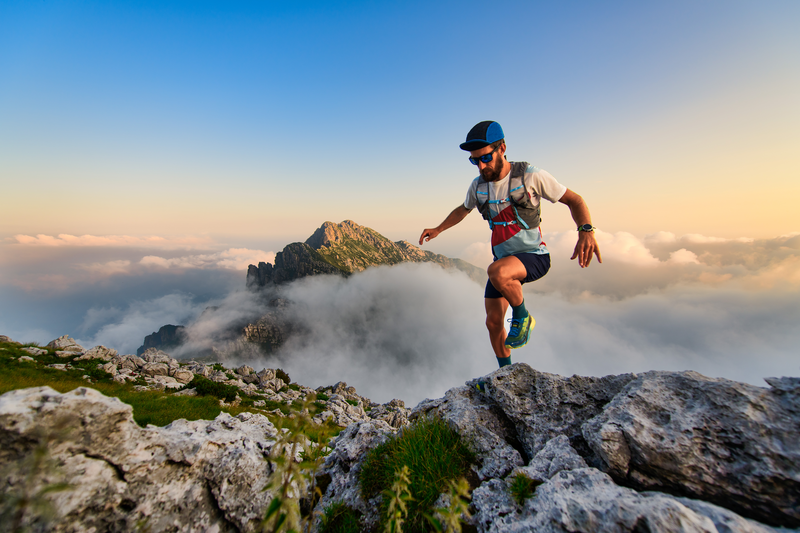
95% of researchers rate our articles as excellent or good
Learn more about the work of our research integrity team to safeguard the quality of each article we publish.
Find out more
ORIGINAL RESEARCH article
Front. Mater. , 08 January 2020
Sec. Ceramics and Glass
Volume 6 - 2019 | https://doi.org/10.3389/fmats.2019.00330
This article is part of the Research Topic Advanced Electroceramics for Energy Conversion and Storage View all 9 articles
Metal halide perovskite solar cells based on all-inorganic CsPbBr3 have attracted considerable attentions recently, due to their high open-circuit voltage and good stability. However, the fabrication of CsPbBr3 film is limited by the poor solubility of cesium precursors in organic solvents by the one-step method. Here, we successfully fabricated CsPbBr3 film solar cells by employing colloid nanocrystal. The effects of technique parameters, including purification times, anneal temperatures, and spin-coating times on film morphology, optical spectra, and device performance are investigated in detail. The highest power conversion efficiency of 4.57% has been achieved based on a large open-circuit voltage of 1.45 V and a large short-circuit current of 9.41 mA cm−2. A large open-circuit voltage results from the reduced non-radiative energy loss channels and defect states while a large short-circuit current is related to the high conductivity induced by the removal of organic ligands with the increased nanocrystal electronic coupling. Furthermore, excellent stability in air is disclosed on the unencapsulated device suggesting the enormous potential for developing high open-circuit photovoltaic devices with high stability in future.
The past few years have witnessed the significant development of organic-inorganic halide perovskite photovoltaics. The highest certified power conversion efficiency (PCE) of perovskite solar cells (PSCs) has reached up to 25.2% until now (NREL, 2019), which is close to that of the commercial polycrystalline silicon arising from high charge carrier mobility, long carrier diffusion length, and high light absorptivity across whole visible region (Green et al., 2014; Huang et al., 2017; Yang et al., 2018). Unfortunately, organic-inorganic halide perovskites suffer from intrinsicly thermal instability and easy-degradation under oxygen and water, which could be overcome by employing the all-inorganic halide perovskite CsPbX3 (X=Cl, Br, I) without volatile organic components (Cho et al., 2018). It is worth mentioning that the stability of perovskite solar cells is determined by the stability of perovskite materials to some extent, so the performance degradation is unavoidable using unstable perovskite composition. Bulk CsPbI3 with cubic structure has a small bandgap of 1.73 eV, which is ideal sunlight absorbing material. However, it undergoes immediate phase transition from cubic to orthorhombic phase when exposed to ambient condition which is due to the orthorhombic yellow phase is thermodynamically preferred (Swarnkar et al., 2016; Sanehira et al., 2017; Zhang et al., 2017; Li B. et al., 2018; Wang et al., 2019). The large bandgap of CsPbCl3 makes it impossible in photovoltaic applications. CsPbBr3 has a direct gap of 2.36 eV, as well as high phase stability, thermal stability, and photo-stability. Although the overall PCE is limited by a relatively larger bandgap, CsPbBr3 has been targeted as a potential material for stable high-voltage perovskite solar cells (Akkerman et al., 2016; Liang et al., 2016; Hoffman et al., 2017; Duan et al., 2018). More importantly, the all-inorganic PSCs using CsSnBr3 QDs as interfacial decoration produce a maximized PCE of 10.60%, which is the highest reported PCE of CsPbBr3 PSCs to date (Zhao et al., 2019). Compared with PSCs based on pure CsPbBr3 nanocrystals (NCs), ion-doped or mixed PSCs have also attracted increased interests. CsPbBrI2 has reasonable broad bandgap, and stable cubic phase at room temperature, with a continuously improved PCE (Sutton et al., 2016; Niezgoda et al., 2017; Zeng et al., 2018; Zhang J. et al., 2018). It was reported that Cs0.91Rb0.09PbBr3 tailored solar cell achieved a high PCE of 9.86% resulted from the suppressed non-radiative losses and radiative recombination (Li Y. et al., 2018). The CsPbI3−xBrx solar cell reported by Ye et al. achieved an open circuit voltage of 1.25 V and a highest PCE of 18.64% for all-inorganic PSCs. By inserting LiF in SnO2 layer, the passivation of interface defects and optimized band alignement are achieved (Ye et al., 2019).
The fabrication technique has a significant impact on the film morphology and final device performance. Both one-step method and two-step method have been successfully employed to fabricate organic-inorganic halide PSCs with a high PCE exceeding 20% (Jeon et al., 2014; Seok et al., 2018). However, the solution-based deposition methods are difficult to directly adapt for CsPbBr3 because of the low solubility of cesium precursors in commonly used solvents (Kulbak et al., 2015; Chang et al., 2016; Hoffman et al., 2017). Besides, both methods are sensitive to processing conditions because crystal quality, film morphology, and optoelectronic performance are controlled in the same optimization step (Akkerman et al., 2016). Perovskite NCs provide an option to solve the above problems, and the crystal structure, as well as the optical/electrical properties were summarized and discussed in detail (Deng et al., 2019). The perovskite NCs are synthesized at the high temperature to guarantee the excellent crystal quality. The unique luminous properties, including a high photoluminescence quantum yield (PLQY) and a narrow PL emission line width are related to the low defect density and reduced non-radiative recombination process (Song et al., 2015, 2016), which is beneficial to obtain PSC with a high PCE. Moreover, perovskite NCs with a large surface/volume ratio have been demonstrated to restrain the generation of yellow phase and improve air stability (Swarnkar et al., 2016; Cho et al., 2018). Despite these advantages, a series of post-treatment techniques including purification and anneal temperature need to be optimized to fabricate compact and thickness-controllable perovskite NC films by layer-by-layer deposition method.
In this work, the morphology and optical spectra of CsPbBr3 NCs are characterized. The purification and annealing temperature was effectively adjusted to obtain uniform and compact NC films with the purpose of improving conductivity. X-ray diffraction (XRD), PL spectra and transmission spectra were employed to study the influence of purification time on the crystal and the surface property of NC films. The impact of annealing temperature and film thickness on photovoltaic performance were investigated. Finally, the current density-voltage curves under different sweep rates and external quantum efficiency (EQE) were obtained on the device with the highest PCE, and its stability was also evaluated.
CsPbBr3 NCs were synthetized using a hot injection method according to previous recipe. Briefly, Cs-oleate was synthetized by dissolving 0.1 g Cs2CO3 into 0.5 mL oleic acid and 3.7 mL 1-octadecene in a 100 mL round bottom 3-necked flask under N2 flow at 120°C for 30 min. Pb-oleate was synthetized by blending 0.28 g PbBr2, 2 mL oleylamine, 2 mL oleic acid, and 20 mL 1-octadecene in a round bottom flask under the same temperature. Then, 2 mL of Cs-oleate solution was promptly injected in Pb-oleate solution. The reaction flask was immediately transferred in an ice-water bath to complete the growth.
A typical purification procedure was as follows. Firstly, 2 mL hexane and 4 mL ethyl acetate were added into 2 mL CsPbBr3 NCs crude solution. The precipitates were collected via centrifugation with a high-speed of 10,000 rpm for 5 min. Next, they were dispersed in 1 mL hexane and an equal amount of ethyl acetate for the second purification. The added solvent and antisolvent volumes reduced to 0.5 mL for the third purification. Finally, precipitates were dispersed in 0.2 mL mixed solvent hexane/octane (3:1 v/v).
A 100-nm-thick TiO2 layer was firstly deposited on a patterned FTO glass by spin-coating a Tetrabutyl titanate acidic solution at a speed of 3,500 rpm with a follow-on an air-annealing at a temperature of 400°C for 30 min. Then CsPbBr3 NC solution was deposited on the TiO2 layer by spin-coating at 1,500 rpm, and then the NC films were annealed in air at different temperatures for 15 min. The thickness of the film is increased by repeating the above step. Next, a mixed solution of 72.3 mg 2,2′,7,7′-tetrakis-(N,N-di-p-methoxyphenylamine)9,9′-spirobifluorene(Spiro-OMeTAD) in 1 mL chlorobenzene is spin-coated to form the hole-transporting layer at speed of 4,000 rpm for 60 s. At last, an Au electrode with a thick ness of 80 nm was coated by thermal evaporation.
Current density-voltage curves were characterized using a Keithley 2400 source meter under an AM1.5G solar simulator (SS-150, Sciencetech Inc.) in air. The EQE was measured by a certified incident photon-to-current conversion efficiency (IPCE) instrument (Beijing 7-Star Optical Instruments Co., Ltd.). The electrochemical impedance spectroscopy (EIS) was measured by an electrochemical workstation (CHI660D, Shanghai Chenhua Inc.) at a frequency range of 1 Hz−100 kHz and the amplitudes of 0–1.2 V. Microstructure was determined by a scanning electron microscope (SEM, Quanta 250, FEI) and a transmission electron micrograph (TEM, Arm2000F, Japan). Absorption and transmittance spectra were measured by a UV-Visible spectrophotometer (V-570, Jasco). PL spectra were carried out by a setup (Gilden Photonic Sens-9000) with a xenon lamp as the excitation source. XRD patterns were obtained by an X-ray diffraction spectrometer (D/max-2400, Rigaku, Japan).
The TEM images of CsPbBr3 NCs are shown in Figure 1A. Uniform nanocubes are clearly observed with an interplanar spacing of 0.58 nm, which is consistent with the (200) plane in cubic phase (Protesescu et al., 2015; Zhang M. et al., 2018). Figure 1B shows a narrow size distribution with an average size of 19.47 nm for CsPbBr3 NCs, which is in accordance with the narrow PL full width at the half maximum (FWHM) of 110 meV in Figure 1C. CsPbBr3 has a Bohr exciton diameter of 7 nm and an exciton binding energy of 40 meV, which are responsible for a sharp excitonic absorption peak at 511 nm showed in Figure 1C. The PL peak at 525 nm indicates that no obvious quantum size effect is observed because the size of NCs is larger than Bohr exciton diameter, and a small Stokes shift of about 70 meV is obtained. CsPbBr3 has a direct bandgap and the determined bandgap from the Tauc plot in Figure 1D is 2.30 eV, which is larger than that of bulk (2.25 eV) but a little smaller than that of nanosheet (2.32 eV) (Stoumpos et al., 2013; Song et al., 2016).
Figure 1. (A) TEM images of CsPbBr3 NCs, the inset is HRTEM images, (B) size distribution histogram, 100 particles were counted. (C) Optical absorption and PL spectra of CsPbBr3 NCs solution, the inset is the photograph of NCs solution under UV excitation. (D) Tauc plot curve obtained from optical absorbance spectra.
The colloid CsPbBr3 perovskite NCs are stably dispersed in non-polar solvents because of the hydrophobic oleic acid and oleylamine ligands. In order to purify NCs, polar solvents are added as antisolvents to achieve centrifugation separation. Unfortunately, perovskite NCs are unstable (prone to dissolution) in polar solvents, resulting from their intrinsic ionic nature. As a result, antisolvent species and amounts, centrifugation speeds, and purification times are optimized to obtain well-dispersed NCs with high concentrations in order to meet the requirement of spin-coating NC films (Kim et al., 2015). Ethyl acetate was demonstrated to have the minimal side effect as an antisolvent (Li et al., 2016), but amounts and purification times are varied from batch to batch. The effects of purification times on the morphology of NC films are shown in Figures 2A–C. A large area of the uncovered substrate is observed for one-time-washed film, and NCs are unclear because of lots of residual organic ligands. Increased film coverage and dark yellow are obvious with the increase of purification times. For three-time-washed film, a high resolution of NCs suggests a high conductivity, and compact film is the prerequisite of reducing leakage current in solar cells. XRD patterns in Figure 2D show CsPbBr3 NCs still maintain cubic phase after different purification times. The PL peak intensity is obviously decreased along with a slight red-shift of PL peak (Figure 2E), indicating an increased NCs electronic coupling led by the removal of organic ligands. Moreover, transmittance spectra are used to verify the film thickness, as given in Figure 2F. With the increase of spin-coating time, the film thickness is found to increase for 3-time-washed film than 1-time-washed film because NC films can be redissolved during the layer-by-layer spin-coating deposition resulting from residual organic species for 1-time-washed NCs.
Figure 2. The effects of purification times on morphology, crystal, and optical spectra of CsPbBr3 NC films. SEM images of (A) 1 time (B) 2 times (C) 3 times washed NC films. The insets are corresponding photographs of NC films with different purification times. (D) XRD, (E) PL spectra, and (F) transmittance spectra of the NC films washed at different times. The inset in (E) is magnified PL spectra to show PL peak shift.
Figures 3A–C exhibits the surface morphology images of three NC films annealed at different temperatures, showing increased CsPbBr3 crystal sizes with increasing anneal temperature. The XRD patterns in Figure 3D show that CsPbBr3 NC maintains the cubic phase even after high-temperature annealing, suggesting no phase change during air-annealing. Figures 3E,F shows anneal temperature-dependent photovoltaic performance, which is mainly caused by different JSC. The maximum PCE obtained for NC films annealed at 120°C results from its largest JSC, which is a result of increasing carrier mobility due to decreased amount of grain boundary, in accordance with Zeng et al.'s results (Zeng et al., 2018). Although temperature annealing is beneficial to increase grain size and improve carrier mobility, the JSC decreases with increasing temperature to 150°C. It is strange that increased grain size does not contribute to an improved conductivity. It may result from that the partial oxidization of surface can improve the contact resistance of grains. Therefore, an inert atmosphere annealing may be helpful to improve conductivity under the high-temperature annealing.
Figure 3. The effects of annealing temperature on morphology and crystal of CsPbBr3 NC films and solar cell performance. SEM images of (A) as-prepared (B) 90°C and (C) 180°C. (D) XRD patterns, (E) current density-voltage curves of NC film solar cells under AM 1.5 illumination, (F) the relationship between PCE and anneal temperatures.
Another parameter spin-coating time has a significant effect on NC film thickness and final solar cell performance. The photovoltaic performance of CsPbBr3 NC films is shown in Figure 4A. Both monotonically increased Voc and Jsc are found with the increasing spin-coating times. The device using 1-spin-coated NC film failed because low film coverage leads to current leakage. The Voc of 1.45 V for 7-spin-coated NC film is quite close to the highest report of 1.5 V for perovskite solar cells (Akkerman et al., 2016). The Eloss can be described by the equation: Eloss = Eg – eVOC, and a small Eloss of 0.85 eV is obtained, resulting from the reduced non-radiative energy loss channels and defect states (Zeng et al., 2018). The Jsc of 4.5 mA cm−2 is not high enough compared with the highest values of 5.6 mA cm−2 for CsPbBr3 NC film solar cell and 7.5 mA cm−2 for CsPbBr3 solar cell by one-step method (Akkerman et al., 2016; Liang et al., 2016), and low Jsc is attributed to low NC film conductivity. The relationship among spin-coating times, film thickness and device performance are summarized in Figure 4B and a linearly increased film thickness are observed. The highest PCE is obtained from 7-spin-coated NC film with a thickness of 600 nm. The increased PCE with increasing spin-coating times is attributed to increased photo-generated excitons separation due to enhanced visible light absorption and reduced current leakage.
Figure 4. (A) Current density-voltage curves of CsPbBr3 NC film solar cells under AM 1.5 illumination, (B) The impact of spin-coating times on film thickness and PCE.
A typical CsPbBr3 NC film solar cell structure is FTO/TiO2/CsPbBr3 NC film/Spiro-OMeTAD/Au, and the thickness of every layer can be seen in Figure 5A. The best-performing solar cell exhibits a high PCE of 4.57% in Figure 5B, and we compare reported all-inorganic perovskite solar cells in Table 1. The smaller JSC resulting from narrow visible light absorption is the reason for lower PCE compared with CsPbI3 and CsPbBrI2 solar cells, but their large bandgap contributes to larger VOC. We can find increased JSC with increased scan rate in Figure 5C, according to previous results. The EQE spectra of the device is shown in Figure 5D, and the cut-off wavelength is about 550 nm resulting from wide bandgap of CsPbBr3, explaining its smaller JSC compared with CsPbI3 and MAPbI3 solar cells (Swarnkar et al., 2016; Sanehira et al., 2017; Zhang et al., 2017; Li B. et al., 2018). The interfacial charge transfer process of device under illumination is revealed by the EIS spectra as given in Figure 5E. It is clearly observed decreased charge transfer resistance (Rct) with the increase of applied bias voltage, which is resulted from the efficient extraction of more carriers. At the same time, the charge recombination resistance (Rr) is decreased by significantly enhancement of the free charge carriers in perovskite layer (Yang et al., 2015). The low Rct and Rr under illumination illustrate the ideal contact interface between perovskite and transport layer. Finally, the unencapsulated device exhibits excellent stability in Figure 5F. It retains around 90% of initial PCE after 40 days of storage in air, and a reduced PCE is mainly caused by decreased JSC, which is possibly attributed to the damage of the interface between perovskite and organic HTL. Therefore, the high air-stability contributes to solidifying CsPbBr3 NC film solar cell as a kind of high-VOC and stable device.
Figure 5. CsPbBr3 NC film photovoltaic device structure and performance. (A) Cross-sectional SEM images of device, (B) the current density-voltage curve of the best device under backward scan, (C) the current density-voltage curves obtained at different scan rate, (D) wavelength-dependent EQE curve. (E) Nyquist plots of device measured at different bias voltages under AM 1.5 illumination. (F) PCE stability of device without encapsulations stored in air.
In summary, we have fabricated planar structure CsPbBr3 NCs solar cells, and the average size of colloid CsPbBr3 NC is 19.47 nm. The effects of purification times, anneal temperature and spin-coating times on morphology and crystal of CsPbBr3 NC films were investigated. The compact and high conductivity NC films were prepared using a 3-time purification NC solution, and an increased NCs electronic coupling resulting from the removal of organic ligands was revealed by a slight red-shift of PL peak. We observed increased CsPbBr3 crystal sizes with increasing annealing temperature, contributing to the increased solar cell PCE. Besides, we also found monotonically increased Voc and Jsc with increasing spin-coating times due to increased film thickness. After the above parameters optimization, the best-performing solar cell with a high PCE of 4.57% was obtained, and the unencapsulated device exhibits great stability exceed 40 daysin air, illustrating the enormous potential of high-VOC CsPbBr3 NC film solar cell.
All datasets generated for this study are included in the article/Supplementary Material.
JL conceived the idea, directed, and supervised the project. LeZ took part in the device fabrication and characterization. TH, LeZ, HL, LiZ, ZL, and GW analyzed the data. All authors contributed to discussions.
We acknowledge the support from National Natural Science Foundation of China (Grant Nos. 51802182, 51972263, 51802142, 51572214, 61604122, and 51372196), China Postdoctoral Science Foundation (Grant No. 2019M660253), and 111 Program (Grant No. B14040). JL thanks the Fundamental Research Funds for the Central Universities (Grant No. sxjh012019011) and the Foundation of Shenzhen Science and Technology Innovation Committee (Grant Nos. JCYJ20180302174439113, JCYJ20180504170444967). The authors declare no competing financial interests.
The authors declare that the research was conducted in the absence of any commercial or financial relationships that could be construed as a potential conflict of interest.
Associate professor Zhi Yang helped prepared the paper. We thank him for his language editing.
The Supplementary Material for this article can be found online at: https://www.frontiersin.org/articles/10.3389/fmats.2019.00330/full#supplementary-material
Data Sheet 1. XRD pattern of CsPbBr3 nanocrystals.
Akkerman, Q. A., Gandini, M., Di Stasio, F., Rastogi, P., Palazon, F., Bertoni, G., et al. (2016). Strongly emissive perovskite nanocrystal inks for high-voltage solar cells. Nat. Energy 2:16194. doi: 10.1038/nenergy.2016.194
Chang, X., Li, W., Zhu, L., Liu, H., Geng, H., Xiang, S., et al. (2016). Carbon-based CsPbBr3 perovskite solar cells: all-ambient processes and high thermal stability. ACS Appl. Mater. Interfaces 8, 33649–33655. doi: 10.1021/acsami.6b11393
Cho, H., Kim, Y. H., Wolf, C., Lee, H. D., and Lee, T. W. (2018). Improving the stability of metal halide perovskite materials and light-emitting diodes. Adv. Mater. 30:1704587. doi: 10.1002/adma.201704587
Deng, J., Li, J., Yang, Z., and Wang, M. (2019). All-inorganic lead halide perovskites: a promising choice for photovoltaics and detectors. J. Mater. Chem. C 7, 12415–12440. doi: 10.1039/C9TC04164H
Duan, J., Zhao, Y., He, B., and Tang, Q. (2018). High-purity inorganic perovskite films for solar cells with 9.72% efficiency. Angew. Chem. 57, 3787–3791. doi: 10.1002/anie.201800019
Green, M. A., Anita, H. B., and Snaith, H. J. (2014). The emergence of perovskite solar cells. Nat. Photonics 8, 506–514. doi: 10.1038/nphoton.2014.134
Hoffman, J. B., Zaiats, G., Wappes, I., and Kamat, P. V. (2017). CsPbBr3 solar cells: controlled film growth through layer-by-layer quantum dot deposition. Chem. Mater. 29, 9767–9774. doi: 10.1021/acs.chemmater.7b03751
Huang, J., Yuan, Y., Shao, Y., and Yan, Y. (2017). Understanding the physical properties of hybrid perovskites for photovoltaic applications. Nat. Rev. Mater. 2:17042. doi: 10.1038/natrevmats.2017.42
Jeon, N. J., Noh, J. H., Kim, Y. C., Yang, W. S., Ryu, S., and Seok, S. I. (2014). Solvent engineering for high-performance inorganic–organic hybrid perovskite solar cells. Nat. Mater. 13, 897–903. doi: 10.1038/nmat4014
Kim, Y., Yassitepe, E., Voznyy, O., Comin, R., Walters, G., Gong, X., et al. (2015). Efficient luminescence from perovskite quantum dot solids. ACS Appl. Mater. Interfaces 7, 25007–250313. doi: 10.1021/acsami.5b09084
Kulbak, M., Cahen, D., and Hodes, G. (2015). How important is the organic part of lead halide perovskite photovoltaic cells? Efficient CsPbBr3 cells. J. Phys. Chem. Lett. 6, 2452–2456. doi: 10.1021/acs.jpclett.5b00968
Li, B., Zhang, Y., Fu, L., Yu, T., Zhou, S., Zhang, L., et al. (2018). Surface passivation engineering strategy to fully-inorganic cubic CsPbI3 perovskites for high-performance solar cells. Nat. Commun. 9:1076. doi: 10.1038/s41467-018-03169-0
Li, J., Xu, L., Wang, T., Song, J., Chen, J., Xue, J., et al. (2016). 50-Fold EQE improvement up to 6.27% of solution-processed all-inorganic perovskite CsPbBr3 QLEDs via surface ligand density control. Adv. Mater. 28:4861. doi: 10.1002/adma.201603885
Li, Y., Duan, J., Yuan, H., Zhao, Y., He, B., and Tang, Q. (2018). Lattice modulation of alkali metal cations doped Cs1−xRxPbBr3 halides for inorganic perovskite solar cells. Sol. RRL. 2:1800164. doi: 10.1002/solr.201800164
Liang, J., Wang, C., Wang, Y., Xu, Z., Lu, Z., Ma, Y., et al. (2016). All-inorganic perovskite solar cells. J. Am. Chem. Soc. 138, 15829–15832. doi: 10.1021/jacs.6b10227
Niezgoda, J. S., Foley, B. J., Chen, A. Z., and Choi, J. J. (2017). Improved charge collection in highlyefficient CsPbBrI2 solar cells with light-induced dealloying. ACS Energy Lett. 2, 1043–1049. doi: 10.1021/acsenergylett.7b00258
NREL (2019). Best Research-Cell Efficiencies. https://www.nrel.gov/pv/assets/pdfs/best-reserch-cell-efficiencies.20190802.pdf.
Protesescu, L., Yakunin, S., Bodnarchuk, M. I., Krieg, F., Caputo, R., Hendon, C. H., et al. (2015). Nanocrystals of cesium lead halide perovskites (CsPbX3, X = Cl, Br, and I): novel optoelectronic materials showing bright emission with wide color gamut. Nano Lett. 15, 3692–3696. doi: 10.1021/nl5048779
Sanehira, E. M., Marshall, A. R., Christians, J. A., Harvey, S. P., Ciesielski, P. N., Wheeler, L. M., et al. (2017). Enhanced mobility CsPbI3 quantum dot arrays for record-efficiency, high-voltage photovoltaic cells. Sci. Adv. 3:4204. doi: 10.1126/sciadv.aao4204
Seok, S. I., Grätzel, M., and Park, N. G. (2018). Methodologies toward highly efficient perovskite solar cells. Small 14:1704177. doi: 10.1002/smll.201704177
Song, J., Li, J., Li, X., Xu, L., Dong, Y., and Zeng, H. (2015). Quantum dot light-emitting diodes based on inorganic perovskite cesium lead halides (CsPbX3). Adv. Mater. 27, 7162–7167. doi: 10.1002/adma.201502567
Song, J., Xu, L., Li, J., Xue, J., Dong, Y., Li, X., et al. (2016). Monolayer and few-layer all-inorganic perovskites as a new family of two-dimensional semiconductors for printable optoelectronic devices. Adv. Mater. 28, 4861–4869. doi: 10.1002/adma.201600225
Stoumpos, C. C., Malliakas, C. D., Peters, J. A., Liu, Z., Sebastian, M., Im, J., et al. (2013). Crystal growth of the perovskite semiconductor CsPbBr3: a new material for high-energy radiation detection. Cryst. Growth Des. 13, 2722–2727. doi: 10.1021/cg400645t
Sutton, R. J., Eperon, G. E., Miranda, L., Parrott, E. S, Kamino, B. A., Patel, J. B., et al. (2016). Bandgap-tunable cesium lead halide perovskites with high thermal stability for efficient solar cells. Adv. Energy Mater. 6:1502458. doi: 10.1002/aenm.201502458
Swarnkar, A., Marshall, A. R., Sanehira, E. M., Chernomordik, B., Moore, D. T., Christians, J. A., et al. (2016). Quantum dot–induced phase stabilization of α-CsPbI3 perovskite for high-efficiency photovoltaics. Science 354, 92–95. doi: 10.1126/science.aag2700
Wang, Y., Dar, M. I., Zhang, T. Y., Kan, M. W., Li, Y., Zhang, L. J., et al. (2019). Thermodynamically stabilized β-CsPbI3-based perovskite solar cells with efficiencies >18%. Science 365, 591–595. doi: 10.1126/science.aav8680
Yang, Z., Wang, M., Ding, J., Sun, Z., Li, L., Huang, J., et al. (2015). Semi-transparentZnO-CuI/CuSCN photodiode detector with narrow-band UV photoresponse. ACS Appl. Mater. Interfaces 7, 21235–24244. doi: 10.1021/acsami.5b05222
Yang, Z., Wang, M., Qiu, H., Yao, X., Lao, X., Xu, S., et al. (2018). Engineering the exciton dissociation in quantum-confined 2D CsPbBr3 nanosheet films. Adv. Funct. Mater. 28:1705908. doi: 10.1002/adfm.201705908
Ye, Q. F., Zhao, Y., Mu, S. Q., Ma, F., Gao, F., Chu, Z. M., et al. (2019). Cesium lead inorganic solar cell with efficiency beyond 18% via reduced charge recombination. Adv. Mater. 31:1905143. doi: 10.1002/adma.201905143
Zeng, Q., Zhang, X., Feng, X., Lu, S., Chen, Z., Yong, X., et al. (2018). Polymer-passivated inorganic cesium lead mixed-halide perovskites for stable and efficient solar cells with high open-circuit voltage over 1.3 V. Adv. Mater. 30:1705393. doi: 10.1002/adma.201705393
Zhang, J., Bai, D., Jin, Z., Bian, H., Wang, K., Sun, J., et al. (2018). 3D-2D-0D interface profiling for record efficiency all-inorganic CsPbBrI2 perovskite solar cells with superior stability. Adv. Energy Mater. 30:1703246. doi: 10.1002/aenm.201703246
Zhang, M., Wang, M., Yang, Z., Li, J., and Qiu, H. (2018). Preparation of all-inorganic perovskite quantum dots-polymer composite for white LEDs application. J. Alloys Compd. 748, 537–545. doi: 10.1016/j.jallcom.2018.03.179
Zhang, T., Dar, M. I., Li, G., Xu, F., Guo, N., Grätzel, M., et al. (2017). Bication lead iodide 2D perovskite component to stabilize inorganic α-CsPbI3 perovskite phase for high-efficiency solar cells. Sci. Adv. 3:1700841. doi: 10.1126/sciadv.1700841
Zhao, Y., Duan, J., Yuan, H., Wang, Y., Yang, X., He, B., and Tang, Q. (2019). Using SnO2 QDs and CsMBr3 (M = Sn, Bi, Cu) QDs as charge-transporting materials for 10.6%-efficiency all-inorganic CsPbBr3 perovskite solar cells with an ultrahigh open-circuit voltage of 1.610 V. Sol. RRL 3:1800284. doi: 10.1002/solr.201800284
Keywords: inorganic perovskite, solar cell, stability, open-circuit voltage, nanocrystals
Citation: Zhang L, Hu T, Li J, Zhang L, Li H, Lu Z and Wang G (2020) All-Inorganic Perovskite Solar Cells With Both High Open-Circuit Voltage and Stability. Front. Mater. 6:330. doi: 10.3389/fmats.2019.00330
Received: 06 November 2019; Accepted: 03 December 2019;
Published: 08 January 2020.
Edited by:
Kalisadhan Mukherjee, Pandit Deendayal Petroleum University, IndiaReviewed by:
Changrong Zhou, Guilin University of Technology, ChinaCopyright © 2020 Zhang, Hu, Li, Zhang, Li, Lu and Wang. This is an open-access article distributed under the terms of the Creative Commons Attribution License (CC BY). The use, distribution or reproduction in other forums is permitted, provided the original author(s) and the copyright owner(s) are credited and that the original publication in this journal is cited, in accordance with accepted academic practice. No use, distribution or reproduction is permitted which does not comply with these terms.
*Correspondence: Jinglei Li, bGlqaW5nbGVpQHhqdHUuZWR1LmNu
Disclaimer: All claims expressed in this article are solely those of the authors and do not necessarily represent those of their affiliated organizations, or those of the publisher, the editors and the reviewers. Any product that may be evaluated in this article or claim that may be made by its manufacturer is not guaranteed or endorsed by the publisher.
Research integrity at Frontiers
Learn more about the work of our research integrity team to safeguard the quality of each article we publish.