Formal Analysis, Resources, Writing—Review & Editing. GB: Visualization, Writing—Review & Editing. AB: Writing—Original Draft. MM:Writing—Original Draft. SS: Resources, Writing—Review & Editing. FC: Conceptualization, Resources, Writing—Review & Editing, Supervision, Funding acquisition.
Funding
This study was financed in part by the Coordenação de Aperfeiçoamento de Pessoal de Nível Superior—Brasil (CAPES)—Finance Code 001 (scholarship of IG and GB). There was no fund received for open access publication fees.
Conflict of Interest
The authors declare that the research was conducted in the absence of any commercial or financial relationships that could be construed as a potential conflict of interest.
Acknowledgments
AB acknowledge the scholarship during his Ph.D. studies from the Imam AbdulRahman bin Faisal University, Dammam, Saudi Arabia, and the Saudi Arabia Cultural Mission.
References
Altmann, A. S. P., Collares, F. M., Balbinot, G. S., Leitune, V. C. B., Takimi, A. S., and Samuel, S. M. W. (2017). Niobium pentoxide phosphate invert glass as a mineralizing agent in an experimental orthodontic adhesive. Angle Orthod. 87, 759–765. doi:10.2319/122417-140.1
Balbinot, G. S., Collares, F. M., Herpich, T. L., Visioli, F., Samuel, S. M. W., and Leitune, V. C. B. (2020a). Niobium containing bioactive glasses as remineralizing filler for adhesive resins. Dent. Mater. 36, 221–228. doi:10.1016/j.dental.2019.11.014
Balbinot, G. S., Collares, F. M., Visioli, F., Soares, P. B. F., Takimi, A. S., Samuel, S. M. W., et al. (2018). Niobium addition to sol-gel derived bioactive glass powders and scaffolds: in vitro characterization and effect on pre-osteoblastic cell behavior. Dent. Mater. 34, 1449–1458. doi:10.1016/j.dental.2018.06.014
Balbinot, G. S., Leitune, V. C. B., Ogliari, F. A., and Collares, F. M. (2020b). Niobium silicate particles promote in vitro mineral deposition on dental adhesive resins. J. Dent. 101, 103449. doi:10.1016/j.jdent.2020.103449
Balbinot, G. S., Leitune, V. C. B., Ponzoni, D., and Collares, F. M. (2019). Bone healing with niobium-containing bioactive glass composition in rat femur model: a micro-CT study. Dent. Mater. 35, 1490–1497. doi:10.1016/j.dental.2019.07.012
Balhaddad, A. A., Kansara, A. A., Hidan, D., Weir, M. D., Xu, H. H. K., and Melo, M. A. S. (2019). Toward dental caries: exploring nanoparticle-based platforms and calcium phosphate compounds for dental restorative materials. Bioact. Mater. 4, 43–55. doi:10.1016/j.bioactmat.2018.12.002
Belli, R., Kreppel, S., Petschelt, A., Hornberger, H., Boccaccini, A. R., and Lohbauer, U. (2014). Strengthening of dental adhesives via particle reinforcement. J Mech. Behav. Biomed. Mater. 37, 100–108. doi:10.1016/j.jmbbm.2014.05.007
Bendary, I. M., Garcia, I. M., Collares, F. M., Takimi, A., Samuel, S. M. W., and Leitune, V. C. B. (2020). Wollastonite as filler of an experimental dental adhesive. J. Dent. 102, 103472. doi:10.1016/j.jdent.2020.103472
Braga, R. R., and Fronza, B. M. (2020). The use of bioactive particles and biomimetic analogues for increasing the longevity of resin-dentin interfaces: a literature review. Dent. Mater. J. 39, 62–68. doi:10.4012/dmj.2019-293
Brouwer, F., Askar, H., Paris, S., and Schwendicke, F. (2016). Detecting secondary caries lesions: a systematic review and meta-analysis. J. Dent. Res. 95, 143–151. doi:10.1177/0022034515611041
Collares, F. M., Ogliari, F. A., Zanchi, C. H., Petzhold, C. L., Piva, E., and Samuel, S. M. (2011). Influence of 2-hydroxyethyl methacrylate concentration on polymer network of adhesive resin. J. Adhesive Dent. 13, 125–129. doi:10.3290/j.jad.a18781
Collares, F. M., Portella, F. F., Leitune, V. C., and Samuel, S. M. (2013). Discrepancies in degree of conversion measurements by FTIR. Braz. Oral Res. 27, 453–454. doi:10.1590/S1806-83242013000600002
Collares, F. M., Portella, F. F., Fraga, G. C. S., Semeunka, S. M., Almeida, L. C. B., Santos, E. R., et al. (2014). Mineral deposition at dental adhesive resin containing niobium pentoxide. Applied Adhesion Science 2, 6. doi:10.1186/s40563-014-0022-0
Ferracane, J. L. (1985). Correlation between hardness and degree of conversion during the setting reaction of unfilled dental restorative resins. Dent. Mater. 1, 11–14. doi:10.1016/S0109-5641(85)80058-0
Ferracane, J. L. (2006). Hygroscopic and hydrolytic effects in dental polymer networks. Dent. Mater. 22, 211–222. doi:10.1016/j.dental.2005.05.005
Garcia, I. M., Leitune, V. C. B., Balbinot, G. S., Samuel, S. M. W., and Collares, F. M. (2016). Influence of niobium pentoxide addition on the properties of glass ionomer cements. Acta Biomater. Odontol. Scand. 2, 138–143. doi:10.1080/23337931.2016.1239182
Garcia, I. M., Leitune, V. C. B., Ferreira, C. J., and Collares, F. M. (2018). Tantalum oxide as filler for dental adhesive resin. Dent. Mater. J. 37, 897–903. doi:10.4012/dmj.2017-308
Garcia, I. M., Leitune, V. C. B., Samuel, S. M. W., and Collares, F. M. (2017). Influence of different calcium phosphates on an experimental adhesive resin. J. Adhesive Dent. 19, 1–6. doi:10.3290/j.jad.a38997
Garcia, I. M., Souza, V. S., Souza, J. D., Visioli, F., Leitune, V. C. B., Scholten, J. D., et al. (2020b). Zinc-based particle with ionic liquid as a hybrid filler for dental adhesive resin. J. Dent. 102, 103477. doi:10.1016/j.jdent.2020.103477
Garcia, I. M., Leitune, V. C. B., Takimi, A. S., Bergmann, C. P., Samuel, S. M. W., Melo, M. A., et al. (2020a). Cerium dioxide particles to tune radiopacity of dental adhesives: microstructural and physico-chemical evaluation. J. Funct. Biomater. 11. doi:10.3390/jfb11010007
Garcia, I. M., Souza, V. S., Hellriegel, C., Scholten, J. D., and Collares, F. M. (2019). Ionic liquid-stabilized titania quantum dots applied in adhesive resin. J. Dent. Res. 98, 682–688. doi:10.1177/0022034519835203
Hass, V., Dobrovolski, M., Zander-Grande, C., Martins, G. C., Gordillo, L. A., Rodrigues Accorinte, Mde. L., et al. (2013). Correlation between degree of conversion, resin-dentin bond strength and nanoleakage of simplified etch-and-rinse adhesives. Dent. Mater. 29, 921–928. doi:10.1016/j.dental.2013.05.001
Ibrahim, M. S., Balhaddad, A. A., Garcia, I. M., Collares, F. M., Weir, M. D., Xu, H. H. K., et al. (2020a). pH-responsive calcium and phosphate-ion releasing antibacterial sealants on carious enamel lesions in vitro. J. Dent. 97, 103323. doi:10.1016/j.jdent.2020.103323
Ibrahim, M. S., Balhaddad, A. A., Garcia, I. M., Hefni, E., Collares, F. M., Martinho, F. C., et al. (2020b). Tooth sealing formulation with bacteria-killing surface and on-demand ion release/recharge inhibits early childhood caries key pathogens. 108, 3217–3227. doi:10.1002/jbm.b.34659
International Organization for Standardization (2009). ISO 4049:2009—dentistry—Polymer-based restorative materials. Geneva, Switzerland:International Organization for Standardization.
Karlinsey, R. L., Hara, A. T., Yi, K., and Duhn, C. W. (2006). Bioactivity of novel self-assembled crystalline Nb2O5 microstructures in simulated and human salivas. Biomed. Mater. 1, 16–23. doi:10.1088/1748-6041/1/1/003
Karlinsey, R. L., and Yi, K. (2008). Self-assembly and bioactive response of a crystalline metal oxide in a simulated blood fluid. J. Mater. Sci. Mater. Med. 19, 1349–1354. doi:10.1007/s10856-007-3164-9
Leitune, V. C., Collares, F. M., Takimi, A., de Lima, G. B., Petzhold, C. L., Bergmann, C. P., et al. (2013a). Niobium pentoxide as a novel filler for dental adhesive resin. J. Dent. 41, 106–113. doi:10.1016/j.jdent.2012.04.022
Leitune, V. C., Takimi, A., Collares, F. M., Santos, P. D., Provenzi, C., Bergmann, C. P., et al. (2013b). Niobium pentoxide as a new filler for methacrylate-based root canal sealers. Int. Endod. J. 46, 205–210. doi:10.1111/j.1365-2591.2012.02107.x
Lohbauer, U., Wagner, A., Belli, R., Stoetzel, C., Hilpert, A., Kurland, H. D., et al. (2010). Zirconia nanoparticles prepared by laser vaporization as fillers for dental adhesives. Acta Biomater. 6, 4539–4546. doi:10.1016/j.actbio.2010.07.002
Lopes, J. H., Magalhães, A., Mazali, I. O., and Bertran, C. A. (2014). Effect of niobium oxide on the structure and properties of melt-derived bioactive glasses. J. Am. Ceram. Soc. 97, 3843–3852. doi:10.1111/jace.13222
Marins, N. H., Lee, B. E. J., E Silva, R. M., Raghavan, A., Villarreal Carreño, N. L., and Grandfield, K. (2019). Niobium pentoxide and hydroxyapatite particle loaded electrospun polycaprolactone/gelatin membranes for bone tissue engineering. Colloids Surf. B Biointerfaces 182, 110386. doi:10.1016/j.colsurfb.2019.110386
Marins, N. H., Silva, R. M., Ferrua, C. P., Łukowiec, D., Barbosa, A. M., Ribeiro, J. S., et al. (2020). Fabrication of electrospun poly(lactic acid) nanoporous membrane loaded with niobium pentoxide nanoparticles as a potential scaffold for biomaterial applications. J. Biomed. Mater. Res. B Appl. Biomater. 108, 1559–1567. doi:10.1002/jbm.b.34503
Marins, N. H., Meereis, C. T. W., Silva, R. M., Ruas, C. P., Takimi, A. S., Carreño, N. L. V., et al. (2018). Radiopaque dental adhesive with addition of niobium pentoxide nanoparticles. Polym. Bull. 75, 2301–2314. doi:10.1007/s00289-017-2150-8
Mazur, M., Kalisz, M., Wojcieszak, D., Grobelny, M., Mazur, P., Kaczmarek, D., et al. (2015). Determination of structural, mechanical and corrosion properties of Nb2O5 and (NbyCu 1-y)Ox thin films deposited on Ti6Al4V alloy substrates for dental implant applications. Mater. Sci. Eng. C. Mater. Biol. Appl. 47, 211–221. doi:10.1016/j.msec.2014.11.047
Mjor, I. A. (2005). Clinical diagnosis of recurrent caries. J. Am. Dent. Assoc. 136, 1426–1433. doi:10.14219/jada.archive.2005.0057
Münchow, E. A., and Bottino, M. C. (2017). Recent advances in adhesive bonding: the role of biomolecules, nanocompounds, and bonding strategies in enhancing resin bonding to dental substrates. Curr. Oral Health Rep. 4, 215–227. doi:10.1007/s40496-017-0146-y
Nakajima, K., Baba, Y., Noma, R., Kitano, M., Kondo, J. N., Hayashi, S., et al. (2011). Nb2O5·nH2O as a heterogeneous catalyst with water-tolerant Lewis acid sites. J. Am. Chem. Soc. 133, 4224–4227. doi:10.1021/ja110482r
Nowak, I., and Ziolek, M. (1999). Niobium compounds: preparation, characterization, and application in heterogeneous catalysis. Chem. Rev. 99, 3603–3624. doi:10.1021/cr9800208
Obata, A., Takahashi, Y., Miyajima, T., Ueda, K., Narushima, T., and Kasuga, T. (2012). Effects of niobium ions released from calcium phosphate invert glasses containing Nb2O5 on osteoblast-like cell functions. ACS Appl. Mater. Interfaces 4, 5684–5690. doi:10.1021/am301614a
Ogliari, F. A., de Sordi, M. L., Ceschi, M. A., Petzhold, C. L., Demarco, F. F., and Piva, E. (2006). 2,3-Epithiopropyl methacrylate as functionalized monomer in a dental adhesive. J. Dent. 34, 472–477. doi:10.1016/j.jdent.2005.11.001
Par, M., Spanovic, N., Mohn, D., Attin, T., Tauböck, T. T., and Tarle, Z. (2020). Curing potential of experimental resin composites filled with bioactive glass: a comparison between Bis-EMA and UDMA based resin systems. Dent. Mater. 36, 711–723. doi:10.1016/j.dental.2020.03.015
Profeta, A. C. (2014). Dentine bonding agents comprising calcium-silicates to support proactive dental care: origins, development and future. Dent. Mater. J. 33, 443–452. doi:10.4012/dmj.2013-267
Rodrigues, S. B., Collares, F. M., Leitune, V. C., Schneider, L. F., Ogliari, F. A., Petzhold, C. L., et al. (2015). Influence of hydroxyethyl acrylamide addition to dental adhesive resin. Dent. Mater. 31, 1579–1586. doi:10.1016/j.dental.2015.10.005
Salehi, S., Gwinner, F., Mitchell, J. C., Pfeifer, C., and Ferracane, J. L. (2015). Cytotoxicity of resin composites containing bioactive glass fillers. Dent. Mater. 31, 195–203. doi:10.1016/j.dental.2014.12.004
Schneider, L. F., Moraes, R. R., Cavalcante, L. M., Sinhoreti, M. A., Correr-Sobrinho, L., and Consani, S. (2008). Cross-link density evaluation through softening tests: effect of ethanol concentration. Dent. Mater. 24, 199–203. doi:10.1016/j.dental.2007.03.010
Shortall, A. C. (2005). How light source and product shade influence cure depth for a contemporary composite. J. Oral Rehabil. 32, 906–911. doi:10.1111/j.1365-2842.2005.01523.x
Skrodczky, K., Antunes, M. M., Han, X., Santangelo, S., Scholz, G., Valente, A. A., et al. (2019). Niobium pentoxide nanomaterials with distorted structures as efficient acid catalysts. Communications Chemistry 2, 11–13. doi:10.1038/s42004-019-0231-3
Stürmer, M., Garcia, I. M., Souza, V. S., Visioli, F., Scholten, J. D., Samuel, S. M. W., et al. (2020). Titanium dioxide nanotubes with triazine-methacrylate monomer to improve physicochemical and biological properties of adhesives. Dent. Mater. doi:10.1016/j.dental.2020.11.004
Tanabe, K. (2003). Catalytic application of niobium compounds. Catal. Today 78, 65–77. doi:10.1016/s0920-5861(02)00343-7
Toledano, M., Osorio, R., Osorio, E., García-Godoy, F., Toledano-Osorio, M., and Aguilera, F. S. (2016). Advanced zinc-doped adhesives for high performance at the resin-carious dentin interface. J. Mech. Behav. Biomed. Mater. 62, 247–267. doi:10.1016/j.jmbbm.2016.05.013
Yao, C., Ahmed, M. H., Li, X., Nedeljkovic, I., Vandooren, J., Mercelis, B., et al. (2020). Zinc-calcium-fluoride bioglass-based innovative multifunctional dental adhesive with thick adhesive resin film thickness, ACS Appl. Mater. Interfaces 12, 30120–30135. doi:10.1021/acsami.0c06865
Keywords: niobium, dentin-bonding agents, polymerization, mechanical phenomena, dental materials
Citation: Garcia IM, Leitune VCB, Balbinot GS, Balhaddad AA, Melo MAS, Samuel SMW and Collares FM (2021) Physicochemical Effects of Niobic Acid Addition Into Dental Adhesives. Front. Mater. 7:601078. doi: 10.3389/fmats.2020.601078
Received: 31 August 2020; Accepted: 21 December 2020;
Published: 02 February 2021.
Edited by:
Masoud Mozafari, University of Toronto, CanadaReviewed by:
Matej Par, University of Zagreb, BrazilVictor Pinheiro, Feitosa, Faculdade Paulo Picanço, Brazil
Copyright © 2021 Garcia, Leitune, Balbinot, Balhaddad, Melo, Samuel and Collares. This is an open-access article distributed under the terms of Creative Commons Attribution License (CC BY). The use, distribution or reproduction in other forums is permitted, provided the original author(s) and the copyright owner(s) are credited and that the original publication in this journal is cited, in accordance with accepted academic practice. No use, distribution or reproduction is permitted which does not comply with these terms.
*Correspondence: Fabrício Mezzomo Collares, ZmFicmljaW8uY29sbGFyZXNAdWZyZ3MuYnI=
†ORCID:
Isadora Martini Garcia
https://orcid.org/0000-0002-7388-0200
Vicente Castelo Branco Leitune
https://orcid.org/0000-0002-5415-1731
Gabriela de Souza Balbinot
https://orcid.org/0000-0001-9076-2460
AbdulRahman A. Balhaddad
https://orcid.org/0000-0001-6678-7940
Mary Anne S. Melo
https://orcid.org/0000-0002-0007-2966
Susana Maria Werner Samuel
https://orcid.org/0000-0001-5467-2636
Fabrício Mezzomo Collares
https://orcid.org/0000-0002-1382-0150