- 1Laboratory of Mineral Characterization, Institute of Geosciences, Federal University of Pará, Belém, Brazil
- 2Laboratory of Conservation, Restoration and Rehabilitation, Institute of Technology, Federal University of Pará, Belém, Brazil
One of the most important studies from historic mortars is the binder:aggregate ratio, which is usually obtained through wet chemical analysis. Instrumental techniques and benchtop equipment have become increasingly important tools in the characterization of historic materials. The analysis of such materials has become more practical, faster and more accurate, and the sample preparation methods require less and less material. Thus, this article aims to investigate the validity of the results obtained by some of the methods and techniques used in historic materials analysis and determine the possibility of estimating the binder:aggregate ratio with adequate accuracy and precision. For this purpose, historic mortars from Belém do Pará, in northern Brazil, were selected, and the following quantification techniques were employed: wet chemical analysis, XRD, DSC and XRF. The results showed that the amounts of the components in the mortars could be quantified with the use of approximately 3 g of sample, thus providing one of the main pieces of information needed for the production of a restoration mortar: the binder:aggregate ratio.
Introduction
Historic masonry structures often require restorative interventions, and the render and plaster mortars is the portion of structures that most often requires restoration because it is the area of greatest exposure to weathering and mechanical impacts. This is the most common material used by builders due to its ease of formulation, preparation and application and its low cost.
In the past, mortars were quite heterogeneous in terms of their particle size distribution, chemical and mineralogical composition, degree of mixing and component ratio. This heterogeneity produced buildings and/or environments that were unique “pieces” and not a series of equal “pieces”, as is common today. Thus, the composition and properties of these materials deserve a thorough investigation when restoration is required (Hormes et al., 2016).
The main binder used until the end of the 19th century was air lime, which was later replaced by Portland cement. The latter gave the mortars a high mechanical strength, low permeability and shorter curing time. However, when applied to historic constructions, Portland cement causes the appearance of moisture stains, salt efflorescence, flaking and fissures. These effects reinforce the need to develop alternatives for use in restoration (Pacheco-Torgal et al., 2012; Barbero-Barrera et al., 2014). Therefore, studies on alternative materials that are more compatible with and representative of the original materials are increasingly common. In addition, there is an ongoing discussion about the best use of analytical methods to more accurately and precisely estimate the composition of an original “piece”.
The different techniques and characterization methods, together with historic data, are used to determine which components were used in the original material and to obtain data on their state of conservation. Based on such information, strategies are adopted for the restoration and preservation of historic assets to avoid or minimize incompatibilities between the new and preexisting materials. Using some techniques and methods, such as X-ray fluorescence (XRF), X-ray diffractometry (XRD), Optical Microscopy (OM) differential scanning calorimetry (DSC), and scanning electron microscopy and chemical microanalysis (SEM-EDS), the chemical and mineralogical composition of a material can be determinate to estimate its main characteristics and properties without the need for physical and mechanical tests, which are infeasible when only small irregular fragments are available for characterization (Franzini et al., 2000).
The binder:aggregate ratio used in the formulation of historic mortars is one of the most important information that needs to be determined and is usually obtained through wet chemical analysis (Casadio et al., 2005; Middendorf et al., 2005). For this purpose, in calcitic air limed mortars, an assay based on the acid dissolution of the binder may be used, and the aggregate is considered to remain intact. Then, the result is given by a simple mass balance that considers the following points: 1) all the “Ca(OH)2” binder in contact with atmospheric CO2 is transformed into CaCO3, according to Eq 1; 2) all CaCO3 is dissolved by an HCl solution (Eq 2); and 3) the undissolved component is the aggregate, according to Eq 3.
The wet chemical analysis method, when used alone, does not provide complete information about a mortar or its state of preservation and may raise doubts about whether the aggregate is partially or completely dissolved by the acid (Casadio et al., 2005; Middendorf et al., 2005). Thus, for a more detailed understanding of the historic material to be restored, techniques and analytical methods must be combined, for example, by combining total chemical analysis, mineralogical analysis and textural analysis. In addition, consulting historic information that helps to determine the material composition, such as the date of construction and the architectural style, is important (Middendorf et al., 2005).
With advances in instrumental techniques and the emergence of benchtop equipment, the aforementioned analyses have become more practical, faster and more accurate, and the sample preparation methods consume less material compared to the methods used for wet chemical analysis, as previously discussed.
The question that emerges in this context is as follows: how can the binder:aggregate ratio of air lime-based historic mortars be estimated for restoration? It is noteworthy that, in addition to the already known and well-discussed wet chemical analysis method (acid attack), a combination of techniques is already used. Instrumental techniques already used for this purpose include DSC, XRD, XRF, OM and SEM (Hughes & Cuthbert, 2000; Moropoulou et al., 2000; Callebaut et al., 2001; Moropoulou et al., 2002; Maravelaki-Kalaitzaki et al., 2003; Casadio et al., 2005; Middendorf et al., 2005; Böke et al., 2006; Adriano et al., 2009; Fiori et al., 2009; Gleize et al., 2009; Gliozzo et al., 2009; Ciarallo et al., 2010; Aggelakopoulou et al., 2011; Theodoridou et al., 2013; Borges et al., 2014; Matias et al., 2014; Singh et al., 2016; Botas et al., 2017; Damas et al., 2018).
Thus, this study aimed to investigate the validity of the results obtained by some of the methods and techniques listed above and assess the possibility of estimating the binder:aggregate ratio with adequate accuracy and precision. Furthermore, the study aims to determine the compatibility of the results obtained by each technique and the necessity of combining various techniques to better characterize a material.
Finally, the work also aimed to show how statistical data can be explored in order to give more reliability to the results, indicating which parameters should be used in each technique, mainly in XRD, which is not so explored by other studies that involve determination of the binder: aggregate ratio in historic mortars.
Materials and Methods
Description of Samples
To meet the main goal of the study, which was to compare different techniques and methods to determine the binder:aggregate ratio and discuss the advantages and disadvantages of each, two samples of lime-based mortars from historic buildings in Belém do Pará, Brazil were selected. The buildings include the Arquivo Público do Estado do Pará and Casa Quintino, described in Figure 1. It is noteworthy that, in this study, all carbonate material was considered as a binder to determine the binder:aggregate ratio, including shell fragments, even knowing that behave as an aggregate. Although this choice may be questionable, this decision has been taken because the technics used in this paper cannot distinguish the carbonate binder and the carbonate aggregate, and the results can be useful when there is no carbonate aggregate. Thus, knowing the limitation of each method, it is possible to make more accurate decisions to protect cultural heritage.
Material Characterization
In this assay, a sample weighing ∼20 g was used, with ∼10 g for each replicate and follow the recommendations of similar studies (Alvarez et al., 1999; Gleize et al., 2000; Middendorf et al., 2005). The acid attack of the samples (total sample) was performed using a 1:4 solution of hydrochloric acid and distilled water at room temperature. At the end of the attack, only the insoluble fraction remained (quartz and kaolinite aggregates), and this was filtered and carefully washing with deionized water, and then, the residue was dried and weighed. The binder:aggregate ratio (soluble fraction:insoluble fraction) was determined by gravimetry.
X-Ray Diffraction (XRD) - Rietveld
For the mineralogical analysis, ∼3 g of total sample was pulverized in an agate pestle, the powders were back-loaded into an aluminum holder and then measured by XRD. Subsequently, 20% of an internal standard (fluorite) was added to the total mass to estimate the potential contribution of the amorphous phase, and XRD measurements were taken again.
The samples were analyzed in a PANalytical EMPYREAN diffractometer, with a θ/θ goniometer, a ceramic X-ray tube and a Co anode (Kα1 = 1.789 Å), with Generator Settings 40 mA voltage and 40 kV electric current, and a Kβ nickel filter. The divergence slit = 1/4° rad, the ant-scattering slit = 1/2° rad, and anti-scattering slit of 10 mm diffracted beam. Diffraction profiles were obtained in the 3–111 [°2Th] range using a step size 0.0070 [°2Th], and sample in a circular motion with frequency of 1 rotation/sec. Total analysis time was ∼20 min. Phase quantification was performed by the Rietveld method using the PANalytical X'Pert HighScore Plus software. Structural models for all the phases were taken from the ICSD database: quartz (code 98–011-5,342), calcite (code 98–011-0,798), kaolinite (code 98–005-2,637), and fluorite (code 98–002-1,688).
The statistical indices used as a criterion to judge the quality of the Rietveld refinement were: 1) Rwp = minimized value by the least-squares method, 2) Rexp = minimum value for Rwp that can be expected statistically, and 3) χ2 = chi-square value (Rwp/Rexp)2.
Rational Calculation for Mineralogical Quantification (XRD Combined With XRF)
To estimate the mineralogical composition, ∼3 g of total sample was pulverized in an agate pestle and then the total oxides identified by XRF (Al2O3, SiO2 and CaO) were converted into the minerals identified by XRD, assuming logical considerations regarding the partitioning of the elements.
The following conversions were identified:
Because the XRF analysis is destructive, the XRD measurements were performed first, so that the same material used for phase identification could be used to determine the chemical composition. Sample preparation for XRF was performed using fused beads containing 1 g of total sample pulverized (<200 μm) to 6 g of lithium tetraborate (flux). The loss on ignition was determined by the mass difference after calcination of 1 g of total sample at 1,000°C for approximately 2 h (previously dried at 105°C).
The XRD analyses followed the specifications mentioned in section 2.2.2, and the XRF analyses were performed in a PANalytical Axios-Minerals X-ray Fluorescence Spectrometer, with a ceramic X-ray tube, a rhodium anode (Rh), and 4 kW of power. The data were acquired using the SuperQ Manager software, and the data were treated using the PANalytical Ominian software.
Differential Scanning Calorimetry (DSC) Combined With XRD (Phases Identification)
For this analysis, the mineral phases were first identified by XRD following the specifications mentioned in section 2.2.2. Next, ∼30 mg of total sample was subjected to DSC analysis using the NETZSCH STA 449F3 Jupiter equipment with a simultaneous thermal analyzer from NETStanton Redcroft Ltda, a vertical cylindrical platinum furnace, a temperature range from 25°C to 1,000°C, a nitrogen flow rate of 50 ml/min, a heating rate of 5°C/min and a platinum crucible as a reference. The total measurement time was 3 h 15 min.
The results were interpreted using the Proteus software from NETZSCH. The calibration factor was determined using the following substances and their respective boiling points: In (156.6°C), Sn (231.9°C), Bi (271.4°C), Zn (419.6°C), Al (660.6°C), and Au (1,064.4°C).
The calcite content was determined using a shell fragment found in one of the mortars as a standard because shells were used as a raw material to obtain lime (Loureiro et al., 2020). It emphasizes that, in this study, the shell fragments were considered as a binder.
The applied methodology was based on the study developed by Paz et al. (Paz et al., 2017). According to this methodology, the heat of the decarbonation reaction measured by DSC ∼750°C can be used for phase quantification because the ratio between the peak area and the enthalpy can be determined using a temperature-dependent calibration factor. Thus, the peak A area depends on the sample mass m (g), the enthalpy of the reaction ΔH (J g−1) and an empirical constant K (sensor sensitivity) (Eq 12).
With this information, the percentage of calcite in the samples was determined by the ratio between the ΔH value of CaCO3 decarbonation and the ΔH value of standard CaCO3 decarbonation (shell fragment) and subsequently multiplied by 100 (Eq 13).
Based on the percentage of calcite, the aggregate content present in the samples (kaolinite and quartz aggregates) was determined according to Eq 5, and thus, the binder:aggregate ratio of the mortars could be determined.
Determination of the binder:aggregate Ratio in Volume
The mass percentages of the binder and aggregate found through the analytical techniques were converted into a binder:aggregate ratio in volume, considering a unit mass of the aggregate of 1.35 g/cm³ and a unit mass of the calcium hydroxide of 0.46 g/cm³; these values were defined in the characterization of the material by Loureiro et al. (Loureiro et al., 2020).
Results and Discussion
Wet Chemical Separation (Acid Dissolution) to Determine Binder and Aggregate Contents
As shown in Figure 2, using the acid dissolution technique, the binder content of all of the mortars was lower than the aggregate content. The binder:aggregate ratios in volume were 1:1.4 and 1:8.8 for Arquivo Público and Casa Quintino, respectively.
With this technique, it was not possible to identify degradation products to indicate the presence of soluble salts or to determine precisely which types of aggregate were present, for example, the amount of quartz and kaolinite in the aggregate, and also is limited when there is carbonate aggregate, because this is dissolved by the acid hydrochloric. However, after the acid attack, it was possible to perform assays to determine the particle size distribution of the aggregate, which is of great value for a good historic material restoration.
In Figure 2, it is possible to verify that the results obtained by each replicate are statistically equal, demonstrating that the results are reliable. Although it is a widespread technique for characterizing historic mortars, statistical data are not shown frequently, which could be further explored and, thus, give more reliability to the results presented.
(Casadio et al., 2005), shows that the use of some acids is not suitable for determining the binder: aggregate ratio when there is carbonate aggregate, including HCl since it overestimates the binder content because there is the significant dissolution of the aggregate fraction, which also occurred in this study. However, the aim of the present study was to show how to determine the binder:aggregate ratio considering that shell fragments are binder, as already mentioned. Thus, we expose each applied method limitations are exposed, making it possible to make better decisions to protect cultural heritage.
X-Ray Diffraction–Rietveld Method
The diffractograms revealed three mineral phases: calcite, kaolinite and quartz. Calcite indicates that air lime was the only binder used in the mixture, whereas quartz and kaolinite indicate the use of a sandy aggregate with a small amount of clay, typical characteristics of historic mortars from Belém do Pará, Brazil (Loureiro et al., 2015; Loureiro et al., 2020).
As expected, the XRD-Rietveld analysis revealed that the aggregate content was higher than the binder content, and the mortars with the highest binder:aggregate ratio were those belonging to the Arquivo Público. These results agree with the results obtained by the wet chemical analysis. Thus, the binder:aggregate ratios were 1:1.5 and 1:10.3 for Arquivo Público and Casa Quintino, respectively (Figure 3).
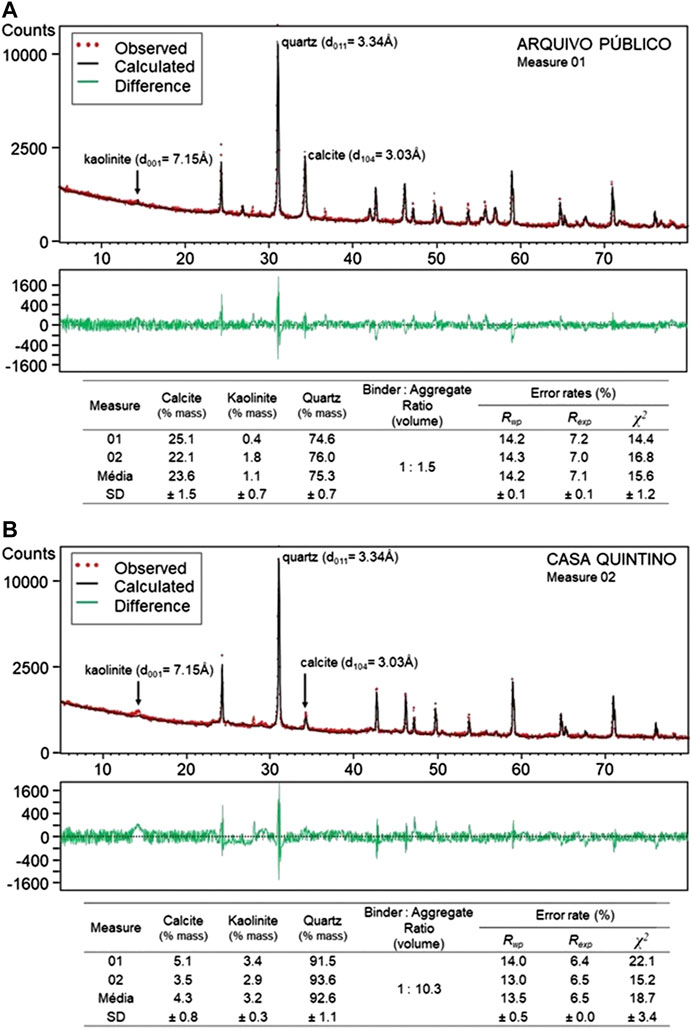
FIGURE 3. Diffractogram of historic mortars and the content of each mineral identified: (A) Arquivo Público and (B) Casa Quintino.
The statistical indices show that the Rietveld refinement for samples without an internal standard was not so satisfactory when compared to another study developed by Piovesan et al., 2013, in which the Rwp indexes varied from 6.5 to 11.5, which may indicate that the proposed refinement for the mortar samples from Belém do Pará did not achieve good results, which the Rwp were 14.2 and 13.5 for Arquivo Público and Casa Quintino, respectively. On the other hand, it is known that the low values of the error indexes do not always indicate the best refinement, as this is only one of the criteria to evaluate the quality of the Rietveld refinement, and it is also necessary to have a great value graphic analysis to verify if there is a good refinement (Toby, 2006).
It is worth noting that, although other studies also explore the Rietveld refinement, e.g., Casadio et al. (Casadio et al., 2005) and Elsen et al. (Elsen et al., 2010), only a few show the error rates, e.g., Piosevan et al. (2013), which can provide a precious information for other research of similar approach.
The addition of the internal standard made it possible to define the amorphous phase content and showed that the results did not diverge from the results of the XRD analysis without an internal standard, which means that the results were statistically equal. Thus, the quantification indicated binder:aggregate ratios of 1:1.7 and 1:12 for Arquivo Público and Casa Quintino, respectively. The addition of the internal standard improved the values of the error indices Rwp, Rexp and χ2, i.e., there was an improvement in the Rietveld refinement, which indicates greater accuracy in the results (Figure 4).
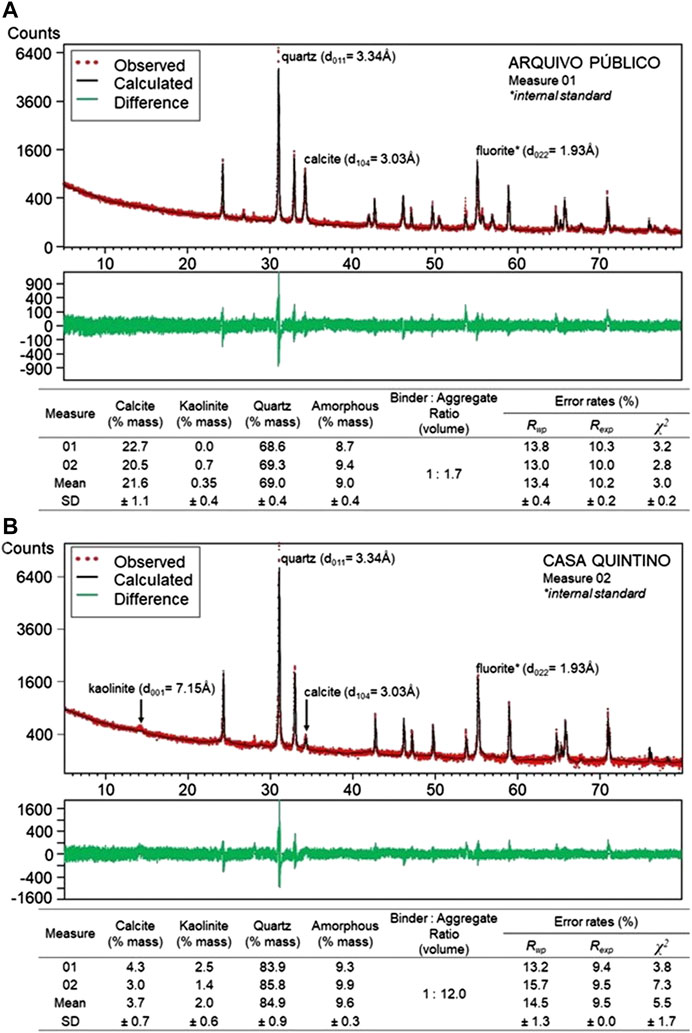
FIGURE 4. Diffractogram of historic mortars with internal standard and the content of each mineral identified: (A) Arquivo Público and (B) Casa Quintino.
The diffractograms with and without internal standards showed that the calculated graph has a lower intensity than the observed graph, which has a direct effect on the quantification. This may occur due to defects in the crystalline structure of the minerals, which increase and decrease the intensity of the peaks. This difference leads to an underestimation of the content of the minerals being quantified (da Paz, 2016).
The χ2 values can be considered satisfactory, particularly for the quantification by XRD with an internal standard, for which χ2 < 5 in the Arquivo Público mortars and χ2 ∼ 5 in the Casa Quintino mortars. The Rwp and Rexp values were also satisfactory, since the minimum value for Rwp that can be expected statistically (Rexp) was close to the Rwp found in the Rietveld refinement.
Rational Calculation for Mineralogical Quantification
Figure 5 shows the major chemical elements (representation in total oxides) quantified by XRF, which were used for the mineralogical quantification by rational calculation. The results indicated that the highest CaO contents were observed in the Arquivo Público mortars, while the highest Al2O3 and SiO2 contents were in the Casa Quintino mortars.
The rational calculation showed the same trend as the methods and analytical techniques described earlier: a predominance of aggregate in all the samples and a higher binder:aggregate ratio in the Arquivo Público mortars. Thus, the quantification indicated binder:aggregate ratios of 1:1.6 and 1:9.9 for Arquivo Público and Casa Quintino, respectively (Figure 6).
In the rational calculation method, all the Al2O3 quantified by XRF was assumed to belong to kaolinite; therefore, the possible presence of other mineral(s) with aluminum was disregarded in this estimate, which may have resulted in an overestimation of the kaolinite content. The most likely hypothesis for the presence of another phase containing aluminum is attributed to metakaolinite (Si2Al2O7) because this amorphous material could be included in the historic mortars from Belém do Pará (Loureiro et al., 2020).
In general, the kaolinite content determined by rational calculation was higher than that found by the Rietveld method, which can be attributed to three factors: 1) the kaolinite content was underestimated by the Rietveld quantification, as shown in section 3.2; 2) the detection limit of XRF was higher; and 3) the kaolinite content was overestimated in the rational calculation method due to the presence of metakaolinite.
It should be noted that there was a small difference between the theoretical loss on ignition and the experimental loss on ignition, as shown in Figure 7. This may be related to the high limit of detection of XRF and the possible presence of organic matter, which may sometimes be present in the matrix of historic mortars.
The binder content determined by the rational calculation method, which combined XRD and XRF, was consistent with that determined by the other methods and analytical techniques, indicating a satisfactory binder:aggregate ratio, i.e., it was possible to extract the main information aimed in this study. On the other hand, although the techniques show that it is possible to obtain the aggregate content correctly, it is not possible to rely on the kaolinite and quartz contents separately, because the kaolinite content was different from the kaolinite content obtained by other techniques, which can lead to a misinterpretation.
Differential Scanning Calorimetry Combined With XRD (Phases Identification)
Figure 8 shows the temperature and enthalpy of decarbonation of the standard calcium carbonate (shell fragment), which were 736.7°C and −1322 J/g, respectively. These results indicate that this standard is in accordance with what is found in air lime-based historic mortars because endothermic reactions above 500°C indicate calcite, which is nothing more than carbonated lime (Aggelakopoulou et al., 2011; Borges et al., 2014; Silva et al., 2010; Gameiro et al., 2014; Bakolas et al., 1995; Bakolas et al., 1995).
Figure 9 shows the main events in the samples during the DSC analysis: 1) kaolinite dehydroxylation, 2) transformation of alpha quartz into beta quartz, 3) calcite decarbonation, and 4) crystallization of mullite/spinel. This figure also shows the thermal region of decarbonation and the area formed by this endothermic reaction, which is used to quantify the calcite. Thus, binder:aggregate ratios of 1:1.6 and 1:41.6 for Arquivo Público and Casa Quintino, respectively, were estimated.
Differences were observed between the decarbonation temperatures of the samples due to three factors: 1) the presence of a microcrystalline structure with smaller crystals than a natural material (standard calcium carbonate), 2) the defective state of the crystalline structure, and 3) the different calcite content in these samples (Moropoulou et al., 1995; Bakolas et al., 1998; Alvarez et al., 2000).
Although the value obtained using DSC for the calcite contained in the Casa Quintino sample was underestimated when compared to the results of the other techniques, the same trend was revealed (analysis in duplicate) for the binder:aggregate ratio: the Arquivo Público mortar has at least 3x more binder than the Casa Quintino mortar.
An endothermic event at ∼450°C, which is related to kaolinite dehydroxylation, was observed in the two samples, with a smaller area in the Arquivo Público sample. Additionally, an exothermic event ∼900°C, attributed to the formation of mullite/spinel from metakaolinite, showed similar areas in the two samples, which indicates the possible presence of metakaolinite in the Arquivo Público sample.
Comparison of Methods and Techniques
Figure 10 shows the binder: aggregate ratio in volume identified by all methods and analytical techniques: 1) Arquivo Público and 2) Casa Quintino.
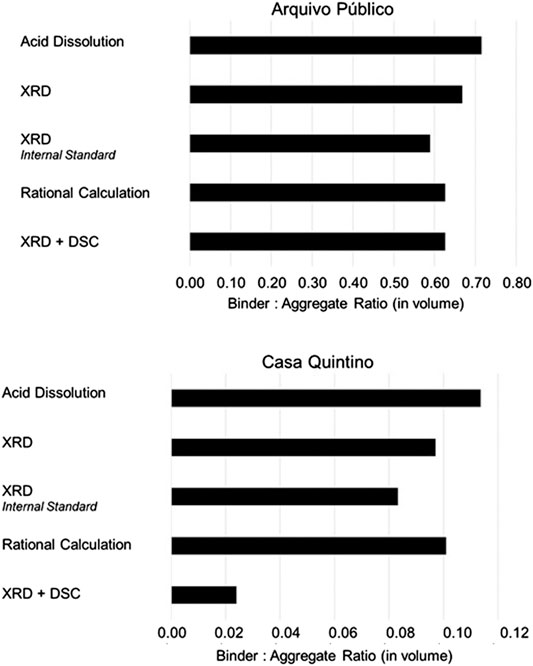
FIGURE 10. Binder: aggregate ratio in volume identified by all methods and analytical techniques: (A) Arquivo Público and (B) Casa Quintino.
Based on the binder:aggregate ratio obtained by each technique, it is possible to state that the Arquivo Público has a strong mortar because the high amount of binder ensures a better bond between the components and a greater mechanical strength, unlike the Casa Quintino mortar. This result is consistent with the fact that the Arquivo Público is a public building that was probably considered to be more noble at the time it was built and therefore of better construction (Velosa and Veiga, 2016). In addition, by analogy with other mortars of the same location and of the same period (Loureiro et al., 2020), it is possible to estimate that the Arquivo Público mortar has a higher capillary coefficient and is more deformable than the Casa Quintino mortars.
In general, because it is a very heterogeneous material, the standard deviation in almost all analyzes was high. This heterogeneity may be related to the rudimentary preparation techniques of historic mortars, which makes it difficult to obtain very close results. This also makes clear the importance of having at least duplicate analyzes in the case of historic mortars, in order to achieve the most representative value for restoring the structure.
The binder value estimated by acid dissolution had more approximate values with the analysis of XRD, XRD with an internal standard, and FRX, which differed, respectively, 0.7, 1.4, and 0.8%, in Casa Quintino mortar and 1.6, 3.6, and 3.2% in Arquivo Público mortar. On the other hand, DSC analysis was the one that most distanced itself from the other results obtained, with a difference of 3.9% in Casa Quinino mortars and 15.1% in Arquivo Público mortar.
In the wet chemical analysis, everything dissolved by the acid is considered to be the binder, which may involve soluble components other than calcite and carbonate aggregates; this is most likely the reason why this technique has the highest binder:aggregate ratio. Such analysis demands a sample mass that can sometimes be unfeasible depending on the restoration project. Another slightly more general observation about the use of this technique involves safety in the workplace. The use of a strong and concentrated acid makes the environment unhealthy and dangerous. The reagent used is highly controlled by public safety agencies (in Brazil, it is controlled by the Army), and a specific authorization is required to purchase the product. However, among the techniques used in this study, this was the only assay that allowed the determination of the particle size of the aggregate because it is not necessary for the sample to be previously ground.
In the XRD analysis, the addition of an internal standard increases the accuracy of the results; however, for the studied samples, there was no significant difference between the results with and without an internal standard. The importance of the internal standard is due to the possibility of detecting the amorphous phase; however, once the standard is added, there is no possibility of removing it, which precludes the reuse of the mortar for other analytical techniques.
The rational calculation showed agreement in the binder:aggregate ratios obtained by the other techniques, but a predominance of kaolinite over calcite was observed in the Casa Quintino mortar, which indicated an overestimated kaolinite content. This makes quantification doubtful, but there was good accuracy in the quantification of the binder:aggregate ratio, which was the main information to be determined in this study.
Although the XRD with internal standard indicated the presence of an amorphous phase, only DSC analysis indicated that this amorphous phase could be metakaolinite. This hypothesis has been validated with the high-temperature phase that appears in an exothermic event ∼900°C. However, quantitatively, the results obtained by DSC showed a significant difference in the calcite content in the Casa Quintino mortar, although this analysis was compatible with the other techniques in the quantification of calcite in the Arquivo Público mortar. This may be an indication that quantification becomes infeasible in the case of mortars with a low binder content.
Conclusions
The research showed that the binder:aggregate ratio estimates provided by wet chemical analysis and other proposed analytical techniques, namely, XRD, XRD + XRF, XRD + DSC, were statistically equal, except for the quantification of calcite by DSC in the Casa Quintino mortar. However, more than twice the sample mass was used in the wet chemical analysis compared to the other techniques.
The XRD analysis with an internal standard is the only method that can quantitatively indicate the amorphous material content, but DSC is able to more identify the kind of amorphous material present, e.g., the possible presence of metakaolinite in the Arquivo Público samples.
In general, with approximately 3 g of sample, the content of the components present in lime-based mortars could be quantified to provide one of the main necessary pieces of information for the production of a restoration mortar: the binder:aggregate ratio.
Despite the application of common methods in relatively simple mortar samples, the authors believe that the results obtained and the use of auxiliary statistical data help in the perception of which results are reliable and, thus, the limitations of each method are evident, which makes it is possible to make better decisions to protect cultural heritage and enrich a more scientific discussion about the data provided by each of the techniques.
However, old mortars are sometimes more complex, with more than one binder, aggregates with different natures, additives and degradation products. Therefore, whenever possible, a combination of techniques should be used, namely, some of the techniques described in this paper, to obtain a more reliable and complete characterization.
Data Availability Statement
The raw data supporting the conclusions of this article will be made available by the authors, without undue reservation.
Author Contributions
AL: Conceptualization, Methodology, Formal Analysis, Investigation, Resources, Data Curation, Writing–Original Draft, Writing–Review and Editing, Visualization, Project administration. SP: Conceptualization, Methodology, Formal Analysis, Investigation, Resources, Data Curation, Writing–Original Draft, Writing–Review and Editing, Visualization, Supervision, Project administration. RA: Conceptualization, Methodology, Formal Analysis, Investigation, Resources, Data Curation, Writing–Original Draft, Writing–Review and Editing, Visualization, Supervision, Project administration
Conflict of Interest
The authors declare that the research was conducted in the absence of any commercial or financial relationships that could be construed as a potential conflict of interest.
Acknowledgments
The authors thank the Brazilian agencies CAPES (Brazilian Federal Agency for Support and Evaluation of Graduate Education) and CNPq (National Council for Scientific and Technological Development) for the doctoral scholarship granted to the first author of this article. We also thank the laboratories where the analyses were performed: a) the Mineral Characterization Laboratory (LCM) and b) the Conservation, Restoration and Rehabilitation Laboratory (LACORE). We thank Rosário Veiga (LNEC) for the critical reading of and contributions to the manuscript. We also thank DPHAC (Department of Historic, Artistic and Cultural Heritage of Pará) for permission to collect the samples used in this study. The authors also thank the Dean’s Office for Graduate and Research Studies (Education) (Federal University of Pará) for funding the translation of the article through the Support Program for Translation and Publication of Brazilian Authors (PUBLIC NOTICE PAPQ 2019).
References
Adriano, P., Santos Silva, A., Veiga, R., Mirão, J., and Candeias, A. E. (2009). Microscopic characterisation of old mortars from the santa maria church in évora. Mater. Char. 60, 610–620. doi:10.1016/j.matchar.2008.11.008
Aggelakopoulou, E., Bakolas, A., and Moropoulou, A. (2011). Properties of lime-metakolin mortars for the restoration of historic masonries. Appl. Clay Sci. 53, 15–19. doi:10.1016/j.clay.2011.04.005
Alvarez, J. I., Navarro, I., Martı́n, A., and Garcı́a Casado, P. J. (2000). A study of the ancient mortars in the north tower of Pamplona’s San Cernin church. Cement Concr. Res. 30, 1413–1419. doi:10.1016/S0008-8846(00)00325-2
Alvarez, J. I., Martı́n, A., Garcı́a Casado, P. J., Navarro, I., and Zornoza, A. (1999). Methodology and validation of a hot hydrochloric acid attack for the characterization of ancient mortars, Cement Concr. Res. 29, 1061–1065. doi:10.1016/s0008-8846(99)00090-3
Bakolas, A., Biscontin, G., Contardi, V., Franceschi, E., Moropoulou, A., Palazzi, D., et al. (1995). Thermoanalytical research on traditional mortars in venice. Thermochim. Acta 269–270, 817–828. doi:10.1016/0040-6031(95)02574-X
Bakolas, A., Biscontin, G., Moropoulou, A., and Zendri, E. (1998). Characterization of structural byzantine mortars by thermogravimetric analysis. Thermochim. Acta 321, 151–160. doi:10.1016/S0040-6031(98)00454-7
Bakolas, A., Biscontin, G., Moropoulou, A., and Zendri, E. (1995). Characterization of the lumps in the mortars of historic masonry. Thermochim. Acta 269–270, 809–816. doi:10.1016/0040-6031(95)02573-1
Barbero-Barrera, M. d. M., Maldonado-Ramos, L., Van Balen, K., García-Santos, A., and Neila-González, F. J. (2014). Lime render layers: an overview of their properties. J. Cult. Herit. 15, 326–330. doi:10.1016/j.culher.2013.07.004
Böke, H., Akkurt, S., İpekoğlu, B., and Uğurlu, E. (2006). Characteristics of brick used as aggregate in historic brick-lime mortars and plasters. Cement Concr. Res. 36, 1115–1122. doi:10.1016/j.cemconres.2006.03.011
Borges, C., Santos Silva, A., and Veiga, R. (2014). Durability of ancient lime mortars in humid environment. Construct. Build. Mater. 66, 606–620. doi:10.1016/j.conbuildmat.2014.05.019
Botas, S., Veiga, R., and Velosa, A. (2017). Air lime mortars for conservation of historic tiles: bond strength of new mortars to old tiles. Construct. Build. Mater. 145, 426–434. doi:10.1016/j.conbuildmat.2017.04.027
Callebaut, K., Elsen, J., Van Balen, K., and Viaene, W. (2001). Nineteenth century hydraulic restoration mortars in the Saint Michael’s Church (Leuven, Belgium). Cement Concr. Res. 31, 397–403. doi:10.1016/S0008-8846(00)00499-3
Casadio, F., Chiari, G., and Simon, S. (2005). Evaluation of binder/aggregate ratios in archaeological lime mortars with carbonate aggregate: a comparative assessment of chemical, mechanical and microscopic approaches*. Archaeometry 47 (4), 671–689. doi:10.1111/j.1475-4754.2005.00226.x
Ciarallo, A., Gazineo, F., Barca, D., Crisci, G. M., Bloise, A., De Rose, T., et al. (2010). Characterisation of archaeological mortars from Pompeii (Campania, Italy) and identification of construction phases by compositional data analysis. J. Archaeol. Sci. 37, 2207–2223. doi:10.1016/j.jas.2010.03.019
da Paz, S. P. A. (2016). Desenvolvimento e otimização de métodos de controle de qualidade e de processo de beneficiamento para bauxitas gibbsíticas tipo-Paragominas. São Paulo: Universidade de São Paulo, 220.
Damas, A. L., Veiga, M. d. R., Faria, P., and Silva, A. S. (2018). Characterisation of old azulejos setting mortars: a contribution to the conservation of this type of coatings. Construct. Build. Mater. 171, 128–139. doi:10.1016/j.conbuildmat.2018.03.103
Gleize, P., Silva, D. A., Nappi, S., and De Pesquisa, Â. (2000). Ancient rendering mortars from a Brazilian palace. Cement Concr. Res. 30, 1609–1614. doi:10.1016/s0008-8846(00)00408-7
Gliozzo, E., Dalconi, M., Chiara Cruciani, G., and Turbanti Memmi, I. (2009). Application of the Rietveld method for the investigation of mortars: a case study on the archaeological site of Thamusida (Morocco). Ejm 21 (21), 457–465. doi:10.1127/0935-1221/2009/0021-1905
Elsen, J., Balen, K., and Mertens, G. (2012). Hydraulicity in historic lime mortars: a review. Historic Mortars Conference 2, 125–139. doi:10.1007/978-94-007-4635-0_10
Fiori, C., Vandini, M., Prati, S., and Chiavari, G. (2009). Vaterite in the mortars of a mosaic in the saint peter basilica, vatican (rome). J. Cult. Herit. 10, 248–257. doi:10.1016/j.culher.2008.07.011
Franzini, M., Leoni, L., and Lezzerini, M. (2000). A procedure for determining the chemical composition of binder and aggregate in ancient mortars: its application to mortars from some medieval buildings in Pisa. J. Cult. Herit. 1, 365–373. doi:10.1016/S1296-2074(00)01092-X
Gameiro, A., Santos Silva, A., Faria, P., Grilo, J., Branco, T., Veiga, R., et al. (2014). Physical and chemical assessment of lime-metakaolin mortars: influence of binder:aggregate ratio. Cement Concr. Compos. 45, 264–271. doi:10.1016/j.cemconcomp.2013.06.010
Gleize, P. J. P., Motta, E. V., Silva, D. A., and Roman, H. R. (2009). Characterization of historical mortars from santa catarina (Brazil). Cement Concr. Compos. 31, 342–346. doi:10.1016/j.cemconcomp.2009.02.013
Hormes, J., Diekamp, A., Klysubun, W., Bovenkamp, G.-L., and Börste, N. (2016). The characterization of historic mortars: a comparison between powder diffraction and synchrotron radiation based X-ray absorption and X-ray fluorescence spectroscopy. Microchem. J. 125, 190–195. doi:10.1016/j.microc.2015.11.034
Hughes, J. J., and Cuthbert, S. J. (2000). The petrography and microstructure of medieval lime mortars from the west of Scotland: implications for the formulation of repair and replacement mortars. Mater. Struct. 33, 594–600. doi:10.1007/BF02480541
Loureiro, A. M. S., Angélica, R. S., Sanjad, T. A. B. C., Oliveira, M. M. d., and Costa, M. L. d. (2015). Eflorescência salina na igreja de Santo Alexandre, Belém–PA. Ambient. constr. 15, 71–83. doi:10.1590/s1678-86212015000300027
Loureiro, A. M. S., Paz, S. P. A. d., Veiga, M. d. R., and Angélica, R. S. (2020). Investigation of historical mortars from Belém do Pará, northern Brazil. Construct. Build. Mater. 233, 117284. doi:10.1016/j.conbuildmat.2019.117284
Maravelaki-Kalaitzaki, P., Bakolas, A., and Moropoulou, A. (2003). Physico-chemical study of Cretan ancient mortars. Cement Concr. Res. 33, 651–661. doi:10.1016/S0008-8846(02)01030-X
Matias, G., Faria, P., and Torres, I. (2014). Lime mortars with heat treated clays and ceramic waste: a review. Construct. Build. Mater. 73, 125–136. doi:10.1016/j.conbuildmat.2014.09.028
Middendorf, B., Hughes, J. J., Callebaut, K., Baronio, G., and Papayianni, I. (2005). Investigative methods for the characterisation of historic mortars-Part 1: mineralogical characterisation. Mater. Struct. 38, 761–769. doi:10.1617/14281 doi:10.1007/bf02479289
Middendorf, B., Hughes, J. J., Callebaut, K., Baronio, G., and Papayianni, I. (2005). Investigative methods for the characterisation of historic mortars-Part 1: mineralogical characterisation. Mater. Struct. 38, 761–769. doi:10.1617/14281 doi:10.1007/bf02479289
Moropoulou, A., Bakolas, A., and Bisbikou, K. (1995). Characterization of ancient, byzantine and later historic mortars by thermal and X-ray diffraction techniques. Thermochim. Acta 269–270, 779–795. doi:10.1016/0040-6031(95)02571-5
Moropoulou, A., Bakolas, A., and Bisbikou, K. (2000). Investigation of the technology of historic mortars. J. Cult. Herit. 1, 45–58. doi:10.1016/S1296-2074(99)00118-1
Moropoulou, A., Cakmak, A. S., Biscontin, G., Bakolas, A., and Zendri, E. (2002). Advanced byzantine cement based composites resisting earthquake stresses: the crushed brick/lime mortars of Justinian’s Hagia Sophia. Construct. Build. Mater. 16, 543–552. doi:10.1016/S0950-0618(02)00005-3
Pacheco-Torgal, F., Faria, J., and Jalali, S. (2012). Some considerations about the use of lime-cement mortars for building conservation purposes in Portugal: a reprehensible option or a lesser evil? Construct. Build. Mater. 30, 488–494. doi:10.1016/j.conbuildmat.2011.12.003
Paz, S. P. A., Torres, P. W. T. S., Angélica, R. S., and Kahn, H. (2017). Synthesis, Rietveld refinement and DSC analysis of Al-goethites to support mineralogical quantification of gibbsitic bauxites. J. Therm. Anal. Calorim. 128, 841–854. doi:10.1007/s10973-016-5987-4
Piovesan, R., Dalconi, M. C., Maritan, L., and Mazzoli, C. (2013). X-ray powder diffraction clustering and quantitative phase analysis on historic mortars. Eur. J. Mineral. 25, 165–175. doi:10.1127/0935-1221/2013/0025-2263
Silva, A. S., Borsoi, G., Veiga, R., Fragata, A., Tavares, M., and Llera, F. (2010). Physico-chemical characterization of the plasters from the church of santissimo sacramento in alcântara. Lisbon, Portugal: RILEM Publications SARL, Vol. 6, 345–357.
Singh, M., Vinodh Kumar, S., Waghmare, S. A., and Sabale, P. D. (2016). Aragonite-vaterite-calcite: polymorphs of CaCO3 in 7th century CE lime plasters of Alampur group of temples, India. Construct. Build. Mater., 112, 386. doi:10.1016/j.conbuildmat.2016.02.191
Theodoridou, M., Ioannou, I., and Philokyprou, M. (2013). New evidence of early use of artificial pozzolanic material in mortars. J. Archaeol. Sci. 40, 3263–3269. doi:10.1016/j.jas.2013.03.027
Toby, B. H. (2006). R factors in Rietveld analysis: how good is good enough? Powder Diffr. 21, 67–70. doi:10.1154/1.2179804
Velosa, A. L., and Veiga, M. R. (2016). “Mortars of the historic heritage: knowing to allow conservation and rehabilitation,” in CINPAR–XII international conference on structural repair and rehabilitation, October 26–29, 2016 (Porto, Portugal: Faculdade de Engenharia da Universidade do Porto), 12–19.
Keywords: wet chemical Analysis, XRD, DSC, historic mortars, binder: aggregate ratio, brazilian mortars, old mortars, Rietveld Method
Citation: Loureiro AMS, Paz SPAd and Angélica RS (2020) How to Estimate the Binder: Aggregate Ratio From Lime-Based Historic Mortars for Restoration?. Front. Mater. 7:597411. doi: 10.3389/fmats.2020.597411
Received: 21 August 2020; Accepted: 11 November 2020;
Published: 11 December 2020.
Edited by:
Radu Claudiu Fierascu, National Institute for Research & Development in Chemistry and Petrochemistry (ICECHIM), RomaniaReviewed by:
Veronica Calderón, University of Burgos, SpainYunpeng Liu, Wuhan University of Technology, China
Copyright © 2020 Loureiro, Paz and Angélica. This is an open-access article distributed under the terms of the Creative Commons Attribution License (CC BY). The use, distribution or reproduction in other forums is permitted, provided the original author(s) and the copyright owner(s) are credited and that the original publication in this journal is cited, in accordance with accepted academic practice. No use, distribution or reproduction is permitted which does not comply with these terms.
*Correspondence: Alexandre Máximo Silva Loureiro, bG91cmVpcm8xQHVmcGEuYnI=