- 1State Key Laboratory of Structural Chemistry, Fujian Institute of Research on the Structure of Matter, Chinese Academy of Sciences, Fuzhou, China
- 2College of Chemistry and Materials Science, Fujian Normal University, Fuzhou, China
A novel photoactive semiconductor (named as IDTOT-4F) with an A-π-D-π-A-type configuration is synthesized. It contains an electron-donating fused ring (D) as the core flanked with two π-spacers and is end-capped with two electron-withdrawing units (A). The intramolecular charge transfer effect endows IDTOT-4F with strong and broad light absorption and a relatively narrow band gap (1.46 eV). Thin-film optoelectrical devices based on IDTOT-4F exhibit both n-type and p-type switching behaviors. Besides, the p-channel device shows significantly photoresponsive performance with the maximum P (photo/dark current ratio), R (photoresponsivity), and D* (detectivity) values of around 60, 0.07 AW−1, and 2.5 × 1010 Jones, respectively. Further, IDTOT-4F based optoelectrical devices exhibit good optical memory characteristics with a time constant τ1 of 4.6 h, indicating its applicability to nonvolatile optical memory devices. The results provide new insights into the photoresponsive behavior of fused-ring semiconductors and pave the way for the design of nonvolatile optical memory devices.
Introduction
Organic photoresponsive semiconductors have drawn tremendous attentions as an exciting candidate for photodetectors (de Arquer et al., 2017; Park et al., 2018; Li et al., 2019; Huang et al., 2020), artificial synaptic devices (Park and Lee, 2017; Dai et al., 2019; Deng et al., 2019; Yu et al., 2019; Shi et al., 2020), and particularly nonvolatile memory (Leydecker et al., 2016; Cheng et al., 2018; Liu et al., 2019; Yu et al., 2019), owing to their facile structure modification, excellent optoelectronic properties, large scale fabrication, low temperature processing, and mechanical flexibility. However, the number of semiconductors with good intrinsic optical memory behavior is small, and systematic studies on the photoresponsive semiconductors are desired. In recent years, A-π-D-π-A-type fused ring semiconductors have been widely used as non-fullerene acceptors in organic solar cells because of their wide spectral response ranging from visible region to near-infrared region, narrow band gaps, and high absorption coefficients (Yao et al., 2017; Hou et al., 2018; Liu et al., 2018; Yan et al., 2018). Relying on these unique optical properties, it is believed that these A-π-D-π-A-type fused ring acceptors would be ideal candidates for organic photodetectors, near-infrared (NIR) image sensors, and even optical memory devices (Lee et al., 2019; Huang et al., 2020). Notably, compared with the A-D-A-type structure, the π-spacer in the A-π-D-π-A-type structure can expand the effective conjugation length of acceptors and further extend their absorptions (Li et al., 2020). To date, systematic studies on the photoresponsive behaviors of these A-π-D-π-A-type acceptors are scarce, which might hamper their application on the light stimuli response electronic devices.
In this work, we synthesize a new A-π-D-π-A-type small molecule named as IDTOT-4F and systematically study the photoresponse of devices based on IDTOT-4F. IDTOT-4F uses indacenodithiophene as the central electron-donor unit, which is flanked with two thienyl units as the π-spacers and terminated with 2-(5,6-difluoro-3-oxo-2,3-dihydro-1H-inden-1-ylidene)malononitrile (IC-2F) as the electron-acceptor unit. As expected, IDTOT-4F possesses a high molar extinction coefficient of 2.51 × 105 M−1 cm−1, a broad absorption band ranging from 550 to 850 nm, and a narrow band gap of 1.46 eV. Interestingly, IDTOT-4F based devices exhibit both n-type and p-type switching behaviors, and the p-channel device based on IDTOT-4F shows good photoresponse properties. Upon white light irradiation (4,200 μW cm−2) for 10 sec, the p-type optoelectrical device raises the saturated Id from around 100 nA to 1.14 μA (Vg = Vd = − 100 V) with the photo/dark current ratio (P) of 60, photoresponsivity (R) of 0.07 A W−1, and detectivity (D*) of 2.5 × 1010 Jones. The light intensity and pulse width could promote the photogenerated charge carriers, thus leading to enhanced photoresponsive behaviors and higher hole mobilities. More importantly, IDTOT-4F based optoelectrical devices exhibit good photocurrent memory characteristics with a time constant τ1 of 4.6 h, indicating its applicability to nonvolatile optical memory devices. The results provide new insights into the photoresponsive behavior of fused-ring semiconductors and pave the way for the design of nonvolatile optical memory devices.
Materials and Methods
Material Synthesis
The synthetic route of IDTOT-4F is outlined in Scheme 1.
Compound 2
Compound 1 (500 mg, 0.40 mmol), which was synthesized following the reported literature (Liu et al., 2018), and trimethyl (4-octylthiophen-2-yl)stannane (320 mg, 0.89 mmol) were dissolved in anhydrous toluene (20 ml). The mixture was deoxygenated with nitrogen for 10 min before and after adding the catalyst Pd(PPh3)4 (23 mg, 0.02 mmol) to the solution, and then stirred at 120°C for 48 h. After cooling down to room temperature, the solvent was evaporated, and the resulting mixture was purified by silica gel column chromatography with petroleum ether as the eluent, affording a yellow solid (515 mg, 86%). 1H NMR (500M, CDCl3, δ): 7.26 (s, 2H) overlapping 7.26 (CHCl3), 7.25 (d, J = 8.2 Hz, 8H), 7.06 (d, J = 8.3 Hz, 8H), 7.04 (s, 2H), 6.79 (s, 2H), 3.17 (t, J = 7.0 Hz, 4H), 2.56 (t, J = 7.75 Hz, 12H), 1.63–1.56 (m, 12H), 1.48–1.42 (m, 4H), 1.32–1.26 (m, 44H), 1.25–1.19 (m, 4H), 1.14–1.02 (m, 8H), 0.89–0.83 (m, 24H). 13C (125M, CDCl3, δ): 154.88, 148.89, 143.28, 141.51, 139.84, 137.25, 135.13, 135.01, 128.58, 128.10, 125.05, 124.83, 119.17, 116.21, 72.87, 63.81, 35.57, 31.90, 31.62, 31.37, 30.45, 29.84, 29.45, 29.34, 29.28, 29.13, 14.11, 14.02. MALDI-TOF MS (m/z): [M] calcd. for C100H134O2S4, 1,494.93 [M + H]+ found, 1,495.134.
IDTOT
POCl3 (0.5 ml) was added to a solution of Compound 2 (400 mg, 0.27 mmol) in a mixed solvent of N,N-dimethylformamide (DMF, 2.0 ml) and 1,2-dichloroethane (20 ml) at 0°C under nitrogen atmosphere. The mixture was stirred at this temperature for 1 h, then warmed to 80°C and reacted overnight. After cooling down to room temperature, the mixture was poured into ice water, neutralized with saturated Na2CO3 solutions, and extracted with dichloromethane. The combined organic phases were dried over anhydrous Na2SO4, filtered, and concentrated by reduced pressure. The resulting crude product was further purified by silica gel column chromatography (petroleum ether/dichloromethane = 1:2 v/v) to obtain an orange solid (377 mg, 90%). 1H NMR (500M, CDCl3, δ):9.96 (s, 2H), 7.30 (s, 2H), 7.23 (d, J = 8.2 Hz, 8H), 7.09 (s, 2H), 7.08 (d, J = 8.4 Hz, 8H), 3.23 (t, J = 7.2 Hz, 4H), 2.90 (t. J = 7.6 Hz, 4H), 2.57 (t, J = 7.6 Hz, 8H), 1.70–1.64 (m, 4H), 1.61–1.55 (m, 8H) overlapping 1.58 (H2O), 1.52–1.48 (m, 4H), 1.35–1.24 (m, 44H), 1.23–1.20 (m, 4H), 1.15–1.03 (m, 8H), 0.89–0.83 (m, 24H). 13C (125M, CDCl3, δ): 181.54, 155.69, 153.30, 151.40, 149.21, 144.44, 141.95, 140.58, 139.03, 135.24, 135.08, 130.55, 128.50, 128.28, 127.72, 125.94, 124.13, 116.71, 73.52, 63.98, 35.55, 31.85, 31.72, 31.53, 31.39, 31.35, 29.81, 29.37, 29.33, 29.19, 29.11, 28.46, 25.16, 22.65, 25.61, 14.10, 14.00. MALDI-TOF MS (m/z): [M] calcd. for C102H134O4S4, 1,550.92 [M + H]+ found, 1,552.358.
IDTOT-4F
The electron-withdrawing end group 2-(5,6-difluoro-3-oxo-2,3-dihydro-1H-inden-1-ylidene)malononitrile (IC-2F) was synthesized following the reported literature (Yao et al., 2017). IDTOT (200 mg, 0.13 mmol) and IC-2F (89 mg, 0.39 mmol) were dissolved in a mixed solvent of pyridine (1 ml) and chloroform (25 ml). The mixture was deoxygenated with nitrogen for 10 min and then stirred at room temperature for 16 h. After poured the mixture into methanol (200 ml), the resulting precipitates were filtered and further purified by silica gel column chromatography (petroleum ether/dichloromethane = 1:1 v/v) to obtain a dark blue solid (210 mg, 82%). 1H NMR (500M, CDCl3, δ): 8.93 (s, 2H), 8.53–8.50 (m, 2H), 7.59 (t, J = 7.5 Hz, 2H), 7.37 (s, 2H), 7.27–7.25 (m, 10H) overlapping 7.26 (CHCl3), 7.11 (d, J = 8.4 Hz, 8H), 3.35 (t, J = 7.1 Hz, 4H), 2.94 (t, J = 7.5 Hz, 4H), 2.59 (t, J = 7.6 Hz, 8H), 1.78–1.72 (m, 4H), 1.70–1.64 (m, 4H), 1.63–1.56 (m, 8H), 1.43–1.37 (m, 4H), 1.35–1.26 (m, 44H), 1.23–1.13 (m, 8H), 0.89–0.84 (m, 24H). 13C (125M, CDCl3, δ): 185.65, 162.28, 159.42, 156.54, 155.23, 153.80, 153.03, 144.25, 142.29, 138.54, 136.49, 136.43, 135.68, 134.55, 134.33, 131.66, 128.49, 128.45, 126.39, 118.90, 117.10, 115.20, 114.95, 114.81, 114.63, 112.25, 112.10, 74.22, 67.43, 64.09, 35.55, 31.87, 31.72, 31.62, 31.37, 30.02, 29.66, 29.42, 29.19, 29.10, 25.20,22.67, 22.61, 22.54, 14.11, 14.07. MALDI-TOF MS (m/z): [M] calcd. for C126H138F4N4O4S4, 1,974.95; [M + H]+ found, 1,976.355.
Device Fabrication and Characterization
The structure of optoelectrical device is illustrated as Figure 1A. A solution of IDTOT-4F in o-dichlorobenzene (0.5 mg/ml) was drop casted on the pre-cleaned SiO2/Si wafers, where the thickness of SiO2 is approximate 300 nm with a capacitance of 11.5 nF/cm2, and the thickness of the semiconductor layer is around 150 nm. Subsequently, the semiconductor layer was annealed at 120°C for 20 min and then transferred to the glove box. Finally, the source and drain electrodes of Au (50 nm) were thermally evaporated on the semiconductor layer through a shadow mask at a rate of 0.5 Å/sec. The channel width (W) is 5,600 µm and the channel length (L) is 200 µm. Electrical and photoresponse properties of IDTOT-4F-based devices were characterized by a Keithley (4200 SCS) semiconductor analyzer in air.
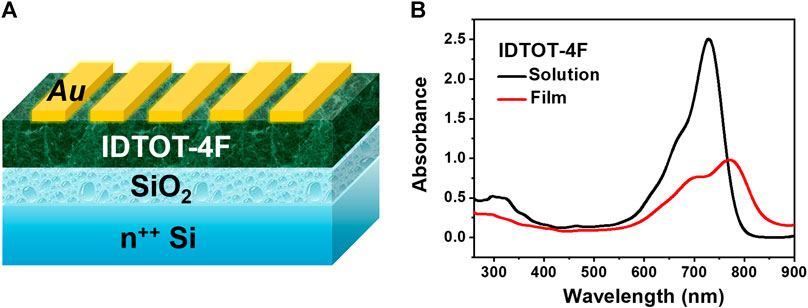
FIGURE 1. (A) The structure of IDTOT-4F based optoelectrical devices; (B) The UV-vis absorption spectrum of IDTOT-4F in chloroform solution (concentration: 1.0 × 10–5 mol/L) and as thin film.
Results and Discussion
Optical and Electrochemical Properties
According to the above-mentioned synthetic route (Scheme 1), IDTOT-4F was successfully synthesized with an A-π-D-π-A-type configuration, using indacenodithiophene as the central electron-donor (D) unit flanked with two thienyl units as the π-spacers and terminated with 2-(5,6-difluoro-3-oxo-2,3-dihydro-1H-inden-1-ylidene)malononitrile as the electron-acceptor (A) unit. Benefiting from the strong intramolecular charge transfer effect (Yao et al., 2017), IDTOT-4F in dilute chloroform solutions exhibits a broad and intense absorption band in the range of 550–800 nm with a maximum molar extinction coefficient (ε) of 2.51 × 105 M−1 cm−1, as shown in Figure 1B. In going from solutions to films, the absorption maxima of IDTOT-4F shows a significant red-shift of 40 nm with an enhanced shoulder peak, indicating the formation of some molecular self-organization in the film (Lin et al., 2015). The optical bandgap of IDTOT-4F estimated from the absorption onset (850 nm) is 1.46 eV, following the equation Eg,opt = 1,240/λonset. The oxidation and reduction onset potentials of IDTOT-4F are measured to be 0.87 and −0.85 V, respectively, through the electrochemical cyclic voltammetry characterization (Supplementary Figure S1). According to the equation EHOMO/LUMO = −e [Eox/red,onset − E(Fc/Fc+) + 4.80], where E(Fc/Fc+) is measured to be 0.14 V, the highest occupied molecular orbital and the lowest unoccupied molecular orbital energy levels of IDTOT-4F were calculated to be −5.53 and −3.81 eV, respectively.
Electrical Properties of Device
Considering IDTOT-4F possesses a high molar extinction coefficient and broad absorption band, which is beneficial for increasing photogenerated charge carriers during irradiation, we next study the electrical properties of its optoelectrical devices. In the dark, IDTOT-4F based devices exhibit both n-type and p-type switching behaviors. As shown in Supplementary Figure S2, the n-channel device obtains a saturated source-drain current (Id) of around 50 nA and an electron mobility of 1.8 × 10–4 cm2 V−1 sec−1 under vacuum at Vg = Vd = 100 V; and Figure 2A shows that the p-type channel device achieves an Id of 96 nA and a hole mobility of 1.0 × 10–4 cm2 V−1 sec−1 at Vg = Vd = − 100 V. Noticeably, when the p-type device is irradiated with white light (4,200 μW cm−2) for 10 sec, the saturated Id is significantly enhanced to 1.14 μA, with an increased hole mobility of 8.4 × 10–4 cm2 V−1 sec−1 and a maximum P value (Iphoto/Idark) of 62 at Vg = − 20 V. Following the reported formulas (Han et al., 2019), the values of photoresponsivity (R) and detectivity (D*) are almost positively correlated with gate voltage (Supplementary Figure S3), with maximum values of around 0.07 A W−1 and 2.5 × 1010 Jones, respectively, obtained at Vg = − 100 V. Subsequently, the influence of light intensity on IDTOD-4F based p-type optoelectrical devices is systematically investigated. The white light pulse width is 10 sec. As exhibited in Figure 2B, the source-drain current increases steadily as the light intensity increases from 0 to 4,200 μW cm−2 and the saturated Id is positively correlated with light intensity (Figure 2C). The same trend is observed for the hole mobility, which rises from 9.0 × 10–5 to 8.4 × 10–4 cm2 V−1 sec−1. In addition, Supplementary Figure S3 shows that P also increases as the light intensity increases, but R and D* are less correlated with the light intensity. According to equation R=Iphoto/(p × A), where p is the light intensity and A is the area of the semiconductor on the channel of device, Iphoto/p should be a constant because Iphoto shows a good linear relationship with light intensity (Figure 2C); therefore, R should be a constant and there exists no clear relationship between R (and corresponding D*) and the light intensity.
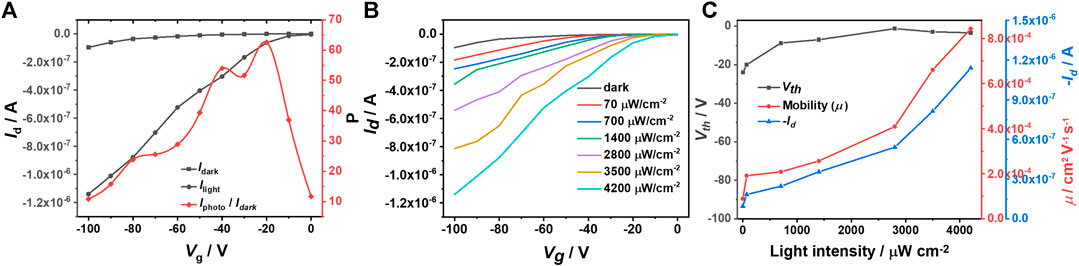
FIGURE 2. (A) Transfer curves of (IDTOT-4F) based optoelectrical devices in the dark and under illumination, and the corresponding P values vs. Vg; (B) Transfer curves of optoelectrical devices at different light intensities with a constant pulse with of 10 sec; (C) Source-drain currents, threshold voltages (Vth) and mobilities (μ) vs. light intensities. The Vd values for the above measurements are set as −100 V.
Apart from the light intensity, the light pulse width could also improve the saturated Id due to the formation of more photogenerated charge carriers in the channel with longer irradiation time. Figure 3 indicates that the saturated Id and the hole mobility increase linearly with the pulse width at a constant light intensity of 700 μW cm−2. Noticeably, here p and A are constants, and therefore, according to equation R=Iphoto/(p × A), R should be positively correlated with Iphoto that means extending the pulse width could improve p, R and D* values simultaneously, as shown in Supplementary Figure S4. Additionally, IDTOT-4F based optoelectrical devices exhibit obvious bias stress behavior. As shown in Supplementary Figure S5A, the saturated Id drops dramatically when the device is measured continuously (every 30 sec). Fortunately, the resilience of these devices is good and Supplementary Figure S5B shows that the saturated Id could reach the initial value when the device is measured every 5 min. Therefore, the above-mentioned measurements with different light intensity and pulse width are conducted every 5 min.
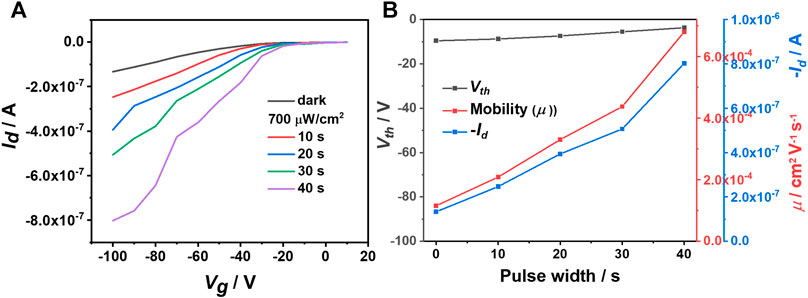
FIGURE 3. (A) Transfer curves of IDTOT-4F based optoelectrical devices under illumination with different pulse widths (Vd = −100 V); (B) Source-drain currents, threshold voltages (Vth) and mobilities (μ) vs. light pulse widths.
Optical Memory Characteristics
Surprisingly, we notice that this semiconductor exhibits good optical memory behavior. As shown in Figure 4A, we measure transfer curves of IDTOT-4F based p-type optoelectrical devices at different retention time (Vg = Vd = − 100 V). The initially saturated Id value of the optoelectrical device under dark conditions is 133 nA. Once the device is irradiated at 4,200 μW cm−2 for 1 min, the saturated Id value is dramatically improved to 1.67 μA. It is worth noting that after keeping the device in the dark for 24 h, the saturated Id value still maintains over 800 nA, which is much higher than the initial value (133 nA). Even after 44 h, the device still shows some optical memory behaviors with the saturated Id value around 222 nA. Biexponential fitting of the current decay curve gives τ1 of 4.6 h and τ2 of 14,842.4 h (Supplementary Figure S6). In order to verify the optical memory nature of IDTOT-4F based optoelectrical devices, we conduct a blank control experiment with the device measured in the dark during the whole time (Supplementary Figure S7A). The saturated Id value drops from the initial 145 to 84 nA after 24 h. As shown in Figure 4B, comparing the retention characteristics between the dark current and the photocurrent, it is found that IDTOT-4F based optoelectrical devices possess good optical memory ability. Another control experiment with P3HT as the semiconducting layer provides further evidence for the good optical memory behavior of IDTOT-4F (Supplementary Figure S7B). The optical memory behavior of IDTOT-4F is presumably elucidated as following: under dark conditions, some holes induced by the gate voltage are trapped at the IDTOT-4F/SiO2 interface, thus suppressing the current. Upon illumination, beneficial from the strong light-absorbing and broad absorption band of IDTOT-4F, the devices gain a lot of photogenerated excitons, which could be dissociated into carriers very fast driven by the large drain voltage. Under the lateral electric field, the separated electron and hole would accumulate at source/drain electrodes, respectively, leading to band bending at semiconductor surfaces and lowering the potential barrier for hole injection. Consequently, more holes are injected into the channel, together with photogenerated holes, the Id are significantly promoted. Further, because of the low intrinsic hole mobility (less than 10–3 cm2 V−1 sec−1) of IDTOT-4F, the photogenerated carriers could not be injected into the channel in a timely manner and therefore a certain number of photogenerated carriers still remain in the semiconductor layer. The minority carriers recombine slowly and could persist for a long time, thus slowing the recovery of current and prolonging the optical memory time.
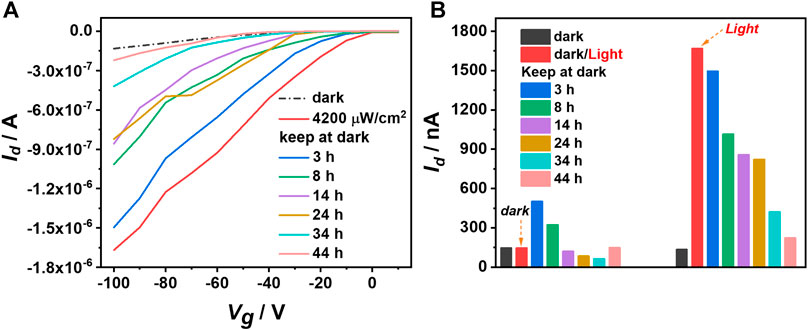
FIGURE 4. (A) Transfer curves of the photocurrent retention characteristics for IDTOT-4F based optoelectrical devices (Vd = −100 V); (B) Histogram contrast between the memory behaviors of the dark current and the photocurrent.
Conclusion
In conclusion, we synthesize a novel A-π-D-π-A-type organic semiconductor named as IDTOT-4F. Benefiting from the strong intramolecular charge transfer effect, IDTOT-4F possesses a broad absorption band ranging from 550 to 850 nm, a narrow bandgap of 1.46 eV, and a high molar extinction coefficient of 2.51 × 105 M−1 cm−1. The optoelectrical devices based on IDTOT-4F exhibit both n-type and p-type switching behaviors. Noticeably, the p-channel devices based on IDTOT-4F exhibit good photoresponse properties. Upon white light irradiation (4,200 μW cm−2) for 10 sec, the p-type device achieves a saturated Id of 1.14 μA (Vg = Vd = − 100 V) with P, R and D* values of around 60, 0.07 A W−1 and 2.5 × 1010 Jones, respectively. The light intensity and pulse width could promote the photogenerated charge carriers, thus leading to enhanced photoresponsive behaviors and higher hole mobilities. More importantly, IDTOT-4F based optoelectrical devices exhibit good optical memory characteristics and biexponential fitting of the current decay curve gives τ1 of 4.6 h, indicating its applicability to nonvolatile optical memory devices. The results provide new insights into the photoresponsive behavior of fused-ring semiconductors and pave the way for the design of nonvolatile optical memory devices.
Data Availability Statement
The raw data supporting the conclusions of this article will be made available by the authors, without undue reservation.
Author Contributions
SF performed the experiments and wrote the manuscript. WH directed the project. All authors contributed to the data analyses.
Conflict of Interest
The authors declare that the research was conducted in the absence of any commercial or financial relationships that could be construed as a potential conflict of interest.
Acknowledgments
This work was supported by the start-up funding from FJIRSM-CAS, and National Natural Science foundation of China (51803214). WH is also grateful for the award of “The Recruitment Program of Global Youth Experts.”
Supplementary Material
The Supplementary Material for this article can be found online at: https://www.frontiersin.org/articles/10.3389/fmats.2020.567031/full#supplementary-material
References
Cheng, S.-W., Han, T., Huang, T.-Y., Chang Chien, Y.-H., Liu, C.-L., Tang, B. Z., et al. (2018). Novel organic phototransistor-based nonvolatile memory integrated with UV-sensing/green-emissive aggregation enhanced emission (AEE)-active aromatic polyamide electret layer. ACS Appl. Mater. Interfaces 10, 18281–18288. doi:10.1021/acsami.8b02560
Dai, S., Zhao, Y., Wang, Y., Zhang, J., Fang, L., Jin, S., et al. (2019). Recent advances in transistor-based artificial synapses. Adv. Funct. Mater. 29, 1903700. doi:10.1002/adfm.201903700
de Arquer, F. P. G., Armin, A., Meredith, P., and Sargent, E. H. (2017). Solution-processed semiconductors for next-generation photodetectors. Nat. Rev. Mater. 2, 16100. doi:10.1038/natrevmats.2016.100
Deng, W., Zhang, X., Jia, R., Huang, L., Zhang, X., and Jie, J., (2019). Organic molecular crystal-based photosynaptic devices for an artificial visual-perception system. NPG Asia Mater. 11, 77. doi:10.1038/s41427-019-0182-2
Han, T., Shou, M., Liu, L., Xie, Z., Ying, L., Jiang, C., et al. (2019). Ultrahigh photosensitive organic phototransistors by photoelectric dual control. J. Mater. Chem. C 7, 4725–4732. doi:10.1039/c9tc00324j
Hou, J., Inganäs, O., Friend, R. H., and Gao, F. (2018). Organic solar cells based on non-fullerene acceptors. Nat. Mater. 17, 119–128. doi:10.1038/nmat5063
Huang, J., Lee, J., Vollbrecht, J., Brus, V. V., Dixon, A. L., Cao, D. X., et al. (2020). A high-performance solution-processed organic photodetector for near-infrared sensing. Adv. Mater. 32, 1906027. doi:10.1002/adma.201906027
Lee, J., Ko, S.-J., Lee, H., Huang, J., Zhu, Z., Seifrid, M., et al. (2019). Side-chain engineering of nonfullerene acceptors for near-infrared organic photodetectors and photovoltaics. ACS Energy Lett. 4, 1401–1409. doi:10.1021/acsenergylett.9b00721
Leydecker, T., Herder, M., Pavlica, E., Bratina, G., Hecht, S., Orgiu, E., et al. (2016). Flexible non-volatile optical memory thin-film transistor device with over 256 distinct levels based on an organic bicomponent blend. Nat. Nanotechnol. 11, 769–775. doi:10.1038/nnano.2016.87
Li, M., Liu, Y., Zhou, Y., Yang, L., Shen, S., Song, J., et al. (2020). Photovoltaic performances of fused ring acceptors with isomerized ladder-type dipyran cores. ACS Appl. Mater. Interfaces 12, 4887–4894. doi:10.1021/acsami.9b19676
Li, W., Xu, Y., Meng, X., Xiao, Z., Li, R., Jiang, L., et al. (2019). Visible to near-infrared photodetection based on ternary organic heterojunctions. Adv. Funct. Mater. 29, 1808948. doi:10.1002/adfm.201808948
Lin, Y., Wang, J., Zhang, Z.-G., Bai, H., Li, Y., Zhu, D., et al. (2015). An electron acceptor challenging fullerenes for efficient polymer solar cells. Adv. Mater. 27, 1170–1174. doi:10.1002/adma.201404317
Liu, Y., Li, M., Zhou, X., Jia, Q.-Q., Feng, S., Jiang, P., et al. (2018). Nonfullerene acceptors with enhanced solubility and ordered packing for high-efficiency polymer solar cells. ACS Energy Lett. 3, 1832–1839. doi:10.1021/acsenergylett.8b00928.
Liu, Y., Yang, Y., Shi, D., Xiao, M., Jiang, L., Tian, J., et al. (2019). Photo-/thermal-responsive field-effect transistor upon blending polymeric semiconductor with hexaarylbiimidazole toward photonically programmable and thermally erasable memory device. Adv. Mater. 31, 1902576. doi:10.1002/adma.201902576
Park, S., Fukuda, K., Wang, M., Lee, C., Yokota, T., Jin, H., et al. (2018). Ultraflexible near-infrared organic photodetectors for conformal photoplethysmogram sensors. Adv. Mater. 30, 1802359. doi:10.1002/adma.201802359
Park, Y., and Lee, J.-S. (2017). Artificial synapses with short- and long-term memory for spiking neural networks based on renewable materials. ACS Nano 11, 8962–8969. doi:10.1021/acsnano.7b03347
Shi, W., Guo, Y., and Liu, Y. (2020). When flexible organic field-effect transistors meet biomimetics: a prospective view of the internet of things. Adv. Mater. 32, 1901493. doi:10.1002/adma.201901493
Yan, C., Barlow, S., Wang, Z., Yan, H., Jen, A. K.-Y., Marder, S. R., et al. (2018). Non-fullerene acceptors for organic solar cells. Nat. Rev. Mater. 3, 18003. doi:10.1038/natrevmats.2018.3
Yao, H., Cui, Y., Yu, R., Gao, B., Zhang, H., and Hou, J. (2017). Design, synthesis, and photovoltaic characterization of a small molecular acceptor with an ultra-narrow band gap. Angew. Chem. Int. Ed. 56, 3045–3049. doi:10.1002/anie.201610944
Keywords: photo response, optical memory, A-π-D-π-A-type, photoactive semiconductor, optoelectrical device
Citation: Feng S, Dai D, Lin Y, Chen S, Wu X and Huang W (2020) An A-π-D-π-A-Type Organic Semiconductor Based Optoelectrical Device With Photo Response and Optical Memory Behaviors. Front. Mater. 7:567031. doi: 10.3389/fmats.2020.567031
Received: 29 May 2020; Accepted: 28 August 2020;
Published: 21 September 2020.
Edited by:
Tao Li, Shanghai Jiao Tong University, ChinaReviewed by:
Xiaotao Zhang, Tianjin University, ChinaYunlong Guo, Institute of Chemistry (CAS), China
Copyright © 2020 Feng, Dai, Lin, Chen, Wu and Huang. This is an open-access article distributed under the terms of the Creative Commons Attribution License (CC BY). The use, distribution or reproduction in other forums is permitted, provided the original author(s) and the copyright owner(s) are credited and that the original publication in this journal is cited, in accordance with accepted academic practice. No use, distribution or reproduction is permitted which does not comply with these terms.
*Correspondence: Shiyu Feng, fengshiyu@fjirsm.ac.cn, Weiguo Huang, whuang@fjirsm.ac.cn