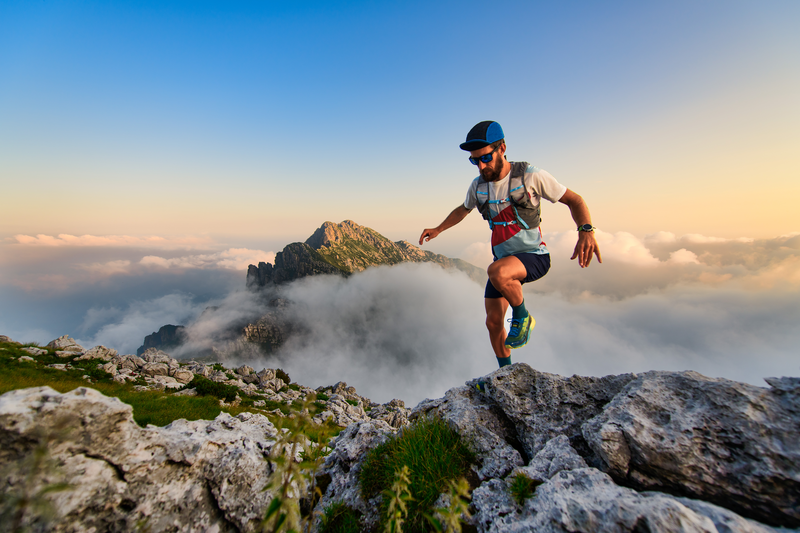
94% of researchers rate our articles as excellent or good
Learn more about the work of our research integrity team to safeguard the quality of each article we publish.
Find out more
ORIGINAL RESEARCH article
Front. Mater. , 30 October 2020
Sec. Environmental Degradation of Materials
Volume 7 - 2020 | https://doi.org/10.3389/fmats.2020.581979
This article is part of the Research Topic Degradation of Cultural Heritage Artifacts: From Archaeometry to Materials Development View all 8 articles
Natural stone has long been used as a building material because of its physical-mechanical resistance and its esthetic appeal. However, over the last century increasing industrial activity has produced more acidic environments, such as polluted urban areas, that can cause serious damage to many buildings and historic monuments, and in particular those made with carbonate rocks, which are most prone to decay. In order to mitigate the physicochemical processes that degrade these buildings, a number of phosphate-based consolidants have been developed, which are highly compatible with the carbonated substrate. Research about the role of the surface and its different possible finishes in the transmission of the agents that damage or protect the stone would therefore be very useful, both when choosing the most suitable stone for new constructions and when restoring historic buildings. The main objective of this research was to determine whether the roughness of three types of surface finish (saw-cut, honed and bush-hammered) influences the durability of four types of natural stone (two calcarenites, one travertine and one sandstone) widely used in Andalusia (Spain). The efficacy of a calcium phosphate-based consolidant as a mitigator of deterioration in polluted urban environments was also studied and to this end the physical properties of untreated and treated samples were measured and compared. The samples were exposed to artificial atmospheres with SO2 pollution in order to assess the damage caused to each surface finish. The results indicate that all the surface finishes were vulnerable to the decay caused by acidic atmospheres, although the saw-cut finish was less affected, perhaps because it did not require additional industrial processing. The mineral composition and texture of the rocks were critical factors in terms of the amount and type of decay they suffered, and the travertine and sandstone were more resistant to deterioration than the calcarenites. Similarly, the pore system of each rock was decisive in the penetration of the consolidant. Application of the consolidant improved the behavior of the treated samples by making them more resistant to acid attack without significantly altering the water vapor permeability, the color or the roughness of the surface.
Natural stone must satisfy a series of esthetic and durability requirements to enable it to be used as a building or ornamental material in both the restoration of historic buildings and in new constructions. Durability is one of the most important, most complex concepts that must be considered when choosing the type of stone to be used in a building project. An accurate assessment of the intrinsic properties (mineralogy, texture and pore system, etc.) of the stone and of its alterability is therefore required to enable quality criteria to be established regarding its use (Vázquez et al., 2013a). Various different authors have analyzed, via a range of accelerated aging tests, the relationship between a stone’s intrinsic properties and its resistance to decay (Tamrakar et al., 2007; Fort et al., 2013; Molina et al., 2013; De Kock et al., 2017; Fatah et al., 2018; Saba et al., 2019). However, less research has been done on the effects of the surface properties of the stone on its durability (e.g., Schiavon, 2000; Turkington et al., 2003; Vázquez et al., 2011; Urosevic et al., 2013; Vázquez et al., 2013b; Martinez-Martinez et al., 2019).
In architecture, the artistic and historic values of the stone are conveyed through its surface, which can be finished in different ways (Delgado Rodrigues, 2007). With the exception of granites and marbles, most of the rocks used in construction, and especially those of sedimentary origin, cannot be polished due to the fact that they have clastic textures and high porosity. These kinds of rock include limestones and sandstones, which have long been a popular choice for builders due to their abundant supply and the ease with which they can be worked. They were frequently used in historic buildings with surface finishes with varying degree of roughness, many of which have suffered decay over the centuries. The decay processes affecting rocks normally begin on the surface, which often suffer significant damage long before the interior is affected (Vázquez et al., 2011; Moses et al., 2014). The type and degree of decay suffered by a rock surface will depend on the alteration processes associated with external agents, both natural and anthropic, such as salt efflorescence, ice, biodeterioration, graffiti, acid rain, etc. (Přikryl and Smith, 2007; Price and Doehne, 2011; La Russa et al., 2013; Pinheiro et al., 2019; Hatir, 2020, and references therein). The increase in the concentration of certain chemical species in the atmosphere due to industrial activity and the combustion of fossil fuels in the 20th and early 21st century has become a very common cause of decay (Hamilton et al., 2009). The esthetic beauty of the surface of the stone is also impaired due to the development of crusts (Brimblecombe and Grossi, 2004). It is difficult to quantify the influence of atmospheric pollution on decay compared to other causes but the irreversible damage it can cause to rock has been demonstrated (Cultrone et al., 2000; McKinley et al., 2001; Behlen et al., 2008; Török, 2008; Unterwurzacher and Mirwald, 2008) and its impact is intensified by the presence of pollutant acids. One of these pollutants is SO2, which reacts with the moisture in the atmosphere, together with other pollutants, to produce acid rain, which in turn reacts quickly with the carbonated materials forming patinas and crusts (composed above all of gypsum crystals) on the surface of the stone (Saiz Jiménez et al., 2004; La Russa et al., 2017). Other authors established a relationship between the roughness of the surface and the likelihood of it being covered by black crusts or particles (Pio et al., 1998; Grossi et al., 2003), which indicates that in addition to external and internal factors, the roughness of this exposed area must also be considered when assessing the durability of natural stone.
In an attempt to slow down the stone decay process, research has been conducted since at least the end of the 19th century on a wide range of consolidant products (both organic and inorganic) for use in new construction and in stone restoration and conservation work (Wheeler, 2005). These include ethyl silicates, silicone resins, oligomeric siloxanes, silanes, organic aluminum compounds, phosphates, acrylic resins, vinyls, epoxy resins, waxes, fluosilicates, barium-hydroxide, limewater, etc. (e.g., Amoroso, 2002; Price and Doehne, 2011; Burgos-Cara et al., 2017; Sierra-Fernandez et al., 2017). Some of these consolidants also include biocide treatments to reduce the impact of micro-organisms (Fidanza and Caneva, 2019). Today there are numerous studies about the consolidant products used to protect stone (e.g., Miliani et al., 2007; Maravelaki-Kalaitzaki et al., 2008; Zárraga et al., 2010; Ferreira Pinto and Delgado Rodrigues, 2012; Slavíkova et al., 2012; Pinho and Mosquera, 2013; Bonazza et al., 2017; Delgado Rodrigues et al., 2018). However, most of these products are designed for use with silicate rocks and have proved less effective on carbonate stone. In recent years, they have begun to use consolidants based on calcium phosphates, such as dibasic ammonium phosphate, which reacts with the calcium carbonate in the stone causing the precipitation of different phosphate phases that help consolidate the carbonate rocks (Sassoni et al., 2011). Various recent studies have shown the beneficial effects of this form of consolidation against a range of different alteration factors (Matteini et al., 2011; Naidu et al., 2011; Sassoni et al., 2012; Sassoni et al., 2013; Naidu and Scherer, 2014; Franzoni et al., 2015a; Franzoni et al., 2015b; Naidu et al., 2015; Naidu et al., 2016; Graziani et al., 2016; Barriuso et al., 2017; Sassoni et al., 2020 and references therein; Murru and Fort, 2020). It has even been proposed that the gypsum-rich decay crust could be used as an alternative source of calcium for the consolidation process while at the same time helping remove the crust, so reducing its impact on the carbonate substrate (Snethlage et al., 2008; Molina et al., 2017; Sassoni et al., 2018).
This research has two fundamental objectives. Firstly, to assess which type of surface finish (saw-cut, honed and bush-hammered) has least impact on the durability of four varieties of natural stone currently used in the building industry (two calcarenites, a travertine and a sandstone) in an acid-polluted environment. By considering three surface finishes with different degrees of roughness, we were also seeking to determine whether there was any relationship between the roughness of the surface and its gradual decay. The second aim was to determine the degree of consolidation produced by the DAP in each type of rock and surface finish. To this end, four varieties of fresh (as received from the supplier), natural stone with different surface finishes were analyzed and characterized, together with similar samples that had been treated with DAP. Once they had been characterized, all the samples were subjected to an accelerated aging test with SO2 in the presence of moisture. The changes that took place were analyzed in relation to the surface finish, so as to identify decay patterns and assess the effectiveness of the consolidant treatment. The influence of the pore system and the changes to it were also assessed, as this is the route by which liquids, gases and even salts can move within the stone, so causing decay. Finally, all the data were statistically processed to ascertain whether the differences in the results obtained depending on the type of rock, the surface finish and/or the presence of consolidant could allow us to conclude that these were determining factors in the decay.
For this study four varieties of natural stone were selected, which are currently available on the market as building materials. Three of them have been quarried for centuries for use in the historic monuments in the cities of Granada, Ronda and Montoro (Andalusia, Spain):
- Calcarenita de Santa Pudia (SP). This is a bioclastic calcirudite-to-calcarenite from the Tortonian age which was quarried in Escuzar (Granada). It is very porous (it can reach up to 33% of porosity), with very little cement and with a clast size that varies from just a few micrometers to 2–5 mm (Molina et al., 2011). The most common finishes on sale are saw-cut and bush-hammered. This building material was used in many of the best-known monuments in the city of Granada, such as the Cathedral, the Palace of Charles V in the Alhambra, the Monastery of San Jerónimo and the Royal Chancellery.
- Travertino Amarillo (TA). This yellow travertine has a porosity of around 8% and a large abundance of macropores (centimetric size). It has a banded texture and a high degree of compactness, which allows any kind of surface finish to be applied (Molina et al., 2011). This carbonate rock is from Albox (Almeria) and dates from the Pliocene age. The research interest in this stone is due to the fact that it can be used as an alternative for the travertine from Alfacar (Granada), which was exhausted decades ago and was used in the construction of important historical buildings in Granada such as the Hospital Real (the seat of the rectorate of the University of Granada) and the Church of San Justo y Pastor.
- Arenisca Ronda (AR). This is a calcarenite from the Late Miocene, which has a pinkish color, a medium grain size and a porosity of around 17% (Molina et al., 2015). It is quarried in Ronda (Malaga). It does not acquire a shine when polished, and the main finishes on sale are saw-cut, honed and bush-hammered. It has been widely used in the city’s architecture, such as the New Bridge (Puente Nuevo), the Islamic walls and the Churches of Padre Jesús, Santa Catalina and Espíritu Santo.
- Molinaza Roja (MR). This is a reddish-colored arkose with a fine-medium grain size from the Buntsandstein age. It owes its color to the presence of iron oxides. It has a laminated texture with a preferential orientation of the clasts. It has a porosity of 15% and three types of cement can be identified, namely ferruginous, siliceous and calcitic (Molina et al., 2015). It is quarried in Montoro (Cordoba) and is commercialized with different finishes (saw-cut, honed, bush-hammered and aged). This stone has been used in the construction of various historical buildings in Montoro, such as for example the Donadas Bridge, most of the churches and the Town Hall (Clementson Lope, 2012).
According to the glossary on stone deterioration patterns (Vergès-Belmin, 2008), the main degradation phenomena affecting these sedimentary rocks can be divided into two types (Figure 1): firstly, the processes that take place in SP, AR and MR due to their clastic texture and structures, such as blistering, scaling, delamination, crumbling, sanding, alveolization, differential erosion, or black crust, among others related with human activities; and secondly the processes of crumbling, splintering, fragmentation, microkarst, pitting and black crust witnessed in TA, due to its crystalline texture. The photographs of SP and TA are of stones used in historic buildings (several centuries old), while the ashlars of AR and MR rocks are relatively modern (less than 30–40 years). These images illustrate that even though some of these ashlars are not very old, they can suffer from serious deterioration processes due to their exposure to the elements, and even damage caused by the capillary rise of fluids, as can be seen for example, in the facade made with AR. These examples highlight that building stone can be vulnerable to deterioration even in relatively recent constructions.
FIGURE 1. Photographs showing some of the main deterioration phenomena, such as sanding, peeling, scaling, blistering, flacking, differential erosion in Calcarenita de Santa Pudia (SP), Arenisca Ronda (AR) and Molinaza Roja (MR), and microkarst, pitting and black crust in Travertino Amarillo (TA).
Three surface finishes were selected for this study: “saw-cut (S),” which is obtained by direct sawing of the stone to produce a more or less flat surface with a rough feel; “honed (H),” which is obtained by applying abrasives with a progressively finer grain size, without reaching a polished finish; and “bush-hammered (BH),” a finish obtained by hitting the stone with hammers with steel teeth, so creating a rougher finish. These finishes were applied in the factories where the stones are processed and prepared for sale.
The samples were treated in a solution in distilled water with a concentration of 1 M of DAP. The treatment was applied to eight samples per surface finish and lithotype (96 samples in total) by placing the surface finish directly in contact with the solution so that the consolidation process could occur due to capillarity. This would enable us to identify any differences between the samples caused by the different finishes. This process lasted for 24 h at a temperature of 20°C and was performed in closed vessels so as to prevent the solution from evaporating. Once this process had been completed, any excess phosphate that had not reacted was removed by washing the samples with distilled water once a day for a further 3 days. Lastly, the samples were allowed to dry at a constant temperature of 20°C and were tested again a month later, so as to make sure that the reaction had completely finished.
The mineralogy of the rocks and of the new phases formed by the reaction between the DAP and the rocky substrate was characterized using X-ray diffraction (XRD). This was done with a PANanlytical X’Pert PRO diffractometer with the following measurement conditions: radiation CuKα (λ = 1.5405 Å), voltage 45 kV, current 40 mA, scanning angle 3–60° 2θ and goniometer speed 0.1 2θ s−1.
The textural changes were observed with a Quanta 400 environmental scanning electron microscope (ESEM), coupled with energy dispersive x-ray microanalysis (EDX).
The pore system is the main way in which fluids and decay agents circulate around the stone (Benavente et al., 2007). The external surface of the rock with its different possible finishes acts as an interface between the rock itself and the atmospheric conditions to which it is exposed. Mercury injection porosimetry was used to assess the changes in the pore system before and after consolidating the fresh samples. For this purpose, a Micromeritics Autopore III 9410 porosimeter was used, which can generate a pressure of 414 MPa and covers a pore diameter range from approximately 0.003–360 μm. The fragments of the treated samples had a base area of approximately 1 × 1 cm and were 0.5 cm thick. This thickness was chosen with a view to observing and recording the changes in the pore system near the surface of the rock, given the penetration capacity of the consolidant. Three Mercury injection porosimetry measurements were made per lithotype.
In addition, prismatic samples measuring 20 × 20 × 10 mm were used to measure the water vapor permeability according to the UNE-EN15803 (2010) standard, a test that was carried out on both untreated (UT) and treated (T) samples to determine the changes in their pore systems. The vapor permeability coefficient (KV, in kg/m×s×Pa) was obtained as follows:
where ΔM is the difference between the final and initial mass of the device used in the experiment (in kg), A is the surface area of the samples (in m2), Δt is the time (in s), Δpv is the difference in water vapor pressure across the sample (in Pa), and D is the thickness of the sample (in m). Δpv was calculated according to UNE-EN ISO 12572 (2002) as follows:
where φ is the relative humidity (0.35) and θ is the temperature (20°C).
The characterization was carried out using a Konica-Minolta CM-700d portable spectrophotometer equipped with a xenon lamp and diffuse reflectance geometry. The UNE-EN 15886 (2011) standard was followed. The CIELab system (1976) was used and the L* (lightness, from 0 to 100), a* and b* (chromatism, from −60 to +60) parameters were calculated using reflectance values. The measurement conditions were: measurement area of 8 mm, D65 standard illuminant and observer 10° with SCI mode and a wavelength range from 360 to 740 nm with a wavelength interval of 10 nm. Twenty measurements per stone were performed and the color difference (ΔE*) was calculated as follows (UNE-EN 15886, 2011):
To characterize the roughness, the differences between the four varieties of rock and their different finishes were recorded. For this task, a Leica VDM2000 videomicroscope was used and the data and images were processed using the Leica Application Suite v.3.8.0 and Leica Maps Start v.6 (Leica Microsystems©) software. The main geometric element of texture was the area and the following height parameters: Sa (mean arithmetic value of the absolute values of height within a specific area in mm), Sku (kurtosis value for the surface) and Sz (sum of the maximum peak height and the maximum trough depth within a defined area, in mm), as defined in the UNE-EN ISO 25178-2 (2013) and UNE-EN ISO 25178-3 (2013) standards.
To evaluate the degree of consolidation achieved in the samples on the basis of the different surface finishes, the resistance to penetration test was performed using micro-drilling (RD, in N). Fifteen perforations were made per finish and per lithotype before and after application of the consolidant. This was done with a DRMS Cordless drill (SINT Technology) equipped with a 5 mm diameter flat edge drill-bit with a diamond covered tip under the following working conditions: 600 rpm (revolution speed), 10 mm/min (penetration rate) and 10 mm (hole depth). Measurements were constantly controlled using a calibration standard (ARS, Sint Technology) so as to correct possible deviations due to wear on the bit, and when the value obtained with respect to the calibration standard was not acceptable, the worn bit was replaced with a new one. The results were divided by the diameter of the bit and expressed in N/mm (Pamplona et al., 2007).
This test assesses the resistance to decay of the four types of stone when exposed to the effects of SO2 in the presence of moisture and was performed in accordance with the UNE-EN 13919 (2003). The samples, measuring 20 × 20 × 10 mm, were placed in hermetically sealed vessels with a volume of 50 L. Two solutions were prepared. The first (Solution A) had a concentration of 500 ml of H2SO3 per 150 ml of distilled water while the second (Solution B) had a concentration of 150 ml of H2SO3 per 500 ml of distilled water. A small glass with a solution was placed in each vessel. The samples were then placed inside the vessels at a distance of 100 mm from the solution. In each vessel there were three untreated samples and three treated samples for each surface finish and lithotype so as to enable us to assess the possible differences between them under identical test conditions. A total of 72 samples were analyzed for each solution. The vessels were kept closed for 21 days at a constant temperature of 25°C. To evaluate the changes produced by the acid attack, the test was divided into three stages.
Stage 1) The fresh samples were weighed after spending 72 h at 40°C in a ventilated oven so as to ensure total elimination of any possible moisture retained inside them. The color was measured using spectrophotometry and the roughness was also measured.
Stage 2) After performing the aging test, the samples were dried in a ventilated oven at low temperature (30°C) for 120 h so as to remove any excess moisture. After that, the weight of the samples (rock plus alteration product) was measured again, as were the roughness of the surface and the color difference, comparing the values with those for fresh samples. At the same time, samples were taken of the crust that had formed so as to analyze its mineralogy using XRD.
Stage 3) The aged samples were desalinated by immersion in distilled water, which was changed every day. By measuring the conductivity of the water in which the samples had been desalinated, it was found that after 20 days all the surface crust had been removed. The samples were then analyzed again, measuring the total weight loss, the water vapor permeability, the color and the roughness. Small fragments were taken from the samples during Stages 2 and 3 for ESEM analysis.
All the data were subjected to analysis of variance (ANOVA) using SPSS statistical software (version 22.0). In particular, the vapor permeability coefficient, the micro-drilling resistance, the difference in the color of the samples after desalination and the change in the weight of the samples altered by acid attack (both with and without a gypsum crust) were subjected to ANOVA as dependent variables, while the type of natural stone, the surface finish, the application of consolidant and the SO2 aging test were used as independent variables.
To highlight the results of the analysis, only the level of significance was included, which quantifies whether a dependent variable varied in relation to the independent variables and the interactions between the variables (Supplementary Table S1, in Supplementary Material). The level of significance of the p-value was classified below 0.001, 0.01 and 0.05. No significant dependence (ns) was also shown and when the degree of freedom was zero, analysis of variance was not carried out.
XRD was used to identify the mineral phases that had precipitated on the fresh samples of rock after the dissolution of DAP 1 M. Hydroxyapatite (HAP) was identified in low concentration with respect to the calcite in SP, TA and AR or the quartz in MR (Figure 2). Octacalcium phosphate (OCP) was also detected in MR. This phase is considered a precursor of HAP (Dorozhkin, 2009) and its presence is probably due to the fact that MR has a lower concentration of Ca compared to the carbonate rocks. The presence of OCP has no negative impact on the protective properties of the consolidant and sooner or later (these reactions can take from hours to months) it will be transformed into HAP (Sassoni, 2018).
FIGURE 2. Diffractograms for the treated samples of Calcarenita de Santa Pudia (SP), Travertino Amarillo (TA), Arenisca Ronda (AR) and Molinaza Roja (MR). Legend: Cal, calcite; Qz, quartz; Fsp, feldspar; HAP, hydroxyapatite; OCP, octacalcium phosphate. The value of the 2θ angle is indicated on the X-axis.
The microtextural characterization using ESEM (Figure 3) showed that in SP and TA there were large areas in which the consolidant appeared as a film (Figures 3A,E) with variations in the morphology of the HAP crystals. For example, in SP, pseudo-spherical crystals were observed with a size of between 1 and 5 µm (Figure 3B), and plate-like crystals of less than 5 µm (Figure 3C); while in TA there were above all botryoidal/globular habits of approximately 5 µm (Figures 3F,G). In AR, the HAP was mainly associated with the clasts with micrite (Figure 3I-red arrows- and Figure 3J) or with a clay matrix (Figure 3K). The habit observed in these crystals was fundamentally plate-like. In MR, HAP was observed in greatest abundance in areas rich in carbonated cement or in the clayey matrix, and showed greater morphological variety, with crystals with needle-shaped (Figure 3M), massive (Figure 3N), and plate-like (Figure 3O) habits. In general, the different habits found (spherules, plate-like, needles) corresponded to HAP crystals (Naidu and Scherer, 2014; Possenti et al., 2016) and the average thickness of the HAP film was no more than 5 µm after identifying phosphorus (P) using EDX microanalysis at the various analysis points (Figures 3D,H,L and P), while the presence of other elements such as Si, Mg, Al and/or K was due to the proximity of areas rich in clayey cement.
FIGURE 3. ESEM microphotographs of the different habits of the HAP crystals in the samples of Calcarenita de Santa Pudia (SP, A–C), Yellow travertine (TA, E–G), Arenisca Ronda (AR, I–K) and Molinaza Roja (MR, M–O). The EDX microanalyses that corroborate the presence of HAP are shown in images (D, H, L, and P).
The pore system of each rock has certain intrinsic values that can be measured. These include the porosity (percentage of total volume occupied by pores), the pore size distribution (Figure 4A, black lines) and the degree of interconnection (Molina et al., 2011; Molina et al., 2015). In SP the pore system led to higher permeability values while TA by contrast was the least permeable (Figure 4B). AR and MR showed similar values to each other, falling between those obtained by the other two rock varieties (Figures 4A,B).
FIGURE 4. Pore size distribution curves (A) for untreated (black line) and treated specimens (red line), and (B) water vapor permeability values (KV, in Kg/m×s×Pa) for the samples exposed to Solution A with regard to each surface finish (S: saw-cut, H: honed and BH: bush-hammered) in Calcarenita de Santa Pudia (SP), Travertino Amarillo (TA), Arenisca Ronda (AR) and Molinaza Roja (MR). Legend: Po, open porosity (in %); a and a′, fresh samples and desalinated samples without HAP, respectively; A and A′, fresh samples and desalinated samples with HAP, respectively; the black, red and green bars show the KV value for the S, H and BH surface finishes, respectively.
In the pore size distribution of the treated samples (Figure 4A, black lines), a general trend could be observed in which there was an increase in the number of pores measuring between 1 and 2 μm, and a proportional fall in the pores of between 0.1 and 1 μm, and to a lesser extent in those of over 5 µm. The reduction observed in the latter groups was due to the precipitation of the consolidant in these pores, as previously observed by ESEM (Figure 3). This change in the pore size distribution is of particular interest in that the quantity of pores of between 0.1 and 1 µm can enhance or mitigate the damage produced by salt crystallization (Rodríguez-Navarro and Doehne, 1999), so affecting the durability of these lithotypes. A reduction in the number of pores in these size ranges, and in particular in the smallest pores, would therefore make these lithotypes more durable. After consolidation, the KV value (Figure 4B, A) fell slightly in all cases and no particular trends could be observed on the basis of the type of finish. The minimal fall noted in the water vapor permeability value was consistent with the values obtained by other authors working with similar rocks treated with DAP (Shekofteh et al., 2019; Sassoni et al., 2020).
Table 1 shows the mean lightness (L*) values and the chromatic parameters (a* and b*) for the different surface finishes in the treated (T) and untreated (UT) samples. In the untreated samples, the highest lightness values were observed in SP (L* = 86), due to the characteristic whitish tone of this variety of rock, while MR showed the lowest values (L* = 58). In general, the different stones had very homogeneous L* values, except for TA, the rock with the greatest dispersion from the mean. This dispersion is due to the heterogeneous texture of TA, a characteristic feature of many travertines (García-del-Cura et al., 2017). As regards chromaticity, the general trend is toward yellowish tones (positive b* values), a trend that is particularly notable in TA, with values of between 17 and 31.
TABLE 1. Spectrophotometry results for untreated (UT) and treated samples (T) of Calcarenita de Santa Pudia (SP), Travertino Amarillo (TA), Arenisca Ronda (AR) and Molinaza Roja (MR) with saw-cut (S), honed (H) and bush-hammered (BH) surface finishes. Legend: L*, lightness; a* and b*, chromatic parameters; ΔE*: color difference. The mean value (Φ) and its standard deviation (σ) and the maximum (Max) and minimum (min) values were obtained by taking 20 measurements for each surface finish. ΔE* values of 3 units or more are highlighted in bold type.
The chromatic parameters for the different surface finishes show that the honed (H) finish has the most intense color tones (higher a* and b* values) and darker hues (lower L* values). Given that the differences in color are very low, the values for the saw cut finish (S) were considered as the reference to calculate the difference in color between the different finishes (ΔE* columns UT, Table 1), as this finish does not require any additional processing. The results show that the bush-hammered finish (BH) is the one that most differs in color from S, although these differences were between 1 and 3 units, and could not therefore be perceived by the human eye (Berns, 2000; Völz, 2001; Benavente et al., 2003; Grossi et al., 2007). The only exception was in TA in which the ΔE* value was 15 units for the H finish and 10 units for the BH finish, which means that the differences can be observed with the naked eye. This means that with the exception of the travertine from Albox, the consolidant has no obvious visual impact, which is a positive finding when it comes to its possible application in the restoration of Architectural Heritage.
The roughness of the untreated (UT) and treated (T) samples was measured using the parameters Sa, Sku and Sz (Table 2). No important differences were observed between UT and T in this case either, which means that the application of this consolidant does not alter the roughness of the samples and it would seem to precipitate above all in the interior of the samples and less on the surface (Sa). The degree of heterogeneity in the roughness is therefore due exclusively to the textures of each variety of rock and is not affected by the consolidant.
TABLE 2. Mean roughness values for intact samples (Fresh) and for altered samples from which the gypsum crust has been removed (Desalinated), untreated (UT) and treated (T) in relation to each surface finish (saw-cut - S, honed - H and bush-hammered - BH) of Calcarenita de Santa Pudia (SP), Travertino Amarillo (TA), Arenisca Ronda (AR) and Molinaza Roja (MR). Legend: Sa, is the arithmetic mean value of the absolute values of the heights within a defined area (in mm); Sku, is the kurtosis value for the surface; Sz, is the sum of the maximum peak height and the maximum trough depth within a defined area (in mm).
The microdrilling test is a quick way to find out how well the product has been absorbed and how deep it has penetrated (Ferreira Pinto and Delgado Rodrigues, 2012). The fact that the consolidant was applied by capillarity enables us to directly assess the effects of the surface finish and of the pore system of each lithotype on the consolidation process. The results obtained for treated (T) and untreated (UT) samples can be seen in Figure 4.
Of the various untreated samples, SP obtained the lowest average RD values (RD ≈ 0.7 N/mm) because its clasts are poorly cemented (Molina et al., 2011). These values were also quite heterogeneous due to the perforation of different fossils. AR showed a slightly higher level of resistance (≈1.1 N/mm) because it is better cemented than SP (Molina et al., 2015). For their part, TA and MR obtained the highest values (RD ≈ 5.7 and 3.3 N/mm, respectively), although their results were quite heterogeneous. In the case of TA its high resistance was due to the compactness of the rock itself, while the heterogeneity could be due to the appearance of vuggy porosity. The resistance in MR was due to its mineralogy, above all the abrasive capacity of the quartz. Likewise, the heterogeneity of the values was due to the banded texture and to the mineralogical composition of the cement which varied from ferruginous to siliceous, carbonated or argillic.
The treated samples behaved in a similar way to the untreated ones but with slightly higher RD values. This indicates that consolidation took place independently of the mineralogy and the texture and was not affected by the roughness of the surface, because the consolidant could penetrate several millimeters. Apparently, this process was most effective from the surface down to a depth of 2 mm in SP and AR, while in TA and MR the consolidating effect penetrated more deeply (Figure 5), as can be observed from the fact that the curves for these two varieties of rock present a similar trend to the untreated ones, i.e., approximately parallel, but with slightly greater resistance in the treated samples due to the effect of the consolidant. This different trend between SP-AR and TA-MR may be due to a faster drying effect in the two calcarenites, which have a higher drying index, and to the anisotropic behavior exhibited by TA and MR due to their lamination (Molina et al., 2011; Molina et al., 2015). These characteristics enhance performance throughout the entire thickness of the samples rather than concentrating the consolidant effect in the first few millimeters near the surface. To enhance this process, some authors propose the use of ethanol, and/or other additives, which favor the precipitation and formation of HAP, simultaneously avoiding capillary suction toward the drying surface of the consolidant (e.g., Sassoni, 2017). These results suggest that capillarity is therefore a viable method of application for all these surface finishes although further research on the effects of the additives would be advisable.
FIGURE 5. Diagrams for resistance to drilling (RD) of fresh samples (Fresh) and of altered samples exposed to Solution A (Sol. A) untreated (black line) and treated (red line) for the four varieties of rock and the three different surface finishes (S-saw-cut, H-honed and BH-bush-hammered). The resistance values are represented on the y-axis (RD, in N/mm) and the depth of penetration (in mm) on the X-axis. Legend: SP, Calcarenita de Santa Pudia; TA, Travertino Amarillo; AR, Arenisca Ronda; MR, Molinaza Roja. The numbers inside the plots represent the average value of RD and their respective standard deviation (in brackets).
After the samples had been subjected to the aging test, various notable differences could be appreciated with the naked eye, depending on the type of rock and the type of solution applied (A and B), and to a lesser extent also due to the type of surface finish and to consolidation with DAP. Samples were tested with Solutions A and B according to the UNE standard recommendations and similar results were obtained for both solutions. We therefore decided only to present the results for Solution A, which was slightly more aggressive.
In hand samples, one of the main changes observed by the naked eye was the presence of a whitish powdery material in most of the samples (Supplementary Figure S1). This was later identified by XRD as gypsum, a result that might be expected given that gypsum is produced by the reaction between the SO2 and the calcite of which three of the rock varieties are formed (SP, TA and AR) and which is also present in the cement in MR. Specifically, SP did not develop a gypsum crust. In TA, gypsum crystals with more or less well-defined habits were formed in both the treated and untreated samples. These crystals were concentrated in particular regions of the surface, while in other areas a fine gypsum crust developed. In most of the AR samples, a significant gypsum crust appeared across the whole surface. In the treated samples, darker areas appeared within the gypsum crust, related with the development of organic matter, which also appeared in other lithotypes. In the untreated samples of MR, the gypsum crystals appeared in occasional aggregates across the whole surface, while in the treated samples these clusters were larger and more abundant. In the latter, it was possible to observe with the naked eye that the H finish had the highest proportion of gypsum aggregates followed by BH and S. Additionally, in the SP samples ochre/yellowish-colored areas appeared that had not been observed on the fresh samples, and in MR, these were also visible once the gypsum crust had been removed. These yellowish stains could be caused by dyes produced by the release of oxides. According to Haneef et al. (1992) these dyes appear due to the simultaneous release during the acid attack of the iron and manganese oxides contained in the carbonated and ferruginous cements and/or in the clayey matrix.
Figure 6 shows the changes in the weight of each sample. The weight gain due to the precipitation of the gypsum was slightly higher in the treated samples, but after removing the gypsum crust, a generalized weight loss was noted in all the samples. The textural characteristics of the rocks help them resist the decay process, irrespective of the different surface finishes, although in general the H finish obtained the poorest results, especially in AR and MR.
FIGURE 6. Difference in weight (ΔM/M, in %) of the four varieties of rock depending on the saw-cut (S), honed (H) and bush-hammered (BH) finishes exposed to Solution A of the non-consolidated (a and a′) and consolidated (A and A′) samples. Legend: SP, Calcarenita de Santa Pudia; TA, Travertino Amarillo; AR, Arenisca Ronda; MR, Molinaza Roja; a and a′, Difference in weight between the altered samples before and after removal of the gypsum crust compared to the fresh samples, respectively; A and A′, Difference in weight between the altered samples before and after removal of the gypsum crust compared to the fresh and consolidated samples, respectively.
The results of the textural study performed with ESEM on the samples exposed to Solution A without and with HAP (Supplementary Figures S2 and S3, respectively) were similar to those for the samples exposed to Solution B, albeit more intense. Although to the naked eye, the only changes observed in the altered samples of SP were the yellow-stained areas on the surface, ESEM revealed that large gypsum crystals with a prismatic habit had developed (as confirmed by EDX analysis) above all in the intraclastic pores (Supplementary Figures S2-a and S3-a). Some of these gypsum crystals penetrated between the calcite crystals (Supplementary Figure S3-b), so giving rise to incipient intraclastic fissuring. In TA the gypsum crust was more abundant than in SP (Supplementary Figures S2-c and S3-f−g). In AR a large quantity of gypsum crystals precipitated with an acicular habit, forming flower-like structures (Supplementary Figure S2-e and Supplementary Figure S3-k−l) and fissures began to appear. These were above all intraclastic fissures, as happened in SP. In both rocks there were small chambers of fossils which led to over-saturation and the precipitation of gypsum crystals. Lastly, gypsum crystal aggregates developed in MR with an acicular habit, forming flower-like structures. In the untreated samples these crystals were equidimensional (Supplementary Figure S2-g), while in the treated samples growth was more heterogeneous in that some of the crystals reached a size of approximately 500 µm long and 80 µm wide (Supplementary Figure S3-p−q).
After removing the gypsum crust, a slight sanding of the clasts could be observed in the untreated samples of SP (Supplementary Figure S2-b), while in the treated samples it was also possible to observe that organic matter had developed on the surface (Supplementary Figure S3-c, darker-colored areas), clasts had been lost due to sanding (Supplementary Figure S3-d) and in some areas the film of HAP had come off (Supplementary Figure S3-e). These forms of damage were similar to those described by Cardell et al. (2008) and were caused above all by the crystallization of gypsum. In TA, dissolution of the calcite crystals was observed giving rise to a V-in-V etching morphology (Supplementary Figures S2-d and S3-i, respectively). In some areas there was superficial scaling of the HAP film (Supplementary Figure S3-h), while in other areas it remained intact (Supplementary Figure S3-j). The alteration observed in AR was a cross between that observed in SP and TA, in that there was slight sanding and dissolution of the calcite in etched cleavage and V-in-V etching (Supplementary Figure S2-f), while in the treated samples the most obvious change was the fissuring in the film of HAP (Supplementary Figure S3-m−n). Given that these forms of dissolution of the calcite appeared in both untreated and treated samples (Supplementary Figure S3-o), they must have been produced by the acid attack and it is impossible to establish whether or not the consolidant enhanced or mitigated the dissolution process in any way. Crumbling was also evident in MR due to the loss of interclastic material, the appearance of small fissures associated with the splitting of the grains, and a slight increase in the spacing between the laminas of the phyllosilicates, the presence of organic matter (Supplementary Figure S2-h), corroded and decayed potassium feldspar crystals (Supplementary Figure S3-r) with an incipient process of argillization (Supplementary Figure S3-s). In the areas with a high presence of clayey matrix, the loss of material was more evident in the treated samples than in the untreated ones (Supplementary Figure S3-s−t).
The different forms and processes of decay observed in the four types of rock show that these are strongly influenced by the texture and mineralogy of each rock, and to a lesser extent by the presence of consolidant. In fact, various authors have suggested that the forms of decay, the habits of the gypsum crystals and the development of the gypsum crust depend on the intrinsic (crystal size, mineralogical composition, pore system, surface finish) and extrinsic (degree of relative humidity, atmospheric composition, temperature, etc.) properties of the rock (Elfving et al., 1994a; Malaga-Starzec et al., 2003; Charola et al., 2007; Thornbush and Viles, 2007; Urosevic et al., 2013). In particular, the clast/crystal size could be an important factor in the different alteration processes observed in the four types of rock studied (Gauri et al., 1982): in the rocks with a smaller grain size such as TA, AR and especially MR, more gypsum appeared, something that seemed to occur regardless of the type of rock, given that in MR much smaller amounts of calcite were available compared to any of the other three types. According to Müller (2008), under exposure to acid conditions calcium may also be released by feldspars and clays, phases of which are present in MR, so favoring the precipitation of gypsum. It has also been shown that when the solution contains iron oxides, these facilitate the absorption of the SO2 and act as catalysts (Charola and Ware, 2002), so giving rise to calcium sulfate (Elfving et al., 1994b). This catalyst effect could explain, at least in part, the differences between SP and MR with regard to the amount of gypsum. Lastly, it was also observed that the treated samples suffered partial loss of the film of consolidant, especially in SP, TA and AR, due to the crystallization of the gypsum. This suggests that the decay affects the consolidant rather than the rocky substrate, and that the consolidant is therefore an effective way of protecting the natural stone, although further applications may be required over the course of time.
The water vapor permeability was measured again in order to assess the influence of the surface finish and the changes that had taken place in the pore system of the samples after exposure to SO2 and after being desalinated so as to remove the gypsum crust (Figures 4B, a′ and A′).
In spite of the reduction in the KV value due to application of the consolidant, after aging with Solution A no significant changes were observed in the KV value in any group of samples, although the samples treated after removal of the gypsum crust showed a slightly higher increase in KV compared to the untreated samples.
In SP, TA and AR the BH finish was the most permeable (green bars, Figure 4B), while in MR it was the H finish (red bars, Figure 4B), although in general the KV values for each rock were very similar whatever the finish. This indicates that water vapor permeability was only very minimally affected by roughness, and that the pore system of each variety of rock was a much more decisive factor.
The color difference (ΔE*) in the samples exposed to acid attack is shown in Figure 7. In most cases, the tendency in the ΔE* values in the altered samples (columns a and A) was maintained after they were desalinated (columns a' and A′). The changes were due to the presence of gypsum, increasing lightness (L*) and a reduction in the chromatic parameters (a* and b*). In any case, the changes that took place were almost always below the threshold perceivable by the human eye (3 units). After washing to remove the gypsum crust, AR was the only rock in which there were no important changes, except in the S finish in which the ΔE* value was closer to the threshold (black bar, A′). This change was due above all to an increase in the b* value, in other words there was a yellowing of the sample, due to staining by the oxides present in the sample. In SP, the S and H finishes also presented the same trend, with an increase in b*. This increase in the b* value due to acid attack on limestone was also described by Grossi et al. (2007), although according to these authors its implications on the esthetics of the rock were almost unnoticeable. Vázquez et al. (2016) reported very similar behavior to that observed in this study, finding that the total color difference (ΔE*) was also unappreciable. For their part, Urosevic et al. (2010) and Benavente et al. (2003) observed that the polished finish underwent more changes than the rough finish (the H finish in this study), showing an increase in lightness and a fall in the chromatic parameters. They also indicated that these changes were related not only with variations in roughness but also with chemical reactions. These changes were observed above all in TA and in the H finish in MR.
FIGURE 7. Measurements of the color difference (ΔE*) for each surface finish (S: saw-cut, H: honed and BH: bush-hammered) of the four varieties of rock from Solution A without HAP (a and a′) and with HAP (A and A′). Legend: Calcarenita de Santa Pudia (SP); Travertino Amarillo (TA); Arenisca Ronda (AR); Molinaza Roja (MR); a and a′, Difference between the altered samples before and after removal of the gypsum crust compared to the fresh and untreated samples, respectively; A and A′, Difference between the samples before and after removal of the gypsum crust compared to the fresh and treated samples (A and A′, respectively). The broken line marks the limit for appreciable color difference ΔE* = 3.
The difference in roughness between the fresh samples and the samples that had been desalinated after the SO2 aging test are presented in Table 2 and Figure 8. The most important variations in SP and TA were due to the deepening of pre-existing trough areas due to the dissolution of the calcite. In AR and MR, which have smaller and more homogenously-sized clasts than SP and TA, the changes in roughness were associated with a sanding process. In AR, this process occurs at random points, while in MR the decay on the surface occurs parallel to the lamination, so causing the material to flake off in layers (Table 2, Desalinated). When these roughness values are compared with those for fresh samples (Table 2, Fresh), a slight increase can be observed in all samples (Sa). There is also an increase in the difference between the deepest trough and the highest peak (Sz). This is due to the dissolution of the calcite and the sanding of the samples as mentioned earlier. As regards the Sku value, it is interesting to note that it fell in SP and TA, which only suffered alterations by dissolution, while in the AR and MR rocks, which were also affected by sanding, the kurtosis value increased. No important differences could be observed between the treated and untreated samples. In spite of this, it is important to bear in mind that the aging test was performed just once over a period of 21 consecutive days, which means that we should be cautious about reaching the conclusion that perhaps the treated samples would behave better over a longer period of time, given that hydroxyapatite is less soluble than calcite when subjected to acid attack (Dorozhkin, 2011).
FIGURE 8. Most representative topographic maps of roughness in 3D (bush-hammered finish, BH) in Calcarenita de Santa Pudia (SP), Travertino Amarillo (TA), Arenisca Ronda (AR) and Molinaza Roja (MR). The difference shown here (in mm or in µm) is between fresh samples (Fresh) and altered samples after removing the gypsum crust (Altered).
If the honed finish (H, with the lowest roughness) is likened to the most intact surface and the saw-cut (S, intermediate roughness) and bush-hammered (BH, greater roughness) finishes to the most deteriorated surfaces, these results were unable to establish a clear relationship between surface finishes and the damage that had taken place, which indicated that increasing roughness did not lead to higher levels of decay. In fact, the honed finish behaved worse in terms of change in weight (Figure 5). In spite of this, it would seem that the samples with honed and bush-hammered finishes underwent greater variation in their roughness levels than the saw-cut samples, which indicates that the mechanical treatment applied to the rock in the factory to produce both these finishes could affect the durability of the rock.
In the untreated samples (Supplementary Figure S4), no relation could be observed between roughness and color change, although it is worth highlighting the inverse relationship between L* and C*, in that L* values increase while C* values fall. However, if the difference in roughness (Sa) is compared with the color difference (ΔE*), the influence of roughness in color change appears to be minimal, or at least not the main factor. This is important to note in TA, where the difference in roughness is practically identical for all the finishes and the important color change is linked to changes in the chromophore minerals rather than to roughness. In the other rocks, the difference in roughness does not appear to affect the differences in color. In the treated samples (Supplementary Figure S5), the L* values show that TA is the only rock in which there is a direct relationship between increases in roughness and lightness, while MR displays an inverse tendency. As regards the C* values, in all the lithotypes there was an inverse relationship between C* and L* values, as occurred in the untreated samples. If we compare the last column in Supplementary Figures S4 and S5, certain differences can be observed compared to the untreated samples, in which there does seem to be a certain relationship between roughness and color change, as can be seen in MR and in particular in TA. However, this possible relationship could be due to the presence of the consolidant itself rather than to changes in roughness.
The results of the drilling test (RD) for the altered samples without gypsum are shown in Figure 5 (“Sol. A″). In general terms, the samples treated with HAP were more resistant. This increased resistance indicates that although the rocks suffered some damage, the consolidant did have a positive impact by increasing their resistance, so minimizing at least in part the damage to the samples. More specifically, in SP and AR the values for treated and untreated samples were quite similar and there was little difference between them, although this was not the case in TA and MR in which greater differences could be observed. In these two types of rock in addition to the consolidant effect produced by HAP, textural differences could also be observed, due to the presence of pores or of a clayey matrix and/or cement. As in other cases, no noteworthy differences were observed in terms of surface finish in this test.
The results obtained in the different tests on the fresh, treated and untreated samples, and on those altered by SO2 aging did not show any significant differences that could establish a clear tendency or pattern as regards the resistance of these materials with respect to their surface finish. For this reason, it was decided to check the data by performing an ANOVA analysis (Supplementary Table S1), so as to ascertain whether in statistical terms there was any variable that was more significant than others, considering for this purpose the variety of natural rock (N), the surface finish (F), the consolidant treatment (C) and the aging test by acid attack (D). The results obtained in the water vapor permeability test (KV), the resistance to drilling test (RD), the variation in weight (ΔW) in samples with and without the gypsum crust and the color difference values (ΔE) were also considered.
For the permeability test (KV), the type of rock (N) and the aging test (D) were significantly determinant factors (p < 0.001), while the surface finish (F) and the application of consolidant (C) were not. If two independent variables are compared, the combinations “N*F” and “F*C” did not influence the permeability results. Quite the opposite is true if the lithotypes and the application of the consolidant “N*C”, or the combination “N*D”, are considered, while the combination “C*D” is slightly significant. Combinations of three of the four variables were also considered, although these did not produce any significant values except for the combination “N*C*D”, as the F and C variables were not significant when analyzed separately. Lastly, when all four variables were analyzed in combination they were not found to be different in any statistically significant way. This means that the pore system of each lithotype is the factor that determines the weight of the variable N with respect to its hydric behavior This confirms that the pore system is the decisive factor in the durability of the four types of rock (Molina et al., 2011; Molina et al., 2015).
The importance of rock type (N) compared to other variables is also evident when analyzing the change in weight of the altered samples with the gypsum crust (“ΔW (%) Altered samples”) and the altered samples without the gypsum crust (“ΔW (%) Desalinated samples”). Nonetheless, for the variation in weight factor (ΔW), the variable F is of relative importance depending on whether the samples have been treated or not. This is due to the fact that the H finish showed the worst results, which indicates that this surface finish is perhaps less recommendable in polluted environments.
When considering the possible use of consolidants in the restoration of monuments, the importance of changes in color and whether or not these are appreciable to the naked eye must bear in mind. In any case these changes may be due to a wide range of factors such as for example the precipitation of secondary phases, patinas of oxides, microbe colonization or even changes in roughness (Benavente et al., 2003). It is therefore vital to objectively evaluate the importance of color change given its possible visual impact on buildings. To this end, and in spite of the fact that the ΔE* value was less than three units, it was confirmed that N and C were the most significant variables in terms of color difference, while the type of surface finish was irrelevant in terms of the resistance of the rock when exposed to an acidic atmosphere. This means that the type of surface finish has no influence on the visual impact of the decay suffered by any of these lithotypes including those treated with DAP. It is also important to point out that the ANOVA analysis of color difference was only performed on the altered samples without a crust, as in the samples with a crust the color difference was due to an increase in the L* value caused by the crust.
One last objective was to evaluate the effect of the consolidant on these four varieties of natural rock. Once again, the variables N and C proved to be determinant in the results obtained, while F and D were not. These results show that the degree of consolidation depends above all on the variety of rock and that in general the consolidant was effective in protecting the rock. In the same way, when combinations of two or three variables were analyzed, the positive effects of the consolidant were corroborated statistically, as was the important influence of the lithotype.
In this research, the durability of two calcarenites, one travertine and one sandstone with three different surface finishes (saw-cut, honed and bush-hammered) were evaluated, considering that are widely used in heritage buildings in Andalusia and also in new constructions. The efficacy of a consolidant based on DAP has also been demonstrated by analyzing the changes that took place in the different petrophysical properties in treated and untreated samples that had been exposed to an accelerated aging test by SO2 attack. On the basis of this comparative study of fresh and altered samples with and without consolidant the following conclusions were reached:
- The use of the consolidant based on DAP to generate hydroxyapatite under the conditions applied in this research led to an improvement in the behavior of the rock when exposed to acid attack, as compared to the samples that had not been treated. Application of the consolidant led to a reduction in water vapor permeability, but it did not significantly alter the pore system, nor did it affect surface roughness. However, a slight variation in the color of the treated samples was observed with an increase in lightness L*, although this change was not appreciable to the naked eye.
- The study of the samples exposed to the SO2 aging test in the presence of moisture revealed that the product that developed on the surface of the rock due to alteration was gypsum in the form of isolated or aggregated crystals and sometimes crusts. This varied depending on the type of rock, but was not affected by the surface finish. At a microscopic level, all the samples suffered damage, such as incipient interclastic fissuring, the loss of cement material, dissolution of the calcite and decay due to sanding. These processes occurred in both the treated and untreated samples.
- There is no clear relation between color difference and the roughness of the surface. The most plausible explanation for color differences between the samples appears to be the presence of areas with yellowish stains due to the release of oxides.
- The increase in drilling resistance confirmed that HAP had had a positive effect. However, the method of application by capillarity may be not recommended due to the rapid drying of the sample, which can concentrate the consolidant in the part closest to the surface, so preventing deeper consolidating action. Consequently, the application of the consolidant with additives that encourage deeper action or better control of the drying conditions of the treated samples should be considered.
- As regards the possible influence of different surface finishes, in the fresh samples the behavior of the petrophysical properties identified was unaffected by the type of finish. After SO2 attack, however, the aged samples with the honed finish (H) provided the worst results, as in most cases more gypsum precipitated, causing greater loss of material, changes in color, and alterations in the permeability levels. At the other end of the scale, the finish that performed best was the saw-cut finish (S), although this achieved quite similar results to the bush-hammered finish (BH). As a result, application of the honed finish should not be recommended if the rocks are to be exposed to acid-polluted atmospheres. Likewise, the mechanical process used to create the BH and H finishes could have resulted in lower levels of resistance compared to the S finish.
- The ANOVA analysis revealed that the most significant variables in terms of the durability of the rocks studied were the lithotype (considering the mineralogy, texture and especially the pore system) and the use of the consolidant, while the surface finish and the aging test had less statistical influence on the durability responses of the natural stones.
All datasets presented in this study are included in the article/Supplementary Material.
EM, DB, and GC contributed to the conception and design of this study. EM and AA carried out the laboratory tests. DB performed the statistical analysis. EM wrote the first draft of the manuscript. AA, DB, and GC contributed to the discussion of the results and collaborated in the writing of the different sections of the manuscript. All authors contributed to the reading and review of the manuscript, and approved the submitted version.
Financial support for this work was provided by Research Group RNM179 of the Junta de Andalucía and Research Project MAT2016-75889-R.
The authors declare that the research was conducted in the absence of any commercial or financial relationships that could be construed as a potential conflict of interest.
We are grateful to Nigel Walkington for his assistance in translating the original text. We would like to thank the companies Los Linos, S.L.; Travertino Amarillo Oro, S.L.; Talleres José Madueño Ruíz, S.L., and Mármoles Aguilera, S.L. for providing the materials. We would also like to thank Prof. María Jesús Mosquera (Universidad de Cadiz) for allowing us to carry out the microdrilling test in her laboratory.
The Supplementary Material for this article can be found online at: https://www.frontiersin.org/articles/10.3389/fmats.2020.581979/full#supplementary-material
Amoroso, G. G. (2002). Treatise on the science of conservation of monuments: ethics of conservation, degradation of monuments, conservation, consolidating and protective interventions. Florence, Italy: ALINEA Editrice [in Italian, with English summary].
Barriuso, B. C., Botticelli, G., Cuzman, O. A., Osticioli, I., Tiano, P., and Matteini, M. (2017). Conservation of calcareous stone monuments: screening different diammonium phosphate based formulations for countering phototrophic colonization. J. Cult. Herit. 27, 97–106.
Behlen, A., Steiger, M., and Dannecker, W. (2008). Deposition of sulfur dioxide to building stones: the influence of the ambient concentration on the deposition velocity. Environ. Geol. 56, 595–603. doi:10.1007/s00254-008-1414-x
Benavente, D., Cueto, N., Martínez-Martínez, J., García-del-Cura, M. A., and Cañaveras, J. C. (2007). The influence of petrophysical properties on the salt weathering of porous building rocks. Environ. Geol. 52, 197–206. doi:10.1007/s00254-006-0475-y
Benavente, D., Martínez-Verdú, F., Bernabeu, A., Viqueira, V., Fort, R., García del Cura, M. A., et al. (2003). Influence of surface roughness on color changes in building stones. Color Res. Appl. 28, 343–351. doi:10.1002/col.10178
Bonazza, A., Vidorni, G., Natali, I., Ciantelli, C., Giosuè, C., and Tittarelli, F. (2017). Durability assessment to environmental impact of nano-structured consolidants on Carrara marble by field exposure tests. Sci. Total Environ. 575, 23–32. doi:10.1016/j.scitotenv.2016.10.004
Brimblecombe, P., and Grossi, C. M. (2004). “The rate of darkening of material surfaces,” in Air pollution and cultural Heritage. Editors Saiz, C. (Leiden, Netherlands: Balkema Publishers), 193–198.
Burgos-Cara, A., Ruiz-Agudo, E., and Rodríguez-Navarro, C. (2017). Effectiveness of oxalic acid treatments for the protection of marble surfaces. Mater. Des. 115, 82–92. doi:10.1016/j.matdes.2016.11.037
Cardell, C., Benavente, D., and Rodríguez-Gordillo, J. (2008). Weathering of limestone building material by mixed sulfate solutions. Characterization of stone microstructure, reaction products and decay forms. Mater. Char. 59, 1371–1385. doi:10.1016/j.matchar.2007.12.003
Charola, A. E., Pühringer, J., and Steiger, M. (2007). Gypsum: a review of its role in the deterioration of building materials. Environ Geol. 52, 339–352. doi:10.1007/s00254-006-0566-9
Charola, A. E., and Ware, R. (2002). “Acid deposition and the deterioration of stone: a brief review of a broad topic,” in Natural stone, weathering phenomena, conservation strategies and case studies. Editors Siegesmund, S., Weiss, T., and Vollbrecht, A. (London, UK: Geological Society, London, Special Publications) 205, 393–406.
Clementson Lope, J. A. (2012). Characterization of the properties of the Montoro sandstone, its alterations and its applicability for construction. PhD Thesis. Cordova, Spain: University of Cordoba. [in Spanish, with English summary].
Cultrone, G., de la Torre, M. J., Sebastián, E. M., Cazalla, O., and Rodriguez-Navarro, C. (2000). Behavior of brick samples in aggressive environments. Water, Air, Soil Pollut. 119, 191–207. doi:10.1023/a:1005142612180
De Kock, T., Turmel, A., Fronteau, G., and Cnudde, V. (2017). Rock fabric heterogeneity and its influence on the petrophysical properties of a building limestone: lede stone (Belgium) as an example. Eng. Geol. 216, 31–41. doi:10.1016/j.enggeo.2016.11.007
Delgado Rodrigues, J. (2007). “Bulk and surface properties in stone conservation,” in Preservation of natural stone and rock weathering. Editors Sola, P., Estaire, J., and Olalla, C. (London, UK: Taylor & Francis), 13–16.
Delgado Rodrigues, J., Ferreira Pinto, A. P., Nogueira, R., and Gomes, A. (2018). Consolidation of lime mortars with ethyl silicate, nanolime and barium hydroxide. Effectiveness assessment with microdrilling data. J. Cult. Herit. 29, 43–53. doi:10.1016/j.culher.2017.07.006
Dorozhkin, S. (2009). Calcium orthophosphates in nature, biology and medicine. Materials 2, 399–498. doi:10.3390/ma2020399
Dorozhkin, S. V. (2011). Calcium orthophosphates: occurrence, properties, biomineralization, pathological calcification and biomimetic applications. Biomatter 1, 121–164. doi:10.4161/biom.18790
Elfving, P., Panas, I., and Lindqvist, O. (1994a). Model study of the first steps in the deterioration of calcareous stone I. Initial surface sulphite formation on calcite. Appl. Surf. Sci. 74, 91–98. doi:10.1016/0169-4332(94)90103-1
Elfving, P., Panas, I., and Lindqvist, O. (1994b). Model study of the first steps in the deterioration of calcareous stone III. Manganese and iron mediated sulphation of natural stone. Appl. Surf. Sci. 78, 373–384. doi:10.1016/0169-4332(94)90062-0
Fatah, N., Boumezbeur, A., and Benavente, D. (2018). Influence of the petrophysical and durability properties of carbonate rocks on the deterioration of historic constructions in Tebessa (northeastern Algeria). Bull. Eng. Geol. Environ. 78, 3969–3981. doi:10.1007/s10064-018-1410-7
Ferreira Pinto, A. P., and Delgado Rodrigues, J. (2012). Consolidation of carbonate stones: influence of treatment procedures on the strengthening action of consolidants. J. Cult. Herit. 13, 154–166. doi:10.1016/j.culher.2011.07.003
Fidanza, M. R., and Caneva, G. (2019). Natural biocides for the conservation of stone cultural heritage: a review. J. Cult. Herit. 38, 271–286. doi:10.1016/j.culher.2019.01.005
Fort, R., Alvarez de Buergo, M., and Perez-Monserrat, E. M. (2013). Non-destructive testing for the assessment of granite decay in heritage structures compared to quarry Stone. Int. J. Rock Mech. Min. Sci. 61, 296–305. doi:10.1016/j.ijrmms.2012.12.048
Franzoni, E., Graziani, G., and Sassoni, E. (2015b). TEOS-based treatments for stone consolidation: acceleration of hydrolysis-condensation reactions by poulticing. J. Sol. Gel Sci. Technol. 74, 398–405. doi:10.1007/s10971-014-3610-3
Franzoni, E., Sassoni, E., and Graziani, G. (2015a). Brushing, poultice or immersion? The role of the application technique on the performance of a novel hydroxyapatite-based consolidating treatment for limestone. J. Cult. Herit. 16, 173–184. doi:10.1016/j.culher.2014.05.009
García-del-Cura, M. Á., Benavente, D., Martínez-Martínez, J., and Ordóñez, S. (2017). Travertinos coloreados en la Cordillera Bética (SE de la Península Ibérica). Situación geológica y características petrofísicas. B. Geol. Min. 128, 467–483. doi:10.21701/bolgeomin.128.2.013
Gauri, K. l., Popli, R., and Sarma, A. C. (1982). Effect of relative humidity and grain size on the reaction rates of marble at high concentrations of SO2. Durab. Build. Mater. 1, 209–216.
Graziani, G., Sassoni, E., Franzoni, E., and Scherer, G. W. (2016). Hydroxyapatite coatings for marble protection: optimization of calcite covering and acid resistance. Appl. Surf. Sci. 368, 241–257. doi:10.1016/j.apsusc.2016.01.202
Grossi, C. M., Brimblecombe, P., Esbert, R. M., and Alonso, F. J. (2007). Color changes in architectural limestones from pollution and cleaning. Color Res. Appl. 32, 320–331. doi:10.1002/col.20322
Grossi, C. M., Esbert, R. M., Dı́az-Pache, F., and Alonso, F. J. (2003). Soiling of building stones in urban environments. Build. Environ. 38, 147–159. doi:10.1016/s0360-1323(02)00017-3
Hamilton, R., Kucera, V., Tidblad, J., and Watt, J. (2009). The effects of air pollution on cultural heritage. Berlin, Germany: Springer, 308.
Haneef, S. J., Johnson, J. B., Dickinson, C., Thompson, G. E., and Wood, G. C. (1992). Effect of dry deposition of NOx and SO2 gaseous pollutants on the degradation of calcareous building stones. Atmos. Environ. Part A. General Topics. 26, 2963–2974. doi:10.1016/0960-1686(92)90288-v
Hatir, M. E. (2020). Determining the weathering classification of stone cultural heritage via the analytic hierarchy process and fuzzy inference system. J. Cult. Herit. 44, 120–134. doi:10.1016/j.culher.2020.02.011
La Russa, M. F., Fermo, P., Comite, V., Belfiore, C. M., Barca, D., Cerioni, A., et al. (2017). The Oceanus statue of the Fontana di Trevi (Rome): the analysis of black crust as a tool to investigate the urban air pollution and its impact on the stone degradation. Sci. Total Environ. 593-594, 297–309. doi:10.1016/j.scitotenv.2017.03.185
La Russa, M. F., Ruffolo, S. A., Belfiore, C. M., Aloise, P., Randazzo, L., Rovella, N., et al. (2013). Study of the effects of salt crystallization on degradation of limestone rocks. Period. Mineral. 82, 113–127. doi:10.2451/2013PM0007
Müller, U. (2008). The mineralogical composition of sandstone and its effect on sulphur dioxide deposition. Mater. Construcción 58, 81–95. doi:10.3989/mc.2008.v58.i289-290.86
Malaga-Starzec, K., Panas, I., Lindqvist, J. E., and Lindqvist, O. (2003). Efflorescence on thin sections of calcareous stones. J. Cult. Herit. 4, 313–318. doi:10.1016/j.culher.2003.09.002
Maravelaki-Kalaitzaki, P., Kallithrakas-Kontos, N., Agioutantis, Z., Maurigiannakis, S., and Korakaki, D. (2008). A comparative study of porous limestones treated with silicon-based strengthening agents. Prog. Org. Coating. 62, 49–60. doi:10.1016/j.porgcoat.2007.09.020
Martinez-Martinez, J., Benavente, D., Pérez-Huerta, A., Cueto, N., and García-del-Cura, M. A. (2019). Changes on the surface properties of foliated marbles at different cutting orientations. Construct. Build. Mater. 222, 493–499. doi:10.1016/j.conbuildmat.2019.06.099
Matteini, M., Rescic, S., Fratini, F., and Botticelli, G. (2011). Ammonium phosphates as consolidating agents for carbonatic stone materials used in architecture and cultural heritage: preliminary research. Int. J. Architect. Herit. 5, 717–736. doi:10.1080/15583058.2010.495445
McKinley, J. M., Curran, J. M., and Turkington, A. V. (2001). Gypsum formation in non-calcareous building sandstone: a case study of Scrabo sandstone. Earth Surf. Process. Landforms. 26, 869–875. doi:10.1002/esp.232
Miliani, C., Velo-Simpson, M. L., and Scherer, G. W. (2007). Particle-modified consolidants: a study on the effect of particles on sol-gel properties and consolidation effectiveness. J. Cult. Herit. 8, 1–6. doi:10.1016/j.culher.2006.10.002
Molina, E., Benavente, D., Sebastian, E., and Cultrone, G. (2015). The influence of rock fabric in the durability of two sandstones used in the Andalusian Architectural Heritage (Montoro and Ronda, Spain). Eng. Geol. 197, 67–81. doi:10.1016/j.enggeo.2015.08.009
Molina, E., Cultrone, G., Sebastian, E., and Alonso, F. J. (2013). Evaluation of stone durability using a combination of ultrasound, mechanical and accelerated aging tests. J. Geophys. Eng. 10, 035003. doi:10.1088/1742-2132/10/3/035003
Molina, E., Cultrone, G., Sebastian, E., Alonso, F. J., Carrizo, L., Gisbert, J., et al. (2011). The pore system of sedimentary rocks as a key factor in the durability of building materials. Eng. Geol. 118, 110–121. doi:10.1016/j.enggeo.2011.01.008
Molina, E., Rueda-Quero, L., Benavente, D., Burgos-Cara, A., Ruiz-Agudo, E., and Cultrone, G. (2017). Gypsum crust as a source of calcium for the consolidation of carbonate stones using a calcium phosphate-based consolidant. Construct. Build. Mater. 143, 298–311. doi:10.1016/j.conbuildmat.2017.03.155
Moses, W. W., Robinson, D., and Barlow, J. (2014). Methods for measuring rock surface weathering and erosion: a critical review. Earth Sci. Rev. 135, 141–148. doi:10.1016/j.earscirev.2014.04.006
Murru, A., and Fort, R. (2020). Diammonium hydrogen phosphate (DAP) as a consolidant in carbonate stones: impact of application methods on effectiveness. J. Cult. Herit. 42, 45–55. doi:10.1016/j.culher.2019.09.003
Naidu, S., Blair, J., and Scherer, G. W. (2016). Acid-resistant coatings on marble. J. Am. Ceram. Soc. 99, 3421–3428. doi:10.1111/jace.14355
Naidu, S., Liu, C., and Scherer, G. W. (2015). Hydroxyapatite-based consolidant and the acceleration of hydrolysis of silicate-based consolidants. J. Cult. Herit. 16, 94–101. doi:10.1016/j.culher.2014.01.001
Naidu, S., Sassoni, E., and Scherer, G. W. (2011). “New treatment for corrosion-resistant coatings for marble and consolidation of limestone,” in Jardins de Pierres—conservation of stone in parks, gardens and cemeteries, Paris. Editors Stefanaggi, M., and Vergès-Belmin, V. 289–294. ISBN: 2-905430-17-6.
Naidu, S., and Scherer, G. W. (2014). Nucleation, growth and evolution of calcium phosphate films on calcite. J. Colloid Interface Sci. 435, 128–137. doi:10.1016/j.jcis.2014.08.018
Pamplona, M., Kocher, M., Snethlage, R., and Aires Barros, L. A. (2007). Drilling resistance: overview and outlook. Z. dt. Ges. Geowiss. 158, 665–679. doi:10.1127/1860-1804/2007/0158-0665
Pinheiro, A. C., Mesquita, N., Trovão, J., Soares, F., Tiago, I., Coelho, C., et al. (2019). Limestone biodeterioration: a review on the Portuguese cultural heritage scenario. J. Cult. Herit. 36, 275–285. doi:10.1016/j.culher.2018.07.008
Pinho, L., and Mosquera, M. J. (2013). Photocatalytic activity of TiO2-SiO2 nanocomposites applied to buildings: influence of particle size and loading. Appl. Catal. B Environ. 134-135, 205–221. doi:10.1016/j.apcatb.2013.01.021
Pio, C. A., Ramos, M. M., and Duarte, A. C. (1998). Atmospheric aerosol and soiling of external surfaces in an urban environment. Atmos. Environ. 32, 1979–1989. doi:10.1016/s1352-2310(97)00507-4
Possenti, E., Colombo, C., Bersani, D., Bertasa, M., Botteon, A., Conti, C., et al. (2016). New insight on the interaction of diammonium hydrogenphosphate conservation treatment with carbonatic substrates: a multi-analytical approach. Microchem. J. 127, 79–86. doi:10.1016/j.microc.2016.02.008
Price, C. A., and Doehne, E. (2011). Stone conservation: an overview of current research. 2nd Edn. Los Angeles, CA: Getty Conservation Institute, 164.
Přikryl, R., and Smith, B. J. (2007). Building stone decay: from diagnosis to conservation. London, UK: Geological Society of London, Vol. 271, 330.
Rodríguez-Navarro, C., and Doehne, E. (1999). Salt weathering: influence of evaporation rate, supersaturation and crystallization pattern. Earth Surf. Process. Landforms. 24 (3), 191–209. doi:10.1002/(sici)1096-9837(199903)24:3<191::aid-esp942>3.0.co;2-g
Saba, M., Hernandez-Romero, L. N., Lizarazo-Marriaga, J., and Quiñones-Bolaños, E. E. (2019). Petrographic of limestone cultural heritage as the basis of a methodology to rock replacement and masonry assessment: cartagena de Indias case of study. Case Stud. Constr. 11, e00281. doi:10.1016/j.cscm.2019.e00281
Saiz Jiménez, C., Brimblecombe, P., Camuffo, D., Lefèvre, R. A., and Van Grieken, R. (2004). “Damages caused to European monuments by air pollution: assessment and preventive measures,” in Air pollution and cultural heritage. Editors Saiz-Jimenes, C. (Leiden, Netherlands: Balkema Publishers), 91–109.
Sassoni, E., Franzoni, E., Pigino, B., Scherer, G. W., and Naidu, S. (2012). “Effectiveness of hydroxyapatite as a consolidating treatment for lithotypes with varying carbonate content and porosity,” in Proceedings of 5th international congress science and technology for the safeguard of cultural heritage in the Mediterranean Basin, Istanbul, Turkey, November 2011, 338–343.
Sassoni, E., Franzoni, E., Pigino, B., Scherer, G. W., and Naidu, S. (2013). Consolidation if calcareous and siliceous sandstones by hydroxiapatite: comparision with a TEOS based consolidant. J. Cult. Herit. 14, 103–108. doi:10.1016/j.culher.2012.11.029
Sassoni, E., Franzoni, E., Stefanova, M., Kamenarov, Z., Scopece, P., and Verga Falzacappa, E. (2020). Comparative study between ammonium phosphate and ethyl silicate towards conservation of prehistoric paintings in the magura cave (Bulgaria). Coatings 10, 250. doi:10.3390/coatings10030250
Sassoni, E., Graziani, G., Franzoni, E., and Scherer, G. W. (2018). Conversion of calcium sulfate dihydrate into calcium phosphates as a route for conservation of gypsum stuccoes and sulfated marble. Construct. Build. Mater. 170, 290–301. doi:10.1016/j.conbuildmat.2018.03.075
Sassoni, E. (2017). Phosphate-based treatments for conservation of stone. RILEM Tech Lett. 2, 14–19. doi:10.21809/rilemtechlett.2017.34
Sassoni, E. (2018). Hydroxyapatite and other calcium phosphates for the conservation of cultural heritage: a review. Materials 11, 554. doi:10.3390/ma11040557
Sassoni, E., Naidu, S., and Scherer, G. W. (2011). The use of hydroxyapatite as a new inorganic consolidant for damaged carbonate stones. J. Cult. Herit. 12, 346–355. doi:10.1016/j.culher.2011.02.005
Schiavon, N. (2000). “Granitic building stone decay in an urban environment: a case of authigenic kaolinite formation by heterogeneous sulphur dioxide attack,”in Proceedings of the 9th international congress on deterioration and conservation of stone. Editors Fassina, V. (Venice), 411–421.
Shekofteh, A., Molina, E., Rueda-Quero, L., Arizzi, A., and Cultrone, G. (2019). The efficiency of nanolime and dibasic ammonium phosphate in the consolidation of beige limestone from the Pasargadae World Heritage Site. Archaeol. Anthropol. Sci. 11, 5065–5080. doi:10.1007/s12520-019-00863-y
Sierra-Fernandez, A., Gomez-Villalba, L. S., Rabanal, M. E., and Fort, R. (2017). New nanomaterials for applications in conservation and restoration of stony materials: a review. Mater. Construcción 67, 107. doi:10.3989/mc.2017.07616
Slavíkova, M., Frantisek, K. F., Zemlicka, J., Pech, M., Kotlík, P., and Jakubek, J. (2012). X-ray radiography and tomography for monitoring the penetration depth of consolidants in Opuka - the building stone of Prague monuments. J. Cult. Herit. 13, 357–364. doi:10.1016/j.culher.2012.01.010
Snethlage, R., Gruber, C., Tucic, V., and Wendler, V. (2008). “Transforming gypsum into calcium phosphate: a better way to preserve lime paint layers on natural stone?,” in Stone consolidation in cultural heritage research and practice: Proceedings of the International Symposium, Lisbon, Portugal, May 6–7, 2008, Editors Mimoso, J.M., and Delgado Rodrigues, J., 1–14.
Tamrakar, N. K., Yokota, S., and Shrestha, S. D. (2007). Relationships among mechanical, physical and petrographic properties of Siwalik sandstones, Central Nepal Sub-Himalayas. Eng. Geol. 90, 105–123. doi:10.1016/j.enggeo.2006.10.005
Thornbush, M. J., and Viles, H. A. (2007). Simulation of the dissolution of weathered versus unweathered limestone in carbonic acid solutions of varying strength. Earth Surf. Process. Landforms. 32, 841–852. doi:10.1002/esp.1441
Török, Á. (2008). Black crusts on travertine: factors controlling development and stability. Environ. Geol. 56, 583–594. doi:10.1007/s00254-008-1297-x
Turkington, A. V., Martin, E., Viles, H. A., and Smith, B. J. (2003). Surface change and decay of sandstone samples exposed to a polluted urban atmosphere over a six-year period: belfast, Northern Ireland. Build. Environ. 38, 1205–1216. doi:10.1016/s0360-1323(03)00077-5
UNE-EN 13919. (2003). Métodos de ensayo para piedra natural. Determinación de la resistencia al envejecimiento por la acción de SO2 en presencia de humedad. Madrid, Spain: AENOR.
UNE-EN 15803. (2010). Conservation of cultural property. Test methods. Determination of water vapour permeavility. Madrid, Spain: AENOR.
UNE-EN 15886. (2011). Conservation of cultural property. Test methods. Colour measurement of surfaces. Madrid, Spain: AENOR.
UNE-EN ISO 12572. (2002). Hygrothermal performance of building materials and products. Determination of water vapour transmission properties. Madrid, Spain: AENOR.
UNE-EN ISO 25178-2 (2013). Geometrical product specifications (GPS). Surface texture: areal. Part 2: terms, definitions and Surface texture parameters. Madrid, Spain: AENOR.
UNE-EN ISO 25178-3. (2013). Geometrical product specifications (GPS). Surface texture: areal. Part 3: specification operators. Madrid, Spain: AENOR.
Unterwurzacher, M., and Mirwald, P. W. (2008). Initial stages of carbonate weathering: climate chamber studies under realistic pollution conditions. Environ. Geol. 56, 507–519. doi:10.1007/s00254-008-1440-8
Urosevic, M., Sebastián, E., and Cardell, C. (2013). An experimental study on the influence of surface finishing on the weathering of a building low-porous limestone in coastal environments. Eng. Geol. 154, 131–141. doi:10.1016/j.enggeo.2012.12.013
Urosevic, M., Sebastián-Pardo, E., and Cardell, C. (2010). Rough and polished travertine building stone decay evaluated by a marine aerosol aging test. Construct. Build. Mater. 24, 1439–1448. doi:10.1016/j.conbuildmat.2010.01.011
Vázquez, M. A., Galán, E., Guerrero, M. A., and Ortiz, P. (2011). Digital image processing of weathered stone caused by efflorescences: a tool for mapping and evaluation of stone decay. Construct. Build. Mater. 25, 1603–1611. doi:10.1016/j.conbuildmat.2010.10.003
Vázquez, P., Alonso, F. J., Carrizo, L., Molina, E., Cultrone, G., Blanco, M., et al. (2013a). Evaluation of the petrophysical properties of sedimentary building stones in order to establish quality criteria. Construct. Build. Mater. 41, 868–878. doi:10.1016/j.conbuildmat.2012.12.026
Vázquez, P., Luque, A., Alonso, F. J., and Grossi, C. M. (2013b). Surface changes on crystalline stones due to salt crystallisation. Environ. Earth. Sci. 69, 1237–1248. doi:10.1007/s12665-012-2003-6
Vázquez, P., Carrizo, L., Thomachot-Schneider, C., Gibeaux, S., and Alonso, F. J. (2016). Influence of surface finish and composition on the deterioration of building stones exposed to acid atmospheres. Construct. Build. Mater. 106, 392–403. doi:10.1016/j.conbuildmat.2015.12.125
Vergès-Belmin, V. (2008). Illustrated glossary on stone deterioration Patterns: Illustrated glossary on the forms of weathering of stone. Paris: ICOMOS (International Council on Monuments and Sites) and ISCS (International Scientific Committee for Stone), Vol. 15 [in French, with English summary].
Wheeler, G. (2005). “Historical overview,” in Alkoxysilanes and the consolidation of stone. Research in conservation. Editors Wheeler, G. (Los Angeles, CA: Getty Conservation Institute), 1–11.
Keywords: sedimentary stones, surface finishes, calcium phosphate, acid attack, durability
Citation: Molina E, Arizzi A, Benavente D and Cultrone G (2020) Influence of Surface Finishes and a Calcium Phosphate-Based Consolidant on the Decay of Sedimentary Building Stones Due to Acid Attack. Front. Mater. 7:581979. doi: 10.3389/fmats.2020.581979
Received: 10 July 2020; Accepted: 28 September 2020;
Published: 30 October 2020.
Edited by:
Enrico Sassoni, University of Bologna, ItalyReviewed by:
Mauro La Russa, University of Calabria, ItalyCopyright © 2020 Molina, Arizzi, Benavente and Cultrone. This is an open-access article distributed under the terms of the Creative Commons Attribution License (CC BY). The use, distribution or reproduction in other forums is permitted, provided the original author(s) and the copyright owner(s) are credited and that the original publication in this journal is cited, in accordance with accepted academic practice. No use, distribution or reproduction is permitted which does not comply with these terms.
*Correspondence: E. Molina, ZWR1YXJkby5tb2xpbmFAdWNhLmVz
Disclaimer: All claims expressed in this article are solely those of the authors and do not necessarily represent those of their affiliated organizations, or those of the publisher, the editors and the reviewers. Any product that may be evaluated in this article or claim that may be made by its manufacturer is not guaranteed or endorsed by the publisher.
Research integrity at Frontiers
Learn more about the work of our research integrity team to safeguard the quality of each article we publish.