- 1LEPABE – Laboratory for Process Engineering, Environment, Biotechnology and Energy, Faculty of Engineering, University of Porto, Porto, Portugal
- 2Universidade Católica Portuguesa, CBQF - Centro de Biotecnologia e Química Fina - Laboratório Associado, Escola Superior de Biotecnologia, Porto, Portugal
Calcium phosphates (CaPs) have been widely used in the field of biomedical engineering as bone graft substitutes or as carriers for drug delivery applications. Recent developments have focused on combining CaPs with proteins to obtain functional biomaterials that accommodate a broader spectrum of functional requirements. Silk sericin was considered an unutilized protein by-product from the textile industry, generating tons of residues every year. However, much effort has been dedicated to its recovery after being associated with numerous biological properties such as antioxidant, antibacterial, anti-coagulation and regenerative activities. In the past years, sericin has also demonstrated to be suitable as a template for CaP mineralization. The present review focuses on the recent developments for the production of sericin/CaP composites, exploring their potential applications in bioengineering and opening new avenues in other research fields such as in the cosmetic, food and environmental sectors. In addition, this paper can also be useful as a guideline to design future research based on sericin/CaP biomaterials.
Introduction
Bone matrix consists of both a non-mineralized organic component (~20% of the wet weight) and a mineralized inorganic component (~65–70%), composed mainly by collagen and carbonated calcium phosphate (CaP) crystals, respectively. Crystallographic c-axis of these crystals are arranged parallelly to the longitudinal axis of the fibrous protein, forming a tough and flexible nanocomposite structure (Fuchs et al., 1997; Amini et al., 2012).
Accordingly, calcium phosphates (CaPs) from natural or synthetic nature, are widely used in bone-related applications due to their biocompatibility, low density, chemical stability and crystallinity (Vallet-Regí and González-Calbet, 2004). Among existing CaPs, hydroxyapatite (HAp) is the most studied biomaterial due to its close similarity to human hard tissue in terms of morphology and composition (Habraken et al., 2016).
In a quest to mimic bone tissue, several studies have focused on the synthesis of CaP composites using synthetic polymers [polyglycolic acid (PGA), poly L-lactic acid (PLLA), polylactic-coglycolic acid (PLGA), and polycaprolactone (PCL)] (Rezwan et al., 2006; Lee and Yuk, 2007; Chen et al., 2014), natural polymers (alginate, chitosan, cellulose) (Pighinelli and Kucharska, 2013; Cardoso et al., 2014; Salama, 2019), proteins (collagen, fibrin, keratin, and silk fibroin) (Dias et al., 2010; Bleek and Taubert, 2013; Brown and Barker, 2014; Liu, 2015; Farokhi et al., 2018), or combinations of the foregoing (Chen et al., 2014). Synthetic polymers may induce local and systemic host reactions due to the release of chemicals and monomers from polymer degradation, while natural polymers may lead to products with different characteristics due to the differences in raw materials (Chen et al., 2014).
Proteins have demonstrated to regulate the size, particle size distribution, morphology, and assembly method of nanostructured CaPs, being thus used to fabricate novel materials with biomimetic characteristics for hard tissue repair (Cai and Yao, 2010; Chen et al., 2014). Further, their natural origin confers unique biocompatibility, versatility, and biodegradability properties (Swetha et al., 2010). Collagen has been conventionally used in the synthesis of organic/CaP composites. Although collagen/CaP composites have a greater similarity to natural bone, the clinical application of collagen-type biomaterials is still limited due to its high cost, increased risk of cross-infection and fast degradability (Chen et al., 2014). Alternative protein systems can play a pivotal role during the mineralization of CaP, as a binding-matrix. Keratin/CaP and fibrin/CaP composites have biocompatibility and bioactivity properties suitable for bone tissue engineering. However, these biomaterials lack mechanical properties and processing properties, and have fast biodegrability (Gsib et al., 2017; Shavandi et al., 2017).
In this context silk is a valuable alternative due to its excellent intrinsic properties such as non-toxicity, biodegradability, self-assembly, mechanical stability and controllable structure (Altman et al., 2003; Ha et al., 2013). In nature, silk-like proteins are produced by several organisms such as silkworms, spiders, mollusks, scorpions, bees, and ants (Holland et al., 2019). The use of silk from the domesticated silkworm Bombyx mori is well-established as suitable for biomedical applications due to its abundance, batch-to-batch stability, and clinical track record (Ude et al., 2014). This type of silk comprises a fibrous semi-crystalline silk core, silk fibroin, which is mainly responsible for the load-bearing capacity, and an outer layer of a globular protein, sericin, which serves as gluing agent and has a protective function. While fibroin is processed in large scale in the textile industry, sericin is a by-product generated during this process, in the so-called degumming procedure (Mondal et al., 2006).
Silk fibroin has been widely used and investigated for applications such as sutures (Saxena et al., 2014), artificial ligaments (Farè et al., 2013), tissue engineering constructs (Kasoju and Bora, 2012), and substrates for cell culture (Liu et al., 2012). Fibroin/CaP composites have been reported and are considered suitable for load bearing applications (Yan et al., 2013; Farokhi et al., 2018).
Silk sericin, on the other hand, was until recently considered unfit for biomedical use. Of 1 million tons of silkworm cocoons produced each year worldwide, ~50,000 t of sericin are generated, leading to environmental and economic concerns (Aramwit et al., 2012b). This protein, in its native state, was found to elicit immune and allergic responses when present in virgin silk sutures (Soong and Kenyon, 1984). However, emerging evidence suggests that extracted sericin per se is biocompatible, similarly to silk fibroin or collagen (Chirila et al., 2013; Lamboni et al., 2015). Therefore it has been gaining reputation in the biomedical field with several works showing its potential, as revised elsewhere (Kunz et al., 2016; Ahsan et al., 2018). Thus, the use of sericin/CaP composites not only reduces the inherent environmental impact of sericin disposal but also allows the development of new functional biomaterials. This silk protein increases antioxidant, anti-tyrosinase and anti-inflammatory activity; stimulates collagen production, tumor inhibitory effects; induces the nucleation of bone-like CaPs; and promotes stability and prolonged release in drug delivery systems (Aramwit, 2014; Lamboni et al., 2015).
The molecular weight distribution and even the amino acid composition of sericin were found to be dependent on the extraction method used (Yang et al., 2014c; Kunz et al., 2016). Sericin consists of 18 amino acids, among which serine, histidine, glutamic acid, aspartic acid, threonine, and tyrosine are normally present in higher percentages. Thus, this protein is highly hydrophilic and its molecular weight can range from 20 to 400 kDa (Kunz et al., 2016). Its polar side chains have several functional groups (carboxyl, amine, hydroxyl) responsible for moisturizing and oxidizing properties, allowing the interaction with other compounds through crosslinking, copolymerization or blending to form improved biomaterials (Ahsan et al., 2018). According to the literature, amino acids can highly influence the mineralization process of CaPs. Glutamic acid and aspartic acid play a critical role in controlling HAp nucleation and growth, by attracting calcium and phosphate ions and consequently increasing the local supersaturation, which results in the development of crystals. Histidine and other negatively charged amino acids are involved in HAp nucleation within extracellular matrix proteins (George and Veis, 2008; Tavafoghi and Cerruti, 2016).
Sericin mainly adopts the form of an amorphous random spiral and may also present the form of a β-sheet organized structure. The random spiral easily acquires β-sheet conformation as a consequence of moisture absorption and mechanical elongation, forming a denser, organized, crystalline and less soluble structure. If the protein is dissolved in hot water and subsequently undergoes a temperature decrease, its random coil structure becomes β-sheet, acquiring a gelatinous form (Chen et al., 2015). That is, with the change in temperature the conformation of the protein changes. Upon heating the sericin dissolves in solution, upon cooling the sericin solution jellifies (Sol-Gel properties; Kunz et al., 2016).
Silk Sericin/Calcium Phosphate-Based Materials
Recently, sericin has demonstrated to be suitable as a template for CaP mineralization. In the literature, sericin/CaP biomaterials are usually synthetized by wet mechanochemical processes, where mineralization occurs by spontaneous nucleation (Cai et al., 2009, 2010) or using a surface as template (Takeuchi et al., 2005b; Yang et al., 2014a). The resulting composites generally present a poor crystallinity and the size of the particles generated can range from 20 to 500 nm in length to 3–80 nm in width (Cai et al., 2009, 2010; Veiga et al., 2019). The influence of several experimental conditions on the formation of these sericin/CaPs has been studied by several authors, namely different sericin extraction and storage procedures, mineralization time, sericin concentration, and temperature (Table 1). In the works of Takeuchi et al. (2003a, 2005b), sericin was obtained by extraction in boiling water, using different temperatures and storage procedures. It was found that high molecular weight sericin and β-sheet structure induces apatite deposition. These conditions were achieved for higher extraction temperatures and sericin storage. It is well-known that the extraction method used influences the molecular weight and the amino acid concentration of sericin, which results in different physical and biological properties (Kunz et al., 2016). According to Aramwit et al. (2010), extraction in boiling water is the least toxic procedure to cells and activates the highest collagen production. Acid, alkaline, and urea extraction methods can also be used to obtain sericin that promotes the formation of bone organic matrix. However, these approaches can result in toxicity at higher sericin concentrations (Padamwar and Pawar, 2004). Moreover, acid and alkaline methods have a degrading effect on proteins and considerably increase its solubility. Although urea and enzymatic methods can be used to obtain unchanged sericin, the procedures are expensive and time consuming. On the other hand, hot-water extraction is a simple and environmentally friendly method that preserves the main characteristics of silk sericin (Gulrajani et al., 2000; Dash et al., 2008).
As for the influence of the mineralization time on apatite deposition, it was found that the optimum days for growth of nano HAp crystals with low crystallinity is 7 days. In contrast, after 30 days, the size of HAp crystals increases, and other CaP phases are formed (Sukjai et al., 2012). Further, it was also shown that nucleation of HAp mediated by sericin is a gradual process, since nanoneedle crystals with low crystallinity are only grown after at least 24 h of mineralization (Yang et al., 2014b). In the work of Zhang et al. (2014), the same conclusions were drawn for ethanol-treated sericin films immersed in SBF. A three-dimensional (3D) structure with globular carbonate apatite particles was observed after 7 days. Further, rigid and brittle properties of the film increased with mineralization time, since apatite particles were increasingly being deposited. While the elastic modulus increased significantly after 5 and 7 days (23.027 ± 0.83 to 57.02 ± 1.04 MPa), the tensile strength (day 1: 1.58 ± 0.53, day 7: 0.24 ± 0.18) and the elongation at break of the 3D film decreased (day 1: 112.83 ± 49.02%, day 7: 2.01 ± 1.81%). The increase of the mineralization time and sericin concentration was also reported to lead to an increase in the mean particle size of the particles synthetized (Cai et al., 2009). The recent developments in sericin/CaP composites and its potential for biomedical applications is presented in the next section.
Research Applications
Biomineralization
Although proteins play a crucial role during mineral formation in biological systems, the biomineralization process is still far from being understood (Subburaman et al., 2006). Therefore, most studies on sericin/CaP composites focus on contributing to the understanding of this phenomenon. Sericin/CaP nanoparticle systems obtained are generally nanorod-like crystals with poor crystallinity. Further, sericin promotes crystal growth of HAp along the c-axis, resembling natural biomineralization. Although in the work of Cai et al. (2010), HAp particles similar to mineral bone were achieved in the absence of sericin, this was only verified for specific temperature and pH conditions. Other works argue that the presence of sericin promotes a homogeneous assembly of HAp, under several experimental conditions (Cai et al., 2009; Yang et al., 2014b). The proposed mechanisms for the assembly of CaP crystals in the presence of sericin are usually based on changes in the protein conformation. When dissolved, sericin adopts an amorphous structure in which the hydrophilic sidechains are exposed. However, when submitted to different triggers (e.g., mechanical stretching properties, moisture absorption, temperature, dehydration, or using crosslinking agents), changes in random coil structure for β-sheet easily occur (Teramoto and Miyazawa, 2003; Nayak et al., 2012; Kunz et al., 2016).
According to Takeuchi, the induction sites for HAp nucleation in sericin films are governed by the arrangement of carboxyl groups on the protein (Takeuchi et al., 2005b). When a protein acquires a β-sheet conformation, the amino acid side chains alternate between the two faces of the sheet. Hydrophobic side-chains point in one direction and polar side-chains in the other, which can explain orientation of functional groups in β-sheet sericin (Boyle, 2018). For sericin/HAp particles, the presence of chelated calcium ions in the precipitation medium provides nucleation sites. The formed HAp crystals are rearranged along their c-axis by attaching to the sericin molecular chains (Cai et al., 2009).
The understanding of the biomineralization process can lead to numerous applications in biomedical engineering, namely for the development of advanced bone substitutes (Chen et al., 2019; Veiga et al., 2019). Even though pure sericin has poor mechanical properties, which hinders its utilization in bone tissue engineering (bone-TE), its biological properties can promote bone formation and induce the nucleation of bone-like HAp. In fact, sericin/CaP materials have shown the ability to promote cell differentiation and proliferation of bone marrow-derived mesenchymal stem cells (BMSCs) and of human osteosarcoma cells (MG-63; Table 1). Therefore, most of the developed works on sericin/CaP focus on demonstrating the potential of these composites in the field of bone-TE, as biomaterials for bone filling and repair or as drug delivery systems for tumor therapy, as illustrated in Figure 1.
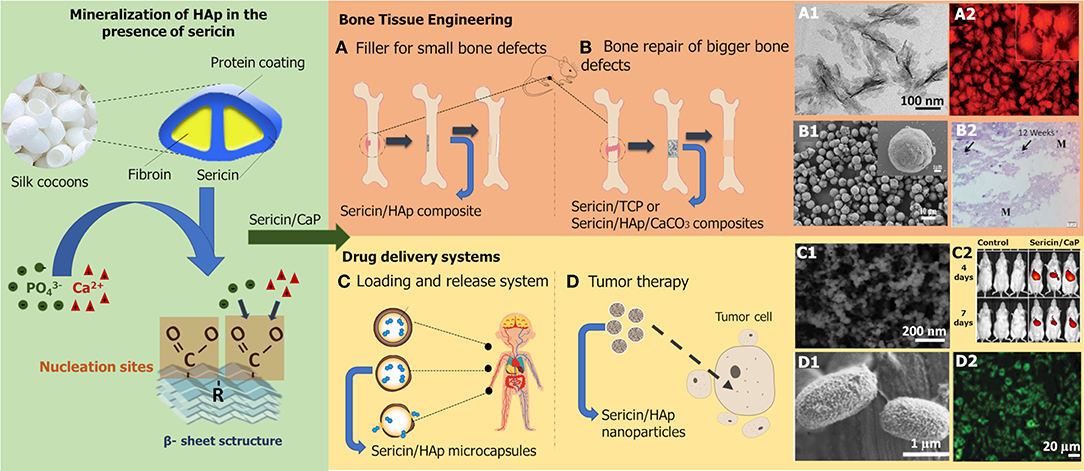
Figure 1. Schematic representation of CaP mineralization process in the presence of sericin and research applications of sericin/CaP composites. Proposed biomineralization mechanism based on the works of Takeuchi et al. (2005b), Cai et al. (2009), and Veiga et al. (2019). (A1) Sericin/HAp composite particles, (A2) cell morphology and differentiation of MG-63 cells after 5 days of contact with the composite reproduced from Cai et al. (2009) with permission from Royal Society of Chemistry; (B1) sericin/CaCO3 composite particles, (B2) mice tissue after 12 weeks of composite implantation reproduced from Zhong et al. (2016) with permission from Elsevier Science & Technology Journals; (C1) sericin/CaP composite nanoparticles, (C2) distribution of the composite in vivo reproduced from Zhao et al. (2017) with permission from John Wiley and Sons; (D1) sericin/HAp composite microcapsules, (D2) cell adhesion activity on HapG-2 cells after 7 days of contact with the composite reproduced from Li et al. (2016) with permission from Royal Society of Chemistry.
Bone Tissue Engineering
Although some authors mention the potential of sericin/CaP as scaffolds for TE (Takeuchi et al., 2003b; Yang et al., 2014b), an ideal 3D scaffold should not only act as a template for tissue growth and has controlled resorbability, but should also elicit a regenerative effect and present adequate mechanical performance over time (Canillas et al., 2017). As previously mentioned, the last requirement is not fulfilled by using only sericin (Ahsan et al., 2018).
Therefore, sericin/CaP biomaterials can better perform as, for instances, a particulate system for bone filling rather than as a 3D regenerative material (Jones, 2013). Accordingly, studies focus on the synthesis of sericin/CaP nanoparticles (Cai et al., 2009), and films (Jones, 2013; Zhang et al., 2014). Sericin/CaP nanoparticles have been described as suitable for bone filling applications due to their biocompatibility, biodegradation, strong ability to promote cell differentiation and proliferation and possibility to deliver therapeutic medicine. Sericin/CaP composite films have also potential in bone-related applications, being biocompatible and promoting cell viability. Further, both particles and films can be used to for fundamental studies of cell–matrix interactions (Table 1).
HAp is a relatively inert material that is retained in vivo for prolonged periods of time due to its slow reabsorption rate (Campana et al., 2014). Thus, sericin/HAp composites have potential as coating in orthopedic or dental implants. This is in agreement with the studies of Klein et al. (1991) and Dhert et al. (1993) where it was found that, when used as a coating material, HAp exhibits excellent bone contact with implanted titanium prosthesis. By comparison, tricalcium phosphate (TCP) materials give rise to more bone remodeling lacunae along the implant surface (Klein et al., 1991). Additionally, it has also been reported that the use of sericin can improve the osseointegration and osteoconduction of orthopedic titanium implants (Nayak et al., 2013), and that sericin/HAp composites have excellent biocompatibility and cell viability (Yang et al., 2014a; Zhang et al., 2014).
In contrast to HAp, TCP-based scaffolds are resorbable, making TCP composites specially suitable for the treatment of small bone defects (Bohner, 2000). In the study of Chasono et al., a TCP-based material led to complete new bone formation after 13–20 weeks of implantation (Chazono et al., 2004). Also, TCP appears to have superior osteoconductivity and bone remodeling, when compared to HAp (Ogose et al., 2005). The co-precipitation of sericin with TCP is demonstrated to decrease the degradation rate and improve the biological performance. According to the results of Takeuchi et al. (2005a), sericin/α-TCP materials show slower degradation in bone defect of rabbits, when compared to pure α-TCP. Other sericin/CaP composites, such as sericin/HAp/calcium carbonate (CaCO3), have also showed controllable degradability properties (Zhao et al., 2013; Yang et al., 2016). In published works, the formation of sericin/HAp composites, was achieved through the conversion of sericin/CaCO3 particles using the assistance of microwave irradiation (Yang et al., 2016) or via a hydrothermal method (Zhong et al., 2016). A higher HAp content was also associated with a slower degradation rate, being therefore appropriate for bone regeneration.
In vivo experiments showed that dicalcium phosphate anhydrous (DCPA), also known as monetite, can resorb faster than most CaP, supporting implant replacement by newly formed tissue (Tamimi et al., 2012). Thus, the sericin/monetite synthetized by Vedakumari et al. (2019) has potential as biodegradable materials.
Drug Delivery Systems
Sericin/CaPs materials have also been proposed as drug delivery systems. Due to their biodegradability and hydrophilicity sericin-based materials may be used as a vehicle to deliver therapeutic medicine (Wang et al., 2015). Ideally, these systems should have controlled degradation, enable easy binding of other molecules and have pH responsiveness (Kunz et al., 2016). Sericin/CaP composites can be designed as microsystems with a good loading and release capability for bioactive molecules to be applied in TE or as an anti-tumor therapeutic system (Table 1). The drug release potential of sericin/CaP microcapsules was studied in a paper by Li et al. (2016), by incubating sericin microcapsules with a supersaturated calcium phosphate solution containing citric acid. The CaP shell protected the inner sericin structure, and consequently the stored bioactive molecules until a pH change occurs. Not only did the capsules showed an improved stability and a longer storage time capability, but also increased cell viability.
In the work of Zhao et al. (2017), sericin/CaP nanoparticles were developed and injected into mice by intravenous injection, to allow particle distribution through the whole body. Analysis of the ex vivo organs showed that 80 nm spherical sericin/CaP nanoparticles are almost completely distributed in the liver cells of mice (≈90%) and can be biodegraded within 2 weeks. However, the degradation rate is not proportional to the amount of composites injected, as 100 and 200 μg of nanoparticles were degraded after 48 h and 13 days, respectively. The targeting percentage was 30% at 7 days post-injection. As no particles were found in other organs, it was concluded that these composites can target specific solid tumors in vivo.
Nowadays, nanoparticle pharmaceutical carriers are probably amongst the most studied sericin/CaP composites, making these very interesting composites in this field. The large surface area/volume ratio provides the capability to load large amounts of biomolecules and the ability to go across the cellular membrane (Jia et al., 2013).
Other Applications
As the study of sericin/CaP composite materials is relatively recent, there are still several areas to be explored, taking advantage of the individual properties of sericin and CaP. For instance, calcium has an important role in maintaining homeostasis in mammalian skin, promoting wound healing (Lansdown, 2002; Kawai et al., 2011). In particular calcium-based nanoparticles have shown to have therapeutic benefits when used in cutaneous wound repair (Kawai et al., 2011). On the other hand, sericin has high antioxidant potential (Prasong, 2011; Kumar and Mandal, 2017), antibacterial activity (Ramesan et al., 2016), mitogenic effect on cells (Terada et al., 2005), potential to inhibit inflammation (Aramwit et al., 2012a), and ability to retain hydration (Padamwar et al., 2005). In the study of Aramwit et al., a sericin-based therapeutic cream formulation improved healing by promoting collagen production and rapid re-epithelialization in rat skin wounds (Aramwit et al., 2009, 2013). Both two-dimensional (films) and three-dimensional (scaffolds) sericin matrices, obtained using different blending methods, have been reported for skin tissue repair (Nayak et al., 2012; Lamboni et al., 2015; Karahaliloglu et al., 2017). Therefore, a sericin/CaP biomaterial could significantly accelerate and improves wound healing and soft tissue replacement. While sericin has been used in many cosmetic products over the years, CaP-based materials are only recently being explored in this area. CaP particles can be used as a bone filler in esthetical treatments for diminishing wrinkles by stimulating conjunctive tissue formation (Lopes et al., 2009). Due to its affinity for biopolymers, crystallites of HAp in the dermis can promote the self-assembly of collagen fibers, smoothing fine lines and even deeper wrinkles (Bell, 2012; Gupta et al., 2019). CaP-based sunscreens have also been studied due to their biocompatible nature that does not generate allergies and other skin irritations, contrary to many organic sunscreens (de Araujo et al., 2010). These properties coupled with sericin strong suppressing activity against UVB-induced acute damage and tumor promotion (Zhaorigetu et al., 2003; Kumar et al., 2018), anti-wrinkle effect and anti-tyrosinase characteristics (Chlapanidas et al., 2013; Kumar and Mandal, 2019) justify the development of sericin/CaP biomaterials for the cosmetic industry.
Different CaP phases are used in food applications, as a supplement to increase calcium and phosphorus intake or as food additives to thicken and stabilize firm foods (Eliaz and Metoki, 2017). Phosphate additives are widely used in processed foods as preservatives, acidifying agents, acidity buffers, and emulsifying agents. Despite their applications, the use of non-naturally occurring phosphates in food can negatively affect the general health of the consumer. Synthetically produced phosphates are effectively absorbed in the gastrointestinal tract, whereas in “organic” phosphates, the absorption percentage ranges between 40 and 60%. This can lead to calcification in blood vessels and organs, being also potentially harmful for patients with chronic kidney disease (Sherman, 2007; Ritz et al., 2012). Studies show that sericin can be used to prevent functional gastrointestinal disorders, improving ion absorption and consequent bioavailability of these elements (zinc, iron, magnesium, and calcium ions) and improving constipation (Sasaki et al., 2000a,b). These characteristics can inspire the synthesis of sericin/CaP materials also for the food industry.
Recent applications of sericin/CaP composites include the removal of micro-pollutants from wastewater. In the work of Koley et al. (2016), sericin/HAp materials were used to purify water, exhibiting excellent adsorption of toxic heavy metal ions. While HAp has stability, sericin is a water-soluble material, capable of forming hydrogen bonds and with strong metal binding properties (Khosa et al., 2014).
Recent Advances
Despite their properties of interest and numerous applications, sericin/CaP composites still have disadvantages to circumvent such as poor mechanical resistance under complex stress states. CaPs and pure sericin have a low mechanical profile when compared to bone tissue, which limits the application of sericin/CaP composites as 3D biomaterials for load-bearing applications (Kunz et al., 2016; Canillas et al., 2017). Of the reviewed papers, only Zhang et al. (2014) reported the mechanical properties of sericin/HAp composites, obtained by mineralization of a flexible ethanol-treated sericin film. Other explored alternatives to enhance these characteristics include the integration of other components in the composite. Adding a structural organic component such as collagen (Griffanti et al., 2019; Jang et al., 2019), chitosan (Chen et al., 2015), and alginate (Zhang et al., 2015); or a synthetic polymer such as polymethyl methacrylate (PMMA) (Tontowi et al., 2017) are examples of some of the most recent approaches. Structural integrity of the constructs can also be obtained by cross-linking the components with glutaraldehyde (GTA) (Bhowmick et al., 2016).
Conclusions
Up to recently, silk sericin was traditionally disposed during silk processing. Nowadays, there has been a growing interest in its recovery due to both its beneficial properties and environmental impact caused by its generation. Works on sericin/CaP composites focus mainly on understanding the biomineralization process, through the study of the effect of sericin in HAp nucleation. Other studies dedicate to the synthesis of these biomaterials for the substitution and regeneration of hard tissues and to the development of new drug delivery systems. Apart from the biomedical field, there are other areas where these composites have a lot of potential, such as in the cosmetic, food an environmental sector.
In the last decade, the number of published papers on sericin/CaP composites has increased substantially. Thus, in the near future, it is clear that the integration of silk sericin in CaP biomaterials will result in advanced composite systems with evident benefits on several research fields, particularly in biomedical and pharmaceutic-related applications.
Author Contributions
All authors contributed to the analysis and writing of the manuscript, and approved it for publication.
Funding
This work was financially supported by: Base Funding – UIDB/00511/2020 of the Laboratory for Process Engineering, Environment, Biotechnology and Energy – LEPABE – funded by national funds through the FCT/MCTES (PIDDAC). The authors also acknowledge Portuguese National Funds from FCT—Fundação para a Ciência e a Tecnologia through project UID/Multi/04044/2019. This work was supported by project Biotherapies-Bioengineered Therapies for Infectious Diseases and Tissue Regeneration and Interreg V-A POCTEP Programme through FEDER funds from the European Union [0245_IBEROS_1_E] C. A.
Conflict of Interest
The authors declare that the research was conducted in the absence of any commercial or financial relationships that could be construed as a potential conflict of interest.
Acknowledgments
The authors also extend their deep appreciation to Department of Chemical Engineering at Faculty of Engineering of the University of Porto, and Centre for Biotechnology and Fine Chemistry at School of Biotechnology of the Portuguese Catholic University.
References
Ahsan, F., Ansari, T., Usmani, S., and Bagga, P. (2018). An insight on silk protein sericin: from processing to biomedical application. Drug Res. 68, 317–327. doi: 10.1055/s-0043-121464.
Altman, G., Diaz, F., Jakuba, C., Calabro, T., Horan, R. L., Chen, T., et al. (2003). Silk-based biomaterials. Biomaterials 24, 401–416. doi: 10.1016/S0142-9612(02)00353-8
Amini, A., Laurencin, C., and Nukavarapu, S. (2012). Bone tissue engineering: recent advances and challenges. Crit. Rev. Biomed. Eng. 40, 363–408. doi: 10.1615/CritRevBiomedEng.v40.i5.10.
Aramwit, P. (2014). “Bio-response to silk sericin,” in Silk Biomaterials for Tissue Engineering and Regenerative Medicine, ed S. C. Kundu (England, Woodhead Publishing; Elsevier), 299–329. doi: 10.1533/9780857097064.2.299
Aramwit, P., Kanokpanont, S., De-Eknamkul, W., and Srichana, T. (2009). Monitoring of inflammatory mediators induced by silk sericin. J. Biosci. Bioeng. 107, 556–561. doi: 10.1016/j.jbiosc.2008.12.012.
Aramwit, P., Kanokpanont, S., Nakpheng, T., and Srichana, T. (2010). The effect of sericin from various extraction methods on cell viability and collagen production. Int. J. Mol. Sci. 11, 2200–2211. doi: 10.3390/ijms11052200.
Aramwit, P., Keongamaroon, O., Siritientong, T., Bang, N., and Supasyndh, O. (2012a). Sericin cream reduces pruritus in hemodialysis patients: a randomized, double-blind, placebo-controlled experimental study. BMC Nephrol. 13:119. doi: 10.1186/1471-2369-13-119.
Aramwit, P., Palapinyo, S., Srichana, T., Chottanapund, S., and Muangman, P. (2013). Silk sericin ameliorates wound healing and its clinical efficacy in burn wounds. Arch. Dermatol. Res. 305, 585–594. doi: 10.1007/s00403-013-1371-4.
Aramwit, P., Siritientong, T., and Srichana, T. (2012b). Potential applications of silk sericin, a natural protein from textile industry by-products. Waste Manage. Res. 30, 217–224. doi: 10.1177/0734242X11404733.
Bell, S. (2012). Therapeutic Calcium Phosphate Particles in Use for Aesthetic of Cosmetic Medicine, and Methods of Manufacture and Use. U.S. Patent No 2012/0207803. Washington, DC: U.S. Patent and Trademark Office.
Bhowmick, S., Scharnweber, D., and Koul, V. (2016). Co-cultivation of keratinocyte-human mesenchymal stem cell (HMSC) on sericin loaded electrospun nanofibrous composite scaffold (cationic gelatin/hyaluronan/chondroitin sulfate) stimulates epithelial differentiation in HMSCs: in vitro study. Biomaterials 88, 83–96. doi: 10.1016/j.biomaterials.2016.02.034.
Bleek, K., and Taubert, A. (2013). New developments in polymer-controlled, bioinspired calcium phosphate mineralization from aqueous solution. Acta Biomater. 9, 6283–6321. doi: 10.1016/j.actbio.2012.12.027.
Bohner, M. (2000). Calcium orthophosphates in medicine: from ceramics to calcium phosphate cements. Int. J. Care Injured. 31:D37–D47. doi: 10.1016/S0020-1383(00)80022-4.
Boyle, L. (2018). “Applications of de novo designed peptides,” in Peptide Applications in Biomedicine, Biotechnology and Bioengineering, ed S. Koutsopoulos (England: Woodhead Publishing; Elsevier), 51–86. doi: 10.1016/B978-0-08-100736-5.00003-X
Brown, C., and Barker, T. (2014). Fibrin-based biomaterials: modulation of macroscopic properties through rational design at the molecular level. Acta Biomater. 10, 1502–1514. doi: 10.1016/j.actbio.2013.09.008.
Cai, Y., Jin, J., Mei, D., Xia, N., and Yao, J. (2009). Effect of silk sericin on assembly of hydroxyapatite nanocrystals into enamel prism-like structure. J. Mater. Chem. 19:5751. doi: 10.1039/b901620a.
Cai, Y., Mei, D., Jiang, T., and Yao, J. (2010). Synthesis of oriented hydroxyapatite crystals: effect of reaction conditions in the presence or absence of silk sericin. Mater. Lett. 64, 2676–2678. doi: 10.1016/j.matlet.2010.08.071
Cai, Y., and Yao, J. (2010). Effect of proteins on the synthesis and assembly of calcium phosphate nanomaterials. Nanoscale 2:1842. doi: 10.1039/c0nr00092b
Campana, V., Milano, G., Pagano, E., Barba, M., Cicione, C., Salonna, G., et al. (2014). Bone substitutes in orthopaedic surgery: from basic science to clinical practice. J. Mater. Sci. 25, 2445–2461. doi: 10.1007/s10856-014-5240-2
Canillas, M., Pena, P., de Aza, A. H., and Rodríguez, M. A. (2017). Calcium phosphates for biomedical applications. Boletín de La Sociedad Española de Cerámica y Vidrio 56, 91–112. doi: 10.1016/j.bsecv.2017.05.001
Cardoso, D., Beucken, J., Both, L., Bender, J., Jansen, J., and Leeuwenburgh, S. C. (2014). Gelation and biocompatibility of injectable alginate-calcium phosphate gels for bone regeneration. J. Biomed. Mater. Res. Part A 102, 808–817. doi: 10.1002/jbm.a.34754.
Chazono, M., Tanaka, T., Komaki, H., and Fujii, K. (2004). Bone formation and bioresorption after implantation of injectable β-tricalcium phosphate granules-hyaluronate complex in rabbit bone defects. J. Biomed. Mater. Res. 70A, 542–549. doi: 10.1002/jbm.a.30094
Chen, L., Hu, J., Ran, J., Shen, C., and Tong, H. (2015). A novel nanocomposite for bone tissue engineering based on chitosan-silk sericin/hydroxyapatite: biomimetic synthesis and its cytocompatibility. RSC Adv. 5, 56410–56422. doi: 10.1039/C5RA08216A
Chen, L., Hu, J., Ran, J., Shen, X., and Tong, H. (2014). Preparation and evaluation of collagen-silk fibroin/hydroxyapatite nanocomposites for bone tissue engineering. Int. J. Biol. Macromol. 65, 1–7. doi: 10.1016/j.ijbiomac.2014.01.003
Chen, Y., Feng, Y., Deveaux, J., Masoud, M., Chandra, F., Chen, H., et al. (2019). Biomineralization forming process and bio-inspired nanomaterials for biomedical application: a review. Minerals 9:68. doi: 10.3390/min9020068
Chirila, T., Suzuki, S., Bray, L., Barnett, N., and Harkin, D. (2013). Evaluation of silk sericin as a biomaterial: in vitro growth of human corneal limbal epithelial cells on Bombyx mori sericin membranes. Progr. Biomater. 2:14. doi: 10.1186/2194-0517-2-14
Chlapanidas, C., Faragò, S., Lucconi, G., Perteghella, S., Galuzzi, M., Mantelli, M., et al. (2013). Sericins exhibit ROS-scavenging, anti-tyrosinase, anti-elastase, and in vitro immunomodulatory activities. Int. J. Biol. Macromol. 58, 47–56. doi: 10.1016/j.ijbiomac.2013.03.054
Dash, R., Mandal, M., Ghosh, S., and Kundu, S. C. (2008). Silk sericin protein of tropical tasar silkworm inhibits UVB-induced apoptosis in human skin keratinocytes. Mol. Cell. Biochem. 311, 111–119. doi: 10.1007/s11010-008-9702-z
de Araujo, T. S., Souza, S. O., and Sousa, E. M. B. (2010). Effect of Zn 2+, Fe 3+ and Cr 3+ addition to hydroxyapatite for its application as an active constituent of sunscreens. J. Phys. 249:012012. doi: 10.1088/1742-6596/249/1/012012.
Dhert, W. J. A., Klein, C. P. A. T., Jansen, J. A., Velde, E. A., Vriesde, R. C., Rozing, P. M., et al. (1993). A histological and histomorphometrical investigation of fluorapatite, magnesiumwhitlockite, and hydroxylapatite plasma-sprayed coatings in goats. J. Biomed. Mater. Res. 27, 127–138. doi: 10.1002/jbm.820270116
Dias, G., Mahoney, P., Swain, M., Kelly, R., Smith, R., and Ali, M. A. (2010). Keratin-hydroxyapatite composites: biocompatibility, osseointegration, and physical properties in an ovine model. J. Biomed. Mater. Res. 95, 1084–1095. doi: 10.1002/jbm.a.32908.
Eliaz, N., and Metoki, N. (2017). Calcium phosphate bioceramics: a review of their history, structure, properties, coating technologies and biomedical applications. Materials 10:334. doi: 10.3390/ma10040334
Farè, S., Torricelli, P., Giavaresi, G., Bertoldi, S., Alessandrino, A., Villa, T., et al. (2013). In vitro study on silk fibroin textile structure for anterior cruciate ligament regeneration. Mater. Sci. Eng. C 33, 3601–3608. doi: 10.1016/j.msec.2013.04.027
Farokhi, M., Mottaghitalab, F., Samani, S., Shokrgozar, M., Kundu, S. C., Reis, R. L., et al. (2018). Silk fibroin/hydroxyapatite composites for bone tissue engineering. Biotechnol. Adv. 36, 68–91. doi: 10.1016/j.biotechadv.2017.10.001
Fuchs, K., Thompson, W., and Warden, S. (1997). “Bone biology,” in Bone Repair Biomaterials, Vol. 11, eds K. M. Pawelec and J. A. Planell (England: Woodhead Publishing; Elsevier), 1–22. doi: 10.1016/S0950-351X(97)80473-9
George, A., and Veis, A. (2008). Phosphorylated proteins and control over apatite nucleation, crystal growth, and inhibition. Chem. Rev. 108, 4670–4693. doi: 10.1021/cr0782729
Griffanti, G., Jiang, W., and Nazhat, S. (2019). Bioinspired mineralization of a functionalized injectable dense collagen hydrogel through silk sericin incorporation. Biomater. Sci. 7, 1064–1077. doi: 10.1039/C8BM01060A
Gsib, O., Egles, C., and Bencherif, S. (2017). Fibrin: an underrated biopolymer for skin tissue engineering. J. Mol. Biol. Biotechnol. 2, 1–4.
Gulrajani, M. L., Agarwal, R., and Chand, S. (2000). Degumming of silk with a fungal protease. Indian J. Fibre Text. Res. 25, 138–142.
Gupta, N., Spiegel, O. L., and Spiegel, J. H. (2019). “RadiesseTM calcium hydroxylapatite injectable filler,” in Neurotoxins and Fillers in Facial Esthetic Surgery (Hoboken, NJ: John Wiley & Sons, Inc.), 71–74. doi: 10.1002/9781119294306.ch5
Ha, T., Quan, T., Vu, D., and Si, D. (2013). “Naturally derived biomaterials: preparation and application,” in Regenerative Medicine and Tissue Engineering, ed J. Andrades (Rijeka: InTech), 247–269. doi: 10.5772/55668
Habraken, W., Habibovic, P., Epple, M., and Bohner, M. (2016). Calcium phosphates in biomedical applications: materials for the future? Mater. Today 19, 69–87. doi: 10.1016/j.mattod.2015.10.008
Holland, C., Numata, K., Rnjak-Kovacina, J., and Seib, F. (2019). The biomedical use of silk: past, present, future. Adv. Healthc. Mater. 8:1800465. doi: 10.1002/adhm.201800465
Jang, J., Lee, E., Kwon, G., and Seo, Y. (2019). The effect of coated nano-hydroxyapatite concentration on scaffolds for osteogenesis. J. Biomater. Appl. 34, 827–839. doi: 10.1177/0885328219875275
Jia, F., Liu, X., Li, L., Mallapragada, S., Narasimhan, B., and Wang, Q. (2013). Multifunctional nanoparticles for targeted delivery of immune activating and cancer therapeutic agents. J. Control. Rel. 172, 1020–1034. doi: 10.1016/j.jconrel.2013.10.012
Jones, J. (2013). “Scaffolds for Tissue Engineering,” in Biodegradable Polymer-Based Scaffolds for Bone Tissue Engineering, ed N. Sultana (Berlin; Heidelberg; Germany: Springer-Verlag; SpringerBriefs in Applied Sciences and Technology), 201–213. doi: 10.1007/978-3-642-34802-0_1
Karahaliloglu, Z., Kilicay, E., and Denkbas, E. (2017). Antibacterial chitosan/silk sericin 3D porous scaffolds as a wound dressing material. Artif. Cells Nanomed. Biotechnol. 45, 1172–1185. doi: 10.1080/21691401.2016.1203796
Kasoju, N., and Bora, U. (2012). Silk fibroin in tissue engineering. Adv. Healthc. Mater. 1, 393–412. doi: 10.1002/adhm.201200097
Kawai, K., Larson, B., Ishise, H., Carre, A., Nishimoto, S., Longaker, M., et al. (2011). Calcium-based nanoparticles accelerate skin wound healing. PLoS ONE 6:e27106. doi: 10.1371/journal.pone.0027106
Khosa, M., Shah, S., and Feng, X. (2014). Metal sericin complexation and ultrafiltration of heavy metals from aqueous solution. Chem. Eng. J. 244, 446–456. doi: 10.1016/j.cej.2014.01.091
Klein, C. P., Patka, P., van der Lubbe, H. B., Wolke, J. G., and de Groot, K. (1991). Plasma-sprayed coatings of tetracalciumphosphate, hydroxyl-apatite, and β-TCP on titanium alloy: an interface study. J. Biomed. Mater. Res. 25, 53–65. doi: 10.1002/jbm.820250105
Koley, P., Sakurai, M., Takei, T., and Aono, M. (2016). Facile fabrication of silk protein sericin-mediated hierarchical hydroxyapatite-based bio-hybrid architectures: excellent adsorption of toxic heavy metals and hazardous dye from wastewater. RSC Adv. 6, 86607–86616. doi: 10.1039/C6RA12818A
Kumar, J., Alam, S., Jain, A., Ansari, K., and Mandal, B. (2018). Protective activity of silk sericin against UV radiation-induced skin damage by downregulating oxidative stress. ACS Appl. Biomater. 1, 2120–2132. doi: 10.1021/acsabm.8b00558
Kumar, P., and Mandal, B. (2017). Antioxidant potential of mulberry and non-mulberry silk sericin and its implications in biomedicine. Free Radic. Biol. Med. 108, 803–818. doi: 10.1016/j.freeradbiomed.2017.05.002
Kumar, P., and Mandal, B. (2019). The inhibitory effect of silk sericin against ultraviolet-induced melanogenesis and its potential use in cosmeceutics as an anti-hyperpigmentation compound. Photochem. Photobiol. Sci. 18, 2497–2508. doi: 10.1039/C9PP00059C
Kunz, R., Brancalhão, R., Ribeiro, L., and Natali, M. (2016). Silkworm sericin: properties and biomedical applications. Biomed Res. Int. 2016:8175701. doi: 10.1155/2016/8175701
Lamboni, L., Gauthier, M., Yang, G., and Wang, Q. (2015). Silk sericin: a versatile material for tissue engineering and drug delivery. Biotechnol. Adv. 33, 1855–1867. doi: 10.1016/j.biotechadv.2015.10.014
Lansdown, B. G. (2002). Calcium: a potential central regulator in wound healing in the skin. Wound Rep. Regener. 10, 271–285. doi: 10.1046/j.1524-475X.2002.10502.x
Lee, Y., and Yuk, S. (2007). Polymeric protein delivery systems. Prog. Polym. Sci. 32, 669–697. doi: 10.1016/j.progpolymsci.2007.04.001
Li, W., Cai, Y., Zhong, Q., Yang, Y., Kundu, C., and Yao, J. (2016). Silk sericin microcapsules with hydroxyapatite shells: protection and modification of organic microcapsules by biomimetic mineralization. J. Mater. Chem. B 4, 340–347. doi: 10.1039/C5TB02328A
Liu, C. (2015). “Hydroxyapatite (HAp) for biomedical applications,” in Structure and Properties of Hydroxyapatite for Biomedical Applications, ed M. Mucalo (England: Woodhead Publishing; Elsevier), 3–19. doi: 10.1016/B978-1-78242-033-0.00010-9
Liu, J., Lawrence, B., Liu, A., Schwab, I., Oliveira, L., and Rosenblatt, M. I. (2012). Silk fibroin as a biomaterial substrate for corneal epithelial cell sheet generation. Invest. Opthalmol. Vis. Sci. 53:4130. doi: 10.1167/iovs.12-9876
Lopes, B., Dias, M., Silva, V., Santos, P., Monteiro, F., and Gomes, P. (2009). Production Method for Calcium Phosphate Nano-Particles With High Purity and Their Use. U.S. Patent No 2009/0263497 A1. Washington, DC: U.S. Patent and Trademark Office.
Mondal, M., Trivedy, K., and Kumar, S. (2006). The silk proteins, sericin and fibroin in silkworm, bombyx mori linn., - a review. Caspian J. Environ. Sci. 25, 77–84.
Nayak, S., Dey, T., Naskar, D., and Kundu, S. (2013). The promotion of osseointegration of titanium surfaces by coating with silk protein sericin. Biomaterials 34, 2855–2864. doi: 10.1016/j.biomaterials.2013.01.019
Nayak, S., Talukdar, S., and Kundu, S. (2012). Potential of 2D crosslinked sericin membranes with improved biostability for skin tissue engineering. Cell Tissue Res. 347, 783–794. doi: 10.1007/s00441-011-1269-4
Ogose, A., Hotta, T., Kawashima, H., Kondo, N., Gu, W., Kamura, T., et al. (2005). Comparison of hydroxyapatite and beta tricalcium phosphate as bone substitutes after excision of bone tumors. J. Biomed. Mater. Res. 72B, 94–101. doi: 10.1002/jbm.b.30136
Padamwar, M., Pawar, A., Daithankar, A., and Mahadik, K. R. (2005). Silk sericin as a moisturizer: an in vivo study. J. Cosmet. Dermatol. 4, 250–257. doi: 10.1111/j.1473-2165.2005.00200.x
Padamwar, M. N., and Pawar, A. P. (2004). Silk sericin and its application : a review. J. Sci. Ind. Res. 63, 323–329.
Pighinelli, L., and Kucharska, M. (2013). Chitosan-hydroxyapatite composites. Carbohydr. Polym. 93, 256–262. doi: 10.1016/j.carbpol.2012.06.004
Prasong, S. (2011). Screening of antioxidant activity of some samia ricini (eri) silks: comparison with Bombyx mori. J. Biol. Sci. 11, 336–339. doi: 10.3923/jbs.2011.336.339
Ramesan, M. T., Athira, V. K., Jayakrishnan, P., and Gopinathan, C. (2016). Preparation, characterization, electrical and antibacterial properties of sericin/poly(vinyl alcohol)/poly(vinyl pyrrolidone) composites. J. Appl. Polym. Sci. 43535. doi: 10.1002/app.43535
Rezwan, K., Chen, Q. Z., Blaker, J. J., and Boccaccini, A. (2006). Biodegradable and bioactive porous polymer/inorganic composite scaffolds for bone tissue engineering. Biomaterials 27, 3413–3431. doi: 10.1016/j.biomaterials.2006.01.039
Ritz, E., Hahn, K., Ketteler, M., Kuhlmann, M., and Mann, J. (2012). Phosphate additives in food. Deutsches Aerzteblatt Online 109, 49–55. doi: 10.3238/arztebl.2012.0049
Salama, A. (2019). Cellulose/Calcium phosphate hybrids: new materials for biomedical and environmental applications. Int. J. Biol. Macromol. 127, 606–617. doi: 10.1016/j.ijbiomac.2019.01.130.
Sasaki, M., Yamada, H., and Kato, N. (2000a). A resistant protein, sericin improves atropine-induced constipation in rats. Food Sci. Technol. Res. 6, 280–283. doi: 10.3136/fstr.6.280
Sasaki, M., Yamada, H., and Kato, N. (2000b). Consumption of silk protein, sericin elevates intestinal absorption of zinc, iron, magnesium and calcium in rats. Nutr. Res. 20, 1505–1511. doi: 10.1016/S0271-5317(00)80031-7
Saxena, T., Karumbaiah, L., and Valmikinathan, C. (2014). “Proteins and poly(amino acids),” in Natural and Synthetic Biomedical Polymers, eds S. Kumbar and M. Deng (Netherlands: Elsevier Science; ScienceDirect), 43–65. doi: 10.1016/B978-0-12-396983-5.00003-X
Shavandi, A., Silva, T.i., Bekhit, A., and Bekhit, A. (2017). Keratin: dissolution, extraction and biomedical application. Biomater. Sci. 5, 1699–1735. doi: 10.1039/c7bm00411g
Sherman, R. (2007). Dietary phosphate restriction and protein intake in dialysis patients: a misdirected focus. Semin. Dial. 20, 16–18. doi: 10.1111/j.1525-139X.2007.00204.x
Soong, K., and Kenyon, K. (1984). Adverse reactions to virgin silk sutures in cataract surgery. Ophthalmology 91, 479–483. doi: 10.1016/S0161-6420(84)34273-7
Subburaman, K., Pernodet, N., Kwak, S. Y., DiMasi, E., Ge, S., Zaitsev, V., et al. (2006). Templated biomineralization on self-assembled protein fibers. Proc. Natl. Acad. Sci. U.S.A. 103, 14672–14677. doi: 10.1073/pnas.0602952103
Sukjai, O., Asanithi, P., Limsuwan, S., and Limsuwan, P. (2012). Growth of hydroxyapatite on sericin coated and non- sericin coated silk fibers using simulated body fluid. Int. J. Sci. Res. Publ. 3, 69–72. doi: 10.1016/j.proeng.2012.02.059
Swetha, M., Sahithi, K, Moorthi, A., Srinivasan, N., Ramasamy, K., and Selvamurugan, N. (2010). Biocomposites containing natural polymers and hydroxyapatite for bone tissue engineering. Int. J. Biol. Macromol. 47, 1–4. doi: 10.1016/j.ijbiomac.2010.03.015
Takeuchi, A., Ohtsuki, C., Kamitakahara, M., Ogata, S., Tanihara, M., Miyazaki, T., et al. (2003a). Apatite deposition on silk sericin in a solution mimicking extracellular fluid: effects of fabrication process of sericin film. Key Eng. Mater. 254–256, 403–406. doi: 10.4028/www.scientific.net/KEM.254-256.403
Takeuchi, A., Ohtsuki, C., Kamitakahara, M., Ogata, S., Tanihara, M., Miyazaki, T., et al. (2005a). Biodegradation of porous alpha-tricalcium phosphate coated with silk sericin. Key Eng. Mater. 284–286, 329–32. doi: 10.4028/www.scientific.net/KEM.284-286.329
Takeuchi, A., Ohtsuki, C., Miyazaki, T., Kamitakahara, M., Ogata, S., Yamazaki, M., et al. (2005b). Heterogeneous nucleation of hydroxyapatite on protein: structural effect of silk sericin. J. R. Soc. Interface 2, 373–78. doi: 10.1098/rsif.2005.0052
Takeuchi, A., Ohtsuki, C., Miyazaki, T., Tanaka, H., Yamazaki, M., and Tanihara, M. (2003b). Deposition of bone-like apatite on silk fiber in a solution that mimics extracellular fluid. J. Biomed. Mater. Res. 65A, 283–289. doi: 10.1002/jbm.a.10456
Tamimi, F., Le Nihouannen, D., Eimar, H., Sheikh, Z., Komarova, S., and Barralet, J. (2012). The effect of autoclaving on the physical and biological properties of dicalcium phosphate dihydrate bioceramics: brushite vs. monetite. Acta Biomater. 8, 3161–3169. doi: 10.1016/j.actbio.2012.04.025
Tavafoghi, M., and Cerruti, M. (2016). The role of amino acids in hydroxyapatite mineralization. J. R. Soc. Interface 13:20160462. doi: 10.1098/rsif.2016.0462
Terada, S., Sasaki, M., Yanagihara, K., and Yamada, H. (2005). Preparation of silk protein sericin as mitogenic factor for better mammalian cell culture. J. Biosci. Bioeng. 100, 667–671. doi: 10.1263/jbb.100.667
Teramoto, H., and Miyazawa, M. (2003). Analysis of structural properties and formation of sericin fiber by infrared spectroscopy. J. Insect Biotecnol. Sericol. 72, 157–163. doi: 10.11416/jibs.72.157
Tontowi, E., Raharjo, K., Sihaloho, R., and Baroroh, D. (2017). Comparison of design method for making composite of (PMMA/HA/Sericin). Mater. Sci. Forum 901, 85–90. doi: 10.4028/www.scientific.net/MSF.901.85
Ude, A. U., Eshkoor, R. A., Zulkifili, R., Ariffin, A. K., Dzuraidah, A. W., Azhari, C. H., et al. (2014). Bombyx mori silk fibre and its composite: a review of contemporary developments. Mater. Des. 57, 298–305. doi: 10.1016/j.matdes.2013.12.052
Vallet-Regí, M., and González-Calbet, J. (2004). Calcium phosphates as substitution of bone tissues. Progr. Solid State Chem. 32, 1–31. doi: 10.1016/j.progsolidstchem.2004.07.001
Vedakumari, S., Jayalakshmi, W., Sanjayan, C. G., Jayavardhini, B., Arya, K., Murugesan, R., et al. (2019). Fabrication of microcomposites based on silk sericin and monetite for bone tissue engineering. Polym. Bull. 77, 475–481. doi: 10.1007/s00289-019-02754-7
Veiga, A., Castro, F., Reis, C., Sousa, A., Oliveira, A. L., and Rocha, F. (2019). Hydroxyapatite/sericin composites: a simple synthesis route under near-physiological conditions of temperature and pH and preliminary study of the effect of sericin on the niomineralization process. Mater. Sci. Eng. 108:110400. doi: 10.1016/j.msec.2019.110400
Wang, Z., Zhang, Y., Zhang, J., Huang, L., Liu, J., Li, Y., et al. (2015). Exploring natural silk protein sericin for regenerative medicine: an injectable, photoluminescent, cell-adhesive 3D hydrogel. Sci. Rep. 4:7064. doi: 10.1038/srep07064
Yan, P., Oliveira, J., Oliveira, A., and Reis, R. (2013). Silk fibroin/nano-CaP bilayered scaffolds for osteochondral tissue engineering. Key Eng. Mater. 587, 245–248. doi: 10.4028/www.scientific.net/kem.587.245
Yang, M., Mandal, N., Shuai, Y., Zhou, G., Min, S., and Zhu, L. (2014a). Mineralization and biocompatibility of Antheraea pernyi (A. pernyi) silk sericin film for potential bone tissue engineering. Biomed. Mater. Eng. 24, 815–824. doi: 10.3233/BME-130873
Yang, M., Shuai, Y., Zhang, S., Chen, C., Zhu, Y., Mao, C., et al. (2014b). Biomimetic nucleation of hydroxyapatite crystals mediated by antheraea pernyi silk sericin promotes osteogenic differentiation of human bone marrow derived mesenchymal stem cells. Biomacromolecules 15, 1185–1193. doi: 10.1021/bm401740x
Yang, M., Shuai, Y., Zhou, G., Mandal, N., Zhu, L., and Mao, C. (2014c). Tuning molecular weights of Bombyx mori (B. mori) silk sericin to modify its assembly structures and materials formation. ACS Appl. Mater. Interfaces 6, 13782–13789. doi: 10.1021/am503214g
Yang, N., Zhong, Q., Zhou, Y., Kundu, S., Yao, J., and Cai, Y. (2016). Controlled degradation pattern of hydroxyapatite/calcium carbonate composite microspheres. Microsc. Res. Tech. 79, 518–524. doi: 10.1002/jemt.22661
Zhang, H. P., Wang, X. Y., Min, S. J., Mandal, M., Yang, M. Y., and Zhu, L. J. (2014). Hydroxyapatite/sericin composite film prepared through mineralization of flexible ethanol-treated sericin film with simulated body fluids. Ceram. Int. 40, 985–991. doi: 10.1016/j.ceramint.2013.06.095
Zhang, Y., Liu, J., Huang, L., Wang, Z., and Wang, L. (2015). Design and performance of a sericin-alginate interpenetrating network hydrogel for cell and drug delivery. Sci. Rep. 5:12374. doi: 10.1038/srep12374
Zhao, R., Han, H., Ding, S., Li, Z., and Kong, X. (2013). Effect of silk sericin on morphology and structure of calcium carbonate crystal. Front. Mater. Sci. 7, 177–183. doi: 10.1007/s11706-013-0202-z
Zhao, R., Ren, X., Xie, C., and Kong, X. (2017). Towards understanding the distribution and tumor targeting of sericin regulated spherical calcium phosphate nanoparticles. Microsc. Res. Tech. 80, 321–330. doi: 10.1002/jemt.22800
Zhaorigetu, S., Yanaka, N, Sasaki, M., Hiromitsu, W., and Kato, N. (2003). Inhibitory effects of silk protein, sericin on UVB-induced acute damage and tumor promotion by reducing oxidative stress in the skin of hairless mouse. J. Photochem. Photobiol. B Biol. 71, 11–17. doi: 10.1016/S1011-1344(03)00092-7
Keywords: biomaterials, bone tissue engineering (bone-TE), calcium phosphates (CaPs), drug delivery, hydroxyapatite (HAp), sericin
Citation: Veiga A, Castro F, Rocha F and Oliveira AL (2020) Recent Advances in Silk Sericin/Calcium Phosphate Biomaterials. Front. Mater. 7:24. doi: 10.3389/fmats.2020.00024
Received: 19 September 2019; Accepted: 21 January 2020;
Published: 18 February 2020.
Edited by:
Antonella Motta, University of Trento, ItalyReviewed by:
Vamsi Yadavalli, Virginia Commonwealth University, United StatesBiman B. Mandal, Indian Institute of Technology Guwahati, India
Pornanong Aramwit, Chulalongkorn University, Thailand
Copyright © 2020 Veiga, Castro, Rocha and Oliveira. This is an open-access article distributed under the terms of the Creative Commons Attribution License (CC BY). The use, distribution or reproduction in other forums is permitted, provided the original author(s) and the copyright owner(s) are credited and that the original publication in this journal is cited, in accordance with accepted academic practice. No use, distribution or reproduction is permitted which does not comply with these terms.
*Correspondence: Filipa Castro, ZmlsaXBhaiYjeDAwMDQwO2ZlLnVwLnB0; Ana L. Oliveira, YWxvbGl2ZWlyYSYjeDAwMDQwO3BvcnRvLnVjcC5wdA==