- 1Guangdong Key Laboratory of Environmental Catalysis and Health Risk Control, Guangzhou Key Laboratory Environmental Catalysis and Pollution Control, School of Environmental Science and Engineering, Institute of Environmental Health and Pollution Control, Guangdong University of Technology, Guangzhou, China
- 2School of Chemistry, University of Southampton, Southampton, United Kingdom
Volatile organic compounds (VOCs) are poisonous and regarded as the paramount source for the formation of secondary organic aerosols, ozone, and photochemical smog, greatly affecting human health and environment quality. In order to abate VOC emission, catalytic oxidation is widely applied in industries and is believed to be an efficient and economically feasible way for the elimination of VOCs. This review is primarily concentrated on summarizing the recent progress and developments in the catalytic oxidation of various VOCs over Pd-supported catalysts. Despite their high catalytic performances at much lower temperatures, the wide use of Pd-supported catalysts in the industry has been limited by their high cost, low thermal stability, and incomplete oxidation of VOCs. Hence, the intrinsic properties of the active sites and supports, the reaction conditions, the deactivation mechanism, and the strategy to reveal the catalytic oxidation pathway are also systematically summarized. This review aims to give a deep comprehension and guidance for the development of efficient and cost-effective Pd-supported catalysts in the near future.
Introduction
It is well known that organic compounds with the boiling point below 250°C and saturated vapor pressure equivalent to the atmospheric pressure (101.325 kPa) are identified as volatile organic compounds (VOCs). The types of released VOCs include aromatics, aliphatic hydrocarbons, oxygenated VOCs, halogenated VOCs, sulfur- or nitrogen-containing VOCs, and so on. Although the release of VOCs originates from the nature and human activities, the anthropogenic source accounts for a large proportion for VOC emission. Urbanization and industrialization have further led to the continuous increase in the emission of VOCs (Boeglin et al., 2006; Qiu et al., 2014). The anthropogenic sources were usually linked with chemical industries, gas station, food processing, petrochemical processing, production of plastics, solvent use, and many other industrial activities (Liotta, 2010; Scire and Liotta, 2012; Liao et al., 2015). Due to the high volatility at ambient conditions, the majority of VOCs can be easily emitted into the atmosphere and be absorbed through the human respiratory tract, skin, and digestive tract, and are harmful to the respiratory system and central nervous system even at low concentrations (Srivastava et al., 2005; Boeglin et al., 2006; Ahn et al., 2017; Odoom-Wubah et al., 2019c). Furthermore, some of the VOCs are used in the formation of secondary organic aerosols (Boltic et al., 2013; Zang et al., 2019), reducing air quality, and may trigger stratospheric ozone depletion (Dumanoglu et al., 2014; Liang et al., 2017). Therefore, efficient VOC destruction is of paramount significance from the perspectives of environment and human health.
The techniques used for the elimination of VOCs include recovery and destruction. Recovery techniques such as absorption, adsorption, and membrane separation are more adaptable to efficient disposal of high concentration VOCs. However, execution of these techniques is restricted by a complicated process and high cost (Iranpour et al., 2005; Vu et al., 2009; Tomatis et al., 2016). Catalytic oxidation appears to be an effective way for the removal of VOCs due to its higher destructive efficiency and lower operating temperatures (Zhang et al., 2016). It can completely mineralize VOCs to carbon dioxide (CO2) and H2O instead of transforming them to by-products with high toxicity. Furthermore, catalytic oxidation can also be applied to eliminate extremely dilute VOC streams.
Preparation of catalysts with high activity and stability is critically important in VOC catalytic oxidation. Catalysts for VOC combustion can be divided into the following categories: 1) non-noble metal oxides (Kim and Shim, 2010), 2) supported noble metals (Liotta, 2010), and 3) alloy metal nanoparticle catalysts (Aguero et al., 2009; Zeng et al., 2015). Among these, noble metal catalysts (Au, Pt, Rh, Pd, etc.) possess superior catalytic activity and durability for VOC catalytic oxidation under low temperature (Gulsnet and Magnoux, 1997). Currently, Pd is preferred in the industrial application of VOC abatement over the other noble metals (Au, Pt, and Ru) (Bendahou et al., 2008; Aznárez et al., 2015; Xiong et al., 2018; Odoom-Wubah et al., 2019a) on account of its unique electron configuration and high chemical stability during VOC catalytic oxidation (Kamal et al., 2016). Significant achievements have been obtained by using Pd as a catalyst for the elimination of VOCs. It has been proved that the catalytic performance of Pd-based catalysts is highly dependent on the particle size, chemical state, loading amount of Pd, and physical and chemical properties of the supporting materials. However, deactivation phenomena frequently appear in the forms of coking, poisoning, and thermal sintering during catalytic VOC oxidation over Pd-based catalysts, which cause a decrease in catalytic activity. Complete understanding of the reaction mechanism is vital for the development of highly efficient catalysts for VOC oxidation. The present work mainly focuses on previous reviews of catalytic oxidation of VOCs over Pd-supported catalysts (Scheme 1). Moreover, the intrinsic properties of catalysts and the reaction conditions for the catalytic performance over Pd catalysts are described. The deactivation mechanism and the tactics to disclose the catalytic oxidation pathway are summarized in detail too. We believe that this review is meaningful in offering a deep understanding and guidance to the preparation of highly efficient and cost-effective Pd catalysts.
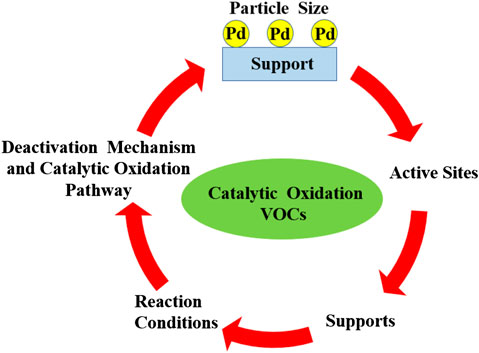
SCHEME 1. Factors influencing the catalytic oxidation of volatile organic compounds over Pd-supported catalysts.
Pd-Supported Catalysts
Generally, supported catalysts consist of two parts: the active site and the supporting material. Therefore, it is reasonable to take these two parts into consideration as factors influencing the catalytic activity of Pd-supported catalysts. As for the active sites, more attention has been paid to the Pd particle size and the chemical state of Pd. In consideration of the supporting materials, the support type, acidity, and metal–support interaction, which are intrinsic properties of the support, play a vital role in the catalytic performance of Pd-supported catalysts. Hence, we mainly introduce the influence of particle size and chemical state of Pd, as well as the intrinsic properties of the supports for the reason that most of the investigations about Pd catalysts are focused on that.
Active Sites
Influence of Pd Particle Size
The size of Pd nanoparticles (Pd NPs) is an interesting and promising factor that influences catalytic performances. It is well known that Pd NPs with high surface-to-volume ratio can greatly enhance the catalytic activity by providing additional active sites per unit area; because of this, the catalytic activity is closely correlated to Pd particle size (Kim and Shim, 2009; Bedia et al., 2010; Wang et al., 2013; Liu J. et al., 2014; Li et al., 2017). On the other hand, high-temperature reduction could decrease the surface Pd particle size by encapsulating and trapping it with the support and subsequently promoting the activation of O2, which is beneficial to the degradation of VOCs (Li et al., 2017). Lupescu et al. stated that Pd below 8.8 nm was essential for the rapid formation of PdO and efficient re-dispersion of Pd (Lupescu et al., 2016). Lowering the size of Pd NPs can increase the interface between the Pd and support, offering more available mobile oxygen (Giraudon et al., 2008a). To obtain superior catalytic activity and higher atom-utilization efficiency, many investigations are concerned with the preparation of catalysts with smaller size of Pd NPs. Single-atom catalysts exhibit great potential in generating metal centers as atomically dispersed sites for achieving high activity and selectivity (Yang et al., 2012). Besides, due to the ultra-small size (less than 2 nm), these metal nanoclusters exhibit unique and even unexpected properties that are not observed in bulk metal and conventional metal nanoparticles (Yang et al., 2012; Flytzani-Stephanopoulos, 2017; Yuan et al., 2020). Dai et al. revealed that single-atom platinum supported on ordered mesoporous iron oxide showed higher catalytic activity and better water resistance than its nanoparticulated counterpart, achieving a benzene conversion of 90% at 198°C (Yang K. et al., 2019). Wang and coworkers also indicated that nanoceria-supported atomic Pt catalysts exhibited superior catalytic performance for the oxidation of methane (Xie et al., 2018). However, there are no reports based on the utilization of single-atom Pd catalysts in the catalytic oxidation of VOCs, which should be paid more attention to in future studies.
Influence of the Pd Chemical State
The chemical state of Pd is one of the important factors affecting Pd catalytic activity, but the effects of Pd state on the catalytic activity remain controversial (Table 1). Numerous studies have demonstrated that the catalytic activity of Pd-supported catalysts is closely related to the oxidation state of Pd (Li et al., 2011; Gil et al., 2015; Zhao et al., 2016; Weng et al., 2019; Zhao X. et al., 2019). Some authors proposed that metallic Pd was more active in VOC oxidation than its oxidized state (Tidahy et al., 2008; Huang and Leung, 2011; Wang et al., 2018a; Zhao X. et al., 2019), as it can provide more active sites for the oxidation of hydrocarbons (Jinjun et al., 2005). The superior catalytic performance of Pd/TiO2 for HCHO oxidation relied on the metallic Pd NP with a strong capacity for oxygen activation (Huang and Leung, 2011). The Pd0 species were also found to be more active than PdO for o-xylene oxidation reaction (Wang et al., 2018a). Jeong et al. reported that hydrogen-treated catalysts showed higher oxidation activities than air-treated catalysts for possessing more metallic Pd (Ihm et al., 2004). However, a series of studies indicate that both Pd0 and PdO act as active sites for oxidation reaction (He et al., 2009; He et al., 2010a; He et al., 2012a; He et al., 2012c). In the presence of O2, a portion of metallic Pd was oxidized to Pd2+ and subsequently reduced by HCs. Therefore, the mixture of metallic Pd and Pd2+ was responsible for the oxidation reaction of benzene, toluene, and ethyl acetate over Pd/SBA-15 catalyst (He et al., 2012c). Navarrete et al. stated that the existence of cerium oxide in Pd/γ-Al2O3–Ce permitted the coexistence of Pd0 and PdO species, which favored the catalytic combustion of benzene and inhibited the deposition of carbonaceous species on the Pd surface (Padilla et al., 2008).
Supporting Materials
The catalytic oxidation of VOCs over Pd-supported catalysts strongly depends on the physical and chemical properties of the support, such as the support type, the acid–base properties, and the metal–support interaction. The supporting materials can profoundly affect the catalytic performance of Pd catalysts by tuning the nanoparticle size, dispersion, and chemical state. It is generally accepted that active phase dispersion is a decisive factor in the determination of catalytic performances for noble-metal–loaded catalysts. In general, support materials with larger specific surface areas permit higher dispersion of the active phase and assist in the easy adsorption of substrate to display enhanced activity, particularly in oxidation reactions. The acid properties of supports were also found to play an important role in affecting the dispersion and oxidation state of Pd NPs, thus influencing the catalytic performance for VOC catalytic oxidation. The existence of a strong interaction between the support and active phase also exerts a constructive effect on increasing the catalytic activity, selectivity, and stability. The development of an active and stable catalyst is one of the most crucial factors in the VOC oxidation process. The traditional supports can no longer satisfy the aforementioned aspects for VOC oxidation because of their weak thermostability and lower specific surface area. As a consequence, several researchers take great interest in the modification of the supporting materials to improve the catalytic performance.
Influence of the Support Type
For Pd-based catalysts, the support effect is profound, and their activity has also been found to be closely related to the type of support materials. Different types of supports have been comprehensively investigated for hydrocarbon catalytic oxidation (Table 2). Giraudon et al. reported that superior catalytic performance of Pd/TiX was observed than of Pd/ZrX for chlorobenzene oxidation. This was attributed to the better reducibility of the TiO2 support (Ti4+ into Ti3+), allowing for enhanced oxygen mobility (Giraudon et al., 2008b). Moulijn stated that the catalytic activity of Pd supported on carbon-based monoliths for combustion of m-xylene was correlated with the surface area of the support, and the mesoporous sample possessed the best catalytic performance compared with the monolithic support and microporous sample (Pérez-Cadenas et al., 2008). The same conclusion was evidenced by He and coworkers, in which they revealed that the excellent catalytic performance of Pd/Co3O4 might be due to the mesoporous structure of the support by exposing more active species (Wang et al., 2015). The supports with mesopores (such as SBA-15 and MCM-48) possessing narrow pore size distribution, high surface area, are considered as one of the best supports for the preparation of Pd catalysts (Shi et al., 2013). On the other hand, metal–organic frameworks are also frequently used in adsorption (Zhang et al., 2019b) and photocatalysis (Zhang et al., 2018). Pd NPs supported on UiO-66 displayed narrow size dispersion and achieved superior catalytic performances for toluene oxidation (Bi et al., 2020). Qi et al. proposed that the size of support also had an impact on the Pd dispersion and influenced the catalytic performance of benzene oxidation due to the number of adsorbed sites and interface effect (Zhao et al., 2011). They studied and compared the synthesis of Pd NPs on nano-sized and micro-sized molecular sieves and concluded on the superior catalytic performance of nano-sized molecular sieves because of their higher external surface area and shorter channel length.
Influence of Acidity of the Supports
It was reported that the acidity of supports significantly affects the catalytic performance of Pd-supported catalysts in VOC oxidation (He et al., 2010a; He et al., 2010d; Venezia et al., 2011; Wang et al., 2018b). Yazawa et al. investigated the catalytic combustion of propane over palladium supported on various carriers (MgO, ZrO2, Al2O3, SiO2, SiO2-ZrO2, SiO2-Al2O3, and SO42--ZrO2), finding that the supports with moderate acidity showed the highest catalytic conversion of VOCs (Yazawa et al., 1999). Wang et al. indicated that a catalyst with a low concentration of strong acid sites and higher redox ability was beneficial for butyl acetate oxidation (Yue et al., 2014). The supported Pd NPs with Brønsted acid sites had been reported to invoke catalytic enhancement by promoting adsorption and activation of reactant molecules during the catalytic process (Zang et al., 2019). The ease of oxidation of Pd NPs on acidic supports in contrast to the neutral or basic supports was justified because of their electrophilic nature forming electron-deficient Pd atoms (Okumura et al., 2003b; Hong et al., 2007; He et al., 2012a). Furthermore, the oxidation state of Pd NPs was dependent on the acid–base characteristics of the support, and the acidic supports are favorable for oxidation of Pd particles (He et al., 2012d). The Al in the support could offer acid sites, which were favorable for the oxidation of Pd NPs, thus improving the catalytic oxidation of toluene (He et al., 2012b). Based on the strength of acidity of support materials, the accelerated transformation of Pd0 to Pd2+ can promote the catalytic oxidation of VOCs (He et al., 2010a). Furthermore, the acidity of the supports is considered to be crucial for the catalysts with high Pd dispersion and found to be beneficial in promoting the catalytic reaction (Tidahy et al., 2006; He et al., 2010c; Jin et al., 2011; Yang et al., 2016; Dai et al., 2018). Okumura et al. reported the support acid property had a significant influence on the dispersion of Pd and the Brønsted acid sites were responsible for the generation of highly dispersed Pd species (Okumura et al., 2003a).
Influence of Metal–Support Interaction
Metal–support interactions have been observed on various supported Pd catalysts for catalytic oxidation of VOCs (Tauster et al., 1978; Ryndin et al., 1981). Rooke et al. investigated the catalytic performance over Pd NP catalysts on various supports. The Pd impregnated on hierarchically porous Nb2O5 or Ta2O supports displayed enhanced catalytic oxidation activity of toluene compared with hierarchically porous TiO2 and ZrO2, which was dependent on the metal–support interaction (Rooke et al., 2012). Hao et al. also found that the strong synergistic effect between Co3O4 and PdO was the main factor determining the catalytic activity but not the amount of the surface palladium species for the catalytic oxidation of toluene (Li et al., 2011). Due to the strong metal–support interaction, enhanced dispersion, and hydrothermal stability, Pd has been employed in various oxidation catalytic reactions (Kim et al., 2016). The interaction between the metal and support strongly affected the state of Pd NPs for VOC catalytic oxidation. The oxidation of Pd into PdO was promoted by the reducible oxides, whereas the non-reducible oxides boosted the reduction of Pd (Bernal et al., 2005; Ramírez-López et al., 2010). Pd NPs supported on ZrO2 were more prone to generate a strong metal–support interaction with Brønsted acid sites rather than the interfacial structure, accelerating toluene oxidation and improving the catalytic efficiency (Yang et al., 2020). The high activity for benzene destruction on calcified shrimp waste–supported Pd NPs was dependent on high Pd metal dispersion, and the synergistic effect between the small Pd NPs and the shrimp waste support (Odoom-Wubah et al., 2019b).
Influence of the Modification of the Support
Garcia et al. stated that the activity of titania-supported Pd catalysts was greatly enhanced by the modification with vanadium for the oxidation of short-chain alkanes, which was attributed to the improved redox properties of the catalysts (Garcia et al., 2005). Barakat et al. verified that doping with foreign ions into the skeleton of the support and noble metal loading offered a stable activity of the catalysts toward the catalytic destruction of toluene at low temperatures (Barakat et al., 2014a). A synergistic effect was observed for the catalytic oxidation of benzene when vanadium was added to Pd/TiO2 catalysts (Garcia et al., 2006). Another study by Li and coworkers reported that doping of Al ions into the skeleton of TiO2 inhibited the electron transfer from TiO2 to Pd by enhancing anatase stabilization and increasing defects in TiO2. The optimal catalytic performance for ethanol conversion and CO2 production over the Pd-supported Al-doped TiO2 was attributed to the enhanced structural properties of Al-doped TiO2 (Zhu et al., 2017). With the addition of Ce to Al2O3, the catalytic activity for VOC oxidation was higher than that of pure Al2O3 for Pd-based catalysts (Pitkäaho et al., 2013; Gil et al., 2015; Liao et al., 2016; Hosseini et al., 2017). Zuo et al. emphasized the role of ceria added to Pd/SP (shell powder) for increasing the surface area and total pore volume and decreasing the sintering ability of SP (Zuo et al., 2013). Chen et al. concluded that the physical/chemical adsorption of Pd/MCM-22 was greatly improved with the addition of Y2O3, which was beneficial to the oxidation reaction (Chen et al., 2019b). He et al. demonstrated that the catalytic oxidation of benzene over Pd-supported ZSM-5/MCM-48 was much higher than that on individual ZSM-5 and MCM-48 support materials (He et al., 2010a).
Alloyed Nanoparticle Catalysts
Although catalysts with a single noble metal possess excellent catalytic activities based on the results reported in the documentation, alloyed NP (bimetallic NPs and trimetallic NPs) catalysts create exceptional physicochemical properties that are distinctly different from those of their monometallic counterparts (Yu et al., 2015; Wei et al., 2020). Moreover, the combination of properties correlated to distinct metals may have a synergetic effect on the catalytic competence of the catalysts (Table 3). Alloyed catalysts exhibit unique and more flexible surface structures than monometallic catalysts, in which these flexible surface structures are beneficial to tailoring the affinity of surface adsorbates to substrates, giving rise to high catalytic activity (Chen and Rodionov, 2016).
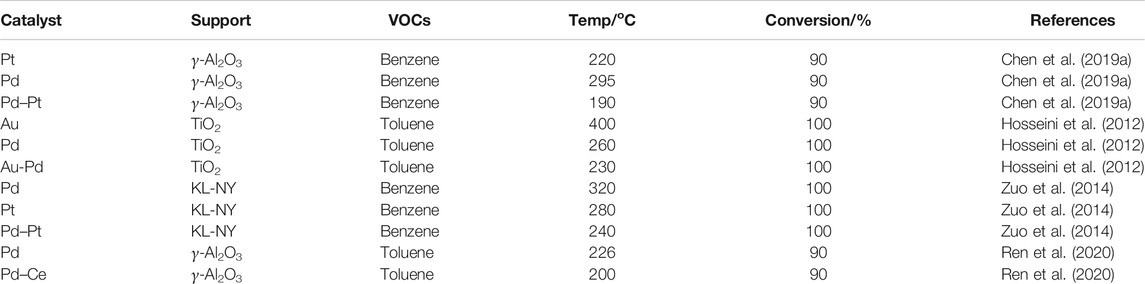
TABLE 3. Reported alloyed nanoparticles catalysts for volatile organic compounds (VOCs) catalytic oxidation.
Bimetallic NP catalysts are extensively applied for the catalytic oxidation of VOCs due to their superior catalytic performance attributed to the extraordinary structure and the synergistic effect between the bimetallic NPs. It has been reported that Au-Pd/meso-Cr2O3 catalysts showed an extraordinary catalytic performance for toluene oxidation because of the strong interaction between Au–Pd NPs and Cr2O3 as compared to the single-metal catalysts (Wu et al., 2016). Chen et al. pointed out that the superior catalytic performance of Pd–Pt NP catalysts in benzene oxidation was primarily due to the nano-effects of Pd–Pt and the interactions among the active substances (Chen et al., 2019a). The catalytic combustion of toluene and propene on Pd (shell)–Au (core)/TiO2 displayed the highest catalytic activity, correlating to the Pd-shell and Au-core morphology (Hosseini et al., 2012). Hutchings et al. investigated the oxidation of toluene over the Au–Pd catalysts and demonstrated that the Au acted as an electronic promoter for Pd (Enache et al., 2006; bin Saiman et al., 2012). The complete conversion of benzene could be achieved at approximately 230°C owing to the enhancement of the oxidation properties over Pd catalysts with the addition of Ce and Pt (Zuo et al., 2014). Researchers have attempted to improve low-temperature activity and stability of Pd-supported catalysts through introducing non-noble metals to the catalysts, concerning the high cost and the rare supply of the noble metals. Ultrafine dispersed Pd–Cu nanoalloys anchored on porous carbon showed high catalytic activity, durability, and tolerance toward ethanol oxidation due to their advanced structure and composition (Yang W. et al., 2019). Pd0.01Mn0.2/Ti catalyst exhibited superior catalytic performance for acetone oxidation than its counterpart catalyst in the absence of Mn due to the presence of high valence states of Mn in the catalyst (Zhao Q. et al., 2019). The bimetallic Pd–Ce/γ-Al2O3 catalyst displayed better catalytic performance than Pd/γ-Al2O3, further indicating that Ce loading improved the catalytic activity by reducing the amount of precious metals incorporated within the catalyst (Ren et al., 2020). The high water-resistant ability of the supported Pd−W bimetallic catalysts was ascribed to the presence of the facile redox cycle of the active Pd2+/Pd0 couple (Liang et al., 2019). The addition of Mo in Pd/Al2O3 catalyst efficiently improved the activity and stability by increasing the Pd dispersion and changing the partial PdO (He et al., 2014). With the introduction of NiO, the activity of Pd/SBA-15 was greatly improved on account of the strong synergistic effect of PdO–NiO (Tang et al., 2017). The synergistic relationship between Pd and W accounted for the high activity in the oxidation of propane. Besides, the presence of W led to the epitaxial orientation of Pd NPs (Taylor et al., 2012).
Influence of Reaction Conditions
As for the Pd-supported catalysts, not only the intrinsic properties of the catalysts but also the reaction conditions as well as the composition of the reactant VOCs greatly influenced the oxidation behaviors of VOCs.
Influence of Humidity
The effect of humidity on the catalytic performance for VOC oxidation over Pd catalysts has been extensively studied as H2O plays a vital role in the catalytic oxidation process. Water often exists in the flue gases released from the industries or as the products of VOC catalytic oxidation.
It is difficult to determine the role of water in VOC catalytic destruction, for the reason that the catalyst component, VOC type, and reaction conditions may exert a measure of influence over it (He et al., 2019). In general, water vapor has been observed to play an inhibiting role in VOC oxidation (Liotta, 2010); the inhibitory role of H2O adsorption under low temperature was reversible, which resulted in the decrease in the catalytic activity. A previous work reported by Madcot et al. proposed that the addition of relatively large quantities of water inhibited the catalytic activity for the oxidation of propane and propene over Pd/Al2O3 catalysts due to the decrease in the active surface (Marécot et al., 1994). Similar results were also reported by Arias and coworkers, suggesting that the high concentration water in the feed favored the catalyst deactivation on account of the competitive adsorption and oxidation of VOCs and water molecules on the active site (de la Peña O’Shea et al., 2005). The adsorption behavior of VOCs on the catalyst surface was correlated to the polarity of the VOC molecules (Xia et al., 2018). In consideration of the hydrophobicity of the n-propane thiol and n-chloropropane, the inhibition effect was observed in the adsorption of VOCs for the blocking effect of the water layer on the catalyst surface (He et al., 2020). Meanwhile, the presence of water was conducive to the adsorption of polar VOCs but caused an inhibition to the desorption of VOCs, hence restraining their degradation. Upon increasing the temperature, the existence of water molecules can lead to the formation of hydroperoxyl intermediate species and hence favor the activation of oxygen in VOC oxidation (Chang et al., 2016). Wang et al. indicated that the introduction of water vapor facilitated the toluene adsorption over Pd-UiO-66-EG and hindered the desorption of toluene (Bi et al., 2020). The inhibitory effect of the existence of water vapor for toluene oxidation disappeared at higher temperature conditions. Although water presumably exists as an inhibitor, in some cases, it may play a positive role in VOC destruction. Gonzalez-Marcos and coworkers found that the presence of water could promote deep oxidation of trichloroethylene to CO2 and thus reduce selectivity of CO over Pd/Al2O3 catalysts. Upon increasing the concentration of water, the promotion of CO complete oxidation by the water–gas shift reaction was observed along with the reduced selectivity for the formation of C2Cl4 and Cl2 (Gonzalez-Velasco et al., 2000). In-depth research focusing on the role of water for VOC oxidation should be investigated for detailed understanding.
Influence of Reactant Composition
Industrial flue gas steams contain various compositions and concentrations of VOCs with different physicochemical properties. Thus, the catalytic removal process of mixtures of VOCs is more complex than that of single-component VOCs. An inhibitory (Santos et al., 2010a; Santos et al., 2010b) or promoting effect (Barakat et al., 2014b) might be observed on the catalytic oxidation of mixed VOCs. However, some research groups have reported the concurrent removal of mixed VOCs. The presence of other VOCs always occupies some active sites over the support through chemisorption, leading to the decrease in the concentration of rate-determinant species. For the catalytic oxidation of VOC mixtures (benzene, toluene, and ethyl acetate) over Pd/SBA-15, Hao et al. reported that the presence of benzene or toluene inhibited the oxidation process of ethyl acetate, and the catalytic oxidation of benzene and toluene restricted each other, while the promotion effect was observed for ethyl acetate on toluene (He et al., 2012c). Dai et al. pointed out that because of the existence of the competitive adsorption of the mixture of the VOCs over the Au–Pd/α-MnO2, a higher temperature was needed for the complete oxidation of these VOCs. The exothermic character of the complete oxidation reaction exerted a promoting effect on VOC oxidation (Xia et al., 2018). However, Barakat et al. stated that Pd/5%V-TiO2 possessed the superior activity for the oxidation of a butanone–toluene mixture than that of single-component butanone (Barakat et al., 2014b). It was because in the presence of toluene, butanone showed a higher affinity to the surface of the catalyst, thus ensuring a faster oxidation process than when alone in the gaseous stream. The actual reasons for the inhibitory and promoting effects in the oxidation of VOC mixtures over the Pd catalysts need further study in the future work.
Deactivation of Pd-Supported Catalysts
Catalyst deactivation is a great concern for the catalysts in the industrial catalytic processes because it is relevant to practical application in light of the cost for regeneration or restoration of their activity. It can take place in the following forms: coke (carbonaceous deposits), chlorine, nitrogen, sulfur poisoning, and thermal sintering.
Formation of Coke
The catalyst deactivation caused by coke is a major problem in the petrochemical industry as it causes a gradual decline in catalytic activity. Generally, the formation of coke occurs in the pores and on the external surface of catalysts (Gulsnet and Magnoux, 1997; Guisnet et al., 2009) via undesired side reactions (Becker and Förster, 1997) during the catalytic oxidation process. It is generally accepted that coke formation includes hydrogen transfer (acid catalysts), dehydrogenation of adsorbed hydrocarbons (bifunctional catalysts), condensation, and rearrangement steps. Besides, retention of the “coke” molecules on the catalysts is mainly ascribed to their strong adsorption and low volatility (gas-phase reactions) or low solubility (liquid-phase reactions) (Guisnet and Magnoux, 2001). The produced carbonaceous intermediates will prevent the reaction VOC oxidation and retard the deep catalytic oxidation process. In principle, coke may affect catalyst activity in two ways: through active site coverage (poisoning) and pore blockages (active sites rendered inaccessible to reactants) (Guisnet and Magnoux, 1994). Yang et al. revealed that carbon was easily deposited on the catalyst surface and significantly restricted the interaction between toluene and the surface active sites, resulting in the catalyst deactivation (Yang, 2020). Similar results were also suggested by Jeong et al. for n-hexane destruction over the Pd/γ-Al2O3 catalysts. The initial activity of reduced catalysts was higher at lower temperatures than the oxidized catalysts which displayed a gradual deactivation under the existence of carbonaceous intermediate species (Ihm et al., 2004). Coke is prone to form on the catalysts with large amounts of acid sites that participate in coke production as a side reaction, thus resulting in catalyst deactivation (He et al., 2009; He et al., 2012d). Dégé et al. claimed that the lower the number of the acid sites, the slower the coke formation and the faster the o-xylene oxidation on the Pd/HFAU catalysts (Dege et al., 2000). Although higher acidity can accelerate the catalytic reaction and bring about higher activity, Pd/beta displayed the highest activity for VOC removal compared with Pd/ZSM-5, Pd/SBA-15, Pd/MCM-48, and Pd/MCM-41, but showed poor stability for its higher acidity, causing the formation of coke (He et al., 2009). The mesoporous SiO2-supported Pt–Pd catalyst exhibited high catalytic activity, and no coke was formed during the catalytic combustion of toluene (Wang H. et al., 2017). In most instances, the catalyst can be regenerated from the coke deactivation by oxidizing under air or treating the catalyst at much high temperature conditions. Hence, more endeavors should be devoted to prepare catalysts with anti-coking ability and finding the equilibrium point between high activity and coke formation over Pd-supported catalysts based on the acidity of the supports.
Chlorine, Nitrogen, and Sulfur Poisoning
A promising and superior candidate for industrial applications should have high catalytic performance and outstanding stability. However, sometimes the catalysts may suffer from deactivation, especially with heteroatom-containing VOCs. Catalyst poisoning occurs in a way by disabling the active sites of the catalysts. The mineralization rate of the VOCs with heteroatoms was lower than that of VOCs without heteroatoms, as the heteroatom-containing functional groups decelerated the mineralization process (He et al., 2020). For example, the presence of chlorine in the catalysts caused deactivation in catalytic oxidation of chlorinated VOCs. The inhibitory effects of chorine were caused by the partial blockage of metal active sites, thus decreasing their ability for the chemisorption of reactants (Kondarides and Verykios, 1998). On the other hand, the formation of less active oxychlorinated species (MOxCly) takes place (Paulis et al., 2001). Aranzabal et al. reported that the increasing amounts of environmentally undesirable chlorine at temperatures below 400°C were produced over Pd/alumina catalyst for dichloroethane gas-phase oxidation due to its activity in Deacon reaction (Aranzabal et al., 2006). Pd catalysts usually display superior activity for the catalytic oxidation of hydrocarbons (Xiong et al., 2018); however, it also suffers from severe deactivation, which can be attributed to the formation of polychlorinated [PhClx (x = 2–6)] by-products through the chlorination of the organic skeleton (van den Brink et al., 2000). Leclercq et al. claimed that the pre-reduced Pd/LaBO3 was more active than the perovskite alone for chlorobenzene (PhCl) transformation but substantially increased the chlorination rate of PhCl (Giraudon et al., 2008a). Cao and his coworkers found that the decrease in the selectivity of the Pd/TiO2 catalyst was observed in the long-term stability test for catalytic combustion of dichloromethane, which was related to the deposition of carbon species and chlorine poisoning, thereby blocking the active sites for dichloromethane decomposition. Furthermore, their results indicated that the adsorption of chlorine species could change the chemical states of Pd and produced inactive species (Cao et al., 2018). However, alloying of gold with Pd supported on the three-dimensionally ordered macroporous CeO2 improved the catalytic stability and chlorine tolerance in trichloroethylene combustion (Zhang et al., 2019a). Nitrogen-containing VOCs caused the deactivation of catalysts and decreased the catalytic performance by the formation of toxic intermediates (HCN, NO, N2O, NH3, CO, etc.) and sulfur-containing VOCs deactivated the catalyst by covering the active sites on the surface. Pd catalysts were unsuitable for nitrile oxidation on account of undesirable generation of NOx (Krocher and Elsener, 2009). Pd/SBA-15 displayed a higher conversion of acetonitrile along with the production of harmful by-products (Zhang et al., 2014). The low porosity of Pd/S-2GA (grafting) resulted in higher NOx yield for n-butylamine combustion, as high porosity can promote the diffusion of NHx and decrease the contact between NHx and Pd active sites, thereby decreasing the yield of NOx (Ma et al., 2020). The presence of OH radicals promotes the conversion of NH3/NH4+ to NO3− through the strong oxidizing ability of the OH radicals (Kim and Choi, 2002). Yu et al. stated that the poisoning effect of sulfur was attributed to the formation of aluminum sulfate on Pd/Al2O3 over 473 K (Yu and Shaw, 1998).
Influence of Thermal Sintering
Although noble metals including Pd are highly active in the catalytic oxidation of VOCs, they can also be deactivated by thermal sintering, which leads to the loss of catalytic surface area and alters the physicochemical properties of catalysts influencing the thermal stability under oxidative conditions (Krocher and Elsener, 2009; Wang N. F. et al., 2017). The catalyst can also be deactivated by a decrease in the number of active sites or change in the active site distribution ascribed to catalyst structural variations induced by thermal sintering (Bartholomew, 2001). Furthermore, the thermal sintering of the active phase is generally irreversible. Thermal sintering of the Pd atoms, clusters, and NPs caused catalyst deactivation during prolonged operation via Ostwald ripening (Xu et al., 2011; Spezzati et al., 2017). Monolayer films of Pd@CeO2 core–shell nanocomposites exhibited excellent thermal stability and strong resistance to sintering in comparison with the bare Pd NPs (Adijanto et al., 2013). Confining noble metal nanoparticles or metal oxide in zeolite crystals prevents the agglomeration of active phases due to the size confinement of zeolite (Wu et al., 2019). The introduction of mesopores into zeolites can avoid the formation of coke and active phase sintering, and promote the mass transfer. Transition metal oxide functionalized mesoporous ZSM-5 single crystals with b-axis-aligned mesopores (M-ZSM-5-Oms) act as efficient and stable support for dispersing PdO. The Pd/M-ZSM-5-Oms displayed extraordinary catalytic performance and thermal stability for the degradation of benzene under low-temperature reaction conditions (Liu F. J. et al., 2014).
Reaction Mechanisms
Catalytic oxidation is a dynamic and complex in situ surface reaction process. The research studies about the surface oxidation mechanisms of VOCs over Pd-supported catalysts play an important role to elucidate the total catalytic process and the relationship between the catalytic performance and the catalyst structure.
Kinetic Models
Many research groups have explored the reaction mechanism of Pd-based catalysts to oxidize VOCs. In general, three degradation mechanisms were proposed to describe the catalytic combustion of VOCs over Pd-supported catalysts: Mars–van Krevelen (MVK) model (two-stage redox model), Langmuir–Hinshelwood (L-H) model, and the Eley–Rideal (E-R) model (Table 4). The MVK model is based on the assumption of a constant oxygen surface concentration on the catalyst, with reaction occurring through the interaction between reactant molecules and an oxidized portion of the catalyst (Tidahy et al., 2006). The L-H mechanism emphasizes the surface reaction between the adsorbed molecules on analogous active sites, and the surface reaction between the adsorbed reactant and molecules from the gas phase was included in the E-R mechanism (Kamal et al., 2016). Among them, the MVK kinetic model is usually adapted to elucidate the VOC oxidation process over Pd-supported catalysts (He et al., 2010a; He et al., 2010b; Jablonska et al., 2015; Liang et al., 2019). However, unlike the conventional Pd-based catalysts in which the MVK mechanism governs the oxidation of VOCs, the catalytic oxidation of toluene over Pd supported on mesoporous activated carbons followed the L-H mechanism (Bedia et al., 2010). The catalytic oxidation of gaseous trichloroethylene on Pd/alumina catalysts was explained by the E-R–type mechanism (Aranzabal, 2003). Therefore, it can be concluded that the validity of each mechanism is determined by the catalyst composition and the VOC composition.
Mechanism Studies of Volatile Organic Compound Oxidation
The reaction mechanism differs under the variations of the reaction circumstances, such as the catalyst elemental composition, physicochemical properties, the pollutant composition, and reaction conditions. Generally, the degradation process of VOCs may contain several steps of surface reactions, following the transformation of long carbon chain to short carbon chain species (CO2 as the final product). The investigation of the reaction mechanism or the oxidation pathway over the catalyst was studied by analyzing the gaseous intermediates and the product accumulated on the catalyst surface on the basis of a series of characterization techniques. Gas chromatography–mass spectrometer is the most widely used analytical instrument for the identification of intermediates based on the discrepancy of the charge–mass ratio (Bi et al., 2020). Proton transfer reaction–mass spectrometry based on the proton affinity of the VOCs can be also applied to investigate the reaction process of VOC oxidation (Zhong et al., 2020). Furthermore, IR spectroscopy, as a common characterization technology, is frequently utilized in detecting the intermediates formed in the oxidation process, such as in situ diffuse reflectance Fourier transform infrared spectroscopy (in situ DRIFT) and Fourier transform infrared spectroscopy (FT-IR) (Ryczkowski, 2001; Yang K. et al., 2019). Based on the changes in vibrational frequency and intensity of the probe, the properties of the active centers can be deduced. Other characterization techniques (H2-TPR, VOC-TPD, XPS, etc.) have also been utilized to elucidate the reaction mechanism.
A series of investigations have been carried out for a deep understanding of the oxidation pathway or reaction mechanism of some typical VOC oxidation. Dong et al. reported the catalytic oxidation mechanism of propane over hierarchical silicalite-1 zeolite enveloping Pd–CeO2 nanowires by analyzing intermediate species through in situ DRIFTS during the catalytic reaction process (Dong et al., 2020). It was proposed that the overall catalytic oxidation reaction included three steps: 1) adsorption of propane over the S-1 shell and reaction with lattice oxygen from cerium oxide to form enolate or acetone species; 2) decomposition of enolate or acetone species and subsequent formation of bicarbonate and formate species by lattice oxygen; and 3) further oxidation of bicarbonate and formate species into H2O and CO2, which underwent refilling of the lattice of cerium oxide by the gaseous oxygen. The benzene oxidation pathway over TiO2/PdW-S was proposed by Liang et al. (Liang et al., 2019) (Figure 1). It was proposed that benzene was initially oxidized by the surface oxygen to form phenolate species, followed by the transformation of phenolate species into the benzoquinone (e.g., o-benzoquinone and p-benzoquinone) species. At the same time, the carboxylate (e.g., acetate and maleate) species were also formed by the dissociation of bezene ring. Finally, the carboxylate species went through further oxidation into H2O and CO2 followed by the recovering of the catalyst. He et al. reported that the MVK kinetic model was valid for toluene oxidation and the oxidation step was decisive for the oxidation activity of Pd-supported catalysts (He et al., 2012b). The transformation of toluene to CO2 and H2O occurred over the oxidized Pd catalyst, and the reduced Pd catalyst was recovered by the adsorption and dissociation of O2. Ning and coworkers investigated the catalytic performance of noble metal (Pd, Pt, Ru, and Rh) catalysts for chlorobenzene catalytic destruction. The reaction mechanism was proposed based on the result of the in situ FTIR analysis (Liu et al., 2019). The oxidation reaction started with the adsorption of chlorobenzene on the catalyst surface. Due to the lower bond energy of C–Cl than C–H, the breakage of C–Cl and the formation of surface phenolate species occurred. The o-benzoquinone or p-benzoquinone species were produced with the further oxidation of phenolate species. Following this, benzene ring was destructed to small-molecule intermediates (maleate, maleic, or anhydride) under nucleophilic oxygen (e.g., O22−, O−, or O2−) attack. Subsequently, the produced intermediates were further oxidized to COx, H2O, HCl, and Cl2. It is well known that the characterization techniques used until now are conducive to get a better comprehension of the reaction mechanism; however, more studies need to be carried out to reflect the whole catalytic process more intuitively.
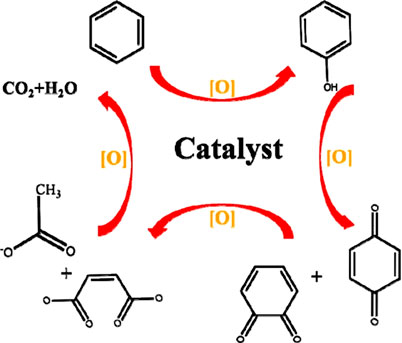
FIGURE 1. Proposed mechanism of benzene oxidation over the TiO2/PdW-S catalyst. Reprinted with permission from ref 151. Copyright (2019) American Chemical Society.
Conclusions
Facing the increasingly urgent demand for the abatement of VOCs, catalytic oxidation has become a promising technique for its high efficiency and economic feasibility, compared with other technologies for VOC removal. Generally, supported noble metal catalysts possess superior activity than non-noble metal oxides and mixed metal catalysts for VOC catalytic oxidation. Pd-supported catalysts are most extensively investigated for the catalytic oxidation of VOCs owing to their excellent catalytic performance. Although Pd-based catalysts usually displayed high activity, they still suffer from several disadvantages, such as lower activity due to the presence of water and multicomponent VOCs, the formation of coke, and low resistance to heteroatom poisoning (such as Cl, S, and N).
Therefore, more attention should be paid to extend the application of Pd-supported catalysts with high activity and stability. Future efforts should be devoted to the following aspects:
(1) With the aim to find the equilibrium point between activity and the formation of coke on the Pd-supported catalysts, the promoting effect of the support’s acidity on the catalytic oxidation of VOCs should be investigated in depth.
(2) It is well known that VOC mixtures are always produced along with industrial emissions. Therefore, the mutual effect on the catalytic oxidation of mixed VOCs should be seriously taken into consideration, which can give insights for developing low-cost and efficient catalysts for industrial applications.
(3) It was demonstrated that the heteroatom-containing (such as S, F, N, Cl, and Br) VOCs are highly toxic to the Pd catalyst due to the formation of strong Pd–heteroatom chemical bond. Therefore, catalysts with high stability and strong resistance to heteroatom poisoning are highly desirable.
(4) New characterization techniques and the combination of existing characterization techniques to study catalyst poisoning and to reveal migration and transformation of intermediate species during the VOC degradation process should be explored.
(5) Efficient degradation of VOCs with low-cost and high efficiency is still a great challenge. Attention should also be paid to the preparation of single-atom catalysts, as single-atom catalysts can obtain 100% atomic utilization, greatly reducing the cost of catalysts.
Author Contributions
SN wrote and formatted this review. SZ and XZ communicated and revised this manuscript. PV revised the manuscript. MW supervised and revised the manuscript.
Funding
This study was financially supported by the National Natural Science Foundation of China (21707020) and the Natural Science Foundation of Guangdong Province, China (2019A1515011469).
Conflict of Interest
The authors declare that the research was conducted in the absence of any commercial or financial relationships that could be construed as a potential conflict of interest.
Acknowledgments
PV would like to acknowledge The Royal Society-Newton International Fellowship for her postdoctoral research funding (NIF\R1\180185) at the University of Southampton.
References
Adijanto, L., Bennett, D. A., Chen, C., Yu, A. S., Cargnello, M., Fornasiero, P., et al. (2013). Exceptional thermal stability of Pd@CeO2 core-shell catalyst nanostructures grafted onto an oxide surface. Nano Lett. 13, 2252–2257. doi:10.1021/nl4008216
Aguero, F. N., Barbero, B. P., Gambaro, L., and Cadús, L. E. (2009). Catalytic combustion of volatile organic compounds in binary mixtures over MnOx/Al2O3 catalyst. Appl. Catal. B Environ. 91 (1–2), 108–112. doi:10.1016/j.apcatb.2009.05.012
Ahn, C. W., You, Y.-W., Heo, I., Hong, J. S., Jeon, J.-K., Ko, Y.-D., et al. (2017). Catalytic combustion of volatile organic compound over spherical-shaped copper–manganese oxide. J. Ind. Eng. Chem. 47, 439–445. doi:10.1016/j.jiec.2016.12.018
Aranzabal, A. (2003). The reaction pathway and kinetic mechanism of the catalytic oxidation of gaseous lean TCE on Pd/alumina catalysts. J. Catal. 214 (1), 130–135. doi:10.1016/S0021-9517(02)00091-X
Aranzabal, A., González-Marcos, J. A., Ayastuy, J. L., and González-Velasco, J. R. (2006). Kinetics of Pd/alumina catalysed 1,2-dichloroethane gas-phase oxidation. Chem. Eng. Sci. 61 (11), 3564–3576. doi:10.1016/j.ces.2005.12.031
Aznárez, A., Gil, A., and Korili, S. A. (2015). Performance of palladium and platinum supported on alumina pillared clays in the catalytic combustion of propene. RSC Adv. 5 (100), 82296–82309. doi:10.1039/C5RA15675K
Barakat, T., Idakiev, V., Cousin, R., Shao, G.-S., Yuan, Z.-Y., Tabakova, T., et al. (2014a). Total oxidation of toluene over noble metal based Ce, Fe and Ni doped titanium oxides. Appl. Catal. B Environ. 146, 138–146. doi:10.1016/j.apcatb.2013.05.064
Barakat, T., Rooke, J. C., Cousin, R., Lamonier, J.-F., Giraudon, J.-M., Su, B.-L., et al. (2014b). Investigation of the elimination of VOC mixtures over a Pd-loaded V-doped TiO2 support. New J. Chem. 38, 2066–2074. doi:10.1039/C3NJ01190A
Bartholomew, C. H. (2001). Mechanisms of catalyst deactivation. Appl. Catal. Gen. 212, 17–60. doi:10.1016/S0926-860X(00)00843-7
Becker, L., and Förster, H. (1997). Investigations of coke deposits formed during deep oxidation of benzene over Pd and Cu exchanged Y-type zeolites. Appl. Catal. Gen. 153, 31–41. doi:10.1016/S0926-860X(96)00344-4
Bedia, J., Rosas, J. M., Rodríguez-Mirasol, J., and Cordero, T. (2010). Pd supported on mesoporous activated carbons with high oxidation resistance as catalysts for toluene oxidation. Appl. Catal. B Environ. 94 (1–2), 8–18. doi:10.1016/j.apcatb.2009.10.015
Bendahou, K., Cherif, L., Siffert, S., Tidahy, H. L., Benaïssa, H., and Aboukaïs, A. (2008). The effect of the use of lanthanum-doped mesoporous SBA-15 on the performance of Pt/SBA-15 and Pd/SBA-15 catalysts for total oxidation of toluene. Appl. Catal. Gen. 351 (1), 82–87. doi:10.1016/j.apcata.2008.09.001
Bernal, S., Blanco, G., Pintado, J. M., Rodríguez-Izquierdo, J. M., and Yeste, M. P. (2005). An alternative way of reporting on the redox behaviour of ceria-based catalytic materials: temperature-chemical environment-oxidation state diagrams. Catal. Commun. 6 (9), 582–585. doi:10.1002/sia.1266
Bi, F., Zhang, X., Chen, J., Yang, Y., and Wang, Y. (2020). Excellent catalytic activity and water resistance of UiO-66-supported highly dispersed Pd nanoparticles for toluene catalytic oxidation. Appl. Catal. B Environ. 269, 118767. doi:10.1016/j.apcatb.2020.118767
bin Saiman, M. I., Brett, G. L., Tiruvalam, R., Forde, M. M., Sharples, K., Thetford, A., et al. (2012). Involvement of surface-bound radicals in the oxidation of toluene using supported Au-Pd nanoparticles. Angew. Chem. Int. Ed. Engl. 51 (24), 5981–5985. doi:10.1002/anie.201201059
Boeglin, M. L., Wessels, D., and Henshel, D. (2006). An investigation of the relationship between air emissions of volatile organic compounds and the incidence of cancer in Indiana counties. Environ. Res. 100 (2), 242–254. doi:10.1016/j.envres.2005.04.004
Boltic, Z., Ruzic, N., Jovanovic, M., Savic, M., Jovanovic, J., and Petrovic, S. (2013). Cleaner production aspects of tablet coating process in pharmaceutical industry: problem of VOCs emission. J. Clean. Prod. 44, 123–132. doi:10.1016/j.jclepro.2013.01.004
Cao, S., Fei, X., Wen, Y., Sun, Z., Wang, H., and Wu, Z. (2018). Bimodal mesoporous TiO2 supported Pt, Pd and Ru catalysts and their catalytic performance and deactivation mechanism for catalytic combustion of dichloromethane (CH2Cl2). Appl. Catal. Gen. 550, 20–27. doi:10.1016/j.apcata.2017.10.006
Chang, C. R., Huang, Z.-Q., and Li, J. (2016). The promotional role of water in heterogeneous catalysis: mechanism insights from computational modeling. Wiley Interdiscip. Rev. Comput. Mol. Sci. 6 (6), 679–693. doi:10.1002/wcms.1272
Chen, T., and Rodionov, V. O. (2016). Controllable catalysis with nanoparticles: bimetallic alloy systems and surface adsorbates. ACS Catal. 6 (6), 4025–4033. doi:10.1021/acscatal.6b00714
Chen, Z., Li, J., Yang, P., Cheng, Z., Li, J., and Zuo, S. (2019a). Ce-modified mesoporous γ-Al2O3 supported Pd-Pt nanoparticle catalysts and their structure-function relationship in complete benzene oxidation. Chem. Eng. J. 356, 255–261. doi:10.1016/j.cej.2018.09.040
Chen, Z., Situ, D., Zheng, J., and Cheng, Z. (2019b). Y-modified MCM-22 supported PdOx nanocrystal catalysts for catalytic oxidation of toluene. Catalysts 9 (11), 902. doi:10.3390/catal9110902
Dai, Q. G., Zhu, Q., Lou, Y., and Wang, X. (2018). Role of Brønsted acid site during catalytic combustion of methane over PdO/ZSM-5: dominant or negligible? J. Catal. 357, 29–40. doi:10.1016/j.jcat.2017.09.022
Dege, P., Pinard, L., Magnoux, P., and Guisnet, M. (2000). Catalytic oxidation of volatile organic compounds II. Influence of the physicochemical characteristics of Pd/HFAU catalysts on the oxidation of o-xylene. Appl. Catal. B Environ. 27, 17–26. doi:10.1016/S0926-3373(00)00135-1
de la Peña O’Shea, V. A., Álvarez-Galván, M. C., Fierro, J. L. G., and Arias, P. L. (2005). Influence of feed composition on the activity of Mn and PdMn/Al2O3 catalysts for combustion of formaldehyde/methanol. Appl. Catal. B Environ. 57 (3), 191–199. doi:10.1016/j.apcatb.2004.11.001
Dong, T., Liu, W., Ma, M., Peng, H., Yang, S., Tao, J., et al. (2020). Hierarchical zeolite enveloping Pd-CeO2 nanowires: an efficient adsorption/catalysis bifunctional catalyst for low temperature propane total degradation. Chem. Eng. J. 393, 124717. doi:10.1016/j.cej.2020.124717
Dumanoglu, Y., Kara, M., Altiok, H., Odabasi, M., Elbir, T., and Bayram, A. (2014). Spatial and seasonal variation and source apportionment of volatile organic compounds (VOCs) in a heavily industrialized region. Atmos. Environ. 98, 168–178. doi:10.1016/j.atmosenv.2014.08.048
Enache, D. I., Edwards, J. K., Landon, P., and Solsona, B. (2006). Solvent-free oxidation of primary alcohols to aldehydes using Au-Pd/TiO2 catalysts. Science 311 (5759), 362–365. doi:10.1126/science.1120560
Flytzani-Stephanopoulos, M. (2017). Supported metal catalysts at the single-atom limit - a viewpoint. Chin. J. Catal. 38 (9), 1432–1442. doi:10.1016/S1872-2067(17)62886-9
Garcia, T., Solsona, B., Cazorlaamoros, D., Linaressolano, A., and Taylor, S. (2006). Total oxidation of volatile organic compounds by vanadium promoted palladium-titania catalysts: comparison of aromatic and polyaromatic compounds. Appl. Catal. B Environ. 62 (1–2), 66–76. doi:10.1016/j.apcatb.2005.06.016
Garcia, T., Solsona, B., Murphy, D., Antcliff, K., and Taylor, S. (2005). Deep oxidation of light alkanes over titania-supported palladium/vanadium catalysts. J. Catal. 229 (1), 1–11. doi:10.1016/j.jcat.2004.09.018
Gil, S., Garcia-Vargas, J., Liotta, L., Pantaleo, G., Ousmane, M., Retailleau, L., et al. (2015). Catalytic oxidation of propene over Pd catalysts supported on CeO2, TiO2, Al2O3 and M/Al2O3 oxides (M = Ce, Ti, Fe, Mn). Catalysts 5 (2), 671–689. doi:10.3390/catal5020671
Giraudon, J. M., Elhachimi, A., and Leclercq, G. (2008a). Catalytic oxidation of chlorobenzene over Pd/perovskites. Appl. Catal. B Environ. 84 (1–2), 251–261. doi:10.1016/j.apcatb.2008.04.023
Giraudon, J. M., Nguyen, T. B., Leclercq, G., Siffert, S., Lamonier, J.-F., Aboukaïs, A., et al. (2008b). Chlorobenzene total oxidation over palladium supported on ZrO2, TiO2 nanostructured supports. Catal. Today. 137 (2–4), 379–384. doi:10.1016/j.cattod.2008.02.019
Gonzalez-Velasco, J. R., Aranzabal, A., López-Fonseca, R., Ferret, R., and González-Marcos, J. A. (2000). Enhancement of the catalytic oxidation of hydrogen-lean chlorinated VOCs in the presence of hydrogen-supplying compounds. Appl. Catal. B Environ. 24, 33–43. doi:10.1016/S0926-3373(99)00087-9
Guisnet, M., Costa, L., and Ribeiro, F. R. (2009). Prevention of zeolite deactivation by coking. J. Mol. Catal. Chem. 305 (1–2), 69–83. doi:10.1016/j.molcata.2008.11.012
Guisnet, M., and Magnoux, P. (1994). Fundamental description of deactivation and regeneration of acid zeolites. Stud. Surf. Sci. Catal. 88, 53–68. doi:10.1016/S0167-2991(08)62729-9
Gulsnet, M., and Magnoux, P. (1997). Deactivation by coking of zeolite catalysts. Prevention of deactivation. Optimal conditions for regeneration. Catal. Today. 36, 477–483. doi:10.1016/S0920-5861(96)00238-6
Guisnet, M., and Magnoux, P. (2001). Organic chemistry of coke formation. Appl. Catal. Gen. 212, 83–96. doi:10.1016/S0926-860X(00)00845-0
He, C., Cheng, J., Zhang, X., Douthwaite, M., Pattisson, S., and Hao, Z. (2019). Recent advances in the catalytic oxidation of volatile organic compounds: a review based on pollutant sorts and sources. Chem. Rev. 119 (7), 4471–4568. doi:10.1021/acs.chemrev.8b00408
He, C., Li, J., Cheng, J., Li, L., Li, P., Hao, Z., et al. (2009). Comparative studies on porous material-supported Pd catalysts for catalytic oxidation of benzene, toluene, and ethyl acetate. Ind. Eng. Chem. Res. 48, 6930–6936. doi:10.1021/ie900412c
He, C., Li, J., Li, P., Cheng, J., Hao, Z., and Xu, Z.-P. (2010a). Comprehensive investigation of Pd/ZSM-5/MCM-48 composite catalysts with enhanced activity and stability for benzene oxidation. Appl. Catal. B Environ. 96 (3–4), 466–475. doi:10.1016/j.apcatb.2010.03.005
He, C., Li, J., Zhang, X., Yin, L., Chen, J., and Gao, S. (2012a). Highly active Pd-based catalysts with hierarchical pore structure for toluene oxidation: catalyst property and reaction determining factor. Chem. Eng. J. 180, 46–56. doi:10.1016/j.cej.2011.10.099
He, C., Li, P., Cheng, J., Hao, Z.-P., and Xu, Z.-P. (2010b). A comprehensive study of deep catalytic oxidation of benzene, toluene, ethyl acetate, and their mixtures over Pd/ZSM-5 catalyst: mutual effects and kinetics. Water Air Soil Pollut. 209, 365–376. doi:10.1007/s11270-009-0205-7
He, C., Li, P., Cheng, J., Li, J., and Hao, Z. (2010c). Preparation and investigation of Pd/Ti-SBA-15 catalysts for catalytic oxidation of benzene. Environ. Prog. Sustain. Energy. 29 (4), 435–442. doi:10.1002/ep.10427
He, C., Li, Q., Li, P., Wang, Y., Zhang, X., Cheng, J., et al. (2010d). Templated silica with increased surface area and expanded microporosity: synthesis, characterization, and catalytic application. Chem. Eng. J. 162 (3), 901–909. doi:10.1016/j.cej.2010.06.037
He, C., Xu, L., Yue, L., Chen, Y., Chen, J., and Hao, Z. (2012b). Supported nanometric Pd hierarchical catalysts for efficient toluene removal: catalyst characterization and activity elucidation. Ind. Eng. Chem. Res. 51 (21), 7211–7222. doi:10.1021/ie201243c
He, C., Yue, L., Zhang, X., Li, P., Dou, B., Ma, C., et al. (2012c). Deep catalytic oxidation of benzene, toluene, ethyl acetate over Pd/SBA-15 catalyst: reaction behaviors and kinetics. Asia Pac. J. Chem. Eng. 7 (5), 705–715. doi:10.1002/apj.621
He, C., Zhang, F., Yue, L., Shang, X., Chen, J., and Hao, Z. (2012d). Nanometric palladium confined in mesoporous silica as efficient catalysts for toluene oxidation at low temperature. Appl. Catal. B Environ. 111, 46–57. doi:10.1016/j.apcatb.2011.09.017
He, F., Muliane, U., Weon, S., and Choi, W. (2020). Substrate-specific mineralization and deactivation behaviors of TiO2 as an air-cleaning photocatalyst. Appl. Catal. B Environ. 275, 119145. doi:10.1016/j.apcatb.2020.119145
He, Z., He, Z., Wang, D., Bo, Q., Fan, T., and Jiang, Y. (2014). Mo-modified Pd/Al2O3 catalysts for benzene catalytic combustion. J. Environ. Sci. 26 (7), 1481–1487. doi:10.1016/j.jes.2014.05.014
Hong, J., Chu, W., Chen, M., Wang, X., and Zhang, T. (2007). Preparation of novel titania supported palladium catalysts for selective hydrogenation of acetylene to ethylene. Catal. Commun. 8 (3), 593–597. doi:10.1016/j.catcom.2006.08.010
Hosseini, M., Barakat, T., Cousin, R., Aboukaïs, A., Su, B.-L., De Weireld, G., et al. (2012). Catalytic performance of core–shell and alloy Pd–Au nanoparticles for total oxidation of VOC: the effect of metal deposition. Appl. Catal. B Environ. 111–112, 218–224. doi:10.1016/j.apcatb.2011.10.002
Hosseini, M., Haghighi, M., Kahforoushan, D., and Zarrabi, M. (2017). Sono-dispersion of ceria and palladium in preparation and characterization of Pd/Al2O3-clinoptilolite-CeO2 nanocatalyst for treatment of polluted air via low temperature VOC oxidation. Process Saf. Environ. Protect. 106, 284–293. doi:10.1016/j.psep.2016.06.028
Huang, H. B., and Leung, D. Y. C., (2011). Complete oxidation of formaldehyde at room temperature using TiO2 supported metallic Pd nanoparticles. ACS Catal. 1 (4), 348–354. doi:10.1021/cs200023p
Ihm, S.-K., Jun, Y.-D., Kim, D.-C., and Jeong, K.-E. (2004). Low-temperature deactivation and oxidation state of Pd/γ-Al2O3 catalysts for total oxidation of n-hexane. Catal. Today. 93–95, 149–154. doi:10.1016/j.cattod.2004.06.096
Iranpour, R., Cox, H. H. J., Deshusses, M. A., and Schroeder, E. D. (2005). Literature review of air pollution control biofilters and biotrickling filters for odor and volatile organic compound removal. Environ. Prog. 24 (3), 254–267. doi:10.1002/ep.10077
Jablonska, M., Król, A., Kukulska-Zając, E., Tarach, K., Girman, V., Chmielarz, L., et al. (2015). Zeolites Y modified with palladium as effective catalysts for low-temperature methanol incineration. Appl. Catal. B Environ. 166–167, 353–365. doi:10.1016/j.apcatb.2014.11.047
Jin, L. Y., Ma, R.-H., Lin, J.-J., Meng, L., Wang, Y.-J., and Luo, M.-F. (2011). Bifunctional Pd/Cr2O3–ZrO2Catalyst for the oxidation of volatile organic compounds. Ind. Eng. Chem. Res. 50 (18), 10878–10882. doi:10.1021/ie200599v
Jinjun, L., Zheng, J., Zhengping, H., Xiuyan, X., and Yahui, Z. (2005). Pillared laponite clays-supported palladium catalysts for the complete oxidation of benzene. J. Mol. Catal. Chem. 225 (2), 173–179. doi:10.1016/j.molcata.2004.08.040
Kamal, M. S., Razzak, S. A., and Hossain, M. M. (2016). Catalytic oxidation of volatile organic compounds (VOCs)-a review. Atmos. Environ. 140, 117–134. doi:10.1016/j.atmosenv.2016.05.031
Kim, M. Y., Kyriakidou, E. A., Choi, J.-S., Toops, T. J., Binder, A. J., Thomas, C., et al. (2016). Enhancing low-temperature activity and durability of Pd-based diesel oxidation catalysts using ZrO2 supports. Appl. Catal. B Environ. 187, 181–194. doi:10.1016/j.apcatb.2016.01.023
Kim, S., and Choi, W. (2002). Kinetics and mechanisms of photocatalytic degradation of (CH3)nNH4-n+ (0 ≤ n ≤ 4) in TiO2 suspension: the role of OH radicals. Environ. Sci. Technol. 36, 2019–2025. doi:10.1021/es015560s
Kim, S. C., and Shim, W. G. (2009). Properties and performance of Pd based catalysts for catalytic oxidation of volatile organic compounds. Appl. Catal. B Environ. 92 (3–4), 429–436. doi:10.1016/j.apcatb.2009.09.001
Kim, S. C., and Shim, W. G. (2010). Catalytic combustion of VOCs over a series of manganese oxide catalysts. Appl. Catal. B Environ. 98 (3–4), 180–185. doi:10.1016/j.apcatb.2010.05.027
Kondarides, D. I., and Verykios, X. E. (1998). Effect of chlorine on the chemisorptive properties of Rh/CeO2 catalysts studied by XPS and temperature programmed desorption techniques. J. Catal. 174, 52–64. doi:10.1006/jcat.1997.1938
Krocher, O., and Elsener, M. (2009). Hydrolysis and oxidation of gaseous HCN over heterogeneous catalysts. Appl. Catal. B Environ. 92, 75–89. doi:10.1016/j.apcatb.2009.07.021
Li, P., He, C., Cheng, J., Ma, C. Y., Dou, B. J., and Hao, Z. P. (2011). Catalytic oxidation of toluene over Pd/Co3AlO catalysts derived from hydrotalcite-like compounds: effects of preparation methods. Appl. Catal. B Environ. 101 (3–4), 570–579. doi:10.1016/j.apcatb.2010.10.030
Li, Y., Zhang, C., Ma, J., Chen, M., Deng, H., and He, H. (2017). High temperature reduction dramatically promotes Pd/TiO2 catalyst for ambient formaldehyde oxidation. Appl. Catal. B Environ. 217, 560–569. doi:10.1016/j.apcatb.2017.06.023
Liang, X., Chen, X., Zhang, J., Shi, T., Sun, X., Fan, L., et al. (2017). Reactivity-based industrial volatile organic compounds emission inventory and its implications for ozone control strategies in China. Atmos. Environ. 162, 115–126. doi:10.1016/j.atmosenv.2017.04.036
Liang, Y., Liu, Y., Deng, J., Zhang, K., Hou, Z., Zhao, X., et al. (2019). Coupled palladium-tungsten bimetallic nanosheets/TiO2 hybrids with enhanced catalytic activity and stability for the oxidative removal of benzene. Environ. Sci. Technol. 53 (10), 5926–5935. doi:10.1021/acs.est.9b00370
Liao, H. C., Zuo, P., and Liu, M. (2016). Study on the correlation between the surface active species of Pd/cordierite monolithic catalyst and its catalytic activity. Mater. Sci. Eng. B 211, 45–52. doi:10.1016/j.mseb.2016.04.015
Liao, H. T., Chou, C. C.-K., Chow, J. C., Watson, J. G., Hopke, P. K., and Wu, C.-F. (2015). Source and risk apportionment of selected VOCs and PM2.5 species using partially constrained receptor models with multiple time resolution data. Environ. Pollut. 205, 121–130. doi:10.1016/j.envpol.2015.05.035
Liotta, L. F. (2010). Catalytic oxidation of volatile organic compounds on supported noble metals. Appl. Catal. B Environ. 100 (3–4), 403–412. doi:10.1016/j.apcatb.2010.08.023
Liu, F. J., Zuo, S., Wang, C., Li, J., Xiao, F.-S., and Qi, C. (2014). Pd/transition metal oxides functionalized ZSM-5 single crystals with b-axis aligned mesopores: efficient and long-lived catalysts for benzene combustion. Appl. Catal. B Environ. 148–149, 106–113. doi:10.1016/j.apcatb.2013.10.054
Liu, J., Wang, H., Chen, Y., Yang, M., and Wu, Y. (2014). Effects of pretreatment atmospheres on the catalytic performance of Pd/γ-Al2O3 catalyst in benzene degradation. Catal. Commun. 46, 11–16. doi:10.1016/j.catcom.2013.10.031
Liu, X., Chen, L., Zhu, T., and Ning, R. (2019). Catalytic oxidation of chlorobenzene over noble metals (Pd, Pt, Ru, Rh) and the distributions of polychlorinated by-products. J. Hazard Mater. 363, 90–98. doi:10.1016/j.jhazmat.2018.09.074
Lupescu, J. A., Schwank, J. W., Fisher, G. B., Chen, X., Peczonczyk, S. L., and Drews, A. R. (2016). Pd model catalysts: effect of aging duration on lean redispersion. Appl. Catal. B Environ. 185, 189–202. doi:10.1016/j.apcatb.2015.12.012
Ma, M., Jian, Y., Chen, C., and He, C. (2020). Spherical-like Pd/SiO2 catalysts for n-butylamine efficient combustion: effect of support property and preparation method. Catal. Today. 339, 181–191. doi:10.1016/j.cattod.2018.11.024
Marécot, P., Fakche, A., Kellali, B., Mabilon, G., Prigent, P., and Barbier, J. (1994). Propane and propene oxidation over platinum and palladium on alumina: effects of chloride and water. Appl. Catal. B Environ. 3, 283–294. doi:10.1016/0926-3373(94)00003-4
Odoom-Wubah, T., Li, Q., Adilov, I., Huang, J., and Li, Q. (2019a). Towards efficient Pd/Mn3O4 catalyst with enhanced acidic sites and low temperature reducibility for benzene abatement. Mol. Catal. 477, 110558. doi:10.1016/j.mcat.2019.110558
Odoom-Wubah, T., Li, Q., Mulka, R., Chen, M., Huang, J., Li, Q., et al. (2019b). Calcified shrimp waste supported Pd NPs as an efficient catalyst toward benzene destruction. ACS Sustain. Chem. Eng. 8 (1), 486–497. doi:10.1021/acssuschemeng.9b05671
Odoom-Wubah, T., Li, Q., Wang, Q., Usha, M. Z. R., Huang, J., and Li, Q. (2019c). Template-free synthesis of carbon self-doped ZnO superstructures as efficient support for ultra fine Pd nanoparticles and their catalytic activity towards benzene oxidation. Mol. Catal. 469, 118–130. doi:10.1016/j.mcat.2019.03.013
Okumura, K., Kobayashi, T., Tanaka, H., and Niwa, M. (2003a). Toluene combustion over palladium supported on various metal oxide supports. Appl. Catal. B Environ. 44 (4), 325–331. doi:10.1016/S0926-3373(03)00101-2
Okumura, K., Matsumoto, S., Nishiaki, N., and Niwa, M. (2003b). Support effect of zeolite on the methane combustionactivity of palladium. Appl. Catal. B Environ. 40, 151–159. doi:10.1016/S0926-3373(02)00149-2
Padilla, J. M., Del Angel, G., and Navarrete, J. (2008). Improved Pd/γ-Al2O3-Ce catalysts for benzene combustion. Catal. Today. 133–135, 541–547. doi:10.1016/j.cattod.2007.12.053
Paulis, M., Peyrard, H., and Montes, M. (2001). Influence of chlorine on the activity and stability of Pt/Al2O3 catalysts in the complete oxidation of toluene. J. Catal. 199 (1), 30–40. doi:10.1006/jcat.2000.3146
Pérez-Cadenas, A. F., Morales-Torres, S., Kapteijn, F., Maldonado-Hódar, F. J., Carrasco-Marín, F., Moreno-Castilla, C., et al. (2008). Carbon-based monolithic supports for palladium catalysts: the role of the porosity in the gas-phase total combustion of m-xylene. Appl. Catal. B Environ. 77 (3–4), 272–277. doi:10.1016/j.apcatb.2007.07.030
Pitkäaho, S., Nevanperä, T., Matejova, L., Ojala, S., and Keiski, R. L. (2013). Oxidation of dichloromethane over Pt, Pd, Rh, and V2O5 catalysts supported on Al2O3, Al2O3-TiO2 and Al2O3-CeO2. Appl. Catal. B Environ. 138–139, 33–42. doi:10.1016/j.apcatb.2013.01.058
Qiu, K., Yang, L., Lin, J., Wang, P., Yang, Y., Ye, D., et al. (2014). Historical industrial emissions of non-methane volatile organic compounds in China for the period of 1980–2010. Atmos. Environ. 86, 102–112. doi:10.1016/j.atmosenv.2013.12.026
Ramírez-López, R., Elizalde-Martinez, I., and Balderas-Tapia, L. (2010). Complete catalytic oxidation of methane over Pd/CeO2-Al2O3: the influence of different ceria loading. Catal. Today. 150 (3–4), 358–362. doi:10.1016/j.cattod.2009.10.007
Ren, S., Liang, W., Li, Q., and Zhu, Y. (2020). Effect of Pd/Ce loading on the performance of Pd-Ce/gamma-Al2O3 catalysts for toluene abatement. Chemosphere 251, 126382. doi:10.1016/j.chemosphere.2020.126382
Rooke, J. C., Barakat, T., Siffert, S., and Su, B.-L. (2012). Total catalytic oxidation of toluene using Pd impregnated on hierarchically porous Nb2O5 and Ta2O5 supports. Catal. Today. 192 (1), 183–188. doi:10.1016/j.cattod.2011.10.011
Ryczkowski, J. (2001). IR spectroscopy in catalysis. Catal. Today. 68, 263–381. doi:10.1016/S0920-5861(01)00334-0
Ryndin, Y. A., Hicks, R. F., Bell, A. T., and Yermakov, Y. I. (1981). Effects of metal-support interactions on the synthesis of methanol over palladium. J. Catal. 70, 287–297. doi:10.1016/0021-9517(81)90341-9
Santos, V. P., Carabineiro, S. A. C., Tavares, P. B., Pereira, M. F. R., Órfão, J. J. M., and Figueiredo, J. L. (2010a). Oxidation of CO, ethanol and toluene over TiO2 supported noble metal catalysts. Appl. Catal. B Environ. 99 (1–2), 198–205. doi:10.1016/j.apcatb.2010.06.020
Santos, V. P., Pereira, M. F. R., Órfão, J. J. M., and Figueiredo, J. L. (2010b). The role of lattice oxygen on the activity of manganese oxides towards the oxidation of volatile organic compounds. Appl. Catal. B Environ. 99 (1–2), 353–363. doi:10.1016/j.apcatb.2010.07.007
Scire, S., and Liotta, L. F. (2012). Supported gold catalysts for the total oxidation of volatile organic compounds. Appl. Catal. B Environ. 125, 222–246. doi:10.1016/j.apcatb.2012.05.047
Shi, H. X., Chen, J., Li, G., Nie, X., Zhao, H., Wong, P.-K., et al. (2013). Synthesis and characterization of novel plasmonic Ag/AgX-CNTs (X = Cl, Br, I) nanocomposite photocatalysts and synergetic degradation of organic pollutant under visible light. ACS Appl. Mater. Interfaces. 5 (15), 6959–6967. doi:10.1021/am401459c
Spezzati, G., Su, Y., Hofmann, J. P., Benavidez, A. D., DeLaRiva, A. T., McCabe, J., et al. (2017). Atomically dispersed Pd-O species on CeO2(111) as highly active sites for low-temperature CO oxidation. ACS Catal. 7 (10), 6887–6891. doi:10.1021/acscatal.7b02001
Srivastava, A., Joseph, A. E., Patil, S., More, A., Dixit, R. C., and Prakash, M. (2005). Air toxics in ambient air of Delhi. Atmos. Environ. 39 (1), 59–71. doi:10.1016/j.atmosenv.2004.09.053
Tang, W., Deng, Y., and Chen, Y. (2017). Promoting effect of acid treatment on Pd-Ni/SBA-15 catalyst for complete oxidation of gaseous benzene. Catal. Commun. 89, 86–90. doi:10.1016/j.catcom.2016.10.032
Tauster, S. J., Fung, S. C., and Garten, R. L. (1978). Strong metal-support interactions. Group 8 noble metals supported on titanium dioxide. J. Am. Chem. Soc. 100 (1), 170–175. doi:10.1021/ja00469a029
Taylor, M. N., Zhou, W., Garcia, T., Solsona, B., Carley, A. F., Kiely, C. J., et al. (2012). Synergy between tungsten and palladium supported on titania for the catalytic total oxidation of propane. J. Catal. 285, 103–114. doi:10.1016/j.jcat.2011.09.019
Tidahy, H. L., Hosseni, M., Siffert, S., Cousin, R., Lamonier, J.-F., Aboukaïs, A., et al. (2008). Nanostructured macro-mesoporous zirconia impregnated by noble metal for catalytic total oxidation of toluene. Catal. Today. 137, 335–339. doi:10.1016/j.cattod.2007.09.008
Tidahy, H. L., Siffert, S., Lamonier, J.-F., Zhilinskaya, E. A., Aboukaïs, A., Yuan, Z.-Y., et al. (2006). New Pd/hierarchical macro-mesoporous ZrO2, TiO2 and ZrO2-TiO2 catalysts for VOCs total oxidation. Appl. Catal. Gen. 310, 61–69. doi:10.1016/j.apcata.2006.05.020
Tomatis, M., Xu, H.-H., He, J., and Zhang, X.-D. (2016). Recent development of catalysts for removal of volatile organic compounds in flue gas by combustion: a review. J. Chem. 2016, 1–15. doi:10.1155/2016/8324826
van den Brink, R. W., Krzan, M., Feijen-Jeurissen, M. M. R., Louw, R., and Mulder, P. (2000). The role of the support and dispersion in the catalytic combustion of chlorobenzene on noble metal based catalysts. Appl. Catal. B Environ. 24, 255–264. doi:10.1016/S0926-3373(99)00113-7
Venezia, A. M., Di Carlob, G., Liottaa, L. F., Pantaleoa, G., and Kantchevac, M. (2011). Effect of Ti(IV) loading on CH4 oxidation activity and SO2 tolerance of Pd catalysts supported on silica SBA-15 and HMS. Appl. Catal. B Environ. 106 (3–4), 529–539. doi:10.1016/j.apcatb.2011.06.013
Vu, V. H., Belkouch, J., Ould-Dris, A., and Taouk, B. (2009). Removal of hazardous chlorinated VOCs over Mn-Cu mixed oxide based catalyst. J. Hazard Mater. 169 (1–3), 758–765. doi:10.1016/j.jhazmat.2009.04.010
Wang, H., Yang, W., Tian, P., Zhou, J., Tang, R., and Wu, S. (2017). A highly active and anti-coking Pd-Pt/SiO2 catalyst for catalytic combustion of toluene at low temperature. Appl. Catal. Gen. 529, 60–67. doi:10.1016/j.apcata.2016.10.016
Wang, N. F., Li, S., Zong, Y., and Yao, Q. (2017). Sintering inhibition of flame-made Pd/CeO2 nanocatalyst for low-temperature methane combustion. J. Aerosol Sci. 105, 64–72. doi:10.1016/j.jaerosci.2016.11.017
Wang, Y., Xiao, L., Zhao, C., Liu, F., and Li, S. (2018a). Catalytic combustion of toluene with Pd/La0.8Ce0.2MnO3 supported on different zeolites. Environ. Prog. Sustain. Energy. 37 (1), 215–220. doi:10.1002/ep.12656
Wang, Y., Zhang, C., and He, H. (2018b). Insight into the role of Pd state on Pd-based catalysts in o-xylene oxidation at low temperature. ChemCatChem 10 (5), 998–1004. doi:10.1002/cctc.201701547
Wang, Y., Zhang, C., Liu, F., and He, H. (2013). Well-dispersed palladium supported on ordered mesoporous Co3O4 for catalytic oxidation of o-xylene. Appl. Catal. B Environ. 142–143, 72–79. doi:10.1016/j.apcatb.2013.05.003
Wang, Y., Zhang, C., Yu, Y., Yue, R., and He, H. (2015). Ordered mesoporous and bulk Co3O4 supported Pd catalysts for catalytic oxidation of o-xylene. Catal. Today. 242, 294–299. doi:10.1016/j.cattod.2014.06.032
Wei, Y., Zhang, X., Liu, Y., Jia, C., and Yang, P. (2020). Ternary PtNiCu self-assembled nanocubes for plasmon-enhanced electrocatalytic hydrogen evolution and methanol oxidation reaction in visible light. Electrochim. Acta. 349, 136366. doi:10.1016/j.electacta.2020.136366
Weng, X., Shi, B., Liu, A., Sun, J., Xiong, Y., Wan, H., et al. (2019). Highly dispersed Pd/modified-Al2O3 catalyst on complete oxidation of toluene: role of basic sites and mechanism insight. Appl. Surf. Sci. 497, 143747. doi:10.1016/j.apsusc.2019.143747
Wu, S. M., Yang, X.-Y., and Janiak, C. (2019). Confinement effects in zeolite-confined noble metals. Angew. Chem. Int. Ed. Engl. 58 (36), 12340–12354. doi:10.1002/anie.201900013
Wu, Z., Deng, J., Xie, S., Yang, H., Zhao, X., Zhang, K., et al. (2016). Mesoporous Cr2O3-supported Au-Pd nanoparticles: high-performance catalysts for the oxidation of toluene. Microporous Mesoporous Mater. 224, 311–322. doi:10.1016/j.micromeso.2015.11.061
Xia, Y., Xia, L., Liu, Y., Yang, T., Deng, J., and Dai, H. (2018). Concurrent catalytic removal of typical volatile organic compound mixtures over Au-Pd/alpha-MnO2 nanotubes. J. Environ. Sci. 64, 276–288. doi:10.1016/j.jes.2017.06.025
Xie, P., Pu, T., Nie, A., Hwang, S., Purdy, S. C., Yu, W., et al. (2018). Nanoceria-supported single-atom platinum catalysts for direct methane conversion. ACS Catal. 8 (5), 4044–4048. doi:10.1021/acscatal.8b00004
Xiong, H. F., Wiebenga, M. H., Carrillo, C., Gaudet, J. R., Pham, H. N., Kunwar, D., et al. (2018). Design considerations for low-temperature hydrocarbon oxidation reactions on Pd based catalysts. Appl. Catal. B Environ. 236, 436–444. doi:10.1016/j.apcatb.2018.05.049
Xu, Q., Kharas, K. C., Croley, B. J., and Datye, A. K. (2011). The sintering of supported Pd automotive catalysts. ChemCatChem 3 (6), 1004–1014. doi:10.1002/cctc.201000392
Yang, K., Liu, Y., Deng, J., Zhao, X., Yang, J., Han, Z., et al. (2019). Three-dimensionally ordered mesoporous iron oxide-supported single-atom platinum: highly active catalysts for benzene combustion. Appl. Catal. B Environ. 244, 650–659. doi:10.1016/j.apcatb.2018.11.077
Yang, L., Ma, J., Hu, W., Dai, Q., Wang, L., Zhan, W., et al. (2016). Low-temperature methane combustion over Pd/H-ZSM-5: active Pd sites with specific electronic properties modulated by acidic sites of H-ZSM-5. ACS Catal. 6 (12), 8127–8139. doi:10.1021/acscatal.6b01801
Yang, W., Wang, H., and Fu, F. (2019). PdCu nanoalloys deposited on porous carbon as a highly efficient catalyst for ethanol oxidation. Mater. Chem. Phys. 228, 175–179. doi:10.1016/j.matchemphys.2019.02.075
Yang, X., Ma, X., Yu, X., and Ge, M. (2020). Exploration of strong metal-support interaction in zirconia supported catalysts for toluene oxidation. Appl. Catal. B Environ. 263, 118355. doi:10.1016/j.apcatb.2019.118355
Yang, X., Wang, A., Qiao, B., Li, J., Liu, J., and Zhang, T. (2012). Single-atom catalysts: a new frontier in heterogeneous catalysis. Acc. Chem. Res. 46, 1740–1748. doi:10.1021/ar300361m
Yang, Y. (2020). Carbon deposits during catalytic combustion of toluene on Pd-Pt-based catalysts. Catal. Sci. Technol. 10, 2452–2461. doi:10.1039/D0CY00101E
Yazawa, Y., Yoshida, H., Takagi, N., Komai, S.-i., Satsuma, A., and Hattori, T. (1999). Acid strength of support materials as a factor controlling oxidation state of palladium catalyst for propane combustion. J. Catal. 187 (1), 15–23. doi:10.1006/jcat.1999.2583
Yu, T. C., and Shaw, H. (1998). The effect of sulfur poisoning on methane oxidation over palladium supported on g-alumina catalysts. Appl. Catal. B Environ. 18, 105–114. doi:10.1016/S0926-3373(98)00031-9
Yu, W. Y., Zhang, L., Mullen, G. M., Henkelman, G., and Buddie Mullins, C. (2015). Oxygen activation and reaction on Pd-Au bimetallic surfaces. J. Phys. Chem. C 119 (21), 11754–11762. doi:10.1021/acs.jpcc.5b02970
Yuan, C. Z., Zhan, L.-Y., Liu, S.-J., Chen, F., Lin, H., Wu, X.-L., et al. (2020). Semi-sacrificial template synthesis of single-atom Ni sites supported on hollow carbon nanospheres for efficient and stable electrochemical CO2 reduction. Inorg. Chem. Front. 7 (8), 1719–1725. doi:10.1039/C9QI01688K
Yue, L., He, C., Hao, Z., Wang, S., and Wang, H. (2014). Effects of metal and acidic sites on the reaction by-products of butyl acetate oxidation over palladium-based catalysts. J. Environ. Sci. 26 (3), 702–707. doi:10.1016/S1001-0742(13)60439-8
Zang, M., Zhao, C., Wang, Y., Liu, X., Cheng, Y., and Chen, S. (2019). Low temperature catalytic combustion of toluene over three-dimensionally ordered La0.8Ce0.2MnO3/cordierite catalysts. Appl. Surf. Sci. 483, 355–362. doi:10.1016/j.apsusc.2019.03.320
Zeng, J., Liu, X., Wang, J., Lv, H., and Zhu, T. (2015). Catalytic oxidation of benzene over MnOx/TiO2 catalysts and the mechanism study. J. Mol. Catal. Chem. 408, 221–227. doi:10.1016/j.molcata.2015.07.024
Zhang, R., Shi, D., Liu, N., Cao, Y., and Chen, B. (2014). Mesoporous SBA-15 promoted by 3d-transition and noble metals for catalytic combustion of acetonitrile. Appl. Catal. B Environ. 146, 79–93. doi:10.1016/j.apcatb.2013.03.028
Zhang, X., Liu, Y., Deng, J., Yu, X., Han, Z., Zhang, K., et al. (2019a). Alloying of gold with palladium: an effective strategy to improve catalytic stability and chlorine-tolerance of the 3DOM CeO2 supported catalysts in trichloroethylene combustion. Appl. Catal. B Environ. 257, 117879, doi:10.1016/j.apcatb.2019.117879
Zhang, X., Lv, X., Shi, X., and Yang, Y. (2019b). Enhanced hydrophobic UiO-66 (University of Oslo 66) metal-organic framework with high capacity and selectivity for toluene capture from high humid air. J. Colloid Interface Sci. 539, 152–160. doi:10.1016/j.jcis.2018.12.056
Zhang, X., Yang, Y., Huang, W., Yang, Y., Wang, Y., He, C., et al. (2018). g-C3N4/UiO-66 nanohybrids with enhanced photocatalytic activities for the oxidation of dye under visible light irradiation. Mater. Res. Bull. 99, 349–358. doi:10.1016/j.materresbull.2017.11.028
Zhang, Z., Jiang, Z., and Shangguan, W. (2016). Low-temperature catalysis for VOCs removal in technology and application: a state-of-the-art review. Catal. Today. 264, 270–278. doi:10.1016/j.cattod.2015.10.040
Zhao, Q., Ge, Y., Fu, K., Zheng, Y., Liu, Q., Song, C., et al. (2019). Catalytic performance of the Pd/TiO2 modified with MnOx catalyst for acetone total oxidation. Appl. Surf. Sci. 496, 143579. doi:10.1016/j.apsusc.2019.143579
Zhao, S., Hu, F., and Li, J. (2016). Hierarchical core–shell Al2O3@Pd-CoAlO microspheres for low-temperature toluene combustion. ACS Catal. 6 (6), 3433–3441. doi:10.1021/acscatal.6b00144
Zhao, W., Liu, Y., Wang, L., Chu, J., Qu, J., Hao, Z., et al. (2011). Catalytic combustion of benzene on the Pd/nanosize Al-HMS. Microporous Mesoporous Mater. 138 (1–3), 215–220. doi:10.1016/j.micromeso.2010.08.013
Zhao, X., Zhang, R., Liu, Y., Deng, J., Xu, P., Lv, S., et al. (2019). Pd/meso-CoO derived from in situ reduction of the one-step synthesized Pd/meso-Co3O4: high performance catalysts for benzene combustion. New J. Chem. 43, 12358–12368. doi:10.1039/C9NJ03039E
Zhong, J., Zeng, Y., Zhang, M., and Feng, W. (2020). Toluene oxidation process and proper mechanism over Co3O4 nanotubes: investigation through in-situ DRIFTS combined with PTR-TOF-MS and quasi in-situ XPS. Chem. Eng. J. 397, 125375. doi:10.1016/j.cej.2020.125375
Zhu, J., Mu, W., Su, L., Li, X., Guo, Y., Zhang, S., et al. (2017). Al-doped TiO2 mesoporous material supported Pd with enhanced catalytic activity for complete oxidation of ethanol. J. Solid State Chem. 248, 142–149. doi:10.1016/j.jssc.2017.01.028
Zuo, S., Du, Y., Liu, F., Han, D., and Qi, C. (2013). Influence of ceria promoter on shell-powder-supported Pd catalyst for the complete oxidation of benzene. Appl. Catal. Gen. 451, 65–70. doi:10.1016/j.apcata.2012.10.041
Keywords: catalytic oxidation, Pd-supported catalysts, volatile organic compounds, deactivation mechanism, reaction pathway
Citation: Song S, Zhang S, Zhang X, Verma P and Wen M (2020) Advances in Catalytic Oxidation of Volatile Organic Compounds over Pd-Supported Catalysts: Recent Trends and Challenges. Front. Mater. 7:595667. doi: 10.3389/fmats.2020.595667
Received: 17 August 2020; Accepted: 08 September 2020;
Published: 09 October 2020.
Edited by:
David Salinas Torres, University of Alicante, SpainCopyright © 2020 Wen, Song, Zhang, Zhang and Verma. This is an open-access article distributed under the terms of the Creative Commons Attribution License (CC BY). The use, distribution or reproduction in other forums is permitted, provided the original author(s) and the copyright owner(s) are credited and that the original publication in this journal is cited, in accordance with accepted academic practice. No use, distribution or reproduction is permitted which does not comply with these terms.
*Correspondence: Meicheng Wen, meicheng.wen@gdut.edu.cn